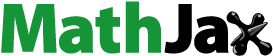
Abstract
Geothermal water contains several important metals, and the separation and recovery of these elements from the geothermal water has attracted interest. However, geothermal water also contains harmful elements, such as boron (B) and arsenic (As), that need to be removed. In the present work, separation of B and pentavalent As(V) from geothermal water was investigated using a glucamine-based chelate fiber as an adsorbent. In single-metal systems, the adsorption of B was high in the neutral pH region, while the adsorption of As(V) was high in the acidic pH region. In a B/As(V) binary system, adsorption of boron at acidic pH was inhibited by the presence of As(V). The adsorption isotherms of the metals in the single-metal systems at three different equilibrium pH values were of the Langmuir type. The adsorption isotherms in the B/As(V) binary system were described by a modified Langmuir model, which indicated that the adsorption was inhibited or promoted by the co-existing metals. Simultaneous removal of the metals from geothermal water was achieved by column adsorption.
1. Introduction
Geothermal water is an attractive form of renewable energy where energy is produced by a geothermal fluid of both vapor and liquid phases (Nasruddin et al. Citation2016). Geothermal water contains several important elements and the separation and recovery of these elements has attracted attention (Sajkowski et al. Citation2023). Among the elements contained in geothermal water, lithium (Li) is one of the attractive metals for separation and recovery (Ighalo et al. Citation2022; Park et al. Citation2012). The geothermal water after power generation is used for heat recovery for binary power generation (Mori Citation2022), and then Li is considered to be recovered by adsorption from the remained geothermal water.
However, geothermal water also contains harmful elements, such as boron (B) and arsenic (As) (Bolan et al. Citation2023; Sajkowski et al. Citation2023). Although B is an essential element for life, the amount required is very low and ingestion of an excess amount of B is a well-known cause of nervous system disease. The World Health Organization currently recommends limiting the concentration of B in drinking water to 2.4 mg/L ("Guidelines for drinking-water quality: fourth edition incorporating the first addendum," Citation2017). Arsenic is one of the most toxic elements and a confirmed carcinogen, and World Health Organization has set the maximum concentration of As in drinking water at 10 μg/L ("Guidelines for drinking-water quality: fourth edition incorporating the first addendum," Citation2017). Japanese Effluent standards requires the maximum permissible levels for B at 10 mg/L and for As at 0.1 mg/L. Removal of these harmful elements is therefore required before re-injection of the geothermal water underground after utilization.
In our previous research, separation of B from geothermal water was investigated using an ion exchange resin or fiber with an N-methyl-d-glucamine group (Recepoğlu et al. Citation2017a; Recepoğlu et al. Citation2017b; Recepoğlu et al. Citation2018). The B in the geothermal water could be removed by an adsorption or adsorption-membrane filtration hybrid process using the ion exchange material. Separation of As from the geothermal water was not investigated in our previous studies, although the As was also adsorbed by the N-methyl-d-glucamine group (Kuraoka et al. Citation2018). Additionally, adsorption of Li with λ-MnO2 was suppressed in the presence of As because of a redox reaction between As and manganese (Mn) in the adsorbent (Park et al. Citation2012). Therefore, removal of the As is also important for effective adsorption of Li from the geothermal water.
In the present work, separation of B and pentavalent As(V) from geothermal water was investigated. An ion exchange fiber (Chelest Fiber GRY-HW) having an N-methyl-d-glucamine group was used as the adsorbent, since the Chelest Fiber GRY-HW was revealed to possess almost same adsorption capacity and higher kinetics than conventional ion exchange resin for the adsorption of B (Nishihama et al. Citation2013). Batchwise adsorption of B and As(V) was first carried out. The effect of pH on the adsorption of B and As(V) and adsorption isotherms of B and As(V) in single and binary systems were investigated to study the interactions of the elements in binary systems. The effect of pH on the adsorption of B from the geothermal water was also investigated. Elution of the B and As(V) was then investigated. Finally, the adsorbent was applied in a column adsorption system to the separation of B and As(V) from the geothermal water.
