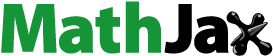
Abstract
A scientific biography is presented as an introduction to a collection of papers by his colleagues and co-workers honouring the scientific contributions and legacy of the late Dr. Timothy J. Lee (1959–2022). Tim Lee performed highly regarded research on the methods and applications of computational quantum chemistry, particularly coupled cluster theory, and computational spectroscopy, with impacts in interstellar chemistry, aiding NASA missions, atmospheric chemistry, as well as fundamental science. His presence is deeply missed.
1. Introduction
It is a pleasure for us to recall our professional and personal interactions with Dr. Timothy J. Lee, who passed away too young in late 2022. It is also a pleasure to acknowledge everyone who has contributed to this Memorial Issue in Tim's memory. This Issue has come together because Tim was not just respected and admired but also because he was very well-liked by his colleagues worldwide. Our goal in this biography is to shed some light on the impressive range of his scientific achievements so that we can remember them. We begin with a short overview of Tim's life, career and awards, and then turn to discussing his main scientific accomplishments, as well as providing a complete list of his scientific publications [Citation1–295].
Tim was born in Denver, Colorado, to Catherine A. Caskins and David T. Lee on 14th December 1959, as their second child alongside his two brothers Dave and Mike. After doing well in high school, Tim did his undergraduate studies at the Colorado School of Mines, where he developed his passion for chemistry. Tim spent the years between 1982 and 1986 completing doctoral research in theoretical quantum chemistry at the Chemistry Department of the University of California at Berkeley. His advisor, Fritz Schaefer, will discuss his doctoral work in the following section. It was in Berkeley that Tim met his future wife, Julia Rice, who was a visiting Ph.D. student from Cambridge. Julia and Tim were married on 18 June 1988, at Clare College, Cambridge. Together they raised three boys (Sebastian, Marcus and Trevor) in Sunnyvale, California.
In 1988, he received the first Dirac Medal from the World Association of Theoretical and Computational Chemists (WATOC) for being an ‘outstanding computational chemist in the world under the age of 40’. After 2 years of postdoctoral research in England, Tim began a prolific 33-year career at NASA Ames Research Center, initially in the Computational Chemistry Branch, and then later in Space Science and Astrobiology Division. Some of the main research areas he touched are described in the following sections. In addition to research, Tim also served as the branch chief of the Astrophysics Branch and the Division Chief of the Space Science and Astrobiology Division for a decade. His work at NASA was recognised with the NASA Exceptional Scientific Achievement Medal in 1998 and, again, in 2011. As the Chief of the Space Science and Astrobiology Division, Tim was instrumental in setting up two science-enabling research areas that are now being utilised by the scientific community: the Mars Climate Modeling Center and the Ames Astrobiology Habitable Environments Database. Among many other things, Tim set up a division-wide, one-day internal conference called the Jamboree that awards one senior and one early career award. The early career award of the Space Science and Astrobiology Division has now been renamed in Tim's honour as the ‘Tim Lee Early Career Award’. Tim was an elected Fellow of the American Physical Society and of the American Association for the Advancement of Science.
2. Doctoral research
Tim Lee received his undergraduate education from the Colorado School of the Mines completing his BS in the Spring of 1982. ‘Mines’, as the university is still called, is located in Golden, Colorado, about 15 miles west of Denver. Golden, of course is better known as the home of the Coors Brewing company. Tim moved from his home state of Colorado to pursue his Ph.D. at the University of California, Berkeley, in the Autumn of the same year. He joined my research group shortly after the start of his doctoral studies. Although Mines has a good undergraduate chemistry program (Tim was one of the original students of the department), Tim's background in physics, mathematics and computer science was not as extensive as those of some of his Berkeley classmates. Perhaps the first thing I (Fritz Schaefer) remember about Tim was his positively tenacious efforts to bring his background up to those of our very best Ph.D. students. Tim was entirely successful in achieving this goal. The same passionate search for deep scientific understandings characterised Tim's entire professional career.
Equally relentless were Tim's efforts as a baseball player. Tim was one of the stars of the Self-Consistent-Fielders softball team. Our team members came from my group, that of Bill Miller, plus one highly gifted player from Bill Lester's research group. For an intramural league, the competition was fierce, with the teams of Chemistry Professors George Pimentel and Yuan T. Lee always among the best. Nevertheless, the Self-Consistent-Fielders took the Berkeley championship in the Spring of 1987. The photograph of our team of champions is truly remarkable.
Tim published 36 papers with yours truly over the years. Of the 36, no less than 29 of these papers were based in whole or in part on Tim's graduate studies at Berkeley. Quite a remarkable record, indeed unique among my 135 Ph.D. graduates!! To keep this discussion manageable, I will primarily mention those nine papers which became ‘Citation Classics’, i.e. having 100 or more citations. Tim was a critical part of the team (including Gustavo Scuseria and Andy Scheiner) that developed analytic gradient methods for coupled cluster (CCSD to begin) methods. The first of these papers [Citation26] has received 417 citations and continues to be cited (present date of writing is November 2023). Tim's paper 9 months earlier [Citation20], comparing CCSD and CISD results has 350 cites. Tim's third-most highly cited paper (334) with me [Citation4] concerned the simple negative ions OH, CN
,
,
and
, and set a new standard for the high-level theoretical treatment of molecular anions.
Tim was the first author (by then at NASA Ames) of an important (1989) paper [Citation37] with a very long title ‘Theoretical Investigations of Molecules Composed only of Fluorine, Oxygen, and Nitrogen – Determination of the Equilibrium Structures of FOOF, (NO), and FNNF and the Transition State Structure for FNNF Cis-Trans Isomerisation’. Julia Rice (by then at IBM San Jose) and Gustavo Scuseria (moved with me to Georgia) were Tim's co-authors. The lengthy title arose from the fact that FOOF and FNNF had turned out to be very challenging molecules for theory. However, the great importance of the paper arose from a new theoretical proposal. This is described clearly in the last sentence of our abstract: ‘A new diagnostic method for determining a priori the reliability of single reference based electron correlation methods is suggested and discussed’. The
diagnostic has been used in nearly countless numbers of theoretical papers since then. Tim's second paper on this topic [Citation36], with his gifted long-time collaborator Peter Taylor, was submitted 8 months later. Taken together, these two papers are among the most important in the field of electronic structure theory from the last two decades of the twentieth century.
Tim's most important applications paper with me was probably his work on the cyclopropenylidene diradical [Citation6]. This research was motivated by a 1983 sabbatical visit to Berkeley by the well-known physical organic chemist Reinhard Hoffmann from the University of Marburg, Germany. Despite great interest, this molecule had not been observed spectroscopically at the time of our interest, which was sparked by conversations with Reinhard Hoffmann. Professor Hoffmann challenged us to study cyclopropenylidene. Tim not only predicted the structure and vibrational frequencies of cyclopropenylidene, but also the infrared intensities, rarely determined by theory at the time. With Tim's infra-red (IR) intensities in hand, Hoffmann and his University of Giessen friend Gunter Maier (another distinguished physical organic chemist), quickly observed and identified the IR spectrum predicted by Tim. Tim continued for years to be interested in cyclopropenylidene [Citation129, Citation159, Citation166, Citation240], his last paper on this subject [Citation258] being published in 2020.
Tim was also first author (co-authors Doug Fox and Russ Pitzer) of the paper ‘Analytic Second Derivatives for Renner-Teller Potential Energy Surfaces. Examples of the Five Distinct Cases’ [Citation1]. This paper contains some nice mathematics and has been well cited (114), although I suspect that number underestimates the importance of this paper. I don't think the five cases of Renner–Teller splittings had been noted previously, and they are splendidly illustrated in Tim's first figure of the paper [Citation1]. The accessibility of analytic second derivative methods brought to light the previously undocumented five cases.
Tim had two other citation classics at Berkeley involving analytic derivatives [Citation12, Citation27]. However, the most Herculean success of all was Tim's research on analytic second derivatives for the general configuration interaction method [Citation14]. Although receiving only 52 cites, this paper was the first to achieve analytic second derivatives for any method more complicated than second-order perturbation theory. For the example of Tim's new method, we chose the HSOH molecule as a more demanding one since it had no elements of point group symmetry.
I visited Tim at NASA Ames on Friday 29 April 2022. The lab was still almost shut down due to the Covid-19 pandemic. Attendance at my lecture was limited to 12 people, but that limitation was unnecessary in light of the tiny number of people in the building. We had an enjoyable casual lunch with Charlie Bauschlicher, Partha Bera and a few others. After lunch I spent 3 full hours with Tim, little realising how soon he would be gone. We discussed science, families and life. Tim and Julia's oldest son Sebastian had spent the Summer between his third and fourth undergraduate years at the CCQC in Georgia. So I wanted to catch up on Sebastian, who had subsequently finished his Ph.D. degree at Cal Tech. Sebastian had fallen in love with a fellow scientist from Germany, and he and she were planning a life together in Germany. We listened to a number of songs written and sung by Tim and Julia's second son, whose full-time work is at Google. We could have talked for many more hours if we had known that Tim's days on earth were very limited. Except for a few extra pounds, Tim appeared in good health, as he always had. To my surprise, I subsequently learned that Tim came into the lab for only a few days in his final weeks following my visit. Tim Lee was a great scientist and a wonderful friend to me and so many others.
3. Electron correlation theory
As I (Martin Head-Gordon) write this, it is interesting to note that much of electronic structure theory can be viewed as reasonably well settled in 2023. Density functional theory (DFT) with the latest, most robust functionals is used for routine calculations, and in cases where the highest levels of accuracy are not needed. On the other hand, coupled cluster theory with singles and doubles (CCSD), followed by a perturbative triples correction (CCSD(T)), or even higher order methods, are used for cases where high accuracy is essential, and the use of the mean-field single reference determinant can still be justified. However, when Tim was a newly minted Berkeley Ph. D. graduate, the CCSD(T) method [Citation296] had not yet been published, and implementations of CCSD were not even available in the most widely used computer codes. In short, it was a time of transition from a previous generation of methods such as configuration interaction (CI) and Møller–Plesset perturbation theory, to CC methods. Tim played a significant part in this transition, as the paragraphs below should reveal, in roughly 5–10 years of intense scientific focus on efficient implementations, carefully selected tests and innovative new ideas. Much of the progress he achieved was described in a book chapter co-written with one of his major collaborators on electron correlation, Gustavo Scuseria [Citation118].
As a graduate student, Tim had already worked closely with Gustavo Scuseria on the implementation of coupled cluster methods in the Schaefer group codes [Citation19, Citation20]. Upon starting his independent work, Tim collaborated closely with Julia Rice while they were both at Cambridge to develop an efficient formalism and an efficient implementation of coupled cluster theory with single and double substitutions (CCSD) for closed shell molecules [Citation35]. Upon arriving at NASA Ames, he collaborated with Gustavo Scuseria, who was now a professor at Rice University, on early and influential assessments of triples corrections to CCSD, including CCSD(T), as well as other alternatives [Citation50]. Tim also made applications of CCSD(T) for studies of ozone [Citation47, Citation48] and tetrahedral N [Citation53], as well as numerous other chemical applications. Tim and Gustavo additionally worked on comparing CCSD and CCSDT against the quadratic configuration interaction (QCI), which was proposed by the Pople group (including this writer) in 1987: they were not supportive of QCI! [Citation49]
Key to the popularisation of CCSD and CCSD(T) was development and implementation of efficient algorithms for the analytic gradient. Working with one of the postdoctoral researchers, Alistair Rendell, they developed an efficient formulation and implementation of the CCSD gradient [Citation57], and then the CCSD(T) gradient [Citation58]. While these projects are simple to describe, they were highly technical to accomplish, and Tim used these projects as a springboard to build his coupled cluster programs, the Titan codes. Like many research programs of this period, it needed an SCF code to drive it, as well as another code to produce transformed two-electron integrals. While this made maintenance and porting Titan challenging, Tim used it for a wide variety of follow-on projects during the early to mid-1990s. With regard to high performance computing (as it was at the time), Alistair and Tim developed vector-parallel implementations that achieved high performance on Cray machines [Citation56, Citation66]. It is standard practice today to minimise storage requirements for CCSD(T) calculations by using resolution-of-the-identity (RI) basis sets that factorise 4-centre 2-electron integrals into sums of products of 2 and 3-centre integrals. Tim was the first to recognise this potential, and report tests of accuracy, in another important paper based on modifying the Titan codes [Citation102].
On a personal level, it was after an awkward software breakup with Gaussian (and long before the new Q-Chem project that caused that was viable), that I first contacted Tim to try to use the Titan codes for early work on generalising ground state CCSD to excited states via linear response theory. Tim was wonderful: he enthusiastically agreed, and my student and I worked on reimplementing our work in Titan after detailed tutorials in Tim's office (an hour's drive away from Berkeley under ideal conditions, which typically never applied). We soon completed an implementation and tests of linear response CCSD as well as the related quadratic CI (QCISD) for excited states [Citation297]. Tim is not an author of that first paper because he refused to be, despite providing lots of technical help. This speaks to his high level of academic ethics. We did then collaborate on an assessment of CCSD versus QCISD for excited states [Citation95]. It turned out that QCISD was missing some physically important excited state contributions, and Tim was quite happy with this result, because he had never liked QCISD for ground states anyway! Though I must note in passing that ground state QCISD was a project [Citation298] I was involved in during graduate school… Later that same year, collaborating with Tim enabled the quick implementation of my CIS(D) idea in his Titan codes [Citation96]. CIS(D) is an excited state analog of the ground state second order Møller–Plesset (MP2) method that offers the same fifth power scaling [Citation299].
One of Tim's most impactful lines of work on electron correlation was developing a diagnostic that can inform when CCSD and CCSD(T) should be expected to work versus when they become questionable, as mentioned above. This work began as he and Julia were finishing their postdoctoral work in Cambridge and preparing to move to California, where it was finished at NASA Ames. In a study of several molecules that were challenging for CCSD, including FOOF and (NO), Tim and co-workers observed that relatively large Euclidean norms of the singles (
) amplitudes could be associated with significant orbital relaxation (i.e. the mean-field reference was relatively poor) [Citation37]. In what is I think his most highly cited paper, Tim and his collaborator, Peter Taylor (then also at NASA Ames), established the ‘
diagnostic’ as a valuable tool for assessing when CCSD and CCSD(T) calculations could be viewed as reliable [Citation36]. Later Tim published other follow-up papers on diagnostics [Citation115], extending the
and
diagnostics to open-shell molecules [Citation156], including one that we cooperated on for the CIS(D) method [Citation134].
Working with his very talented postdoctoral scholar, Dylan Jayatilaka, Tim developed another major line of research that pioneered a new approach to electron correlation methods for open shell molecules [Citation74]. In contrast to the usual approach of employing α or β orbitals for open shell species, Dylan and Tim suggested [Citation74] that there was a computational advantage to using eigenfunctions of instead of
just for the half-occupied orbitals. This led to the development of an open-shell perturbation theory, the so-called z-averaged perturbation theory (ZAPT) [Citation77], which offered reduced computational effort compared to other open shell perturbation methods. Likewise, Dylan and Tim uncovered a computational advantage to performing open shell CCSD using the
basis for the half-occupied levels [Citation83]. This line of work continued with a collaboration with Daniel Crawford and Fritz Schaefer, analysing open shell perturbation theories [Citation122], exploring Brueckner orbitals using the
basis [Citation137], and developing a suitable formulation for CCSD(T) using the same unconventional representation [Citation135].
There is more that should be mentioned. Working with Luc Visscher and Ken Dyall, Tim also worked on extensions of CCSD from the normal non-relativistic regime to include relativistic effects in either 2 or 4-component formalisms [Citation105, Citation125]. However, as the 1990s progressed, the focus of Tim's research moved towards deploying coupled cluster methods for high accuracy calculations for computational spectroscopy, and the range of chemical applications covered in the following sections. Where appropriate or necessary, he also employed DFT while never particularly liking it! I continued to collaborate with Tim over the following two decades on some of those issues. Roughly three to six times per year we would meet at a coffee shop in Dublin, CA, about half-way between NASA Ames and Berkeley. I have wonderful memories of those scientific as well as personal discussions, which also involved Partha Bera for over half that period. Quite a few alumni from my group will remember trips to NASA Ames, as well as the Dublin coffee shop, and the great scientific (and non-scientific) discussions we enjoyed there.
While Tim loved coupled cluster theory, he recognised that it was simply not feasible to apply (in those days) to complex systems that were of great interest to NASA scientists at the time. Specifically, Lou Allamandola put forward his PAH hypothesis: that polycyclic aromatic hydrocarbons (PAHs) which were suspected to be ubiquitous in the interstellar medium could be the carriers of the unassigned diffuse interstellar bands (DIBs) [Citation300] and very likely the unassigned IR (UIR) features also [Citation300, Citation301]. In 1999, I suggested my postdoc, So Hirata implement time-dependent DFT for excited states, and we obtained very encouraging results for radicals [Citation302, Citation303]. With an eye to future applications to the DIB problem, Tim immediately suggested that we assess the quality of TDDFT excitation energies for PAH radical cations, and these results were also beyond expectations (particularly Tim's!) [Citation148].
The very useful accuracy of TDDFT for PAH ions paved the way for a range of follow-on collaborations between Tim and me [Citation150, Citation155], exploring a range of PAH cations of potential relevance to the DIBs, where most of the calculations were performed by my Ph.D. student, Jennifer Weisman. Some of these TDDFT excitation energy calculations connected very nicely to experiments performed at NASA Ames, including a study of the oligorylenes (perylene, terrylene, quaterrylene) [Citation157], followed by polyacenes and dibenzopolyacenes whose larger members exhibit electronic transitions in the IR [Citation160], and nitrogenated PAHs (PANHs) [Citation163]. Another collaboration with Tim with a link to the PAH hypothesis was inspired by possible astrochemical connections to my group's work showing dramatic differences between PAH radicals and ions and their dimers [Citation304], Tim brought together Lou Allamandola with my postdoctoral fellow Young Min Rhee, and we performed calculations that suggested charged PAH clusters may be responsible for galactic extended red emissions or EREs [Citation162]. A perspective article was subsequently written by Hirata et al. on the advances in computational interstellar spectroscopy [Citation171].
Tim loved a good scientific debate as well as good stories, and theoretical chemistry was always well-represented in those he told. Beyond science, Tim was a devoted father and loving husband, and I got to see this at a personal level over the years. Over the years that we become friends, Tim, Julia and their boys would join Teresa and I, and our two daughters on camping trips. Tim and Julia invited us to join large-scale group camping weekends with friends such as Bill Swope (then at IBM), and Les Barnes, and their families. We were also guests at some of their legendary Christmas parties, where the Rice-Lee home was packed with friends enjoying each others' company. In more recent years, Tim, Julia and their boys, Sebastian Marcus and Trevor, were often guests at our house for Thanksgiving (sometimes including Marcus performing a few songs at the end of the evening). With Julia and Teresa, Tim and I also enjoyed many dinner evenings together, typically meeting at the half-way point, just as we did for our scientific discussions. At both professional and personal levels, I miss Tim tremendously, just as I greatly value all the time we spent together. Vale Tim.
4. Precision computational spectroscopy
In the early 2000s, Tim assumed the position of Chief of the Astrophysics Branch in the Space Sciences & Astrobiology Division. This new role further stimulated his interest in molecular IR line list computation for astronomical studies. From space-based Spitzer and Herschel (HiFi) observatory, to air-based Stratospheric Observatory For Infrared Astronomy (SOFIA) observatory, and future James Webb Space Telescope (JWST) and NGRST (or WFIRST at that time) space telescopes, Tim realised a golden age of IR astronomy was coming. He also understood well the gap between the pressing demand for line lists and the limitations of existing databases [Citation305]. The data contained in existing high-resolution IR databases (such as HITRAN2004 [Citation306]) serve as valuable references. However, they are usually localised to specific experimental conditions and spectrometer system capabilities, such as wavenumber range and intensity threshold, for detection. Due to various challenges in measuring and modelling weak bands, high-temperature scenarios or minor isotopologues, many experimental line lists lack sufficient information to meet the growing needs of IR astronomy, particularly across a wide temperature range from a few dozen Kelvin to thousands of Kelvin and/or a broad wavenumber range. To maximise the scientific outcomes of IR astronomy missions, advance our understanding of molecular spectra and improve the high-resolution spectra databases, Tim collaborated with Dr. David Schwenke to combine the strengths of high-level electronic correlation methods with the power of high-resolution experiments. Dr. Schwenke pioneered the field in his 1997 HO study [Citation307] along with Dr. Harry Partridge. Later on, Tim coined the term ‘Best Theory + Reliable High-resolution Experiment’ (BTRHE) to describe this overall strategy. As we introduced and summarised in Refs. [Citation245, Citation273], the core value of BTRHE strategy and resulting line lists is the dependable and consistent predictions that go beyond mere replication of existing data. It is worth noting that some line list projects undertaken by colleagues at ExoMol [Citation308, Citation309] and TheoReTS [Citation310] have also followed similar strategies to refine theoretical IR line lists.
