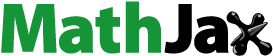
Abstract
A solid oxide fuel cell (SOFC) formed on a stainless-steel plate having through holes was studied. The obtained metal-supported SOFC can be operated at high temperatures such as 750 °C because it comprises an yttria-stabilized zirconia electrolyte. It was confirmed that the aperture ratio of the support metal plate affected the power generation performance of the metal-supported SOFC. The power density of the obtained SOFC at 0.8 V was increased from 0.22 W/cm2 to 0.85 W/cm2 at 750 °C by increasing the aperture ratio from 0.2% to 5.3%. Thus, it was found that the SOFC formed on a support metal plate having through-holes could show a power density comparable to or higher than that of previously reported SOFCs formed on porous sintered metal supports.
1. Introduction
Fuel cells are anticipated to be high efficiency and clean power generation devices. Solid oxide fuel cell (SOFC) has been mainly developed for stationary power source applications and commercialized for residential use (Nakao et al. Citation2019). Hydrogen-rich reformed gas from hydrocarbons such as natural gas is a convenient fuel for stationary fuel cell systems, especially for residential use, in terms of infrastructure. The hydrogen-rich reformed gas can be obtained from catalytic reforming processes, such as steam reforming and partial oxidation reforming. The steam reforming process seems to be desired for stationary fuel cell systems, in which high efficiency is required (Yamazaki et al. Citation2001). This is because H2 is derived from not only hydrocarbons but also H2O by steam reforming. Steam reforming, an endothermic reaction, is expected to be conducted at high temperatures, for example, over 650 °C, to shift the equilibrium to the H2 and CO production side (Tsuda et al. Citation2022). Therefore, an exceedingly high power generation efficiency can be achieved in the system using hydrocarbon raw fuel that consists of an SOFC stack operated over 650 °C because steam reforming can be carried out using the waste heat from the SOFC.
The research and development of metal-supported SOFCs (MSC) have been increasing recently due to MSC’s advantages such as robustness and low cost of materials (Tucker Citation2010; Nielsen et al. Citation2015). Metal supports can be broadly categorized into two types: One is a porous sintered metal made of fine metal powder, and the other is a metal plate equipped with many through-holes.
For the former, several studies on SOFCs equipped with yttria-stabilized zirconia (YSZ) or Scandia-stabilized zirconia (ScSZ) electrolyte, which can be operated at high temperatures such as over 650 °C, have been reported. For example, an SOFC equipped with an aerosol-deposited YSZ electrolyte formed on a porous sintered metal support has been reported. The power density was 0.53 W/cm2 at 0.8 V at 750 °C (Choi et al. Citation2014). Moreover, an SOFC equipped with a YSZ electrolyte fabricated by the gas flow sputtering process formed on a porous sintered metal support has been reported. The power density was 0.64 W/cm2 at 0.8 V at 750 °C (Thaler et al. Citation2019). Moreover, an SOFC equipped with a ScSZ electrolyte fabricated by tape casting and co-sintering process, formed on a porous sintered metal support has been reported. The current density was 0.6 A/cm2 at 0.8 V at 700 °C, and the power density is calculated to be 0.48 W/cm2 (Nielsen et al. Citation2015). An SOFC, equipped with a ScSZ electrolyte fabricated by screen printing and co-sintering processes formed on a porous sintered metal support has also been reported. The power density was 0.43 W/cm2 at 0.8 V at 700 °C (Yamaguchi et al. Citation2023).
Regarding the latter, there has been a lack of report regarding an SOFC equipped with YSZ electrolyte formed on a support metal plate having through-holes.
Manabe et al. (Citation2023) reported an SOFC equipped with an aerosol-deposited YSZ electrolyte fabricated on a support metal plate having through-holes. The aperture ratio of the support metal plate was low, approximately 0.2%, and the power density was also low such as ca. 0.17 W/cm2 at 0.8 V at 750 °C. In this study, the power generation performance of the SOFC equipped with a YSZ electrolyte fabricated on a support metal plate was investigated for improvement.