2. Experimental
2.1. Reagents
Chelest Fiber® GRY-HW was obtained from Chelest Co. (Osaka, Japan) and was conditioned before use by washing 50 g of the adsorbent sequentially with 2 mol/L HCl (250 mL), deionized water (250 mL), 2 mol/L NaOH (250 mL), and deionized water (250 mL). The conditioned adsorbent was dried under vacuum at 25 °C. Na2HAsO4·7H2O was obtained from Alfa Aesar (Lancashire, UK). All other organic and inorganic reagents were purchased from FUJIFILM Wako Pure Chemical Co. (Osaka, Japan) and were of analytical grade.
Aqueous solutions of B and As(V) for the single systems and a B/As(V) solution for the binary system were prepared by dissolving H3BO4 and Na2HAsO4·7H2O in deionized water. To investigate the effect of anions (SO42− and Cl−) on the adsorption of B, aqueous solutions with different pH values were prepared using H3BO4 and Na2SO4/H2SO4 or NaCl/HCl. Geothermal water was obtained from Balcova (Izmir, Turkey) and Kakkonda (Iwate, Japan) and its composition was determined (). Analysis of the As(III) and As(V) contents in the Kakkonda geothermal water revealed that 95.9% of the As was present as As(V). The volume of the Balcova geothermal water sample was not sufficient for our experiments. Consequently, a simulated solution of the Balcova geothermal water was prepared using H3BO4, Na2HAsO4·7H2O, LiCl, NaCl, NaOH, MgSO4, CaCl2, (NH4)2SO4, and NH4CO3. The actual geothermal water sample was used for the Kakkonda geothermal water.
Table 1. Element concentrations (mmol/L) and pH of Balcova and Kakkonda geothermal water samples.
2.2. Batchwise Adsorption
Batchwise adsorption was carried out by shaking 10 mL of the aqueous solution and 20 mg of the adsorbent for 24 h at 25 °C using a thermostatic mechanical shaker. The aqueous solution was then filtered and the concentrations of B and other metal ions were determined using inductively coupled plasma atomic emission spectroscopy (ICP-AES; ICPE-9000, Shimadzu, Kyoto, Japan). The pH was measured by a pH meter (Horiba F-72). The concentrations of sulfate (SO42−) and chloride (Cl−) ions in the aqueous solution were determined using ion chromatography (Prominence, Shimadzu, Japan). The amount of each component (M) adsorbed (qM) was calculated as follows:
(1)
(1)
where [M]feed and [M]eq are initial and equilibrium concentrations of the component (mmol/L), Vaq is the volume of the aqueous solution (L), and w is the mass of the adsorbent (g).
The effect of pH and co-existing ions on adsorption of B were investigated. Additionally, adsorption isotherms were obtained. To investigate the effect of pH on the adsorption of B and As(V) in the single and binary systems, the concentration of each component was fixed at 10 mmol/L and the pH was adjusted by adding an appropriate quantity of HCl or NaOH. Adsorption isotherms of B and As(V) were obtained in both the single and binary systems by varying the concentration of the feed solution but holding the equilibrium pH (pHeq) constant. Aqueous solutions containing the same concentrations of B and As(V) were used to obtain the isotherms in the binary systems. The effects of co-existing components in geothermal water on adsorption of B were also investigated. Simulated geothermal water from Balcova was used as the feed solution, and the pH was adjusted by adding an appropriate volume of HNO3 or NH3 solution. To investigate the effects of SO42− and Cl− as co-existing anions, a feed solution containing 1 mmol/L of B and 15 mmol/L of each co-existing anion was used. The pH was varied by changing the ratio of H2SO4/Na2SO4 or HCl/NaCl.
Elution of B and As(V) from the adsorbent was investigated. Adsorption of B and As(V) was first carried out by shaking 500 mL of the B and As(V) binary solution and 1 g of the adsorbent to obtain the B- and As(V)-loaded adsorbent. The amounts of B and As(V) adsorbed were 0.872 mmol/g and 0.227 mmol/g, respectively. The loaded adsorbent (20 mg) was then eluted with 10 mL of HCl or NaOH at different concentrations with shaking for 4 h at 25 °C. The concentrations of B and As(V) in the eluents were determined by ICP-AES.