The initial molecule of this endeavour was ammonia (NH). In the Summer of 2005, Tim sent an email to Professor Bowman (my Ph.D. advisor at Emory University) inquiring if he was acquainted with someone suitable for an NH
rovibrational postdoctoral project at NASA Ames. As a fresh Ph.D. with limited familiarity with potential energy surface (PES) and vibrational calculations for the isoelectronic H
O
, I (Xinchuan Huang) was surprised and filled with excitement by this exceptional NPP opportunity at NASA. Subsequently, in August 2005, I met Tim for the first time at the ACS Fall meeting in Washington, DC. To this day, I vividly recall his attire and the computer bag he carried on that particular day. He enlightened me on the significance of NH
in Titan's atmosphere, its widespread presence in the interstellar medium (ISM; such as Sgr B2), its sensitivity in spectra to the physical conditions of its surrounding environment, and the potential to eliminate all the ‘weeds’ of NH
IR absorption lines, thereby revealing more interesting ‘flowers’. As a matter of fact, Tim's fascination with ammonia had a long history as Prof. Schaefer discusses above. Back in 1987, Tim contributed to a study pertaining to the harmonic frequency, IR intensity and inversion barrier of NH
[Citation19]. In 1992, Tim reported a quartic force field (QFF) for NH
at the CCSD(T)/cc-pVQZ level [Citation75], which achieved a mean accuracy within 3 cm
of experimental values for vibrational fundamentals, except the characteristic umbrella (inversion) mode
. The QFF was also employed in Schwenke's development of a variational calculation program, VTET [Citation311], for rovibrational energy levels and transition intensities for tetratomic molecules. It has been our main program for rovibrational and line list calculations.
From 2006 to 2012, Tim's leadership has allowed us to successfully produce three NH PES of high quality, namely HSL-1 (2008) [Citation165], HSL-2 (2011) [Citation176, Citation177] and HSL-Pre3 (2012) [Citation189]. In comparison to Yurchenko's 2005 vibrational refinement (
= 0.4 cm
) on a CCSD(T)/CBS+core+rel PES [Citation312], our HSL-1 [Citation165] incorporated Diagonal Born–Oppenheimer Correction (DBOC) and higher order correlation corrections (ACPF/cc-pVTZ) on N–H stretches and achieved a
cm
for
transitions in HITRAN2004 [Citation165]. Through the inclusion of non-adiabatic corrections and the minimisation of the rotational dependence of energy level deviations, the HSL-2 (2011) PES further improved the accuracy by one order of magnitude. With a
cm
for 6002
transitions in HITRAN2008 [Citation313], HSL-2 became the first ‘spectroscopically accurate’ PES ever published for any tetratomic molecule [Citation176]. Building upon the foundation of HSL-2, the HSL-Pre3 (2012) expanded the accuracy to the range of 6300–7000 cm
by incorporating new IR experimental data obtained by our esteemed collaborators, Dr. Keeyoon Sung and Dr. Linda Brown at JPL [Citation189]. From Tim's perspective, the progression of the PES from pure ab initio to HSL-1/2/Pre3 represented an interesting development in the field, particularly the HSL-Pre3 refinement. This refinement serves as a compelling demonstration of the mutually beneficial interaction between experiment and theory, as well as the sustainable growth of semi-empirically refined PES along with new experiments.
The prediction accuracy and consistency of HSL-2 and HSL-Pre3 PES have enabled us to rectify the incorrect assignments in the band of both
and
NH
, to identify new bands from unassigned lines, and to clarify how the 2
band modelling in HITRAN2008 lost accuracy beginning at 1
a and deteriorated for K=5 and higher [Citation176, Citation177]. In the case of the
NH
isotopologue, our predictions also prompted the upgrade of the effective Hamiltonian model for rotational lines [Citation177]. Additionally, they facilitated the assignments for the 6300 cm
–7000 cm
range in 2012 [Citation189] and the assignments at 6000 cm
in 2022 [Citation278]. Even to this day (2023), HSL-Pre3 still provides the most accurate predictions for
energy levels up to 7000 cm
. For instance, a recent analysis at 6000 cm
[Citation278] demonstrated that the
deviations for
and
levels and inversion splittings are only 1/6–1/3 of the
of the UCL-C2018 line list [Citation314]. This result was determined using an experimental data based refinement of the ab initio PES that Polyansky published in 2016 [Citation315]. It should be noted that the work by UCL utilised an approximate nuclear kinetic energy operator, whereas VTET uses an exact nuclear kinetic energy operator. In summary, the extension of the BTRHE strategy from H
O to NH
was a resounding success.
The second molecule on our list was carbon dioxide, CO. As one of the greenhouse effect molecules, CO
is also dominant in the atmospheres of Mars and Venus, and also detected in outer planets, Titan, as well as exoplanets. For the record, Tim's interest in CO
can be traced back to his CCSD(T)/cc-pVT(Q)Z QFF study in 1993 [Citation81]. It is also the molecule we studied for the longest time, from 2009 to 2022. As I can remember, Tim has always been motivated to investigate any issues or celebrate findings regarding the CO
line lists. It is worth noting that the only financial support we have ever received for this CO
research was the funding that David Schwenke obtained from the Venus Express Supporting Scientist program (2006–2014). The first lesson we learned was that an ideal semi-empirical refinement should only include purely experimental and reliable high-resolution energy levels or line positions [Citation186]. In 2014, the Ames-296 K and Ames-1000K line lists [Citation210] for 13 CO
isotopologues were published, utilising the Ames-1 PES [Citation186] and a CCSD(T)/aug-cc-pVQZ dipole moment surface (DMS) [Citation200]. It also included line shape parameters for four temperature ranges: Mars, Earth, Venus and hotter (700–2000K), through a collaboration with Dr. Gamache (UML). In 2022, when CO
was first detected in the atmosphere of an exoplanet (WASP-39b, 1200K) [Citation316], the Ames-1000K(2014) line list was cited for the CO
opacity data in the PICASO 3.0 model [Citation317]. The model was utilised by the JWST Early Release Science teams [Citation318, Citation319] to analyse and simulate the transmission spectrum of WASP-39b.
Tim's commitment to enhancing the CO IR line lists for various fields such as atmospheric chemistry, remote sensing and planetary communities remained unwavering after the year 2014. He directed the second PES refinement, Ames-2, which is still considered the most accurate CO
PES to this day (2023). The Ames-2 PES laid a robust foundation for the intensity predictions in the Ames-2016 [Citation242] IR line lists. Then, he oversaw a systematic investigation to ascertain a more accurate CO
dipole moment surface. The new Ames-2021 DMS was constructed from CCSD(T)/(d-)aug-cc-pV(T/Q/5)Z dipoles extrapolated to CBS limit, boasting an averaged absolute fitting deviation
a.u. up to 15,000 cm
[Citation284]. This significantly augmented the accuracy of the Ames IR intensity predictions for the 2001i and 3001i bands, which are the primary bands monitored by the Orbiting Carbon Observatory satellite missions, OCO-2 and OCO-3 [Citation320]. When compared to three sets of high accurate experiments conducted by NIST [Citation321, Citation322] and DLR [Citation323], our Ames-2021 IR line list [Citation284] exhibited a remarkable consistency of agreements within sub-half percent for all seven OCO-2/3 bands, specifically ±1–4 permille. We have concluded that achieving such intensity accuracy necessitates both an accurate PES and an accurate DMS, and will require an even more precise PES refinement to reach 0.1% (1 permille) or better accuracy. Therefore, in May 2022, Tim proposed a new CO
research project to NASA ROSES, which also happened to be my last proposal co-writing with him, but unfortunately, it was not selected.
Since 2012, Tim has engaged in the IR line list development encompassing various molecules that potentially exert significant influence in star-forming regions and (exo-)planetary atmospheres, such as SO, HCN
HNC, and more recently, N
O, OCS and CS
. For instance, SO
constitutes one of the secondary class ‘weeds’ as categorised for the Herschel Observatory data analysis. Its
bending features are prevalent across the entire 21
range in observations towards Orion IRc2 [Citation270]. Between 2014 and 2018, we presented several Ames-296 K line lists [Citation205, Citation215, Citation237] covering the isotopologues of
S and
O, employing HITRAN-based PES refinements and a CCSD(T)
aug-cc-pV(Q+d)Z DMS. Portions of the data [Citation257] were incorporated into the SO
section of HITRAN2020 [Citation277]. The Ames PES and DMS were utilised in the ExoAmes line list study [Citation231] that the University College London (UCL) group carried out in 2016 focusing on hot
O
up to temperatures of 2000K. It is noteworthy to mention that, during the collaboration with Dr. Naseem Rangwala at NASA Ames, who was affiliated with SOFIA, Tim contributed to the identification and analysis of
band transitions of C
H
(and
CCH
), as well as the
band transitions of HCN/HNC in the EXES IR spectra obtained from the Orion hot core IRc2 [Citation248, Citation270]. Before December 2021, all the Ames-296 K line lists of the first generation for N
O included in this volume [Citation290], OCS and CS
had been successfully computed and made accessible online with Tim's guidance and support.
In recent years, Tim, David Schwenke and I collaborated to explore frontiers and new pathways to enhance the interplay between theory, experiment and modelling, aiming to improve the prediction accuracy of BTRHE molecular line lists. There has been a trend to combine the theoretical intensities of BTRHE-type line lists with more accurate line positions obtained from experiments or Effective Hamiltonian (EH) models, which requires reliable matches of energy levels and transition lines, as exemplified by the Exomol HO [Citation324] and NH
[Citation325] line lists, CO
in HITRAN2016 [Citation326], and our Ames-2021 [Citation284] line list for CO
. However, due to the inherent disparities in algorithms, it is exceedingly challenging to match for many of those lines associated with high energy levels that are crucial at elevated temperatures. To address this issue, our recent work on the AI-3000K line list for hot CO
[Citation286] has employed a novel construction algorithm. It incorporates a PES refinement based on an accurate EH model and uses it to compute billions of CO
transitions that are too weak at room temperature and are associated with high-energy regions where no experimental data is available. These transitions are then combined with the energy levels and line positions from experiment-based CDSD2019 [Citation327], plus the more reliable Ames-2021 intensities [Citation284] for lower energy transitions. The AI-3000K is a comprehensive upgrade from the Ames-1000K [Citation210], and Tim was particularly happy about the agreements we achieved with the experimental data for both line position and intensity at 1000K, when compared to other hot CO
line lists [Citation328–330]. Sadly, Tim passed away after the AI-3000K data generation and the subsequent submission to the HITEMP [Citation328] evaluation, which appears to be progressing favourably.
We also focused on the isotopologue consistency in BTRHE-type molecular IR line lists and its potential applications. Reliable and precise knowledge of isotopologue spectra may help the characterisation of ISM and planetary atmosphere environments, dynamics and chemical evolution, and the detection of isotopic anomalies, including those resulting from biological processes. Additionally, it can also help assess the potential habitability of Earth-like exoplanets in habitable zones. As our line lists are systematically constructed, any small deviations from reliable experiments should exhibit recognisable patterns that can be described using low-order approximations. Using our complete set of CO and SO
isotopologue line lists, we conducted the first quantitative analysis of the isotopic effects on transition dipole moment and Einstein A
coefficient for specific transitions, along with how these effects vary with J in a given band [Citation242, Citation260]. We observed that these patterns can be modelled using terms inversely proportional to isotope masses, with an estimated consistency across isotopologues of 0.1% or higher, surpassing that of most experimental data. Such consistency can help identify less reliable experimental data, and more practically, it also suggests the intensity prediction accuracy of minor isotopologues can be systematically calibrated if highly accurate experimental intensity data become available for a few isotopologues [Citation260]. On the other hand, to enhance the line position accuracy for rare isotopologues, we fit computed SO
pure rotational lines to experimental EH models, then compared the fitted rotational constants and quartic centrifugal distortion constants with experimental EH values. We successfully demonstrated that the differences between the fitted EH(Ames) constants and EH(expt) values for less perturbed vibrational states can also be accurately modelled using terms inversely proportional to mass. Therefore the EH constants of less abundant isotopologues can be predicted with higher accuracy based on the reliable differences determined from more abundant isotopologues. The deviations were reduced to 0.002–0.02 MHz for rotational constants (under linear approximation) and 0.02–0.05 kHz for D
(second-order fit). Compared to the original IR line list, the implementation of the BTRHE strategy at a higher level has resulted in a two-order magnitude improvement in prediction accuracy. This BTRHE+EH approach, when compared to the commonly used mass-independent scaling (a zeroth-order approximation), can be considered a significantly more accurate second-order correction.
The BTRHE+EH approach also has the potential to improve the prediction accuracy for vibrational excited states, providing that they are not strongly affected by couplings. Our study uncovered that the EH(Ames) parameters display clear, systematic and consistent patterns with respect to vibrational state energy or quanta, but the EH(expt) constants for many SO excited states lack the necessary accuracy and consistency to support second-order corrections for some quartic constants [Citation269]. The deficiencies can largely be attributed to the limited precision of experimental line positions, which typically ranges from 10
to 10
cm
(or 3–30 MHz) for mid- and near-IR spectra. While the consistency of the EH(Ames) parameters may aid in identifying unreliable EH parameters and making reliable predictions for minor isotopologues and unobserved vibrational bands, we emphasised that the highly accurate ‘hot’ MW and far-IR transitions measured with kHz (or better) precision should be strongly promoted, because it can significantly enhance the EH(expt) models in a solid and systematic manner [Citation269]. Only after that can the BTRHE prediction accuracy be satisfactorily calibrated for isotopologue and unobserved vibrational excited state series using data on a limited number of vibrational states.
From 2006 to 2022, Tim's contribution to our line list research was that of a driving force and a guiding light. He served as a vital link between our team and colleagues at Ames, such as Mark Marley, Richard Freedman, Natasha Batalha and others. Additionally, Tim fostered collaborations with scientists at the Jet Propulsion Lab (JPL), Center for Astrophysics (Harvard Smithsonian), University College London (UCL), etc., as well as the wider astronomical and modelling communities. His invaluable leadership, financial acumen, and foresight, have allowed us to function properly as the sole US group engaged in the computation of molecular IR line lists using the BTRHE-type approach. Moreover, it was Tim's unwavering commitment and advocacy at NASA Headquarters that guaranteed the U.S.A. an active engagement in this field. His passing not only poses a significant loss to the IR astronomy community but also has the potential to widen the existing gap between the United States and Europe in the realm of highly precise molecular infrared line lists, crucial for the study of exoplanetary atmospheres.
Looking back on the 16 years of working alongside Tim, he always showed great support and encouragement for me to explore new ideas, even if they took a considerable amount of time and did not yield the desired results. For example, in 2020 spring, I told Tim that I really wanted to know how many Ames and Carbon Dioxide Spectroscopic Database (CDSD) ro-vibrational energy levels of can be manually matched with some ideas or tricks that I had hoped useful at the time. He kindly agreed. Then 3 months passed before I eventually conceded that a physically meaningful match above 22,000 cm
was simply beyond our capability, due to the intricate but different (ro-)vibrational couplings in the two extrapolations. I am truly grateful to Tim for granting me ample time to delve into these ‘important but ultimately futile’ details which are challenging to visualise with clarity. On the other hand, Tim attempted many times to promote the concept of ‘limited variational line lists’ [Citation204], which involve the use of highly accurate quartic force fields and potential refinements, as well as ab initio dipole moment surfaces. It undoubtedly offers a much faster solution to the pressing needs of our astrophysics community. However, I must confess that I failed to fully grasp the significance of these insights, although the three mostly cited papers I co-authored with Tim [Citation164, Citation168, Citation179] were all about highly accurate ab initio QFFs.
I will always cherish the moments when Tim played a pivotal role in saving my scientific career on two separate occasions, and my family is deeply appreciative of his sincere advice and help. I also will miss those group dinners at P.F. Chang in the Stanford Shopping Center, as well as those times we spent together in Tim's office(s) in N245. Tim had a passion for personal interactions, but during the pandemic, we had to rely on weekly Skype meetings, which is also where I last heard his voice. In late July 2022, Tim did not feel very well, yet he still hosted Dr. Inostroza's seminar at Ames. That was the last time I saw him in person. He was an exceptional mentor and a dear friend, and I feel truly privileged to have known him.
5. Atmospheric chemistry
The ozone hole – a springtime loss of the stratospheric ozone layer – was discovered in the late 1980s, and atmospheric halocarbons were thought to be responsible for its creation. Tim, after he moved to the NASA Ames, started taking strong interest in atmospheric molecules especially halogenated nitrogen oxides of various types for their role in creating activated radicals that cause ozone depletion. It has been shown that ozone is destroyed by reactive halogen species such as , and that these species are removed from the stratosphere due to their reaction with nitrogen oxides; and that
serves as a large reservoir for chlorine removal in the stratosphere. Yet, not all of the chlorine reservoir could be accounted for from the known concentration of
in the atmosphere, and other chlorine nitrates were suspected to be responsible too. Tim's prolific publication record on various such molecules in the 1990s bear witness to his keen interest in atmospheric chemistry – publishing at least 38 papers of which Tim was the first author in 25 of them. This series of papers started with the first and efficient formulations of analytic gradients for ab initio methods, especially coupled cluster, and their applications on atmospheric molecules [Citation47, Citation48, Citation57, Citation58, Citation70, Citation78, Citation110, Citation112, Citation116, Citation127, Citation145]. Some of these papers are well known for their fundamental developmental work in quantum chemistry rather than for their atmospheric chemistry applications.
Tim's work throughout the 1990s and early 2000s proved crucial in characterising the structures and vibrational spectra of a series of XNO, XONO, and
species (X = F, Cl and Br) [Citation68, Citation69, Citation73, Citation84, Citation88, Citation92, Citation93, Citation97, Citation98, Citation100, Citation101, Citation117, Citation119, Citation121, Citation126, Citation128, Citation133, Citation141, Citation142, Citation149, Citation161]. Most notable of these series of papers were on the structure, vibrational spectra and heats of formation of
, XClO and XOCl species computed using his newly developed coupled cluster codes [Citation97, Citation104, Citation108, Citation109, Citation117, Citation143, Citation158]. While reporting harmonic frequencies were the norm at the time, Tim almost routinely published the QFF-based anharmonic vibrational fundamentals using high-level coupled cluster methods. The value of these results is evident from their large and continued citations to this day. Alistair Rendel and Dylan Jayatilaka, both postdocs at the time at NASA Ames, Joseph Francisco, Chris Dateo and Julia Rice participated in some of these projects with Tim. Towards the end of the 1990s, Srinivasan Parthiban started working as a postdoc with Tim and worked on a number of the atmospheric chemistry projects involving the NOX gases. During this time, Tim also started collaborating and discussing various aspects of application of theoretical chemistry on atmospheric sciences with Joseph Francisco, and that collaboration continued for the next two and half decades.