2. Experimental
Metal-supported SOFCs were prepared using a fabrication procedure similar to that shown in the previous literature (Manabe et al. Citation2023). For the metal support, a ferritic-type stainless-steel plate (Crofer 22 APU) with a thickness of 0.3 mm and a diameter of 25 mm was used. In a region with a radius of 2.5 mm from the center of the metal plate, multiple through-holes were formed in a square reticular pattern by laser beam drilling. An anode layer was formed on the support metal plate to cover the region where through-holes were processed, using screen printing with a slurry containing NiO and 10GDC (Ce0.9Gd0.1O1.95) powders with a 6:4 weight ratio. It was then sintered at 950 °C following degreasing and pressurization. The average thickness of the anode layer was 10 μm. An anode functional layer was formed on the anode layer by screen printing using a slurry containing 10GDC powder. After the degreasing process, the anode functional layer formed on the anode layer was pressurized and heated at 1000 °C. The average thickness of the anode functional layer was 7 μm. The anode functional layer as a buffer did not contain Ni (Echigo et al. Citation2021). A previous report (Manabe et al. Citation2023) indicated that a YSZ electrolyte layer was then formed on a 15 mm × 15 mm region of the anode and anode functional layers–laminated metal plate at room temperature using the aerosol deposition (AD) method. In the AD method, 8 mol% YSZ powder controlled to approximately 0.7 μm in the mode diameter was aerosolized using dry air, which serves as the carrier gas. The YSZ powders contained in the aerosol were deposited onto the anode and anode functional layers–laminated metal plate. The electrolyte layer completely covers the anode functional layer formed on the anode layer and has a contact area with the support metal plate. The average thickness of the electrolyte layer was 5 μm. Furthermore, an interlayer was formed on the electrolyte layer using screen printing with a slurry containing 10GDC powder. After the degreasing process, the interlayer formed on the electrolyte layer was pressurized and heated at 1000 °C. The average thickness of the interlayer was 6 μm. Finally, a cathode layer was formed on the interlayer by tape casting using a slurry containing (La,Sr)(Co,Fe)O3-δ (LSCF) and 10GDC powders with weight ratio of approximately 2:1, which was then heated at 900 °C. The average thickness of the cathode layer was 5 μm. shows the cross-sectional conformation diagram of the metal-supported SOFC and the surface conformation diagram of the support metal plate fabricated in this study. The aperture ratio of the metal plate for the support was calculated according to Equation (1) using symbols r, which represents the radius of the through-hole, and p, which represents the pitch of the through-hole.
(1)
(1)
shows the specifications of the support metal plate used in this study.
Figure 1. Conformation diagram of the metal-supported SOFC and surface conformation diagram of the support metal plate fabricated in this study.

Table 1. Specifications of the metal plate for the support used in this study.
To measure the power generation performance, the obtained metal-supported SOFC was set between two pieces of an alumina double tube in an electric furnace. The operation temperature was measured by a thermocouple located close to the cell inside the alumina double tube. H2 containing 4.2% by volume of H2O was supplied to the anode side of the metal-supported cell, and dry air was supplied to the cathode side. H2O was mixed with H2 through a temperature-controlled bubbler. In the test using dilute H2 as the feed gas to the anode side, N2 was used as the dilution gas. The flow rate of the H2 or H2/N2 mixed gas was kept constant at 50 ml/min, while that of the dry air was also kept constant at 50 ml/min. Three mass flow controllers (HM-1000, HEMMI Slide Rule) were used to control the flow rates of H2, N2, and air. The current-voltage (I-V) characteristics were measured using an electronic load (FK-160L2Z, Takasago) in the temperature range of 600 °C to 750 °C. The hydrogen concentration values were shown in dry base. The area-specific resistance (ASR) was calculated from the current density and cell voltage slope at approximately 0.8 V. The ASR includes the ohmic resistance of the metal-supported SOFC and the activation and gas diffusion resistances of the electrodes. The ratio of ASR (10% H2/100% H2) was calculated by dividing the ASR value of 10% H2 by that of 100% H2 for the feed gas conditions to the anode side.
3. Results and Discussion
3.1. Dependence of the Power Generation Performance at Operation Temperature
shows the power generation performance of the metal-supported SOFC equipped with YSZ electrolyte at the operation temperature. The aperture ratio of the metal plate for the support was 0.2%. shows that the current density increased with increasing operation temperature from 600 °C to 750 °C. For example, the current densities at 0.8 V, which is the desired cell voltage for high power generation efficiency, were 0.043 A/cm2 at 600 °C, 0.083 A/cm2 at 650 °C, 0.15 A/cm2 at 700 °C, and 0.27 A/cm2 at 750 °C. Therefore, the power density increased with increasing operation temperature from 600 °C to 750 °C, as shown in . The power densities at 0.8 V were 0.034 W/cm2 at 600 °C, 0.066 W/cm2 at 650 °C, 0.12 W/cm2 at 700 °C, and 0.22 W/cm2 at 750 °C. Thus, it was confirmed that the power generation performance of the metal-supported SOFC equipped with YSZ electrolyte improved with increasing operation temperature, even over 600 °C. However, the power density at 0.8 V was low, such as 0.22 W/cm2, even at 750 °C compared with that of the SOFC equipped with YSZ electrolyte formed on a porous sintered metal support, as shown in the Introduction. The low power density of the obtained metal-supported SOFC was thought to be due to the exceedingly low aperture ratio of the metal plate. The dependence of the aperture ratio of the metal plate on the power generation performance of the metal-supported thin-film SOFC was then investigated.