2.3. Separation of B and As(V) by Column Adsorption
Separation of B and As(V) from geothermal water was carried out by column adsorption. For the Balcova geothermal water, Chelest Fiber (wet volume: 0.5 mL) was packed in a column (length: 5 cm, inner diameter: 5 mm), and the simulated geothermal water was fed upward at a flow rate of 0.08 mL/min (space velocity = 9.6/h) using a dual-plunger pump (Flom KP-11). After adsorption reached saturation, deionized water was fed into the column to wash out any excess feed solution remaining in the column. Elution was then carried out with 0.9 mol/L H2SO4. For the Kakkonda geothermal water, Chelest Fiber (wet volume: 4.32 mL) was packed in a column (length: 100 mm, inner diameter: 10 mm), and the simulated geothermal water was fed upward at a flow rate of 0.8 mL/min (space velocity= 11.1/h). After adsorption reached saturation, deionized water was fed into the column to wash out excess feed solution remaining in the column. Elution was carried out sequentially with 2.0 mol/L NaOH, deionized water, and 2.0 mol/L HCl. The concentrations of components collected by the fraction collector (EYELA DC-1500) were determined by ICP-AES. The bed volume of the effluent was calculated as follows:
(2)
(2)
where v is flow rate of the solution (mL/min), t is the time the feed solution is supplied for (min), and Vadsorbent is the wet volume of adsorbent in the column (mL).
3. Results and Discussion
3.1. Adsorption Behaviors of B and As(V)
Adsorption behaviors of B and As(V) were investigated. shows the effect of pHeq on the adsorption of B and As(V) in the single systems and B/As(V) binary system. In the single systems, the adsorption of B was high in the neutral pH region (4 < pHeq < 10), while the adsorption of As(V) was high in acidic pH region (pHeq < 6). In the case of B, there are two boron species, B(OH)3 and B(OH)4−, in aqueous solution as shown in EquationEq. 3(3)
(3) (Mesmer et al. Citation1972).
(3)
(3)
Figure 1. Effect of pH on the adsorption of B and As(V) in (a) single systems and (b) B and As(V) in a binary system. (a) [M]feed = 10 mmol/L and (b) [B]feed = [As]feed = 10 mmol/L. The pH was adjusted using HCl/NaOH.
![Figure 1. Effect of pH on the adsorption of B and As(V) in (a) single systems and (b) B and As(V) in a binary system. (a) [M]feed = 10 mmol/L and (b) [B]feed = [As]feed = 10 mmol/L. The pH was adjusted using HCl/NaOH.](/cms/asset/e98d2c06-0c9b-4dac-9ee1-ce1c32b43a35/tjce_a_2297558_f0001_c.jpg)
The complexation of boric acid with the hydroxyl group in the glucamine group liberates proton, and thus the adsorption amount of B in acidic region increases with increasing pH. The decrease in the adsorption amount of B in alkali region is due to the hydrolysis of the boric acid based on EquationEq. 3(3)
(3) (Nishihama et al. Citation2013). In the case of As(V), dissociation of the arsenic acid is shown as EquationEqs. 4–6 (Meng et al. Citation2000).
(4)
(4)
(5)
(5)
(6)
(6)
H2AsO4− and HAsO42− are the predominant species at pH < 6, and the monovalent and divalent anionic species of As was adsorbed with the protonated amine on the adsorbent (Dambies et al. Citation2004).
For the B/As(V) binary system compared with the single systems, adsorption of B in the acidic pH region (2 < pHeq < 6) was inhibited and adsorption of As(V) acidic pH region decreased. The inhibition of the adsorption of B was caused by competitive adsorption of As(V), similar to the effect of anions described in Section 3.2.