Around the time I (Partha Bera) was finishing my dissertation I got in touch with Tim for a postdoctoral fellowship, and after some deliberations we decided that I would write a proposal for a NASA Postdoctoral Program fellowship that combined atmospheric chemistry and astrochemistry. I had worked on the IR frequencies of fluorocarbons in the atmospheric IR window during my Ph.D. After the proposal was accepted by NPP and as I was waiting for my background check to be completed, Tim visited Fritz and me in Athens, Georgia. One day during this visit Tim, Fritz, Mrs. Schaefer, a German postdoc, and I went on a 16 mile hike along the Appalachian Trail in northern Georgia. It was during this hike we discussed the contours of my postdoc, and we decided that I will start with the global warming work as I already had published a paper in that area. A few months later I joined with Tim at NASA Ames (and started collaborating with Prof. Joe Francisco then at Purdue University) on exploring the underlying causes of high potency of some greenhouse gases. After devising a method to extract the dipole derivatives along the normal modes using the SPECTRO code [Citation331], we found that some vibrational modes are extremely potent due to their large dipole derivatives which were responsible for their high absorption efficiency. Thus we published our first paper from my postdoc on the molecular origin of global warming in JPCA [Citation170]. Using this knowledge we devised certain design principles that minimised the environmental impact of industrially used fluorocarbons and published the second paper in this series in PNAS [Citation172, Citation174]. We showed that not only are the carbon-fluorine bonds highly potent infrared absorbers but also that the way those bonds are distributed in the carbon framework dictates their efficiency. These two papers grabbed the attention of media and lately from chemical industry and formed the basis for Tim's second NASA Exceptional Scientific Achievement Medal. Later, when Sara Kokkila–Schumacher came as a summer intern, we devised a group increment scheme for vibrational intensities for hydrofluorocarbons and perfluorocarbons of different types [Citation185] for over 300 such greenhouse gases. Recently, Tim and I joined hands with Dr Jim Burkholder of NOAA to directly compute from quantum chemistry the atmospheric lifetimes, radiative efficiencies, global warming potentials and global temperature change potentials. We reported these results in a recent 2020 paper for all saturated hydrofluorocarbons smaller than five carbon atoms chains for their future usage and policy decisions [Citation266].
6. Interstellar chemistry
Tim often stated that his favourite molecule was cyclopropenylidene, c-CH
, a molecule of notable abundance and significance in the chemistry of the ISM. Such significance alone warrants selection as a favourite, but for Tim this choice was more personal. While a Ph.D. student at Berkeley, Tim along with another graduate student and Prof. Schaefer, performed a complete structural, vibrational and electronic characterisation of c-C
H
in 1985 through the use of two-configuration self-consistent-field (TCSCF) and configuration interaction singles and doubles (CISD) with both a double-ζ (DZ) and DZP basis set [Citation6]. This characterisation was absolutely essential for the laboratory detection of c-C
H
and its subsequent radioastronomical observation that same year toward Cas A, W49, and, most notably, Sgr B2(N), the molecular cloud observed towards and in the vicinity of the super-massive blackhole at the centre of the Milky Way galaxy [Citation332]. This molecule is actually so abundant that all of its major isotopologues have been observed, and its astronomical observation is nearly ubiquitous [Citation333–338]. Likely from the moment of this first detection forward through the subsequent insights that cyclopropenylidene produced in astrophysics, Tim saw the value of quantum chemistry and how it could greatly inform the study of molecules in the ISM.
When Tim began working at NASA Ames in 1989, the Infrared Space Observatory (ISO) was well under development, and the Kuiper Arborne Observatory (a converted C-141 with a hole in the side for a 91.5 cm telescope) was still producing lots of IR data. Hence, the need for reference data for observed molecules was growing. Tim saw a way that quantum chemistry could support astronomical IR observations. IR spectra are, of course, predominantly the result molecular vibrations, and Tim became, among many other things, a major champion for the computation of anharmonic vibrational frequencies for the rest of his career. Tim and I (Ryan C. Fortenberry) collaborated on a discussion for the history of these advances in recent volumes [Citation283, Citation339]. However, his contributions in this area are myriad, and much of his method development during his NASA years was geared towards making quantum chemistry more applicable and useful for astrophysical molecular observations.
Around the same time that Tim began at Ames, coupled cluster theory had emerged as a more potent treatment of electron correlation, and Tim was quick to utilise this in the definition of QFFs, fourth-order Taylor series expansions of the potential portion of the internuclear Watson Hamiltonian. He recognised that the most ‘bang for the buck’ came in using the most accurate but least costly method for this sparse but still geometrically scaling potential energy surface. The first use was for ozone which was needed to characterise properly Kuiper observations as they would pass through the ozone layer, and such unknown telluric lines needed to be removed from the observations [Citation47, Citation48]. This work was done with CCSD(T) QFFs through second-order vibrational perturbation theory (VPT2) as implemented in the Spectro program [Citation331]. Such work was remarkable for its accuracy during the time with the known features producing sub-5.0 cm agreement in most cases.
In many ways, Tim inherited the Spectro code originally developed in his postdoctoral group in Cambridge with Nick Handy [Citation331]. While Prof. Handy and his group continued to develop Spectro to some extent, Tim found new things that this code could be developed to do. Most notably, Tim and Jan Martin took the existing Fermi resonance capability and added Fermi resonance polyads where modes in resonance with a common fundamental could actually be treated in resonance with one another [Citation114, Citation340]. Such interactions increased the accuracy of the VPT2 anharmonic frequencies and allowed for more detailed prediction of gas phase fundamental vibrational frequencies and comparison to those from the laboratory or even from ISO observations.
Tim parlayed this use of CCSD(T) QFFs for VPT2 within the special capabilities of Spectro to produce fundamental vibrational frequencies for a host of molecules and, in many cases, showed where previous experimental characterisations had been incorrect including NO, N
O, CO
, NH
, H
CO, HNO, CH
, C
H
and NH
[Citation61, Citation62, Citation75, Citation81, Citation87, Citation106, Citation114, Citation123]. These greatly influenced how CCSD(T)-based QFFs and VPT2 frequencies were viewed by the community. Tim continued to work on various molecules of applicability to the ISM including revisits to cyclopropenylidene [Citation129], but as he transitioned into management, his time for science was largely coloured by his investment in his NASA colleagues and postdocs as well as his collaborators [Citation154, Citation155] including, most notably, Chris Dateo in the late 1990s [Citation138, Citation146].
Tim's work in interstellar chemistry took a resurgence with the addition of Xinchuan Huang to NASA Ames as a NASA Postdoctoral Program Fellow (NPP) in the mid-to-late 2000s and coincided with the commissioning of the Stratospheric Observatory for Infrared Astronomy (SOFIA) and the developmental stages of the James Webb Space Telescope. Xinchuan and Tim pioneered a new composite approach for producing what were then the most accurate QFFs of the time. Adding inclusions for core electron correlation, complete basis set extrapolation, higher-order electron correlation and relativity produced exceptional accuracy in the resulting VPT2 anharmonic frequencies [Citation164, Citation165, Citation168, Citation169]. Tim always wanted to know how his favourite molecule was formed [Citation40], and one of the first applications of this accurate composite method was in the isomers of CH
with his focus mainly on the cyclic form, work that he and Xinchuan did with his long-time friend Peter Taylor [Citation179, Citation180]. In fact, their spectral characterisation of c-C
H
was so accurate that it significantly informed its detection in the laboratory [Citation341], and allowed for Tim to put together a proposal for SOFIA observing time where he was given the opportunity to ride in the aircraft as part of the award even though the molecule was not detected at that time. Acetylene was, however [Citation248]. Tim also worked with Natalia Inostroza during this same time, and they classified the isomers and spectral features of HC
N isomers and isotopologues [Citation184, Citation203].
In 2009, I (Ryan Fortenberry) first met Tim when he came to visit us at Virginia Tech in a striking similarity to Partha's story. Of course, I was beside myself when I heard that people did quantum chemistry for NASA. Tim was there to work with Ed Valeev on how explicitly correlated coupled cluster theory could be used to define QFFs. This work was published the next year and provided spectral classification to HO, N
H
, NO
, and C
H
and also implied that explicitly correlated methods were not quite up to development enough for use in QFFs [Citation175], something that Tim inspired me to address and develop years later. I met Tim, again, at MQM in Berkeley in 2010 facilitated through my Ph.D. advisor, Daniel Crawford, and Tim graciously agreed to let me come visit him, Xinchuan, and Partha. In the spring of 2011, Tim and Xinchuan taught me how to do QFFs in their highly-accurate method, and I spent the next 3 years from the end of my Ph.D. studies through my postdoc at NASA Ames with Tim and into my first year at my initial faculty job doing just that. We published a slew of papers on lots of mostly tetraatomic molecules with application to interstellar chemistry [Citation181, Citation183, Citation187, Citation190, Citation192–195, Citation197, Citation199, Citation204, Citation206, Citation207, Citation211, Citation217]. These include many anions as I was obsessed with them at the time, but Tim was happy to support me. We also tried to question the supposed detection of l-C
H
, but we turned out to be wrong. It was l-C
H
. I learned a lot from that experience, and Tim helped me to see even more. It wasn't his first rodeo. We also did some variational CI calculation Morse potentials from QFFs, something a few others in the field said couldn't be done. As shown, this was a very productive time for both of us. Xinchuan was transitioning more to the accurate line lists (discussed above), and Tim encouraged and supported my work into the fundamental frequency analysis of novel, possible interstellar molecules.
Even when I disappointed him (told to me jokingly by Lou Allamandola) and left to take a faculty job after spending just a little over 1 year at Ames, he still found ways to keep our collaboration going. While I didn't work on the exact projects that he wanted, he was still incredibly encouraging of my curiosity and helped to shepherd my thoughts into what would be applicable to NASA missions. I would actually argue that we were able to get more done in such an arrangement than if I was still a postdoc at Ames. We explored SiC as a molecular thermometer with Hölger Müller [Citation221] and dove into proton-bound complexes with Joel Bowman's group [Citation220, Citation223] as well as with Joe Francisco [Citation228, Citation234] in addition to protonated acetylene (which took forever to figure out) [Citation230] which was built on his earlier work [Citation13].
During this same time, Tim also found new collaborations with Cameron Mackie and Alessandra Candian (then of the Leiden Observatory) through Xander Tielens, and Tim was instrumental in Cameron's Ph.D. research as a co-advisor with Xander. This team developed the necessary algorithm, code and infrastructure to actually be able to compute anharmonic, fundamental vibrational frequencies for polycyclic aromatic hydrocarbons (PAHs) which are believed to be the carriers for most of the broad IR features observed towards most molecular clouds. PAHs were long thought to be too big for any explicit anharmonic analysis, but Tim, Cameron and the team figured out a way through DFT to be able to compute their vibrations to notable accuracy greatly informing experiment [Citation222, Citation224, Citation232, Citation236, Citation246, Citation247]. They also worked out how to compute the cascade emission spectrum necessary for the characterisation of PAH emission spectra [Citation250, Citation251, Citation275, Citation280], work that actually is ongoing today (and included in this volume) with Tim's last postdoc, Vince Esposito of NASA Ames.
Tim and I continued to publish QFF papers together for ISM chemistry on molecules isoelectronic with cyclopropenylidene and [Citation240] sulfur species with Joe [Citation239, Citation244]. We even branched out into electronically excited states of PAH anions with a few of my students (notably Mallory Theis Green) as well as with Alessandra Candian [Citation213, Citation225, Citation233]. These publications included structural aspects and explicitly computed aromaticity as they pertain to the types of PAHs that could be observed in the ISM [Citation252, Citation281]. We found even more reasons to revisit cyclopropenylidene and its related species [Citation243, Citation249, Citation255, Citation276]. In truth, this last item turned into a bit of a mission for Tim. While the out-of-plane bending (OPB) problem in -hybridised carbons has been discussed for many years, no satisfactory solution or cause has been provided in the literature. Tim knew that if it wasn't solved, PAH spectra would not be correctly described. Such a case once more shows Tim's crossover between theory development once more crossing over between theory development and interstellar chemistry, using the latter to motivate the former. Tim wrote a review on this hoping to generate interest from the theory field [Citation272], but few were open to collaborating on this. We explored this phenomenon with several π-bonded hydrocarbons [Citation256, Citation267] and even found a way of reducing this with proper fitting techniques with a former M.S. student of mine who was finishing his Ph.D. with Prof. Schaefer, W. James Morgan. This work was actually published in this journal [Citation258] and applied to H
S
by James and Tim [Citation253]. However, the issue of non-physical reduction of OPBs for carbon atoms remains, and, on Tim's behalf, I ask for theoreticians to help us to figure out why such exists. The fact that we can't actually, correctly describe potential energy surfaces of carbon, the most common atom in chemistry, is greatly concerning; it certainly was to Tim. Tim would have been so very glad to know that it was solved or alleviated or, at the very least, being actively explored.
Tim also continued to work with me and others on computing QFFs for interesting molecules. Partha Bera even got involved in this portion of Tim's research [Citation262]. Tim, Natalia and I also published papers on isomers of [H, S, C], :CCH
, and :CNH
[Citation259, Citation263] with the deuterated isotopologue for the first of these revisited by Natalia in this volume. Additionally and recently, Tim and I (along with some of my students; see below) characterised and fully benchmarked his most accurate methods [Citation271] laying the ground work for further QFF development in my group, another gift to me from Tim. Finally, within the past few years, Tim's work has directly informed astronomical detection of vibrationally-excited HOCO
in the ISM [Citation187, Citation342], and he was even on a few SOFIA detection papers through work spear-headed by Naseem Rangwala [Citation248, Citation270]. Tim relished these more than he let on. Any quantum chemist loves to be physically validated, and what better way to do it than with astronomical observations?
The last manuscript on which Tim and I actively worked together was a seminal paper for my student, Brent Westbrook. I'd gotten the hair-brained idea many years before to reparameterise semi-empirical methods for PAH QFFs. Tim found this intriguing and carefully advised me into obtaining my first major research grant from NASA to do just this. With Tim's guidance, Brent was able to develop an entire codebase to compute anharmonic PAH spectra largely automatically [Citation343]. However, this was all built, initially at least, to treat PAHs with trained semi-empirical methods, and Tim, Brent and I (along with collaborator and friend Josh Layfield) published this work shortly before Tim's passing [Citation285]. I had no idea it would be our last paper together. Granted, Tim's inputs and thoughts are still continuing in papers in this volume as well as in others still in development. Hence, his name is still appearing in new works within the chemical literature [Citation292–295] as his heart is still present in the science that he loved so much.
However, Tim loved people more than science. He was a connector, a nexus of ideas and the people who had them. Tim initially introduced me to Joe Francisco, and we'd all worked on the HOCO paper together [Citation181], my first foray into this area. Joe and I have continued to work together publishing more than 25 papers together, about half with Tim. Natalia and I have published a half dozen together with several more in development. Vince Esposito and I are continuing to collaborate in Tim's name to apply the anharmonic PAH absorption and, especially, emission computations to JWST data. Even Xinchuan and I have published a paper together since Tim's passing. Without Tim, not only would I not know astrochemistry, I would not know these and dozens of other wonderful people who have made my life better. Tim was the conduit through us all. Faculty at universities get to cheat, as my father says, since our job is to directly impact young people. (Tim hated when I split my infinitives; I blame his years at Cambridge for that.) However, Tim has had direct impact on so many young researchers and such deep impact at that where tenured faculty would be jealous of what his name will always mean.
Tim and I wrote more than 50 papers together mostly on interstellar IR molecular spectral predictions but also in other areas all geared towards expanding our knowledge of the types of molecules lurking in the ISM. No other single collaborator of mine even comes close to this number for me; it would take someone super special for me to even want them to. His impact on my life is measured in more than papers, though. He was the Man in the Yellow Hat for this curious little monkey. Tim also gave me valuable advice on fatherhood, especially fatherhood while working in science. I still can't believe he's gone, and I wish every day that he was not. From Seneca, ‘non est ad astra mollis e terris via’, but Tim has found a way to make it look easy.
7. Astrobiology
After my (Partha) arrival at the NASA-Ames, I started to investigate the formation pathways of small PAHs and their spectroscopic signatures that was a part of my NPP proposal. This work really started while I was still in CCQC in Prof. Fritz Schaefer's group, and shortly after I arrived we published my first paper with Tim on the formation of various isomers of vinylcyanide [Citation167], and we suggested that some of the isomers could be the missing links for interstellar pyrimidine formation. Around 2009, Tim invited me to start participating in discussions with Martin in their regular meetings in Pleasanton, CA. Together we investigated in a series of papers that spanned over a decade, bottom-up synthesis of small cyclic aromatic hydrocarbons starting from the smallest of hydrocarbons [Citation182, Citation191]. We first investigated the dimeric complexes of observed ions and molecules [Citation182], and then we investigated the oxygen containing rings of pyrylium ion [Citation201]. In our investigation into the dimeric complexes of acetylene and ethylene with their ions, we showed that these ionic complexes make robust but small carbocyclic rings via covalent bond formation [Citation191]. At a conference in Leiden University, Tim and I met with Prof. Samy El-Shall and based on a discussion we had there we collaborated on a project where we showed in a joint experiment and theory paper that acetylene cations reacting with hydrogen cyanide can produce pyrimidine and pyrazine cations [Citation208]. Around this time, Tim and I were a part of a large NASA-ROSES funded project ‘Carbon in the Galaxy’ with Lou Allamandola as the PI. As a part of this project, Cesar Contreras and Farid Salama in their COSMIC chamber experiment started making small hydrocarbons by bottom-up synthesis in ionised plasma and we worked on the theory side. Tim and I along with Martin and Roberto Peverati, a postdoc with Martin at the time, published three papers deciphering the bottom-up formation pathways of hydrocarbons up to phenyl cation [Citation209, Citation214, Citation235]. In these papers, we showed that the benzene-like aromatic molecule formation in the gas-phase plasma is possible via ion-molecules type reactions of two-carbon units [Citation214]. However, the growth of larger PAHs beyond the first aromatic ring is limited. The reaction pathways involve a large number of low-lying intermediates and become quite complicated rapidly [Citation235]. In another paper, we showed that the [Citation209] ion possesses double aromaticity and can be synthesised by hydrogen atom sputtering which acts as cooling mechanism for newly formed molecules.
At one of our many meetings I casually suggested to Tim that we should merge Tim's interest in cyclopropenylidine and my interest in cyclic aromatic molecules with a nitrogen in the ring as no such molecule has been observed in the gas phase ISM yet. This seemed to intrigue Tim's interest and led us to work on the formations and spectral characterisations of some important nitrogenated three-membered ring species. The azirinyl [Citation227] cation is one in which a carbon from the ring is replaced by a nitrogen atom. After characterising the low-lying isomers of azirinyl Tim pushed us to calculate the quartic force field ro-vibrational constants and low-temperature line lists for all the isomers and isotopologues [Citation262]. Structural parameters of azirine [Citation254], a cyclic isomer of methyl cyanide, and high-quality anharmonic spectra of diazirine [Citation282], with two nitrogen atoms in the ring were then published. Interestingly, some of these calculations on azirine, diazirine and azirinyl cation were performed by three summer undergraduate interns Claire Dickerson, Kendra Noneman and Sara Kokkila-Schumacher all of whom were inspired enough, after the summer internship with Tim and I, to pursue Ph.D.s in computational chemistry in future, at University of California Los Angeles (UCLA), Carnegie Mellon, and Stanford University, respectively. Some of these astrochemistry studies involved Natalia Inostroza-Pino visiting from Chile by special invitation from Tim, an association that resulted in several papers and a visit by Tim to Chile for Natalia's astrochemistry school.
Around a year after I joined as an NPP fellow at the NASA ARC, I started growing interest in the experimental works of Michel Nuevo and Scott Sandford. During a lunch at the Megabytes cafeteria, Michel and I discussed his experiments on irradiation of nucleic acid bases in ices that piqued my interest and thus began our a decade and half long collaboration that led to several papers and funded joint grant proposals. We initiated the first ever full quantum chemical exploration of the reaction mechanisms of formation of nucleobases from their precursors in irradiated mixed molecular ices [Citation173]. After some trepidation I discussed this new project with Tim. Initially Tim was not keen as he didn't want me distracted with a new project, and tried to dissuade me as it was not part of my NPP proposal. But, as was often the case I later realised, very soon he got interested in the project, and started to enquire and advise. These reaction mechanisms exploration included working with many radicals and ions that were also large open shell systems. Tim's vast experience with developing z-averaged perturbation theory (ZAPT) was especially useful in all the projects in this series. We showed using calculations that the irradiation of pyrimidine in pure and mixed molecular water ices readily produced uracil and that the reactions proceeded via ionic and radical mechanisms [Citation173]. One of the main conclusions out of this work was that the reaction mechanism was helped by the presence of a surrounding water matrix. Additionally, we showed how for uracil, and all other nucleobases, formation was possible in the condensed phase matrices but were not favourable in the gas phase, conclusions that went beyond the experimental results, and it meant that the nucleobases could not be synthesised in the gas phase molecular clouds. We fully explained the experimental results and often suggested new experiments that led to further important conclusions. In their subsequent experiments Chris (Materese), Michel and Scott observed thymine in small quantities after prolonged irradiation experiments [Citation198]. In a joint work, we showed that the formation of thymine was rather difficult as it involved a methyl group substitution in the aromatic ring, and this was the rate limiting step in the whole mechanism [Citation212, Citation229].