3.2. Dependence of Power Generation Performance on Aperture Ratio of the Support Metal Plate and Influence of Hydrogen Concentration in Fuel Gas
shows the power generation performance of SOFCs equipped with YSZ electrolytes using various support metal plates (A, B, C, D) at 750 °C. shows that the current density increased with increasing aperture ratio from 0.2% to 5.3%. For example, when the aperture ratios were 0.2%, 0.8%, 1.8%, and 5.3%, the current densities at 0.8 V were 0.27 A/cm2, 0.61 A/cm2, 0.77 A/cm2, and 1.06 A/cm2, respectively. Therefore, the power density was increased with increasing the aperture ratio from 0.2% to 5.3%, as shown in . For example, the power densities at 0.8 V were 0.22 W/cm2, 0.49 W/cm2, 0.61 W/cm2, and 0.85 W/cm2 at aperture ratios of 0.2%, 0.8%, 1.8%, and 5.3%, respectively. shows the increase in the power density at 0.8 V with increasing aperture ratio. also shows that the increase in the power density at lower aperture ratios is greater than that at higher aperture ratios. The diffusion of fuel H2 to the anode layer at lower aperture ratios is expected to be harder than that at higher aperture ratios. Thus, it can be considered that the improvement of the diffusion of fuel H2 to the anode layer by increasing the aperture ratio affected the increase in the power density.
Figure 3. Power generation performance of SOFCs equipped with YSZ electrolytes using various aperture ratios of the support metal plates (A, B, C, D) at 750 °C, (a) I–V characteristics, (b) I–W characteristics.

The ASR was calculated to confirm that increasing the aperture ratio improved H2 diffusivity. shows the results. The values of the ASR of metal-supported SOFC (MSC) using support metal plates with various aperture ratios (metal plates A, B, and D) increased as the hydrogen concentration decreased from 100% to 10%. This increase in ASR values caused by dilution of H2 is thought to be due to an increase in the resistance of the diffusion-related factors at the anode. They include the activation and gas diffusion resistances. Kishimoto et al. (Citation2019) reported that the activation and gas diffusion resistances at the anode increased as the hydrogen concentration decreased. In our study, as the aperture ratio increased, the influence of H2 dilution, i.e., the ratio of ASR (10% H2/100% H2), decreased. It can be considered that the increase in the resistance of diffusion-related factors at the anode is suppressed by increasing the aperture ratio.
Table 2. Area specific resistance (ASR) of the metal-supported SOFC (MSC) using support metal plates with various aperture ratios (metal plates A, B, and D) at 750 °C and around 0.8 V under hydrogen concentration conditions of 100% and 10%.
For reference, Choi et al. (Citation2014) reported that the maximum power density at 750 °C was 0.71 W/cm2 in an SOFC consisting of an aerosol-deposited YSZ electrolyte formed on a porous sintered metal support, and the power density at 0.8 V was 0.53 W/cm2. Thaler et al. (Citation2019) reported that the power density at 0.8 V was 0.64 W/cm2 in an SOFC formed on a porous metal support. Therefore, it was found that the power density of an SOFC formed on a support metal plate having through-holes with an aperture ratio of several percent can exceed that of previously reported SOFCs formed on porous sintered metal supports.
shows the influence of the hydrogen concentration in the fuel gas supplied to the anode side of the cell on the power generation performance of the metal-supported SOFC at 750 °C. The aperture ratio of the support metal plate was 5.3%. shows that the current density slightly decreased with decreasing hydrogen concentration from 100% to 50%. shows that the power density was then slightly decreased with decreasing hydrogen concentration from 100% to 50%. The power densities at 0.71 A/cm2 were 0.62 W/cm2, 0.60 W/cm2, and 0.59 W/cm2 at hydrogen concentrations of 100%, 70%, and 50%, respectively. In the case of using a hydrocarbon reformed gas as the fuel, the concentration of the fuel (H2 + CO) to the anode may be reduced to approximately 70% (Tsuda et al. Citation2022). Therefore, the diminution rate in the power density is assumed to be 5% when the fuel gas is changed from hydrogen to hydrocarbon reformed gas. It is considered that the obtained metal-supported SOFC, when using a hydrocarbon reformed gas as the fuel, can show comparable or higher power density than the previously reported SOFCs formed on porous sintered metal supports.