Adsorption isotherms for B and As(V) at pHeq 4.4 (pH of the Kakkonda geothermal water), 7, and 8.6 (pH of the Balcova geothermal water) in the single systems were then investigated. All adsorption isotherms () fit well with Langmuir adsorption isotherms, as shown by EquationEq. 7(7)
(7) .
(7)
(7)
where qs is amount adsorbed at saturation (mmol/g), and K is the adsorption constant (L/mmol). The parameters obtained for the adsorption systems are summarized in , and the calculated adsorption isotherms from the parameters are shown in (solid and dotted lines). The amounts of B adsorbed at saturation at the three different pH values were higher than those of As(V). This is consistent with the results shown in . The adsorption constants of B are also higher than those of As(V), which indicates that the N-methyl-d-glucamine group has a higher affinity for B than As(V).
Figure 2. Adsorption isotherms for (a) B and (b) As(V) in single systems. The pH was adjusted using HCl/NaOH. The calculated values of the adsorption isotherms from the Langmuir adsorption model are shown as solid and dotted lines.

Adsorption isotherms of B and As(V) in binary systems were then investigated. shows the adsorption isotherms for B and As(V) at pHeq values of 4.28, 6.95, and 8.64. In the binary systems, the extended Langmuir isotherm (EquationEq. 8(8)
(8) ) could be applied when there was no interaction between the adsorbates (Lee et al. Citation1993; Markham and Benton Citation1931; McKay and Porter Citation1997).
(8)
(8)
The adsorption isotherms of B and As(V) in the binary system were then calculated using the extended Langmuir model with the amounts adsorbed at saturation and adsorption constants determined in the single systems (), although the equilibrium pH was slightly different. The calculated values from the extended Langmuir model are shown in (dotted lines). At pHeq 4.28, the extended Langmuir model indicated that B was selectively adsorbed over As(V); however, the experimental data showed selective adsorption of As(V). At pHeq 6.95 and 8.64, the experimental adsorption of B was higher than that calculated by the extended Langmuir model. These deviations between the experimental results and calculated values could be caused by solute–solute interactions on the adsorbent surface (Lee et al. Citation1993). When the parameters of the extended adsorption isotherm fail to describe the interactions for individual components, a modified Langmuir isotherm (EquationEq. 9(9)
(9) ) that includes additional correlation factors is generally used (Kumar et al. Citation2011).
(9)
(9)
Figure 3. Adsorption isotherms of B and As(V) in binary systems at (a) pHeq = 4.28 ± 0.06, (b) pHeq = 6.95 ± 0.08, and (c) pHeq = 8.64 ± 0.06. The pH was adjusted using HCl/NaOH. The calculated values of the adsorption isotherms from the extended Langmuir model are shown as dotted lines, and those from the modified Langmuir model are shown as solid lines.

Table 2. Adsorption isotherm parameters obtained for B and As(V) in single systems.
The correlation parameters (ni) of B and As(V) were determined using the Solver tool in Microsoft Excel to minimize the residual sum of squares between the experimental data and calculated values using EquationEq. 9(9)
(9) (). The calculated values from the modified Langmuir are shown in (solid lines). The modified Langmuir model could describe the data for the adsorption isotherm; although, there were some deviations between the experimental data and calculated values, especially in the high concentration region. The deviation in the high concentration region, especially at pHeq = 6.95 and 8.64, is because the adsorption amount of B is not constant and is increased at this region. Alternative adsorption mechanism may occur at high concentration region of B/As(V) binary system. The absolute mechanism for this phenomenon should be revealed in the future work. A correlation larger than one indicates that adsorption in the binary system is inhibited more than is estimated by the extended Langmuir model. A correlation smaller than one indicates that adsorption in the binary system is promoted more than is estimated by the extended Langmuir model.
Table 3. Adsorption isotherm parameters obtained for B and As(V) in a binary system.