After those initial nucleic acid studies, we had created a strong team with Scott Sanford, Michel Nuevo, Chris Materese doing experiments, and Tim, Martin and I doing quantum chemistry calculations. Along with Uma Gorti and Maxime Ruaud doing gas-grain simulations for protoplanetary disks, we successfully competed for a 5-year NASA Astrobiology Institute grant. We focused our attention to purine-based nucleobases along with Martin and his new postdoc Tamar Stein. Together we showed that in the laboratory irradiation conditions purine makes hypoxanthine, xanthine, isoguanine, guanine, adenine and its isomers [Citation238]. Our theoretical work led in establishing the formation routes for adenine, guanine and isoguanine all of which were observed in the irradiated laboratory samples. Using a polarised continuum model in the purine work, we established the catalytic role played by the water cluster in the formation of adenine and guanine in abiotic ices [Citation238]. In the irradiated mixed molecular ices, a large quantity of hexamethylene tetramine (HMT) along with its substituted family is produced. Using reliable density functional methods, we calculated the infrared spectra of such substituted molecules and published two experiment-theory papers. All these development and tight collaboration between Tim and Partha's theoretical exploration and Michel, Chris and Scott's experimental work, along with our previous work, led to a comprehensive review in Chemical Reviews in which we covered chemistry of interstellar medium and protoplanetary disks [Citation265]. This Chemical Review paper is very highly cited, garnering 110 citations within 3 years. Writing the Chem. Rev. paper was the last project for which we all could be in a room together before Covid-19 lockdown restrictions kicked in and forced us to work from home for an extended period of time. Very recently, Tim and I along with our exobiology colleagues explored yet more astrobiological processes such as prebiotic metabolic pathways that is yet to be published.
Over the many years of working together with Tim, we became from a mentor and a mentee to colleagues and friends. Our conversations ranged from the many nuances of electronic structure theories, scientific computing, high-resolution spectroscopy, and astrobiology to parenting, baseball, cricket, and countless other topics, and Tim was excited about all of it. I have perhaps learned more about parenting from Tim than from anyone else. I was always impressed by Tim's ability to understand the inner workings of an organisation or a scientific problem remarkably quickly. I appreciated, among many other things, his openness to new ideas, generosity, support, trust, and his encouragement for independence and initiative. I leaned heavily upon Tim's mentoring, and I miss him every day. After the Covid restrictions eased, Fritz visited us at NASA Ames in late April of 2022 for a special seminar; it was a memorable visit for many reasons. The day after Fritz's visit on 30th April 2022, I hosted a BBQ in my backyard for the group to gather to mark the end of the pandemic. Both Tim and Julia were present along with many others. It was one of the last times when I saw Tim completely healthy and joyful, as I always knew him. It was a very enjoyable evening with this group along with their families and is etched in my memory forever. Tim's scientific journey from the development of electronic structure theories to the applications of those theories to solve real-world problems related to atmospheric chemistry, high-resolution spectroscopy, astrochemistry and astrobiology demonstrated his extraordinary breadth of knowledge and open mind towards science in general.
Disclosure statement
No potential conflict of interest was reported by the author(s).
References
- T.J. Lee, D.J. Fox, H.F. Schaefer and R.M. Pitzer, Analytic second derivatives for Renner-Teller potential energy surfaces: Examples of the five distinct cases. J. Chem. Phys. 81, 356–361 (1984). doi:10.1063/1.447313.
- T.J. Lee and H.F. Schaefer, Vibrational frequencies and infrared intensities for H 2CN +, protonated HCN. J. Chem. Phys. 80, 2977–2978 (1984). doi:10.1063/1.447009.
- J. Breulet, T.J. Lee and H.F. Schaefer, Comparison between the s-cis and gauche conformers of 1,3-butadiene. J. Am. Chem. Soc. 106, 6250–6253 (1984). doi:10.1021/ja00333a024.
- T.J. Lee and H.F. Schaefer, Systematic study of molecular anions within the self-consistent-field approximation: OH −, CN −, C 2H −, NH 2−, and CH 3−. J. Chem. Phys. 83, 1784–1794 (1985). doi:10.1063/1.449367.
- G. Fitzgerald, T.J. Lee, H.F. Schaefer and R.J. Bartlett, The open-chain or chemically bonded structure of H 2O 4: The hydroperoxyl radical dimer. J. Chem. Phys. 83, 6275–6282 (1985). doi:10.1063/1.449577.
- T.J. Lee, A. Bunge and H.F. Schaefer, Toward the laboratory identification of cyclopropenylidene. J. Am. Chem. Soc. 107, 137–142 (1985). doi:10.1021/ja00287a025.
- T.J. Lee, H.F. Schaefer and E.A. Magnusson, Geometrical structures of four conformers of the phosphocenium ion, P(C 5H 5) 2+: A phosphorus sandwich? J. Am. Chem. Soc. 107, 7239–7243 (1985). doi:10.1021/ja00311a001.
- R.B. Remington, T.J. Lee and H.F. Schaefer, [5]Paracyclophane: Molecular structure and implications for aromaticity. Chem. Phys. Lett. 124, 199–201 (1986). doi:10.1016/0009-2614(86)87030-0.
- N.C. Handy, T.J. Lee and W.H. Miller, Spin-orbit and diagonal born-oppenheimer corrections for the reaction F+H 2 → HF+H. Chem. Phys. Lett. 125, 12–18 (1986). doi:10.1016/0009-2614(86)85146-6.
- Y. Yamaguchi, M.J. Frisch, T.J. Lee, H.F. Schaefer and J.S. Binkley, Analytic evaluation of infrared intensities and polarizabilities by two-configuration self-consistent field wave functions. Theor. Chim. Acta 69, 337–352 (1986). doi:10.1007/BF00527708.
- G.E. Scuseria, T.J. Lee, R.J. Saykally and H.F. Schaefer, Nitrogen quadrupole coupling constants for HCN and H 2CN +: Explanation of the absence of fine structure in the microwave spectrum of interstellar H 2CN +. J. Chem. Phys. 84, 5711–5714 (1986). doi:10.1063/1.449930.
- J.E. Rice, R.D. Amos, N.C. Handy, T.J. Lee and H.F. Schaefer, The analytic configuration interaction gradient method: Application to the cyclic and open isomers of the S3 molecule. J. Chem. Phys. 85, 963–968 (1986). doi:10.1063/1.451253.
- T.J. Lee and H.F. Schaefer, The classical and nonclassical forms of protonated acetylene, C 2H 3+: Structures, vibrational frequencies, and infrared intensities from explicitly correlated wave functions. J. Chem. Phys. 85, 3437–3443 (1986). doi:10.1063/1.451828.
- T.J. Lee, N.C. Handy, J.E. Rice, A.C. Scheiner and H.F. Schaefer, The efficient evaluation of configuration interaction analytic energy second derivatives: Application to hydrogen thioperoxide, HSOH. J. Chem. Phys. 85, 3930–3938 (1986). doi:10.1063/1.451826.
- G.E. Scuseria, T.J. Lee and H.F. Schaefer, Accelerating the convergence of the coupled-cluster approach. Chem. Phys. Lett. 130, 236–239 (1986). doi:10.1016/0009-2614(86)80461-4.
- J.E. Rice, T.J. Lee and H.F. Schaefer, Molecular structure and infrared spectrum of protonated nitrous oxide. Chem. Phys. Lett. 130, 333–336 (1986). doi:10.1016/0009-2614(86)80479-1.
- N.C. Handy, R.D. Amos, J.F. Gaw, J.E. Rice, E.D. Simandiras, T.J. Lee, R.J. Harrison, W.D. Laidig, G.B. Fitzgerald and R.J. Bartlett, in Geometrical Derivatives of Energy Surfaces and Molecular Properties, P. Jørgensen, J. Simons, edited by (D. Reidel, Dordrecht, 1986), pp. 170–191, “The evaluation of M(B)P(T)-2 second derivatives”.
- T.J. Lee, Ph.D. Thesis, University of California, Berkeley, 1986. The development and application of energy derivative methods for various types of configuration interaction wave functions in ab initio electronic structure theory.
- G.E. Scuseria, A.C. Scheiner, J.E. Rice, T.J. Lee and H.F. Schaefer, Analytic evaluation of energy gradients for the single and double excitation coupled cluster (CCSD) wave function: A comparison with configuration interaction (CISD, CISDT, and CISDTQ) results for the harmonic vibrational frequencies, infrared intensities, dipole moment, and inversion barrier of ammonia. Int. J. Quantum Chem., 495–501 (1987). doi:10.1002/(ISSN)1097-461X.
- G.E. Scuseria, A.C. Scheiner, T.J. Lee, J.E. Rice and H.F. Schaefer, The closed-shell coupled cluster single and double excitation (CCSD) model for the description of electron correlation: A comparison with configuration interaction (CISD) results. J. Chem. Phys. 86, 2881–2890 (1987). doi:10.1063/1.452039.
- T.J. Lee, J.E. Rice and H.F. Schaefer, The infrared spectrum of the acetylene radical cation C 2H 2+: A theoretical study using SCF, MCSCF, and CI methods. J. Chem. Phys. 86, 3051–3053 (1987). doi:10.1063/1.452011.
- M. Okumura, L.I. Yeh, D. Normand, J.J.H. Vandenbiesen, S.W. Bustamente, Y.T. Lee, T.J. Lee, N.C. Handy and H.F. Schaefer, Radiative decay lifetimes of CH 2−. J. Chem. Phys. 86, 3807–3815 (1987). doi:10.1063/1.451939.
- J.E. Rice, T.J. Lee, R.B. Remington, W.D. Allen, D.A. Clabo and H.F. Schaefer, [5]Paracyclophane: An important example of ring strain and aromaticity in hydrocarbon compounds. J. Am. Chem. Soc. 109, 2902–2909 (1987). doi:10.1021/ja00244a008.
- T.J. Lee, G.E. Scuseria, J.E. Rice, A.C. Scheiner and H.F. Schaefer, Comparison of single and double excitation coupled cluster and configuration interaction theories: Determination of structure and equilibrium properties for diatomic molecules. Chem. Phys. Lett. 139, 134–139 (1987). doi:10.1016/0009-2614(87)80165-3.
- T.J. Lee, Theory for externally contracted configuration interaction energy gradients. J. Chem. Phys. 87, 2825–2831 (1987). doi:10.1063/1.453070.
- A.C. Scheiner, G.E. Scuseria, J.E. Rice, T.J. Lee and H.F. Schaefer, Analytic evaluation of energy gradients for the single and double excitation coupled cluster (CCSD) wave function: Theory and application. J. Chem. Phys. 87, 5361–5373 (1987). doi:10.1063/1.453655.
- T.J. Lee, W.D. Allen and H.F. Schaefer, The analytic evaluation of energy first derivatives for two-configuration self-consistent-field configuration interaction (TCSCF-CI) wave functions: Application to ozone and ethylene. J. Chem. Phys. 87, 7062–7075 (1987). doi:10.1063/1.453352.
- E.D. Simandiras, J.E. Rice, T.J. Lee, R.D. Amos and N.C. Handy, On the necessity of f basis functions for bending frequencies. J. Chem. Phys. 88, 3187–3195 (1988). doi:10.1063/1.453963.
- E.D. Simandiras, R.D. Amos, N.C. Handy, T.J. Lee, J.E. Rice, R.B. Remington and H.F. Schaefer, Second-order perturbation theory and configuration interaction theory applied to medium-sized molecules: Cyclopropane, ethylenimine, ethylene oxide, fluoroethane, and acetaldehyde. J. Am. Chem. Soc. 110, 1388–1393 (1988). doi:10.1021/ja00213a010.
- J.E. Rice, T.J. Lee and N.C. Handy, The analytic gradient for the coupled pair functional method: Formula and application for HCl, H 2CO, and the dimer H 2CO··· HCl. J. Chem. Phys. 88, 7011–7023 (1988). doi:10.1063/1.454400.
- T.J. Lee, J.E. Rice, W.D. Allen, R.B. Remington and H.F. Schaefer, How bent can a benzene be? The molecular structure, infrared spectrum and energetics of [6]paracyclophane. Chem. Phys. 123, 1–25 (1988). doi:10.1016/0301-0104(88)87028-9.
- T.J. Lee, R.B. Remington, Y. Yamaguchi and H.F. Schaefer, The effects of triple and quadruple excitations in configuration interaction procedures for the quantum mechanical prediction of molecular properties. J. Chem. Phys. 89, 408–422 (1988). doi:10.1063/1.455482.
- T.J. Lee, J.E. Rice, R.B. Remington and H.F. Schaefer, The molecular structures and energetics of [7] paracyclophane and [8] paracyclophane: An investigation of the boundaries of aromaticity. Chem. Phys. Lett. 150, 63–70 (1988). doi:10.1016/0009-2614(88)80397-X.
- B.F. Yates, H.F. Schaefer, T.J. Lee and J.E. Rice, The infrared spectrum of F −.H 2O. J. Am. Chem. Soc. 110, 6327–6332 (1988). doi:10.1021/ja00227a008.
- T.J. Lee and J.E. Rice, An efficient closed-shell singles and doubles coupled-cluster method. Chem. Phys. Lett. 150, 406–415 (1988). doi:10.1016/0009-2614(88)80427-5.
- T.J. Lee and P.R. Taylor, A diagnostic for determining the quality of single-reference electron correlation methods. Int. J. Quantum Chem. 199–207 (1989).
- T.J. Lee, J.E. Rice, G.E. Scuseria and H.F. Schaefer, Theoretical investigations of molecules composed only of fluorine, oxygen and nitrogen: determination of the equilibrium structures of FOOF, (NO) 2 and FNNF and the transition state structure for FNNF cis-trans isomerization. Theor. Chim. Acta 75, 81–98 (1989). doi:10.1007/BF00527711.
- T.J. Lee and J.E. Rice, Silicocene, (C 5H 5) 2Si: A highly symmetric sandwich compound? J. Am. Chem. Soc. 111, 2011–2017 (1989). doi:10.1021/ja00188a010.
- C.W. Bauschlicher, S.R. Langhoff, T.J. Lee and P.R. Taylor, The effect of higher than double excitations on the F+H 2 → FH+H barrier. J. Chem. Phys. 90, 4296–4300 (1989). doi:10.1063/1.455786.
- T.J. Lee, A. Willetts, J.F. Gaw and N.C. Handy, The molecular structure and vibrational spectrum of the cyclopropenyl cation, C 3H 3+, and its deuterated isotopomers. J. Chem. Phys. 90, 4330–4340 (1989). doi:10.1063/1.456644.
- G.E. Scuseria, T.J. Lee, A.C. Scheiner and H.F. Schaefer, Ordering of the O-O stretching vibrational frequencies in ozone. J. Chem. Phys. 90, 5635–5637 (1989). doi:10.1063/1.456417.
- T.J. Lee, A high-level ab initio study of the anionic hydrogen-bonded complexes FH··· CN −, FH··· NC −, H 2O··· CN −, and H 2O··· NC −. J. Am. Chem. Soc. 111, 7362–7371 (1989). doi:10.1021/ja00201a013.
- J.E. Rice, T.J. Lee and C.M. Rohlfing, The structure of cis-butadiene. Chem. Phys. Lett. 161, 277–284 (1989). doi:10.1016/S0009-2614(89)87074-5.
- P.R. Taylor, T.J. Lee, J.E. Rice and J. Almlof, The polarizabilities of neon. Chem. Phys. Lett. 163, 359–365 (1989). doi:10.1016/0009-2614(89)85150-4.
- T.J. Lee, A.P. Rendell and P.R. Taylor, Theoretical investigations of the structures and binding energies of Be n and Mg n(n = 3−5) clusters. J. Chem. Phys. 92, 489–495 (1990). doi:10.1063/1.458570.
- A.P. Rendell, T.J. Lee and P.R. Taylor, Vibrational frequencies for Be 3 and Be 4. J. Chem. Phys. 92, 7050–7056 (1990). doi:10.1063/1.458245.
- T.J. Lee, On the energy separation between the open and cyclic forms of ozone. Chem. Phys. Lett. 169, 529–533 (1990). doi:10.1016/0009-2614(90)85642-P.
- T.J. Lee and G.E. Scuseria, The vibrational frequencies of ozone. J. Chem. Phys. 93, 489–494 (1990). doi:10.1063/1.459548.
- T.J. Lee, A.P. Rendell and P.R. Taylor, Comparison of the quadratic configuration interaction and coupled-cluster approaches to electron correlation including the effect of triple excitations. J. Phys. Chem. 94, 5463–5468 (1990). doi:10.1021/j100377a008.
- G.E. Scuseria and T.J. Lee, Comparison of coupled-cluster methods which include the effects of connected triple excitations. J. Chem. Phys. 93, 5851–5855 (1990). doi:10.1063/1.459684.
- T.J. Lee, A.P. Rendell and P.R. Taylor, Vibrations in small Mg clusters. J. Chem. Phys. 93, 6636–6641 (1990). doi:10.1063/1.458931.
- J.D. Watts, I. Cernusak, J. Noga, R. Bartlett, C.W. Bauschlicher, T.J. Lee, A.P. Rendell and P.R. Taylor, Triple and quadruple excitation contributions to the binding in Be clusters: Calibration calculations on Be 3. J. Chem. Phys. 93, 8875–8880 (1990). doi:10.1063/1.459226.
- T.J. Lee and J.E. Rice, Theoretical characterization of tetrahedral N 4. J. Chem. Phys. 94, 1215–1221 (1991). doi:10.1063/1.460029.
- T.J. Lee and A.P. Rendell, The structure and energetics of the HCN →HNC transition state. Chem. Phys. Lett. 177, 491–497 (1991). doi:10.1016/0009-2614(91)90073-I.
- J.E. Rice, P.R. Taylor, T.J. Lee and J. Almlof, The determination of accurate dipole polarizabilities α and γ for the noble gases. J. Chem. Phys. 94, 4972–4979 (1991). doi:10.1063/1.460533.
- A.P. Rendell, T.J. Lee and A. Komornicki, A parallel vectorized implementation of triple excitations in CCSD(T): Application to the binding energies of the AlH 3, AlH 2F, AlHF 2 and AlF 3 dimers. Chem. Phys. Lett. 178, 462–470 (1991). doi:10.1016/0009-2614(91)87003-T.
- A.P. Rendell and T.J. Lee, An efficient formulation and implementation of the analytic energy gradient method to the single and double excitation coupled-cluster wave function: Application to Cl 2O 2. J. Chem. Phys. 94, 6219–6228 (1991). doi:10.1063/1.460410.
- T.J. Lee and A.P. Rendell, Analytic gradients for coupled-cluster energies that include noniterative connected triple excitations: Application to cis-HONO and trans-HONO. J. Chem. Phys. 94, 6229–6236 (1991). doi:10.1063/1.460411.
- R. Lindh, J.E. Rice and T.J. Lee, The energy separation between the classical and nonclassical isomers of protonated acetylene: An extensive study in one- and n-particle space saturation. J. Chem. Phys. 94, 8008–8014 (1991). doi:10.1063/1.460134.
- M.R.A. Blomberg, P.E.M. Siegbahn, T.J. Lee, A.P. Rendell and J.E. Rice, Binding energies and bond distances of Ni(CO) x, x = 1−4: An application of coupled-cluster theory. J. Chem. Phys. 95, 5898–5905 (1991). doi:10.1063/1.461611.
- T.J. Lee, Bond distance and vibrational spectrum of the molecular cation NO 2+. Chem. Phys. Lett. 188, 154–158 (1992). doi:10.1016/0009-2614(92)85106-K.
- T.J. Lee and J.E. Rice, Ab initio study of the molecular structure and vibrational spectrum of nitric acid and its protonated forms. J. Phys. Chem. 96, 650–657 (1992). doi:10.1021/j100181a026.
- M. Sodupe, C.W. Bauschlicher and T.J. Lee, The calculation of the vibrational frequencies of CuCO +, NiCO and CuCH 3. Chem. Phys. Lett. 189, 266–272 (1992). doi:10.1016/0009-2614(92)85137-Y.
- J.E. Rice, G.E. Scuseria, T.J. Lee, P.R. Taylor and J. Almlof, Connected triple excitations in coupled-cluster calculations of hyperpolarizabilities: Neon. Chem. Phys. Lett. 191, 23–28 (1992). doi:10.1016/0009-2614(92)85362-E.
- T.J. Lee, R. Kobayashi, N.C. Handy and R.D. Amos, Comparison of the Brueckner and coupled-cluster approaches to electron correlation. J. Chem. Phys. 96, 8931–8937 (1992). doi:10.1063/1.462251.