Figure 5. Dependence of the power generation performance of the SOFC formed on a metal plate C with an aperture ratio of 5.3% on the hydrogen concentration of the fuel gas supplied to the anode at 750 °C, (a) I–V characteristics, (b) I–W characteristics.

Further investigations on the SOFC equipped with YSZ electrolyte formed on a support metal plate, such as the influence of the radius and pitch of the through-holes on the power generation performance and electrochemical analysis, will be reported in the future.
4. Conclusion
The power generation performance of the SOFC equipped with YSZ electrolyte formed on a stainless-steel plate having through-holes was investigated. It was found that the power generation performance of the metal-supported SOFC improved with an increase in the operation temperature from 600 °C to 750 °C, and the power density of the metal-supported SOFC at 0.8 V, the desired cell voltage for a high power generation efficiency, was increased from 0.22 W/cm2 to 0.85 W/cm2 at 750 °C with an increase in the aperture ratio of the support metal plate from 0.2% to 5.3%. It can be considered that the improvement of the diffusion of fuel H2 to the anode layer by increasing the aperture ratio affected the increase in the power density. It is considered that the SOFC formed on the support metal plate with an aperture ratio of 5.3%, in the case of using a hydrocarbon reformed gas as the fuel, can show comparable or higher power density than the previously reported SOFCs formed on porous sintered metal supports.
Disclosure statement
No potential conflict of interest was reported by the author(s).
References
- Choi J-J, Ryu J, Hahn B-D, Ahn C-W, Kim J-W, Yoon W-H, Park D-S. 2014. Low temperature preparation and characterization of solid oxide fuel cells on FeCr-based alloy support by aerosol deposition. Int. J. Hydrogen Energy. 39:12878–12883. doi: 10.1016/j.ijhydene.2014.06.070.
- Echigo M, Ohnishi H, Manabe K, Yamazaki O, Minami K, Tsuda Y. 2021. Metal-supported electrochemical element, solid oxide fuel cell and method of manufacturing such metal-supported electrochemical element. United States Patent. 189:838.
- Kishimoto M, Masuyama A, Iwai H, Yoshida H. 2019. Dual-resolution microstructural analysis of anisotropic pore structure in SOFC anode fabricated by phase-inversion tape casting. ECS Trans. 91:1861–1869. doi: 10.1149/09101.1861ecst.
- Manabe K, Echigo M, Tsuda Y, Minami K, Akedo J, Ohnishi H. 2023. Formation of dense yttria-stabilized zirconia thin film by aerosol deposition method for metal-supported solid oxide fuel cell applications. J. Chem. Eng. 56:2261771.
- Nakao T, Inoue S, Uenoyama S, Takuwa Y, Suzuki M. 2019. Progress of SOFC residential CHP system: over 50,000 units market experience of osaka gas. ECS Trans. 91:43–49. doi: 10.1149/09101.0043ecst.
- Nielsen J, Sudireddy BR, Hagen A, Persson ÅH. 2015. Performance factors and sulfur tolerance of metal supported solid oxide fuel cells with nanostructured Ni:GDC infiltrated anodes. ECS Trans. 68:1361–1372. doi: 10.1149/06801.1361ecst.
- Thaler F, Nenning A, Bischof C, Udomsilp D, de Haart LGJ, Opitz AK, Bram M. 2019. Optimized cell processing as the key of high electrochemical performance of metal-supported solid oxide fuel cells. ECS Trans. 91:887–900. doi: 10.1149/09101.0887ecst.
- Tsuda Y, Shinke N, Echigo M. 2022. Simulation of a thin film solid oxide fuel cell system equipped with an internal fuel reforming unit. J Chem Eng. 55:162–169. doi: 10.1252/jcej.21we110.
- Tucker MC. 2010. Progress in metal-supported solid oxide fuel cells. J. Power Sources. 195:4570–4582. doi: 10.1016/j.jpowsour.2010.02.035.
- Yamaguchi Y, Sumi H, Takahashi H, Aso S, Tanabe S, Shimizu H. 2023. Effect of fabrication processes on electrochemical performance of metal-supported solid oxide fuel cells. ECS Trans. 111:323–330. doi: 10.1149/11106.0323ecst.
- Yamazaki O, Echigo M, Shinke N, Tabata T. 2001. Development of residential PEFC cogeneration system at Osaka gas. Proc. The Fuel Cell Home. 93–102.