3.2. Effect of Anions on Adsorption of B
Adsorption of B from the Balcova simulated geothermal water was investigated. In this case, the pH of the simulated geothermal water was adjusted and the concentration of each component was kept constant. The adsorption amounts of the components in the Balcova simulated geothermal water were shown in . The adsorption amount of B for a solution only containing B is also shown in , to compare adsorption of B in the geothermal water. The pH for the geothermal water was set at less than 8 to prevent precipitation of hydroxides at alkaline pH. With the geothermal water, selective adsorption of B occurred and no adsorption of cationic metal ions (Li+, Na+, Mg2+, and Ca2+) was observed. Additionally, the adsorption of As(V) hardly occurred even though As(V) was more selectively adsorbed than B at pH < 5 (). This is because the concentration of As(V) in the Balcova geothermal water is much lower than that of B, and thus the adsorption of As(V) might be inhibited. The adsorption of B was shifted to a high pH compared with the single B solution. The effects of SO42− and Cl− on the adsorption of B were also investigated. For both B and SO42− or B and Cl−, the co-existing anions were selectively adsorbed in the acidic pH region (). Therefore, inhibition of the adsorption of B from the Balcova simulated geothermal water is caused by the co-existing anions.
Figure 4. Effect of pH on the adsorption of B, As(V), Li+, Na+, Mg2+, and Ca2+ from simulated Balcova geothermal water, and adsorption of B from a solution containing only B ([B]feed = 1 mmol/L). The composition of the simulated Balcova geothermal water is shown in . The pH was adjusted using HNO3/NH3.
![Figure 4. Effect of pH on the adsorption of B, As(V), Li+, Na+, Mg2+, and Ca2+ from simulated Balcova geothermal water, and adsorption of B from a solution containing only B ([B]feed = 1 mmol/L). The composition of the simulated Balcova geothermal water is shown in Table 1. The pH was adjusted using HNO3/NH3.](/cms/asset/3e4c199d-aef9-4ddf-ba23-d52aa106a1f7/tjce_a_2297558_f0004_c.jpg)
3.3. Elution of B and As(V)
Elution of B and As(V) from the adsorbent with different concentrations of HCl and NaOH was investigated (). When HCl was used as the eluent, both B and As(V) were almost quantitatively eluted in all cases, except for elution of As(V) with 1 mol/L HCl. The lower elution yield of As(V) with a low HCl concentration is consistent with the high adsorption amount of As(V) in the acidic pH region (). When NaOH was used as an eluent, a high elution yield of As(V) was obtained, while the elution yield of B was low. The results were consistent with the adsorption of B in the alkaline pH region being higher than that of As(V) (). It is expected that separation of B and As(V) could be achieved by elution with NaOH and then HCl.
3.4. Separation of B and as(V) from Geothermal Water by Column Adsorption
The adsorbent was finally applied to the separation of B and As(V) from the geothermal water. shows the breakthrough curve of B for the Balcova geothermal water, the elution curves of B, As(V), and Ca2+, and the concentration profile of B during washing of the column with deionized water between the adsorption and elution steps (concentration profiles of the other elements are omitted from the figure). Complete adsorption of B was observed until a bed volume of approximately 250, and then breakthrough occurred for B. When the same operation (adsorption, washing, and elution) was examined for a solution containing only B ([B]feed = 1 mmol/L, pHfeed = 8.54), the breakthrough and elution curves were almost the same as those shown in . Therefore, co-existing components in the geothermal water hardly affected the adsorption and elution of B. When the column was washed with deionized water to remove the geothermal water remaining in the column before elution, some B was eluted with the deionized water and this made the concentration of B greater than the feed concentration. In the elution, B was effectively eluted with H2SO4. The adsorption and elution amounts of B were calculated from the breakthrough and elution curves, and were summarized in , together with elution yield. The elution yield was defined as EquationEq. 10(10)
(10) .
(10)
(10)
Figure 7. (a) Breakthrough curve of B, (b) washing profile of B, and (c) elution curves for B, As(V), and Ca2+ in the Balcova geothermal water.