- A.P. Rendell, T.J. Lee and R. Lindh, Quantum chemistry on parallel computer architectures: Coupled-cluster theory applied to the bending potential of fulminic acid. Chem. Phys. Lett. 194, 84–94 (1992). doi:10.1016/0009-2614(92)85747-X.
- T.J. Lee, A.P. Rendell and P.R. Taylor, The structures, binding energies and vibrational frequencies of Ca 3 and Ca 4: An application of the CCSD(T) method. Theor. Chim. Acta 83, 165–175 (1992). International Symp on AB Initio Methods in Quantum Chemistry, AMES, IA, May 10, 1991.doi:10.1007/BF01113249.
- J. Breidung, W. Schneider, W. Thiel and T.J. Lee, The vibrational frequencies of difluoroethyne. J. Chem. Phys. 97, 3498–3499 (1992). doi:10.1063/1.462984.
- T.J. Lee and J.E. Rice, FONO: A difficult case for theory and experiment. J. Chem. Phys. 97, 4223–4232 (1992). doi:10.1063/1.463925.
- T.J. Lee and J.E. Rice, Proton affinity of methyl nitrate: Less than proton affinity of nitric acid. J. Am. Chem. Soc. 114, 8247–8256 (1992). doi:10.1021/ja00047a040.
- J.A. Bentley, J.M. Bowman, B. Gazdy, T.J. Lee and C.E. Dateo, A global ab initio potential for HCN/HNC, exact vibrational energies, and comparison to experiment. Chem. Phys. Lett. 198, 563–569 (1992). doi:10.1016/0009-2614(92)85031-5.
- J.M.L. Martin, T.J. Lee, G.E. Scuseria and P.R. Taylor, Ab initio multireference study of the BN molecule. J. Chem. Phys. 97, 6549–6556 (1992). doi:10.1063/1.463684.
- T.J. Lee, C.M. Rohlfing and J.E. Rice, An extensive ab initio study of the structures, vibrational spectra, quadratic force fields, and relative energetics of three isomers of Cl 2O 2. J. Chem. Phys. 97, 6593–6605 (1992). doi:10.1063/1.463663.
- D. Jayatilaka and T.J. Lee, The form of spin orbitals for open-shell restricted Hartree-Fock reference functions. Chem. Phys. Lett. 199, 211–219 (1992). doi:10.1016/0009-2614(92)80108-N.
- J.M.L. Martin, T.J. Lee and P.R. Taylor, An accurate ab initio quartic force field for ammonia. J. Chem. Phys. 97, 8361–8371 (1992). doi:10.1063/1.463406.
- J.M.L. Martin and T.J. Lee, Accurate ab initio quartic force fields for borane and BeH 2. Chem. Phys. Lett. 200, 502–510 (1992). doi:10.1016/0009-2614(92)80082-M.
- T.J. Lee and D. Jayatilaka, An open-shell restricted Hartree-Fock perturbation theory based on symmetrical spin orbitals. Chem. Phys. Lett. 201, 1–10 (1993). doi:10.1016/0009-2614(93)85024-I.
- J.M.L. Martin and T.J. Lee, The protonation of N 2O reexamined: A case study on the reliability of various electron correlation methods for minima and transition states. J. Chem. Phys. 98, 7951–7957 (1993). doi:10.1063/1.464549.
- A.P. Rendell, T.J. Lee, A. Komornicki and S. Wilson, Evaluation of the contribution from triply excited intermediates to the fourth-order perturbation theory energy on Intel distributed memory supercomputers. Theor. Chim. Acta 84, 271–287 (1993). doi:10.1007/BF01113267.
- G.C. Schatz, S. Florance, T.J. Lee and C.W. Bauschlicher, Ab initio potential energy surface for IHI −: Simulation of IHI − photodetachment spectra. Chem. Phys. Lett. 202, 495–500 (1993). doi:10.1016/0009-2614(93)90037-2.
- J.M.L. Martin, P.R. Taylor and T.J. Lee, Accurate ab initio quartic force fields for the N 2O and CO 2 molecules. Chem. Phys. Lett. 205, 535–542 (1993). doi:10.1016/0009-2614(93)80009-E.
- T.A. Hein, W. Thiel and T.J. Lee, Ab initio study of the stability and vibrational spectra of plumbane, methylplumbane, and homologous compounds. J. Phys. Chem. 97, 4381–4385 (1993). doi:10.1021/j100119a021.
- D. Jayatilaka and T.J. Lee, Open-shell coupled-cluster theory. J. Chem. Phys. 98, 9734–9747 (1993). doi:10.1063/1.464352.
- T.J. Lee and J.E. Rice, Ab initio study of the chlorine nitrate protonation reaction: Implications for loss of ClONO 2 in the stratosphere. J. Phys. Chem. 97, 6637–6644 (1993). doi:10.1021/j100127a012.
- J.M.L. Martin, P.R. Taylor and T.J. Lee, Accurate ab initio quartic force fields for the ions HCO + and HOC +. J. Chem. Phys. 99, 286–292 (1993). (Erratum: J. Chem. Phys. 1993, 99, 9326). doi:10.1063/1.465806.
- J.M. Bowman, B. Gazdy, J.A. Bentley, T.J. Lee and C.E. Dateo, Ab initio calculation of a global potential, vibrational energies, and wave functions for HCN/HNC, and a simulation of the A~−X~ emission spectrum. J. Chem. Phys. 99, 308–323 (1993). doi:10.1063/1.465809.
- J.M.L. Martin, T.J. Lee and P.R. Taylor, An accurate ab initio quartic force field for formaldehyde and its isotopomers. J. Mol. Spectrosc. 160, 105–116 (1993). doi:10.1006/jmsp.1993.1161.
- T.J. Lee and A.P. Rendell, Ab initio characterization of ClOOH: Implications for atmospheric chemistry. J. Phys. Chem. 97, 6999–7002 (1993). doi:10.1021/j100129a014.
- R. Kobayashi, H. Koch, P. Jorgensen and T.J. Lee, Comparison of coupled-cluster and Brueckner coupled-cluster calculations of molecular properties. Chem. Phys. Lett. 211, 94–100 (1993). doi:10.1016/0009-2614(93)80057-V.
- N.E. Klepeis, A.L.L. East, A.G. Csaszar, W.D. Allen, T.J. Lee and D.W. Schwenke, The [FHCI] − molecular anion: Structural aspects, global surface, and vibrational eigenspectrum. J. Chem. Phys. 99, 3865–3897 (1993). doi:10.1063/1.466133.
- T.J. Lee, C.E. Dateo, B. Gazdy and J.M. Bowman, Accurate quartic force fields and vibrational frequencies for HCN and HNC. J. Phys. Chem. 97, 8937–8943 (1993). doi:10.1021/j100137a018.
- T.J. Lee, A coupled-cluster study of XNO (X = H, F, C): An investigation of weak X-N single bonds. J. Chem. Phys. 99, 9783–9789 (1993). doi:10.1063/1.465460.
- T.J. Lee, A coupled cluster study of HNO 2 and FNO 2. Chem. Phys. Lett. 216, 194–199 (1993). doi:10.1016/0009-2614(93)E1232-6.
- T.J. Lee, Ab initio characterization of ClNO 2, cis-ClONO, and trans-ClONO. J. Phys. Chem. 98, 111–115 (1994). doi:10.1021/j100052a019.
- R.J. Rico, T.J. Lee and M. Head-Gordon, The origin of differences between coupled cluster theory and quadratic configuration interaction for excited states. Chem. Phys. Lett. 218, 139–146 (1994). doi:10.1016/0009-2614(93)E1398-Z.
- M. Head-Gordon, R.J. Rico, M. Oumi and T.J. Lee, A doubles correction to electronic excited states from configuration interaction in the space of single substitutions. Chem. Phys. Lett. 219, 21–29 (1994). doi:10.1016/0009-2614(94)00070-0.
- T.J. Lee, A comparative coupled-cluster study of the XOCl and XClO (X = H, F, Cl) isomers: An investigation of hypervalent chlorine compounds. J. Phys. Chem. 98, 3697–3700 (1994). doi:10.1021/j100065a026.
- S.S. Prasad and T.J. Lee, Atmospheric chemistry of the reaction ClO+O 2 ↔ ClO.O 2: Where it stands, what needs to be done, and why? J. Geophys. Res. Atmos. 99, 8225–8230 (1994). doi:10.1029/93JD01911.
- T.J. Lee, A.P. Rendell, K.G. Dyall and D. Jayatilaka, Open-shell restricted Hartree-Fock perturbation theory: Some considerations and comparisons. J. Chem. Phys. 100, 7400–7409 (1994). doi:10.1063/1.466883.
- J.S. Francisco, S.P. Sander, T.J. Lee and A.P. Rendell, Structures, relative stabilities, and spectra of isomers of HClO 2. J. Phys. Chem. 98, 5644–5649 (1994). doi:10.1021/j100073a012.
- T.J. Lee, A couopled -cluster study of XON (X = H, F, Cl), and the XON ↔ XNO transition states. Chem. Phys. Lett. 223, 431–438 (1994). doi:10.1016/0009-2614(94)00498-6.
- A.P. Rendell and T.J. Lee, Coupled-cluster theory employing approximate integrals: An approach to avoid the input/output and storage bottlenecks. J. Chem. Phys. 101, 400–408 (1994). doi:10.1063/1.468148.
- C.E. Dateo, T.J. Lee and D.W. Schwenke, An accurate quartic force field and vibrational frequencies for HNO and DNO. J. Chem. Phys. 101, 5853–5859 (1994). doi:10.1063/1.467301.
- T.J. Lee, C.W. Bauschlicher, C.E. Dateo and J.E. Rice, The molecular structure of Cis-FONO. Chem. Phys. Lett. 228, 583–588 (1994). doi:10.1016/0009-2614(94)00988-0.
- L. Visscher, K.G. Dyall and T.J. Lee, Kramers-restricted closed-shell CCSD theory. Int. J. Quantum Chem. 411–419 (1995). doi:10.1002/(ISSN)1097-461X.
- T.J. Lee, J.M.L. Martin and P.R. Taylor, An accurate ab-initio quartic force field and vibrational frequencies for CH 4 and isotopomers. J. Chem. Phys. 102, 254–261 (1995). doi:10.1063/1.469398.
- P. Jensen, Y. Li, G. Hirsch, R.J. Buenker, T.J. Lee and I.N. Kozin, Fourfold clusters of rovibrational energies in H 2Te studied with an ab initio potential energy function. Chem. Phys. 190, 179–189 (1995). doi:10.1016/0301-0104(94)00262-9.
- T.J. Lee, A coupled-cluster study of the molecular structure, vibrational spectrum, and heats of formation of XONO 2 (X = H, F, CL). J. Phys. Chem. 99, 1943–1948 (1995). doi:10.1021/j100007a025.
- T.J. Lee and S.C. Racine, A coupled-cluster study of the molecular structure, vibrational spectrum and relative energies of the XCN and XNC (X = F, Cl) isomers. Mol. Phys. 84, 717–725 (1995). doi:10.1080/00268979500100491.
- A.M. Grana, T.J. Lee and M. Head-Gordon, Ab initio calculations of singlet and triplet excited states of chlorine nitrate and nitric acid. J. Phys. Chem. 99, 3493–3502 (1995). doi:10.1021/j100011a015.
- T.J. Lee, S.C. Racine, J.E. Rice and A.P. Rendell, On the orbital contribution to analytical derivatives of perturbation theory energies. Mol. Phys. 85, 561–571 (1995). doi:10.1080/00268979500101301.
- P. Borowski, B.O. Roos, S.C. Racine, T.J. Lee and S. Carter, The ozonide anion: A theoretical study. J. Chem. Phys. 103, 266–273 (1995). doi:10.1063/1.469639.
- R. Lindh, T.J. Lee, A. Bernhardsson, B.J. Persson and G. Karlstrom, Extended ab initio and theoretical thermodynamics studies of the Bergman reaction and the energy splitting of the singlet o-, m-, and p-benzynes. J. Am. Chem. Soc. 117, 7186–7194 (1995). doi:10.1021/ja00132a019.
- J.M.L. Martin, T.J. Lee, P.R. Taylor and J.P. Francois, The anharmonic force field of ethylene, C 2H 4, by means of accurate ab initio calculations. J. Chem. Phys. 103, 2589–2602 (1995). doi:10.1063/1.469681.
- T.J. Lee, M. Head-Gordon and A.P. Rendell, Investigation of a diagnostic for perturbation theory: Comparison to the T1 diagnostic of coupled-cluster theory. Chem. Phys. Lett. 243, 402–408 (1995). doi:10.1016/0009-2614(95)00875-5.
- T.J. Lee, Ab initio characterization of triatomic bromine molecules of potential interest in stratospheric chemistry. J. Phys. Chem. 99, 15074–15080 (1995). doi:10.1021/j100041a024.
- T.J. Lee, J.M.L. Martin, C.E. Dateo and P.R. Taylor, Accurate ab initio quartic force fields, vibrational frequencies, and heats of formation for FCN, FNC, ClCN, and ClNC. J. Phys. Chem. 99, 15858–15863 (1995). doi:10.1021/j100043a025.
- T.J. Lee and G.E. Scuseria, in Quantum Mechanical Electronic Structure Calculations with Chemical Accuracy, edited by S. R. Langhoff (Kluwer Academic Publishers, Dordrecht, 1995), pp. 47–108. “Achieving Chemical Accuracy with Coupled-Cluster Theory”.
- T.J. Lee and C.E. Dateo, The heat of formation of HNO. J. Chem. Phys. 103, 9110–9111 (1995). doi:10.1063/1.470022.
- J.M.L. Martin, D.W. Schwenke, T.J. Lee and P.R. Taylor, Is there evidence for detection of cyclic C 4 in IR spectra? An accurate ab initio computed quartic force field. J. Chem. Phys. 104, 4657–4663 (1996). doi:10.1063/1.471212.
- T.J. Lee and J.S. Francisco, The proton affinity of HOBr. Chem. Phys. Lett. 251, 400–404 (1996). doi:10.1016/0009-2614(96)00112-1.
- T.D. Crawford, H.F. Schaefer and T.J. Lee, On the energy invariance of open-shell perturbation theory with respect to unitary transformations of molecular orbitals. J. Chem. Phys. 105, 1060–1069 (1996). doi:10.1063/1.471951.
- J.M.L. Martin and T.J. Lee, Accurate ab initio quartic force field and vibrational frequencies of the NH 4+ ion and its deuterated forms. Chem. Phys. Lett. 258, 129–135 (1996). doi:10.1016/0009-2614(96)00679-3.
- J.M.L. Martin and T.J. Lee, The atomization energy and proton affinity of NH 3: An ab initio calibration study. Chem. Phys. Lett. 258, 136–143 (1996). doi:10.1016/0009-2614(96)00658-6.
- L. Visscher, T.J. Lee and K.G. Dyall, Formulation and implementation of a relativistic unrestricted coupled-cluster method including noniterative connected triples. J. Chem. Phys. 105, 8769–8776 (1996). doi:10.1063/1.472655.
- T.J. Lee, Ab initio characterization of HBrO 2 isomers: Implications for stratospheric bromine chemistry. Chem. Phys. Lett. 262, 559–566 (1996). doi:10.1016/S0009-2614(96)01178-5.
- T.J. Lee, J.E. Rice and C.E. Dateo, The varying nature of fluorine-oxygen bonds. Mol. Phys. 89, 1359–1372 (1996). doi:10.1080/00268979609482546.
- T.J. Lee, Characterization of BrNO 2, cis-BrONO, and trans-BrONO: Implications for atmospheric chemistry. J. Phys. Chem. 100, 19847–19852 (1996). doi:10.1021/jp962605g.
- C.E. Dateo and T.J. Lee, An accurate ab initio quartic force field and vibrational frequencies for cyclopropenylidene. Spectrochim. Acta A 53, 1065–1077 (1997). doi:10.1016/S1386-1425(96)01871-9.
- T.J. Lee, Preface for Special issue – Ab initio and ab initio derived force fields: State of the science. Spectrochim. Acta A 53, R7–R9 (1997). doi:10.1016/S1386-1425(97)00033-4.
- J.M.L. Martin, P.R. Taylor and T.J. Lee, The harmonic frequencies of benzene: A case for atomic natural orbital basis sets. Chem. Phys. Lett. 275, 414–422 (1997). doi:10.1016/S0009-2614(97)00735-5.
- B. Persson, P.R. Taylor and T.J. Lee, Ab initio geometry, quartic force field, and vibrational frequencies for P 4. J. Chem. Phys. 107, 5051–5057 (1997). doi:10.1063/1.474868.
- T.J. Lee, C.W. Bauschlicher and D. Jayatilaka, A challenge for density functional theory: the XONO and XNO 2 (X = F, Cl, and Br) molecules. Theor. Chem. Acc. 97, 185–194 (1997). doi:10.1007/s002140050252.
- M. Oumi, D. Maurice, T.J. Lee and M. Head-Gordon, A diagnostic for the applicability of the CIS and CIS(D) excitation energy methods. Chem. Phys. Lett. 279, 151–157 (1997). doi:10.1016/S0009-2614(97)01028-2.
- T.D. Crawford, T.J. Lee and H.F. Schaefer, A new spin-restricted triple excitation correction for coupled cluster theory. J. Chem. Phys. 107, 7943–7950 (1997). doi:10.1063/1.475081.
- M.E. Alikhani, C.E. Dateo and T.J. Lee, Density functional and coupled-cluster study an the HNO →HON transition state. J. Chem. Phys. 107, 8208–8209 (1997). doi:10.1063/1.475123.
- T.D. Crawford, T.J. Lee, N.C. Handy and H.F. Schaefer, Spin-restricted Brueckner orbitals for coupled-cluster wavefunctions. J. Chem. Phys. 107, 9980–9984 (1997). doi:10.1063/1.475302.
- T.J. Lee and C.E. Dateo, Ab initio quartic force fields for anions:: A benchmark study on 16OH −, 18OH −, and 16OD −. J. Chem. Phys. 107, 10373–10380 (1997). doi:10.1063/1.474200.
- M. Head-Gordon and T.J. Lee, in Recent Advances in Coupled Cluster Methods, edited by R. J. Bartlett (World Scientific, Singapore, 1997), pp. 221–253. “Single Reference Coupled Cluster and Perturbation Theories of Electronic Excitation Energies”.
- J.M.L. Martin, T.J. Lee and P.R. Taylor, A purely ab initio spectroscopic quality quartic force field for acetylene. J. Chem. Phys. 108, 676–691 (1998). doi:10.1063/1.475429.
- S. Parthiban and T.J. Lee, Ab initio investigation of the atmospheric molecule bromine nitrate: Equilibrium structure, vibrational spectrum, and heat of formation. J. Chem. Phys. 109, 525–530 (1998). doi:10.1063/1.476589.
- J.S. Francisco, S. Parthiban and T.J. Lee, Adiabatic electron affinity and ionization potential for BrO radical. J. Chem. Phys. 109, 10818–10822 (1998). doi:10.1063/1.477778.
- T.J. Lee, C.E. Dateo and J.E. Rice, An analysis of chlorine and bromine oxygen bonding and its implications for stratospheric chemistry. Mol. Phys. 96, 633–643 (1999). doi:10.1080/00268979909483000.
- T.J. Lee and M. Head-Gordon, Preface for Special Issue – Theoretical spectroscopy: State of the science. Spectrochim. Acta A 55, 431–432 (1999). doi:10.1016/S1386-1425(98)00251-0.
- T.J. Lee, S. Parthiban and M. Head-Gordon, Accurate calculations on excited states: New theories applied to the −X, −XO, and −XO2 ( X=Cl and Br) chromophores and implications for stratospheric bromine chemistry. Spectrochim. Acta A 55, 561–574 (1999). doi:10.1016/S1386-1425(98)00262-5.
- T.J. Lee and C.E. Dateo, Accurate spectroscopic characterization of 12 C14N −, 13 C14N −, and 12 C15N −. Spectrochim. Acta A 55, 739–747 (1999). doi:10.1016/S1386-1425(98)00276-5.
- J.M.L. Martin, K.K. Baldridge and T.J. Lee, Accurate ab initio anharmonic force field and heat of formation for silane. Mol. Phys. 97, 945–953 (1999). doi:10.1080/00268979909482896.
- S. Hirata, T.J. Lee and M. Head-Gordon, Time-dependent density functional study on the electronic excitation energies of polycyclic aromatic hydrocarbon radical cations of naphthalene, anthracene, pyrene, and perylene. J. Chem. Phys. 111, 8904–8912 (1999). doi:10.1063/1.480235.
- S. Parthiban and T.J. Lee, Theoretical study of XONO 2 (X=Br, OBr, O 2Br): Implications for stratospheric bromine chemistry. J. Chem. Phys. 113, 145–152 (2000). doi:10.1063/1.481781.
- J.L. Weisman, T.J. Lee and M. Head-Gordon, Electronic spectra and ionization potentials of a stable class of closed shell polycyclic aromatic hydrocarbon cations. Spectrochim. Acta A 57, 931–945 (2001). doi:10.1016/S1386-1425(00)00454-6.
- T.J. Lee and C.E. Dateo, Towards the synthesis of the high energy density material T d N 4: Excited electronic states. Chem. Phys. Lett. 345, 295–302 (2001). doi:10.1016/S0009-2614(01)00866-1.
- T.J. Lee and J.M.L. Martin, An accurate quartic force field, fundamental frequencies, and binding energy for the high energy density material T dN 4. Chem. Phys. Lett. 357, 319–325 (2002). doi:10.1016/S0009-2614(02)00550-X.
- G. Chaban, W. Huo and T.J. Lee, Theoretical study of infrared and Raman spectra of hydrated magnesium sulfate salts. J. Chem. Phys. 117, 2532–2537 (2002). doi:10.1063/1.1489997.
- T.J. Lee, C.E. Dateo, M. Rubio and B.O. Roos, An accurate quartic force field and fundamental frequencies for the ozonide anion: A rare positive anharmonicity for the antisymmetric stretch. Coll. Czech. Chem. Comm. 68, 189–201 (2003). doi:10.1135/cccc20030189.
- J.L. Weisman, T.J. Lee, F. Salama and M. Head-Gordon, Time-dependent density functional theory calculations of large compact polycyclic aromatic hydrocarbon cations: Implications for the diffuse interstellar bands. Astrophys. J. 587, 256–261 (2003). doi:10.1086/apj.2003.587.issue-1.
- T.J. Lee, Comparison of the T1 and D1 diagnostics for electronic structure theory: A new definition for the open-shell D1 diagnostic. Chem. Phys. Lett. 372, 362–367 (2003). doi:10.1016/S0009-2614(03)00435-4.
- T. Halasinski, J.L. Weisman, R. Ruiterkamp, T.J. Lee, F. Salama and M. Head-Gordon, Electronic absorption spectra of neutral perylene (C 20H 12), terrylene (C 30H 16), and quaterrylene (C 40H 20) and their positive and negative ions: Ne matrix-isolation spectroscopy and time-dependent density functional theory calculations. J. Phys. Chem. A 107, 3660–3669 (2003). doi:10.1021/jp027394w.
- S. Parthiban, T.J. Lee, S. Guha and J.S. Francisco, Theoretical study of chlorine nitrates: Implications for stratospheric chlorine chemistry. J. Am. Chem. Soc. 125, 10446–10458 (2003). doi:10.1021/ja010297g.
- T.J. Lee and C.E. Dateo, in High Accuracy Potentials for Quantum Dynamics, edited by A. Miani, J. Tennyson, T. van Mourik (CCP6, Daresbury Laboratory, Daresbury, UK, 2003), pp. 77–80. “Accurate Coupled-Cluster Potential Energy Surfaces: Large Calculations on Cyclopropenylidene Anharmonicities”.
- J.L. Weisman, A. Mattioda, T.J. Lee, D.M. Hudgins, L.J. Allamandola, C.W. Bauschlicher and M. Head-Gordon, Electronic transitions in the IR: Matrix isolation spectroscopy and electronic structure theory calculations on polyacenes and dibenzopolyacenes. Phys. Chem. Chem. Phys. 7, 109–118 (2005). doi:10.1039/b415502e.
- T.J. Lee, C. Mejia, G. Beran and M. Head-Gordon, Search for stratospheric bromine reservoir species: Theoretical study of the photostability of mono-, tri-, and pentacoordinated bromine compounds. J. Phys. Chem. A 109, 8133–8139 (2005). doi:10.1021/jp050997y.
- Y.M. Rhee, T.J. Lee, M.S. Gudipati, L.J. Allamandola and M. Head-Gordon, Charged polycyclic aromatic hydrocarbon clusters and the galactic extended red emission. Proc. Natl. Acad. Sci. USA 104, 5274–5278 (2007). doi:10.1073/pnas.0609396104.
- A.L. Mattioda, L. Rutter, J. Parkhill, M. Head-Gordon, T.J. Lee and L.J. Allamandola, Near-infrared spectroscopy of nitrogenated polycyclic aromatic hydrocarbon cations from 0.7 to 2.5 μm. Astrophys. J. 680, 1243–1255 (2008). doi:10.1086/529161.
- X. Huang and T.J. Lee, A procedure for computing accurate ab initio quartic force fields:: Application to HO2+ and H2O. J. Chem. Phys. 129, 044312 (2008). doi:10.1063/1.2957488.
- X. Huang, D.W. Schwenke and T.J. Lee, An accurate global potential energy surface, dipole moment surface, and rovibrational frequencies for NH3. J. Chem. Phys. 129, 214304 (2008). doi:10.1063/1.3025885.
- T.J. Lee, X. Huang and C.E. Dateo, The effect of approximating some molecular integrals in coupled-cluster calculations: Fundamental frequencies and rovibrational spectroscopic constants for isotopologues of cyclopropenylidene. Mol. Phys. 107, 1139–1152 (2009). doi:10.1080/00268970902769455.
- P.P. Bera, T.J. Lee and H.F. Schaefer, III, Are isomers of the vinyl cyanide ion missing links for interstellar pyrimidine formation? J. Chem. Phys. 131, 074303 (2009). doi:10.1063/1.3206298.
- X. Huang and T.J. Lee, Accurate ab initio quartic force fields for NH2− and CCH − and rovibrational spectroscopic constants for their isotopologs. J. Chem. Phys. 131, 104301 (2009). doi:10.1063/1.3212560.
- X. Huang, D.W. Schwenke and T.J. Lee, An approach to include the effects of diffuse functions in potential energy surface calculations. J. Phys. Chem. A 113, 11954–11962 (2009). doi:10.1021/jp9036364.
- P.P. Bera, J.S. Francisco and T.J. Lee, Identifying the molecular origin of Global Warming. J. Phys. Chem. A 113, 12694–12699 (2009). doi:10.1021/jp905097g.
- S. Hirata, P.-D. Fan, M. Head-Gordon, M. Kamiya, M. Keceli, T.J. Lee, T. Shiozaki, J. Szczepanski, M. Vala, E.F. Valeev and K. Yagi, Computational interstellar Chemistry. in Recent Advances in Spectroscopy: Theoretical, Astrophysical and Experimental Perspectives (2010), pp. 21–30. doi:10.1007/978-3-642-10322-3_3.
- P.P. Bera, J.S. Francisco and T.J. Lee, Design strategies to minimize the radiative efficiency of global warming molecules. Proc. Natl. Acad. Sci. USA 107, 9049–9054 (2010). doi:10.1073/pnas.0913590107.
- P.P. Bera, M. Nuevo, S.N. Milam, S.A. Sandford and T.J. Lee, Mechanism for the abiotic synthesis of uracil via UV-induced oxidation of pyrimidine in pure H2O ices under astrophysical conditions. J. Chem. Phys. 133, 104303 (2010). doi:10.1063/1.3478524.
- P.P. Bera, J.S. Francisco and T.J. Lee, Reply to Wallington et al.: Differences in electronic structure of global warming molecules lead to different molecular properties. Proc. Natl. Acad. Sci. USA 107, E180 (2010).
- X. Huang, E.F. Valeev and T.J. Lee, Comparison of one-particle basis set extrapolation to explicitly correlated methods for the calculation of accurate quartic force fields, vibrational frequencies, and spectroscopic constants: Application to H2O, N2H +, NO2+, and C2 H2. J. Chem. Phys. 133, 244108 (2010). doi:10.1063/1.3506341.
- X. Huang, D.W. Schwenke and T.J. Lee, Rovibrational spectra of ammonia. I. Unprecedented accuracy of a potential energy surface used with nonadiabatic corrections. J. Chem. Phys. 134, 044320 (2011). doi:10.1063/1.3541351.
- X. Huang, D.W. Schwenke and T.J. Lee, Rovibrational spectra of ammonia. II. Detailed analysis, comparison, and prediction of spectroscopic assignments for 14 NH3, 15 NH3, and 14 ND3. J. Chem. Phys. 134, 044321 (2011). doi:10.1063/1.3541352.
- X. Huang, D.W. Schwenke and T.J. Lee, Construction of spectroscopically accurate IR linelists for NH3 and CO2. in Proceedings of the 2010 NASA Laboratory Astrophysics Workshop (2011), p. C37.
- X. Huang, P.R. Taylor and T.J. Lee, Highly accurate quartic force fields, vibrational frequencies, and spectroscopic constants for cyclic and linear C3 H3+. J. Phys. Chem. A 115, 5005–5016 (2011). doi:10.1021/jp2019704.
- X. Huang and T.J. Lee, Spectroscopic constants for 13C and deuterium isotopologues of cyclic and linear C3 H3+. Astrophys. J. 736, 33 (2011). doi:10.1088/0004-637X/736/1/33.
- R.C. Fortenberry, X. Huang, J.S. Francisco, T.D. Crawford and T.J. Lee, The trans-HOCO radical: Quartic force fields, vibrational frequencies, and spectroscopic constants. J. Chem. Phys. 135, 134301 (2011). doi:10.1063/1.3643336.
- P.P. Bera, M. Head-Gordon and T.J. Lee, Initiating molecular growth in the interstellar medium via dimeric complexes of observed ions and molecules. Astron. Astrophys. 535, A74 (2011). doi:10.1051/0004-6361/201117103.
- R.C. Fortenberry, X. Huang, J.S. Francisco, T.D. Crawford and T.J. Lee, Vibrational frequencies and spectroscopic constants from quartic force fields for cis-HOCO: The radical and the anion. J. Chem. Phys. 135, 214303 (2011). doi:10.1063/1.3663615.
- N. Inostroza, X. Huang and T.J. Lee, Accurate ab initio quartic force fields of cyclic and bent HC2N isomers. J. Chem. Phys. 135, 244310 (2011). doi:10.1063/1.3671389.
- S.I. Kokkila, P.P. Bera, J.S. Francisco and T.J. Lee, A group increment scheme for infrared absorption intensities of greenhouse gases. J. Mol. Struct. 1009, 89–95 (2012). doi:10.1016/j.molstruc.2011.11.048.
- X. Huang, D.W. Schwenke, S.A. Tashkun and T.J. Lee, An isotopic-independent highly accurate potential energy surface for CO2 isotopologues and an initial 12 C16 O2 infrared line list. J. Chem. Phys. 136, 124311 (2012). doi:10.1063/1.3697540.
- R.C. Fortenberry, X. Huang, J.S. Francisco, T.D. Crawford and T.J. Lee, Quartic force field predictions of the fundamental vibrational frequencies and spectroscopic constants of the cations HOCO + and DOCO +. J. Chem. Phys. 136, 234309 (2012). doi:10.1063/1.4729309.
- J.M. Tadic, G.K. Moortgat, P.P. Bera, M. Loewenstein, E.L. Yates and T.J. Lee, Photochemistry and photophysics of n-butanal, 3-methylbutanal, and 3,3-dimethylbutanal: Experimental and theoretical study. J. Phys. Chem. A 116, 5830–5839 (2012). doi:10.1021/jp208665v.
- K. Sung, L.R. Brown, X. Huang, D.W. Schwenke, T.J. Lee, S.L. Coy and K.K. Lehmann, Extended line positions, intensities, empirical lower state energies and quantum assignments of NH3 from 6300 to 7000 cm −1. J. Quant. Spectrosc. Radiat. Transf. 113, 1066–1083 (2012). doi:10.1016/j.jqsrt.2012.02.037.
- R.C. Fortenberry, X. Huang, J.S. Francisco, T.D. Crawford and T.J. Lee, Fundamental vibrational frequencies and spectroscopic constants of HOCS +, HSCO +, and isotopologues via quartic force fields. J. Phys. Chem. A 116, 9582–9590 (2012). doi:10.1021/jp3073206.
- P.P. Bera, M. Head-Gordon and T.J. Lee, Association mechanisms of unsaturated C2 hydrocarbons with their cations: Acetylene and ethylene. Phys. Chem. Chem. Phys. 15, 2012–2023 (2013). doi:10.1039/C2CP43740F.
- R.C. Fortenberry, T.D. Crawford and T.J. Lee, The possible interstellar anion CH2CN −: Spectroscopic constants, vibrational frequencies, and other considerations. Astrophys. J. 762, 121 (2013). doi:10.1088/0004-637X/762/2/121.
- X. Huang, R.C. Fortenberry and T.J. Lee, Spectroscopic constants and vibrational frequencies for l- C3H + and isotopologues from highly accurate quartic force fields: The detection of l- C3H + in the Horsehead nebula PDR questioned. Astrophys. J. Lett. 768, L25 (2013). doi:10.1088/2041-8205/768/2/L25.
- R.C. Fortenberry, X. Huang, A. Yachmenev, W. Thiel and T.J. Lee, On the use of quartic force fields in variational calculations. Chem. Phys. Lett. 574, 1–12 (2013). doi:10.1016/j.cplett.2013.03.078.
- R.C. Fortenberry, X. Huang, T.D. Crawford and T.J. Lee, High-accuracy quartic force field calculations for the spectroscopic constants and vibrational frequencies of 1 1A′ l- C3H −: A possible link to lines observed in the Horsehead nebula photodissociation region. Astrophys. J. 772, 39 (2013). doi:10.1088/0004-637X/772/1/39.
- X. Huang, R.C. Fortenberry, Y. Wang, J.S. Francisco, T.D. Crawford, J.M. Bowman and T.J. Lee, Dipole surface and infrared intensities for the cis- and trans-HOCO and DOCO radicals. J. Phys. Chem. A 117, 6932–6939 (2013). doi:10.1021/jp3102546.
- X. Huang, R.C. Fortenberry and T.J. Lee, Protonated nitrous oxide, NNOH +: Fundamental vibrational frequencies and spectroscopic constants from quartic force fields. J. Chem. Phys. 139, 084313 (2013). doi:10.1063/1.4819069.
- C.K. Materese, M. Nuevo, P.P. Bera, T.J. Lee and S.A. Sandford, Thymine and other prebiotic molecules produced from the ultraviolet photo-irradiation of pyrimidine in simple astrophysical ice analogs. Astrobiology 13, 948–962 (2013). doi:10.1089/ast.2013.1044.
- R.C. Fortenberry, X. Huang, T.D. Crawford and T.J. Lee, The 1 3A′ HCN and 1 3A′ HCO + vibrational frequencies and spectroscopic constants from quartic force fields. J. Phys. Chem. A 117, 9324–9330 (2013). doi:10.1021/jp309243s.
- X. Huang, R.S. Freedman, S.A. Tashkun, D.W. Schwenke and T.J. Lee, Semi-empirical 12 C16 O2 IR line lists for simulations up to 1500 K and 20,000 cm −1. J. Quant. Spectrosc. Radiat. Transf. 130, 134–146 (2013). doi:10.1016/j.jqsrt.2013.05.018.
- P.P. Bera, M. Head-Gordon and T.J. Lee, Relative energies, structures, vibrational frequencies, and electronic spectra of pyrylium cation, an oxygen-containing carbocyclic ring isoelectronic with benzene, and its isomers. J. Chem. Phys. 139, 174302 (2013). doi:10.1063/1.4826138.
- R.C. Fortenberry, T.D. Crawford and T.J. Lee, Vibrational frequencies and spectroscopic constants for 1 3A′ HNC and 1 3A′ HOC + from high-accuracy quartic force fields. J. Phys. Chem. A 117, 11339–11345 (2013). doi:10.1021/jp408750h.
- N. Inostroza, R.C. Fortenberry, X. Huang and T.J. Lee, Rovibrational spectroscopic constants and fundamental vibrational frequencies for isotopologues of cyclic and bent singlet HC2N isomers. Astrophys. J. 778, 160 (2013). doi:10.1088/0004-637X/778/2/160.
- R.C. Fortenberry, X. Huang, D.W. Schwenke and T.J. Lee, Limited rotational and rovibrational line lists computed with highly accurate quartic force fields and ab initio dipole surfaces. Spectrochim. Acta A 119, 76–83 (2014). doi:10.1016/j.saa.2013.03.092.
- X. Huang, D.W. Schwenke and T.J. Lee, Highly accurate potential energy surface, dipole moment surface, rovibrational energy levels, and infrared line list for 32 S16 O2 up to 8000 cm −1. J. Chem. Phys. 140, 114311 (2014). doi:10.1063/1.4868327.
- R.C. Fortenberry, X. Huang, M.C. McCarthy, T.D. Crawford and T.J. Lee, Fundamental vibrational frequencies and spectroscopic constants of cis- and trans-HOCS, HSCO, and isotopologues via quartic force fields. J. Phys. Chem. B 118, 6498–6510 (2014). doi:10.1021/jp412362h.
- R.C. Fortenberry, X. Huang, T.D. Crawford and T.J. Lee, Quartic force field rovibrational analysis of protonated acetylene, C2 H3+, and its isotopologues. J. Phys. Chem. A 118, 7034–7043 (2014). doi:10.1021/jp506441g.
- A.M. Hamid, P.P. Bera, T.J. Lee, S.G. Aziz, A.O. Alyoubi and M.S. El-Shall, Evidence for the formation of pyrimidine cations from the sequential reactions of hydrogen cyanide with the acetylene radical cation. J. Phys. Chem. Lett. 5, 3392–3398 (2014). doi:10.1021/jz501648q.
- R. Peverati, P.P. Bera, T.J. Lee and M. Head-Gordon, Formation and stability of C6 H3+ isomers. J. Phys. Chem. A 118, 10109–10116 (2014). doi:10.1021/jp5081862.
- X. Huang, R.R. Gamache, R.S. Freedman, D.W. Schwenke and T.J. Lee, Reliable infrared line lists for 13 CO2 isotopologues up to E′=18,000cm−1 and 1500 K, with line shape parameters. J. Quant. Spectrosc. Radiat. Transf. 147, 134–144 (2014). doi:10.1016/j.jqsrt.2014.05.015.
- R.C. Fortenberry, X. Huang, T.D. Crawford and T.J. Lee, Quantum chemical rovibrational data for the interstellar detection of c- C3H −. Astrophys. J. 796, 139 (2014). doi:10.1088/0004-637X/796/2/139.
- S.A. Sandford, P.P. Bera, T.J. Lee, C.K. Materese and M. Nuevo, In Photoinduced Phenomena in Nucleic Acids II: DNA Fragments and Phenomenological Aspects, edited by M. Barbatti, A. Borin, S. Ullrich (Topics in Current Chemistry, 2014), Vol. 356, pp 123–164. “Photosynthesis and photo-stability of nucleic acids in prebiotic extraterrestrial environments”.
- M.L. Theis, A. Candian, A.G.G.M. Tielens, T.J. Lee and R.C. Fortenberry, Electronically excited states of PANH anions. Phys. Chem. Chem. Phys. 17, 14761–14772 (2015). doi:10.1039/C5CP01354B.
- P.P. Bera, R. Peverati, M. Head-Gordon and T.J. Lee, Hydrocarbon growth via ion-molecule reactions: Computational studies of the isomers of C4 H2+, C6 H2+ and C6 H4+ and their formation paths from acetylene and its fragments. Phys. Chem. Chem. Phys. 17, 1859–1869 (2015). doi:10.1039/C4CP04480K.
- X. Huang, D.W. Schwenke and T.J. Lee, Empirical infrared line lists for five SO2 isotopologues: 32/33/34/36 S16 O2 and 32 S18 O2. J. Mol. Spectrosc. 311, 19–24 (2015). doi:10.1016/j.jms.2015.01.010.
- C.J. Mackie, A. Candian, X. Huang, T.J. Lee and A.G.G.M. Tielens, Linear transformation of anharmonic molecular force constants between normal and Cartesian coordinates. J. Chem. Phys. 142, 244107 (2015). doi:10.1063/1.4922891.
- R.C. Fortenberry and T.J. Lee, Rovibrational and energetic analysis of the hydroxyethynyl anion (CCOH −). Mol. Phys. 113, 2012–2017 (2015). doi:10.1080/00268976.2015.1031841.