Table 4. Amounts of adsorption, eluted during washing, and elution of B and As(V) in column operation for geothermal water samples
indicated that quantitative elution could be achieved with the column operation. Additionally, some As(V) and Ca eluted (). The concentration of As(V) in the effluent during adsorption was not measured because the concentration of As(V) in the Balcova geothermal water was quite low. The amount of As(V) eluted was 45.2% of that fed into the column.
shows the breakthrough and elution curves for B, As(V), Li+, K+, and soluble SiO2 in the Kakkonda geothermal water, and the concentration profiles during washing of the column with deionized water between the adsorption and elution steps (concentration profiles of the other elements are omitted from the figure). Effective adsorption of B was observed until the bed volume reached approximately 90, and then breakthrough occurred for B. The effluent concentration of B is lower than the Japanese Effluent standards (10 mg/L) until the bed volume of approximately 110. Additionally, complete adsorption of As(V), less than the Japanese Effluent standards (0.1 mg/L), was achieved until the bed volume reached approximately 40. Although selectivity was higher for As(V) than B in the adsorption isotherms for the B and As(V) binary system at pH = 4.3, breakthrough for As(V) occurred sooner than that for B. Simultaneous removal of B and As(V) was achieved until the bed volume reached approximately 50. Almost no adsorption was observed for other components in the geothermal water. When the column was washed with deionized water to remove the geothermal water stagnated in the column, some B eluted with the deionized water, as was observed for the Balcova geothermal water. The adsorption and elution amounts of B and As(V) are summarized in . For B, almost all B was eluted with acid, which was similar to the results for the Balcova geothermal water. For As(V), 56% of As(V) was eluted with alkali and 40% of A(V) was eluted with acid. An increase in the alkali concentration would be required to achieve separation of B and As(V) during the elution.
4. Conclusions
Separation of B and As(V) from geothermal water was investigated using a glucamine-based chelate adsorbent. In single systems, adsorption of B was high in the neutral pH region and that of As(V) was high in the acidic pH region. Adsorption of B in B and As(V) binary system was inhibited in the acidic pH region by the presence of As(V). The adsorption isotherms of B and As(V) in the single systems at pHeq 4.3, 7.0, and 8.6 were of the Langmuir type and indicated that the adsorbent had higher affinity for B than As(V). In the binary B and As(V) system, the adsorption was inhibited or promoted, and the modified Langmuir equation was required to describe the adsorption isotherm. Adsorption of B was inhibited by co-existing SO42− and Cl− in the aqueous solution. Simultaneous removal of B and As(V) from geothermal water was achieved by column adsorption. Adsorbed B and As(V) were eluted with an acid or combination of an acid and an alkali; however, separation of B and As(V) during elution was not achieved.
Acknowledgments
This work was supported by JSPS (Japan Society for the Promotion of Science) and TUBITAK (The Scientific and Technological Research Council of Turkey, Grant Number TUBITAK-JSPS 214M360) under the Japan–Turkey Research Cooperative Program and JSPS KAKENHI (Grant Number JP19H00842). The adsorption data for As(V) in (a) were obtained by Ms. Kanako Shibata of The University of Kitakyushu. We thank Gabrielle David, PhD, from Edanz (https://jp.edanz.com/ac) for editing a draft of this manuscript.
References
- Bolan S, Wijesekara H, Amarasiri D, Zhang T, Ragályi P, Brdar-Jokanović M, Rékási M, Lin J-Y, Padhye LP, Zhao H, et al. 2023. Boron contamination and its risk management in terrestrial and aquatic environmental settings. Sci Total Environ. 894:164744. doi:10.1016/j.scitotenv.2023.164744.
- Dambies L, Salinaro R, Alexandratos SD. 2004. Immobilized N-methyl-D-glucamine as an arsenate-selective resin. Environ Sci Technol. 38:6139–6146. doi:10.1021/es040312s.
- Guidelines for drinking-water quality: fourth edition incorporating the first addendum, World Health Organization. 2017.
- Ighalo JO, Amaku JF, Olisah C, Adeola AO, Iwuozor KO, Akpomie KG, Conradie J, Adegoke KA, Oyedotun KO. 2022. Utilisation of adsorption as a resource recovery technique for lithium in geothermal water. J Mol Liq. 365:120107. doi:10.1016/j.molliq.2022.120107.