- N. Rangwala, X. Huang, S. Colgan, T. Lee and R. Fortenberry, Identifying new molecules from comparison of Herschel-HIFI spectra with ab initio computational spectra. in Proceedings of the International Astronomical Union (2015), E15.
- X. Huang, D.W. Schwenke and T.J. Lee, SO2 and CO2 IR line lists for atmospheric modeling on Venus and exoplanets. in Proceedings of the International Astronomical Union (2015), E16.
- R.C. Fortenberry, Q. Yu, J.S. Mancini, J.M. Bowman, T.J. Lee, T.D. Crawford, W.F. Klemperer and J.S. Francisco, Communication: Spectroscopic consequences of proton delocalization in OCHCO +. J. Chem. Phys. 143, 071102 (2015). doi:10.1063/1.4929345.
- R.C. Fortenberry, T.J. Lee and H.S.P. Mueller, Excited vibrational level rotational constants for SiC2: A sensitive molecular diagnostic for astrophysical conditions. Mol. Astrophys. 1, 13–19 (2015). doi:10.1016/j.molap.2015.07.001.
- E. Maltseva, A. Petrignani, A. Candian, C.J. Mackie, X. Huang, T.J. Lee, A.G. Tielens, J. Oomens and W.J. Buma, High-resolution IR absorption spectroscopy of polycyclic aromatic hydrocarbons: The realm of anharmonicity. Astrophys. J. 814, 23 (2015). (Erratum: Astrophys. J. 2016, 820, 81).doi:10.1088/0004-637X/814/1/23.
- Q. Yu, J.M. Bowman, R.C. Fortenberry, J.S. Mancini, T.J. Lee, T.D. Crawford, W. Klemperer and J.S. Francisco, Structure, anharmonic vibrational frequencies, and intensities of NNHNN +. J. Phys. Chem. A 119, 11623–11631 (2015). doi:10.1021/acs.jpca.5b09682.
- C.J. Mackie, A. Candian, X. Huang, E. Maltseva, A. Petrignani, J. Oomens, W.J. Buma, T.J. Lee and A.G.G.M. Tielens, The anharmonic quartic force field infrared spectra of three polycyclic aromatic hydrocarbons: Naphthalene, anthracene, and tetracene. J. Chem. Phys. 143, 224314 (2015). doi:10.1063/1.4936779.
- D.S.N. Parker, T. Yang, B.B. Dangi, R.I. Kaiser, P.P. Bera and T.J. Lee, Low temperature formation of nitrogen-substituted polycyclic aromatic hydrocarbons (PANHs)-barrierless routes to dihydro(iso)quinolines. Astrophys. J. 815, 115 (2015). doi:10.1088/0004-637X/815/2/115.
- M.L. Theis, A. Candian, A.G.G.M. Tielens, T.J. Lee and R.C. Fortenberry, Electronically excited states of anisotropically extended singly-deprotonated PAH anions. J. Phys. Chem. A 119, 13048–13054 (2015). doi:10.1021/acs.jpca.5b10421.
- S.I.L. Kokkila-Schumacher, P.P. Bera and T.J. Lee, Characterization of the azirinyl cation and its isomers. J. Phys. Chem. A 120, 1275–1282 (2016). doi:10.1021/acs.jpca.5b12373.
- R.C. Fortenberry, J.S. Francisco and T.J. Lee, Toward the astronomical detection of the proton-bound complex NN-HCO +: implications for the spectra of protoplanetary disks. Astrophys. J. 819, 141 (2016). doi:10.3847/0004-637X/819/2/141.
- P.P. Bera, M. Nuevo, C.K. Materese, S.A. Sandford and T.J. Lee, Mechanisms for the formation of thymine under astrophysical conditions and implications for the origin of life. J. Chem. Phys. 144, 144308 (2016). doi:10.1063/1.4945745.
- R.C. Fortenberry, E. Roueff and T.J. Lee, Inclusion of 13C and D in protonated acetylene. Chem. Phys. Lett. 650, 126–129 (2016). doi:10.1016/j.cplett.2016.02.068.
- D.S. Underwood, J. Tennyson, S.N. Yurchenko, X. Huang, D.W. Schwenke, T.J. Lee, S. Clausen and A. Fateev, ExoMol molecular line lists – XIV: The rotation-vibration spectrum of hot SO2. Mon. Not. R. Astron. Soc. 459, 3890–3899 (2016). doi:10.1093/mnras/stw849.
- C.J. Mackie, A. Candian, X. Huang, E. Maltseva, A. Petrignani, J. Oomens, A.L. Mattioda, W.J. Buma, T.J. Lee and A.G.G.M. Tielens, The anharmonic quartic force field infrared spectra of five non-linear polycyclic aromatic hydrocarbons: Benz[a]anthracene, chrysene, phenanthrene, pyrene, and triphenylene. J. Chem. Phys. 145, 084313 (2016). doi:10.1063/1.4961438.
- R.C. Fortenberry, M.M. Moore and T.J. Lee, Excited state trends in bidirectionally expanded closed-shell PAH and PANH anions. J. Phys. Chem. A 120, 7327–7334 (2016). doi:10.1021/acs.jpca.6b06654.
- R.C. Fortenberry, T.J. Lee and J.S. Francisco, Quantum chemical analysis of the CO-HNN + proton-bound complex. J. Phys. Chem. A 120, 7745–7752 (2016). doi:10.1021/acs.jpca.6b07515.
- R. Peverati, P.P. Bera, T.J. Lee and M. Head-Gordon, Insights into hydrocarbon chain and aromatic ring formation in the interstellar medium: computational study of the isomers of C4 H3+, C6 H3+ and C6 H5+ and their formation pathways. Astrophys. J. 830, 128 (2016). doi:10.3847/0004-637X/830/2/128.
- E. Maltseva, A. Petrignani, A. Candian, C.J. Mackie, X. Huang, T.J. Lee, A.G.G.M. Tielens, J. Oomens and W.J. Buma, High-resolution IR absorption spectroscopy of polycyclic aromatic hydrocarbons in the 3 μm region: Role of periphery. Astrophys. J. 831, 58 (2016). doi:10.3847/0004-637X/831/1/58.
- X. Huang, D.W. Schwenke and T.J. Lee, Ames 32 S16 O18O line list for high-resolution experimental IR analysis. J. Mol. Spectrosc. 330, 101–111 (2016). doi:10.1016/j.jms.2016.08.013.
- P.P. Bera, T. Stein, M. Head-Gordon and T.J. Lee, Mechanisms of the formation of adenine, guanine, and their analogues in UV-irradiated mixed NH3: H2O molecular ices containing purine. Astrobiology 17, 771–785 (2017). doi:10.1089/ast.2016.1614.
- R.C. Fortenberry, R. Thackston, J.S. Francisco and T.J. Lee, Toward the laboratory identification of the not-so-simple NS2 neutral and anion isomers. J. Chem. Phys. 147, 074303 (2017). doi:10.1063/1.4985901.
- R.C. Fortenberry, T.J. Lee and X. Huang, Towards completing the cyclopropenylidene cycle: rovibrational analysis of cyclic N3+, CNN, HCNN +, and CNC −. Phys. Chem. Chem. Phys. 19, 22860–22869 (2017). doi:10.1039/C7CP04257D.
- R.R. Gamache, C. Roller, E. Lopes, I.E. Gordon, L.S. Rothman, O.L. Polyansky, N.F. Zobov, A.A. Kyuberis, J. Tennyson, S.N. Yurchenko, A.G. Császár, T. Furtenbacher, X. Huang, D.W. Schwenke, T.J. Lee, B.J. Drouin, S.A. Tashkun, V.I. Perevalov and R.V. Kochanov, Total internal partition sums for 166 isotopologues of 51 molecules important in planetary atmospheres: Application to HITRAN2016 and beyond. J. Quant. Spectrosc. Radiat. Transf. 203, 70–87 (2017). doi:10.1016/j.jqsrt.2017.03.045.
- X. Huang, D.W. Schwenke, R.S. Freedman and T.J. Lee, Ames-2016 line lists for 13 isotopologues of CO2: Updates, consistency, and remaining issues. J. Quant. Spectrosc. Radiat. Transf. 203, 224–241 (2017). doi:10.1016/j.jqsrt.2017.04.026.
- R.C. Fortenberry, T.J. Lee and J.P. Layfield, Communication: The failure of correlation to describe carbon=carbon bonding in out-of-plane bends. J. Chem. Phys. 147, 221101 (2017). doi:10.1063/1.5013026.
- R.C. Fortenberry, J.S. Francisco and T.J. Lee, Quantum Chemical Rovibrational Analysis of the HOSO Radical. J. Phys. Chem. A 121, 8108–8114 (2017). doi:10.1021/acs.jpca.7b08121.
- X. Huang, D.W. Schwenke and T.J. Lee, Accurate and consistent prediction of molecular IR Line Lists based on ab initio theory and high-resolution experimental data. in Workshop on Astrophysical Opacities, edited by Claudio Mendoza, Sylvaine Turck-Chiéze, and James Colgan, 2018, pp. 155–165. (Astronomical Society of the Pacific Conference Series, Vol. 515).
- C.J. Mackie, A. Candian, X. Huang, E. Maltseva, A. Petrignani, J. Oomens, W.J. Buma, T.J. Lee and A.G.G.M. Tielens, The anharmonic quartic force field infrared spectra of hydrogenated and methylated PAHs. Phys. Chem. Chem. Phys. 20, 1189–1197 (2018). doi:10.1039/C7CP06546A.
- E. Maltseva, C.J. Mackie, A. Candian, A. Petrignani, X. Huang, T.J. Lee, A.G.G.M. Tielens, J. Oomens and W.J. Buma, High-resolution IR absorption spectroscopy of polycyclic aromatic hydrocarbons in the 3 μm region: Role of hydrogenation and alkylation. Astron. Astrophys. 610, A65 (2018). doi:10.1051/0004-6361/201732102.
- N. Rangwala, S.W.J. Colgan, R. Le Gal, K. Acharyya, X. Huang, T.J. Lee, E. Herbst, M. Richter, A. Boogert and M. McKelvey, High spectral resolution SOFIA/EXES observations of C2 H2 toward Orion IRc2. Astrophys. J. 856, 9 (2018). doi:10.3847/1538-4357/aaab66.
- R.C. Fortenberry, C.M. Novak and T.J. Lee, Rovibrational analysis of c- SiC2 H2: Further evidence for out-of-plane bending issues in correlated methods. J. Chem. Phys. 149, 024303 (2018). doi:10.1063/1.5043166.
- C.J. Mackie, T. Chen, A. Candian, T.J. Lee and A.G.G.M. Tielens, Fully anharmonic infrared cascade spectra of polycyclic aromatic hydrocarbons. J. Chem. Phys. 149, 134302 (2018). doi:10.1063/1.5038725.
- T. Chen, C. Mackie, A. Candian, T.J. Lee and A.G.G.M. Tielens, Anharmonicity and the infrared emission spectrum of highly excited polycyclic aromatic hydrocarbons. Astron. Astrophys. 618, A49 (2018). doi:10.1051/0004-6361/201833731.
- R.C. Fortenberry, C.M. Novak, T.J. Lee, P.P. Bera and J.E. Rice, Identifying molecular structural aromaticity for hydrocarbon classification. ACS OMEGA 3, 16035–16039 (2018). doi:10.1021/acsomega.8b02734.
- W.J. Morgan, X. Huang, H.F. Schaefer, III and T.J. Lee, Astrophysical sulfur in diffuse and dark clouds: The fundamental vibrational frequencies and spectroscopic constants of hydrogen sulfide cation ( H2S +). Mon. Not. R. Astron. Soc. 480, 3483–3490 (2018). doi:10.1093/mnras/sty2134.
- C.E. Dickerson, P.P. Bera and T.J. Lee, Characterization of azirine and its structural isomers. J. Phys. Chem. A 122, 8898–8904 (2018). doi:10.1021/acs.jpca.8b07788.
- R.C. Fortenberry, C.M. Novak, J.P. Layfield, E. Matito and T.J. Lee, Overcoming the failure of correlation for out-of-plane motions in a simple aromatic: Rovibrational quantum chemical analysis of c-C 3H 2. J. Chem. Theor. Comput. 14, 2155–2164 (2018). doi:10.1021/acs.jctc.8b00164.
- D. Agbaglo, T.J. Lee, R. Thackston and R.C. Fortenberry, A small molecule with PAH vibrational properties and a detectable rotational spectrum: c-(C) C3 H2, cyclopropenylidenyl carbene. Astrophys. J. 871, 236 (2019). doi:10.3847/1538-4357/aaf85a.
- X. Huang, D.W. Schwenke and T.J. Lee, Quantitative validation of Ames IR intensity and new line lists for 32/33/34 S16 O2, 32 S18 O2 and 16 O32 S18O. J. Quant. Spectrosc. Radiat. Transf. 225, 327–336 (2019). doi:10.1016/j.jqsrt.2018.11.039.
- W.J. Morgan, R.C. Fortenberry, H.F. Schaefer, III and T.J. Lee, Vibrational analysis of the ubiquitous interstellar molecule cyclopropenylidene (c- C3 H2): the importance of numerical stability. Mol. Phys. 118, 221101 (2020). doi:10.1080/00268976.2019.1589007.
- R.C. Fortenberry, T.J. Lee and N. Inostroza-Pino, The possibility of CNH2+ within Titan's atmosphere: Rovibrational analysis of CNH2+ and CCH2. ICARUS 321, 260–265 (2019). doi:10.1016/j.icarus.2018.11.026.
- X. Huang, D.W. Schwenke and T.J. Lee, Isotopologue consistency of semi-empirically computed infrared line lists and further improvement for rare isotopologues: CO2 and SO2 case studies. J. Quant. Spectrosc. Radiat. Transf. 230, 222–246 (2019). doi:10.1016/j.jqsrt.2019.03.002.
- P.P. Bera, S.A. Sandford, T.J. Lee and M. Nuevo, The calculated infrared spectra of functionalized hexamethylenetetramine (HMT) molecules. Astrophys. J. 884, 64 (2019). doi:10.3847/1538-4357/ab3c4f.
- P.P. Bera, X. Huang and T.J. Lee, Highly accurate quartic force field and rovibrational spectroscopic constants for the azirinyl cation (c- C2 NH2+) and its isomers. J. Phys. Chem. A 124, 362–370 (2020). doi:10.1021/acs.jpca.9b10290.
- N. Inostroza-Pino, C.Z. Palmer, T.J. Lee and R.C. Fortenberry, Theoretical rovibrational characterization of the cis/trans-HCSH and H2SC isomers of the known interstellar molecule thioformaldehyde. J. Mol. Spectrosc. 369, 111273 (2020). doi:10.1016/j.jms.2020.111273.
- J. Hendrix, P.P. Bera, T.J. Lee and M. Head-Gordon, Cation, anion, and radical isomers of C4 H4N: Computational characterization and implications for astrophysical and planetary environments. J. Phys. Chem. A 124, 2001–2013 (2020). doi:10.1021/acs.jpca.9b11305.
- S.A. Sandford, M. Nuevo, P.P. Bera and T.J. Lee, Prebiotic astrochemistry and the formation of molecules of astrobiological interest in interstellar clouds and protostellar disks. Chem. Rev. 120, 4616–4659 (2020). doi:10.1021/acs.chemrev.9b00560.
- J.B. Burkholder, P. Marshall, P.P. Bera, J.S. Francisco and T.J. Lee, Climate metrics for C 1- C4 hydrofluorocarbons (HFCs). J. Phys. Chem. A 124, 4793–4800 (2020). doi:10.1021/acs.jpca.0c02679.
- B.R. Westbrook, W.A. Del Rio, T.J. Lee and R.C. Fortenberry, Overcoming the out-of-plane bending issue in an aromatic hydrocarbon: The anharmonic vibrational frequencies of c-(CH) C3 H2+. Phys. Chem. Chem. Phys. 22, 12951–12958 (2020). doi:10.1039/D0CP01889A.
- T. Stein, P.P. Bera, T.J. Lee and M. Head-Gordon, Molecular growth upon ionization of van der Waals clusters containing HCCH and HCN is a pathway to prebiotic molecules. Phys. Chem. Chem. Phys. 22, 20337–20348 (2020). doi:10.1039/D0CP03350B.
- X. Huang, D.W. Schwenke and T.J. Lee, Exploring the limits of the Data-Model-Theory synergy: “Hot” MW transitions for rovibrational IR studies. J. Mol. Struct. 1217, 128260 (2020). doi:10.1016/j.molstruc.2020.128260.
- S. Nickerson, N. Rangwala, S.W.J. Colgan, C. DeWitt, X. Huang, K. Acharyya, M. Drozdovskaya, R.C. Fortenberry, E. Herbst and T.J. Lee, The first mid-infrared detection of HNC in the interstellar medium: probing the extreme environment toward the Orion hot core. Astrophys. J. 907, 51 (2021). doi:10.3847/1538-4357/abca36.
- M.B. Gardner, B.R. Westbrook, R.C. Fortenberry and T.J. Lee, Highly-accurate quartic force fields for the prediction of anharmonic rotational constants and fundamental vibrational frequencies. Spectrochim. Acta A 248, 119184 (2021). doi:10.1016/j.saa.2020.119184.
- T.J. Lee and R.C. Fortenberry, The unsolved issue with out-of-plane bending frequencies for C=C multiply bonded systems. Spectrochim. Acta A 248, 119148 (2021). doi:10.1016/j.saa.2020.119148.
- X. Huang, D.W. Schwenke and T.J. Lee, What it takes to compute highly accurate rovibrational line lists for use in astrochemistry. Acc. Chem. Res. 54, 1311–1321 (2021). doi:10.1021/acs.accounts.0c00624.
- L.J. Allamandola, C. Boersma, T.J. Lee, J.D. Bregman and P. Temi, PAH Spectroscopy from 1 to 5 μm. Astrophys. J. Lett. 917, L35 (2021). doi:10.3847/2041-8213/ac17f0.
- C.J. Mackie, A. Candian, T.J. Lee and A.G.G.M. Tielens, Modeling the infrared cascade spectra of small PAHs: the 11.2 μm band. Theor. Chem. Acc. 140, 124 (2021). doi:10.1007/s00214-021-02807-z.
- B.R. Westbrook, D.J. Patel, J.D. Dallas, G.C. Swartzfager, T.J. Lee and R.C. Fortenberry, Fundamental vibrational frequencies and spectroscopic constants of substituted cyclopropenylidene (c- C3HX, X = F, Cl, CN). J. Phys. Chem. A 125, 8860–8868 (2021). doi:10.1021/acs.jpca.1c06576.
- I.E. Gordon, L.S. Rothman, R.J. Hargreaves, R. Hashemi, E.V. Karlovets, F.M. Skinner, E.K. Conway, C. Hill, R.V. Kochanov, Y. Tan, P. Wcislo, A.A. Finenko, K. Nelson, P.F. Bernath, M. Birk, V. Boudon, A. Campargue, K.V. Chance, A. Coustenis, B.J. Drouin, J.-M. Flaud, R.R. Gamache, J.T. Hodges, D. Jacquemart, E.J. Mlawer, A.V. Nikitin, V.I. Perevalov, M. Rotger, J. Tennyson, G.C. Toon, H. Tran, V.G. Tyuterev, E.M. Adkins, A. Baker, A. Barbe, E. Canè, A.G. Császár, A. Dudaryonok, O. Egorov, A.J. Fleisher, H. Fleurbaey, A. Foltynowicz, T. Furtenbacher, J.J. Harrison, J.-M. Hartmann, V.-M. Horneman, X. Huang, T. Karman, J. Karns, S. Kassi, I. Kleiner, V. Kofman, F. Kwabia-Tchana, N.N. Lavrentieva, T.J. Lee, D.A. Long, A.A. Lukashevskaya, O.M. Lyulin, V.Y. Makhnev, W. Matt, S.T. Massie, M. Melosso, S.N. Mikhailenko, D. Mondelain, H.S.P. Müller, O.V. Naumenko, A. Perrin, O.L. Polyansky, E. Raddaoui, P.L. Raston, Z.D. Reed, M. Rey, C. Richard, R. Tóbiás, I. Sadiek, D.W. Schwenke, E. Starikova, K. Sung, F. Tamassia, S.A. Tashkun, J. Vander Auwera, I.A. Vasilenko, A.A. Vigasin, G.L. Villanueva, B. Vispoel, G. Wagner, A. Yachmenev and S.N. Yurchenko, The HITRAN2020 molecular spectroscopic database. J. Quant. Spectrosc. Radiat. Transf. 277, 107949 (2022). doi:10.1016/j.jqsrt.2021.107949.