- Kumar S, Zafar M, Prajapati JK, Kumar S, Kannepalli S. 2011. Modeling studies on simultaneous adsorption of phenol and resorcinol onto granular activated carbon from simulated aqueous solution. J Hazard Mater. 185:287–294. doi:10.1016/j.jhazmat.2010.09.032.
- Kuraoka A, Umebayashi T, Nishihama S, Yoshizuka K. 2018. Oxidative adsorption of arsenic by N-methylglucamine-modified chelate fiber and manganese dioxide. J Ion Exchange. 29:163–165. doi:10.5182/jaie.29.163.
- Lee SY, Seader JD, Tsai CH, Massoth FE. 1993. Multicomponent liquid-phase diffusin and adsorption in porous catalyst particles. Chem Eng Sci. 48:595–607. doi:10.1016/0009-2509(93)80313-F.
- Markham EC, Benton AF. 1931. The adsorption of gas mixtures by silica. J Am Chem Soc. 53:497–507. doi:10.1021/ja01353a013.
- McKay G, Porter JF. 1997. A comparison of Langmuir based models for predicting multicomponent metal ion equilibrium sorption isotherms on peat. Process Saf Environ Protect. 75:171–180. doi:10.1205/095758297529011.
- Meng X, Bang S, Korfiatis GP. 2000. Effects of silicate, sulfate, and carbonate on arsenic removal by ferric chloride. Water Res. 34:1255–1261. doi:10.1016/S0043-1354(99)00272-9.
- Mesmer RE, Baes CF, Sweeton FH. 1972. Acidity measurements at elevated temperatures. VI. Boric acid equilibriums. Inorg Chem. 11:537–543. doi:10.1021/ic50109a023.
- Mori Y. 2022. Binary power generation used waste heat in factories. J Surf Finish Soc Jpn. 73:608–615. doi:10.4139/sfj.73.608.
- Nasruddin MIA, Daud Y, Surachman A, Sugiyono A, Aditya HB, Mahlia TMI. 2016. Potential of geothermal energy for electricity generation in Indonesia: a review. Renew Sustain Energy Rev 53, 733–740. doi:10.1016/j.rser.2015.09.032.
- Nishihama S, Sumiyoshi Y, Ookubo T, Yoshizuka K. 2013. Adsorption of boron using glucamine-based chelate adsorbents. Desalination. 310:81–86. doi:10.1016/j.desal.2012.06.021.
- Park J, Sato H, Nishihama S, Yoshizuka K. 2012. Lithium Recovery from Geothermal Water by Combined Adsorption Methods. Solvent Extr Ion Exch. 30:398–404. doi:10.1080/07366299.2012.687165.
- Recepoğlu YK, Kabay N, Yılmaz-İpek İ, Arda M, Yüksel M, Yoshizuka K, Nishihama S. 2017a. Deboronation of geothermal water using N-methyl-D-glucamine based chelating resins and a novel fiber adsorbent: batch and column studies. J Chem Technol Biotechnol. 92:1540–1547. doi:10.1002/jctb.5234.
- Recepoğlu YK, Kabay N, Yılmaz-Ipek İ, Arda M, Yüksel M, Yoshizuka K, Nishihama S. 2017b. Elimination of boron and lithium coexisting in geothermal water by adsorption-membrane filtration hybrid process. Sep Sci Technol. 53:856–862. doi:10.1080/01496395.2017.1405985.
- Recepoğlu YK, Kabay N, Ipek IY, Arda M, Yüksel M, Yoshizuka K, Nishihama S. 2018. Packed bed column dynamic study for boron removal from geothermal brine by a chelating fiber and breakthrough curve analysis by using mathematical models. Desalination. 437:1–6. doi:10.1016/j.desal.2018.02.022.
- Sajkowski L, Turnbull R, Rogers K. 2023. A review of critical element concentrations in high enthalpy geothermal fluids in New Zealand. Resources. 12:68. doi:10.3390/resources12060068.