- X. Huang, K. Sung, G.C. Toon, D.W. Schwenke and T.J. Lee, A collaborative 14 NH3 IR spectroscopic analysis at 6000 cm −1. J. Quant. Spectrosc. Radiat. Transf. 280, 108076 (2022). doi:10.1016/j.jqsrt.2022.108076.
- O. Berné, É. Habart, E. Peeters, A. Abergel, E.A. Bergin, J. Bernard-Salas, E. Bron, J. Cami, S. Cazaux, E. Dartois, A. Fuente, J.R. Goicoechea, K.D. Gordon, Y. Okada, T. Onaka, M. Robberto, M. Röllig, A.G.G.M. Tielens, S. Vicente, M.G. Wolfire, F. Alarcon, C. Boersma, A.élie Canin, R. Chown, D. Dicken, D. Languignon, R.L. Gal, M.W. Pound, B. Trahin, T. Simmer, A. Sidhu, D. Van De Putte, S. Cuadrado, C. Guilloteau, A. Maragkoudakis, B.R. Schefter, T.ébaut Schirmer, I. Aleman, L. Allamandola, R. Auchettl, G. Antonio Baratta, S. Bejaoui, P.P. Bera, G. Bilalbegovic, J.H. Black, F. Boulanger, J. Bouwman, B. Brandl, P. Brechignac, S. Brunken, A. Burkhardt, A. Candian, J. Cernicharo, M. Chabot, S. Chakraborty, J. Champion, S.W.J. Colgan, I.R. Cooke, A. Coutens, N.L.J. Cox, K. Demyk, J. Donovan Meyer, Cécile Engrand, S. Foschino, P. Garcia-Lario, L. Gavilan, M. Gerin, M. Godard, C.A. Gottlieb, P. Guillard, A. Gusdorf, P. Hartigan, J. He, E. Herbst, L. Hornekaer, C. Jaeger, E. Janot-Pacheco, C. Joblin, M. Kaufman, F. Kemper, S. Kendrew, M.S. Kirsanova, P. Klaassen, C. Knight, S. Kwok, A. Labiano, T.S.-Y. Lai, T.J. Lee, B. Lefloch, F.Le Petit, A.Li Hendrik Linz, C.J. Mackie, S.C. Madden, J. Mascetti, B.A. McGuire, P. Merino, E.R. Micelotta, K. Misselt, J.A. Morse, G. Mulas, N. Neelamkodan, R. Ohsawa, A. Omont, R. Paladini, M.E. Palumbo, A. Pathak, Y.J. Pendleton, A. Petrignani, T. Pino, E. Puga, N. Rangwala, M. Rapacioli, A. Ricca, J. Roman-Duval, J. Roser, E. Roueff, G. Rouille, F. Salama, D.A. Sales, K. Sandstrom, P. Sarre, E. Sciamma-O'Brien, K. Sellgren, M.J. Shannon, S.S. Shenoy, D. Teyssier, R.D. Thomas, A. Togi, L. Verstraete, A.N. Witt, A. Wootten, N. Ysard, H. Zettergren, Y. Zhang, Z.E. Zhang and J. Zhen, PDRs4All: A JWST early release science program on radiative feedback from massive stars. Publ. Astronom. Soc. Pacific 134, 054301 (2022). doi:10.1088/1538-3873/ac604c.
- C.J. Mackie, A. Candian, T.J. Lee and A.G.G.M. Tielens, Anharmonicity and the IR emission spectrum of neutral interstellar PAH molecules. J. Phys. Chem. A 126, 3198–3209 (2022). doi:10.1021/acs.jpca.2c01849.
- L. Alvarez-Thon, T.J. Lee, R.C. Fortenberry and N. Inostroza-Pino, Quantification of molecular aromaticity as a predictive factor of astrophysical significance. Astron. Astrophys. 662, A106 (2022). doi:10.1051/0004-6361/202243253.
- P.P. Bera, K.K. Noneman and T.J. Lee, Energy landscape and structural and spectroscopic characterization of diazirine and its cyclic isomers. J. Phys. Chem. A 126, 4700–4708 (2022). doi:10.1021/acs.jpca.2c01444.
- R.C. Fortenberry and T.J. Lee, in Vibrational Dynamics of Molecules, edited by J.M. Bowman (World Scientific, 2022), pp. 235–295. “Vibrational and Rovibrational Spectroscopy Applied to Astrochemistry”.
- X. Huang, D.W. Schwenke, R.S. Freedman and T.J. Lee, Ames-2021 CO2 dipole moment surface and IR line lists: Toward 0.1% uncertainty for CO2 IR intensities. J. Phys. Chem. A 126, 5940–5964 (2022). doi:10.1021/acs.jpca.2c01291.
- B.R. Westbrook, J.P. Layfield, T.J. Lee and R.C. Fortenberry, Reparameterized semi-empirical methods for computing anharmonic vibrational frequencies of multiply-bonded hydrocarbons. Electron. Struct. 4, 045003 (2022). doi:10.1088/2516-1075/aca458.
- X. Huang, R.S. Freedman, S. Tashkun, D.W. Schwenke and T.J. Lee, AI-3000K Infrared line list for hot CO2. J. Mol. Spectrosc. 392, 111748 (2023). doi:10.1016/j.jms.2023.111748.
- A.K. Lemmens, C.J. Mackie, A. Candian, T.M.J. Lee, A.G.G.M. Tielens, A.M. Rijs and W.J. Buma, Size distribution of polycyclic aromatic hydrocarbons in space: An old new light on the 11.2/3.3 μm intensity ratio. Faraday Discuss. 245, 380–390 (2023). doi:10.1039/D2FD00180B.
- S. Nickerson, N. Rangwala, S.W.J. Colgan, C. DeWitt, J.S. Monzon, X. Huang, K. Acharyya, M.N. Drozdovskaya, R.C. Fortenberry, E. Herbst and T.J. Lee, The mid-infrared molecular inventory toward Orion IRc2. Astrophys. J. 945, 26 (2023). doi:10.3847/1538-4357/aca6e8.
- S. Bejaoui, P.P. Bera, F. Salama and T. Lee, Cavity ring-down spectroscopy of anthracene, 9-methylanthracene, and 2-methylanthracene in supersonic expansion. J. Phys. Chem. A 127, 2717–2730 (2023). doi:10.1021/acs.jpca.2c08994.
- X. Huang, D.W. Schwenke and T.J. Lee, Highly accurate potential energy surface and dipole moment surface for nitrous oxide and 296K infrared line lists for 14 N216O and minor isotopologues. Mol. Phys., e2232892 (2023). doi:10.1080/00268976.2023.2232892.
- J. Hendrix, P.P. Bera, T.J. Lee and M. Head-Gordon, Quantum chemical exploration of the binding motifs and binding energies of neutral molecules, radicals and ions with small water clusters: Characterisation and astrochemical implications. Mol. Phys., e2252100 (2023). doi:10.1080/00268976.2023.2252100.
- E. Habart, E. Peeters, O. Berné, B. Trahin, A. Canin, R. Chown, A. Sidhu, D. Van De Putte, F. Alarcón, I. Schroetter, E. Dartois, S. Vicente, A. Abergel, E.A. Bergin, J. Bernard-Salas, C. Boersma, E. Bron, J. Cami, S. Cuadrado, D. Dicken, M. Elyajouri, A. Fuente, J.R. Goicoechea, K.D. Gordon, L. Issa, C. Joblin, O. Kannavou, B. Khan, O. Lacinbala, D. Languignon, R. Le Gal, A. Maragkoudakis, R. Meshaka, Y. Okada, T. Onaka, S. Pasquini, M.W. Pound, M. Robberto, M. Röllig, B. Schefter, T. Schirmer, B. Tabone, A.G.G.M. Tielens, M.G. Wolfire, M. Zannese, N. Ysard, M.-A. Miville-Deschenes, I. Aleman, L. Allamandola, R. Auchettl, G.A. Baratta, S. Bejaoui, P.P. Bera, J.H. Black, F. Boulanger, J. Bouwman, B. Brandl, P. Brechignac, S. Brünken, M. Buragohain, R. Burkhardt, A. Candian, S. Cazaux, J. Cernicharo, M. Chabot, S. Chakraborty, J. Champion, S.W.J. Colgan, I.R. Cooke, A. Coutens, N.L.J. Cox, K. Demyk, J. Donovan Meyer, S. Foschino, L. Gavilan, M. Gerin, C.A. Gottlieb, P. Guillard, A. Gusdorf, P. Hartigan, J. He, E. Herbst, L. Hornekaer, C. Jäger, E. Janot-Pacheco, M. Kaufman, F. Kemper, S. Kendrew, M.S. Kirsanova, P. Klaassen, S. Kwok, T.J. Lee, B. Lefloch, F. Le Petit, A. Li, H. Linz, C.J. Mackie, S.C. Madden, J. Mascetti, B.A. McGuire, P. Merino, E.R. Micelotta, K. Misselt, J.A. Morse, G. Mulas, N. Neelamkodan, R. Ohsawa, A. Omont, R. Paladini, M.E. Palumbo, A. Pathak, Y.J. Pendleton, A. Petrignani, T. Pino, E. Puga, N. Rangwala, M. Rapacioli, A. Ricca, J. Roman-Duval, J. Roser, E. Roueff, G. Rouillé, F. Salama, D.A. Sales, K. Sandstrom, P. Sarre, E. Sciamma-O'Brien, K. Sellgren, S.S. Shenoy, D. Teyssier, R.D. Thomas, A. Togi, L. Verstraete, A.N. Witt, A. Wootten, H. Zettergren, Y. Zhang, Z.E. Zhang and J. Zhen, PDRs4All II: JWST's NIR and MIR imaging view of the Orion Nebula (2023), arXiv:2308.16732.
- E. Peeters, E. Habart, O. Berne, A. Sidhu, R. Chown, D. Van De Putte, B. Trahin, I. Schroetter, A. Canin, F. Alarcon, B. Schefter, B. Khan, S. Pasquini, A.G.G.M. Tielens, M.G. Wolfire, E. Dartois, J.R. Goicoechea, A. Maragkoudakis, T. Onaka, M.W. Pound, S. Vicente, A. Abergel, E.A. Bergin, J. Bernard-Salas, C. Boersma, E. Bron, J. Cami, S. Cuadrado, D. Dicken, M. Elyajour, A. Fuente, K.D. Gordon, L. Issa, C. Joblin, O. Kannavou, O. Lacinbala, D. Languignon, R. Le Gal, R. Meshaka, Y. Okada, M. Robberto, M. Roellig, T. Schirmer, B. Tabone, M. Zannese, I. Aleman, L. Allamandola, R. Auchettl, G.A. Baratta, S. Bejaoui, P.P. Bera, J.H. Black, F. Boulanger, J. Bouwman, B. Brandl, P. Brechignac, S. Brunken, M. Buragohain, A. Burkhardt, A. Candian, S. Cazaux, J. Cernicharo, M. Chabot, S. Chakraborty, J. Champion, S.W.J. Colgan, I.R. Cooke, A. Coutens, N.L.J. Cox, K. Demyk, J. Donovan Meyer, S. Foschino, P. Garcia-Lario, M. Gerin, C.A. Gottlieb, P. Guillard, A. Gusdorf, P. Hartigan, J. He, E. Herbst, L. Hornekaer, C. Jager, E. Janot-Pacheco, M. Kaufman, S. Kendrew, M.S. Kirsanova, P. Klaassen, S. Kwok, A. Labiano, T.S.-Y. Lai, T.J. Lee, B. Lefloch, F. Le Petit, A. Li, H. Linz, C.J. Mackie, S.C. Madden, J. Mascetti, B.A. McGuire, P. Merino, E.R. Micelotta, K. Misselt, J.A. Morse, G. Mulas, N. Neelamkodan, R. Ohsawa, R. Paladini, M.E. Palumbo, A. Pathak, Y.J. Pendleton, A. Petrignani, T. Pino, E. Puga, N. Rangwala, M. Rapacioli, A. Ricca, J. Roman-Duval, J. Roser, E. Roueff, G. Rouille, F. Salama, D.A. Sales, K. Sandstrom, P. Sarre, E. Sciamma-O'Brien, K. Sellgren, S.S. Shenoy, D. Teyssier, R.D. Thomas, A. Togi, L. Verstraete, A.N. Witt, A. Wootten, N. Ysard, H. Zettergren, Y. Zhang, Z.E. Zhang and J. Zhen, PDRs4All III: JWST's NIR spectroscopic view of the Orion Bar, (2023), arXiv:2310.08720.
- R. Chown, A. Sidhu, E. Peeters, A.G.G.M. Tielens, J. Cami, O. Berné, E. Habart, F. Alarcón, A. Canin, I. Schroetter, B. Trahin, D. Van De Putte, A. Abergel, E.A. Bergin, J. Bernard-Salas, C. Boersma, E. Bron, S. Cuadrado, E. Dartois, D. Dicken, M. El-Yajouri, A. Fuente, J.R. Goicoechea, K.D. Gordon, L. Issa, C. Joblin, O. Kannavou, B. Khan, O. Lacinbala, D. Languignon, R. Le Gal, A. Maragkoudakis, R. Meshaka, Y. Okada, T. Onaka, S. Pasquini, M.W. Pound, M. Robberto, M. Röllig, B. Schefter, T. Schirmer, S. Vicente, M.G. Wolfire, M. Zannese, I. Aleman, L. Allamandola, R. Auchettl, G.A. Baratta, S. Bejaoui, P.P. Bera, J.H. Black, F. Boulanger, J. Bouwman, B. Brandl, P. Brechignac, S. Brünken, M. Buragohain, A. Burkhardt, A. Candian, S. Cazaux, J. Cernicharo, M. Chabot, S. Chakraborty, J. Champion, S.W.J. Colgan, I.R. Cooke, A. Coutens, N.L.J. Cox, K. Demyk, J.D. Meyer, S. Foschino, P. García-Lario, L. Gavilan, M. Gerin, C.A. Gottlieb, P. Guillard, A. Gusdorf, P. Hartigan, J. He, E. Herbst, L. Hornekaer, C. Jäger, E. Janot-Pacheco, M. Kaufman, F. Kemper, S. Kendrew, M.S. Kirsanova, P. Klaassen, S. Kwok, Á. Labiano, T.S.-Y. Lai, T.J. Lee, B. Lefloch, F. Le Petit, A. Li, H. Linz, C.J. Mackie, S.C. Madden, J.ëlle Mascetti, B.A. McGuire, P. Merino, E.R. Micelotta, K. Misselt, J.A. Morse, G. Mulas, N. Neelamkodan, R. Ohsawa, A. Omont, R. Paladini, M.E. Palumbo, A. Pathak, Y.J. Pendleton, A. Petrignani, T. Pino, E. Puga, N. Rangwala, M. Rapacioli, A. Ricca, J. Roman-Duval, J. Roser, E. Roueff, G. Rouillé, F. Salama, D.A. Sales, K. Sandstrom, P. Sarre, E. Sciamma-O'Brien, K. Sellgren, S.S. Shenoy, D. Teyssier, R.D. Thomas, A. Togi, L. Verstraete, A.N. Witt, A. Wootten, H. Zettergren, Y. Zhang, Z.E. Zhang and J. Zhen, PDRs4All IV. An embarrassment of riches: aromatic infrared bands in the Orion Bar (2023), arXiv:2308.16733.
- C. Boersma, L.J. Allamandola, A. Maragkoudakis, J.D. Bregman, T.J. Lee, P. Temi, V.J. Esposito, R.C. Fortenberry and E. Peeters, JWST: Deuterated PAHs, PAHnitriles, and PAH overtone and combination bands I: program description and first look. Astrophys. J. 959, 74 (2023). doi:10.3847/1538-4357/ad022b.
- K. Raghavachari, G.W. Trucks, J.A. Pople and M. Head-Gordon, A 5th-Order perturbation comparison of electron correlation theories. Chem. Phys. Lett. 157, 479–483 (1989). doi:10.1016/S0009-2614(89)87395-6.
- R.J. Rico and M. Head-Gordon, Single-reference theories of molecular excited states with single and double substitutions. Chem. Phys. Lett. 213, 224–232 (1993). doi:10.1016/0009-2614(93)85124-7.
- J.A. Pople, M. Head-Gordon and K. Raghavachari, Quadratic configuration interaction. A general technique for determining electron correlation energies. J. Chem. Phys. 87, 5968–5975 (1987). doi:10.1063/1.453520.
- M. Head-Gordon, M. Oumi and D. Maurice, Quasidegenerate second-order perturbation corrections to single-excitation configuration interaction. Mol. Phys. 96, 593–602 (1999).
- L. Allamandola, A. Tielens and J. Barker, Interstellar polycyclic aromatic hydrocarbons-the infrared emission bands, the excitation/emission mechanism, and the astrophysical implications. Astrophys. J. Suppl. Ser. 71, 733–775 (1989). doi:10.1086/191396.
- L. Allamandola, D. Hudgins and S. Sandford, Modeling the unidentified infrared emission with combinations of polycyclic aromatic hydrocarbons. Astrophys. J. 511, L115 (1999). doi:10.1086/311843.
- S. Hirata and M. Head-Gordon, Time-dependent density functional theory for radicals: An improved description of excited states with substantial double excitation character. Chem. Phys. Lett. 302, 375–382 (1999). doi:10.1016/S0009-2614(99)00137-2.
- S. Hirata and M. Head-Gordon, Time-dependent density functional theory within the Tamm–Dancoff approximation. Chem. Phys. Lett. 314, 291–299 (1999). doi:10.1016/S0009-2614(99)01149-5.
- D. Small, V. Zaitsev, Y. Jung, S.V. Rosokha, M. Head-Gordon and J.K. Kochi, Intermolecular π-to-π bonding between stacked aromatic dyads. Experimental and theoretical binding energies and near-IR optical transitions for phenalenyl radical/radical versus radical/cation dimerizations. J. Am. Chem. Soc. 126, 13850–13858 (2004). doi:10.1021/ja046770i.
- J. Fortney, T.D. Robinson, S. Domagal-Goldman, A.D.D. Genio, I.E. Gordon, E. Gharib-Nezhad, N. Lewis, C. Sousa-Silva, V. Airapetian, B. Drouin, R.J. Hargreaves, X. Huang, T. Karman, R.M. Ramirez, G.B. Rieker, J. Tennyson, R. Wordsworth, S.N. Yurchenko, A.V. Johnson, T.J. Lee, M.S. Marley, C. Dong, S. Kane, M. López-Morales, T. Fauchez, T. Lee, K. Sung, N. Haghighipour, S. Horst, P. Gao, D.-Y. Kao, C. Dressing, R. Lupu, D.W. Savin, B. Fleury, O. Venot, D. Ascenzi, S. Milam, H. Linnartz, M. Gudipati, G. Gronoff, F. Salama, L. Gavilan, J. Bouwman, M. Turbet, Y. Benilan, B. Henderson, N. Batalha, R. Jensen-Clem, T. Lyons, R. Freedman, E. Schwieterman, J. Goyal, L. Mancini, P. Irwin, J.-M. Desert, K. Molaverdikhani, J. Gizis, J. Taylor, J. Lothringer, R. Pierrehumbert, R. Zellem, N. Batalha, S. Rugheimer, J. Lustig-Yaeger, R. Hu, E. Kempton, G. Arney, M. Line, M. Alam, J. Moses, N. Iro, L. Kreidberg, J. Blecic, T. Louden, P. Mollière, K. Stevenson, M. Swain, K. Bott, N. Madhusudhan, J. Krissansen-Totton, D. Deming, I. Kitiashvili, E. Shkolnik, Z. Rustamkulov, L. Rogers and L. Close, The Need for Laboratory Measurements and ab initio Studies to Aid Understanding of Exoplanetary Atmospheres (2019), arXiv:1905.07064.
- L.S. Rothman, D. Jacquemart, A. Barbe, D. Chris Benner, M. Birk, L.R. Brown, M.R. Carleer, C. Chackerian Jr., K. Chance, L.H. Coudert, V. Dana, V.M. Devi, J.-M. Flaud, R.R. Gamache, A. Goldman, J.-M. Hartmann, K.W. Jucks, A.G. Maki, J.-Y. Mandin, S.T. Massie, J. Orphal, A. Perrin, C.P. Rinsland, M.A.H. Smith, J. Tennyson, R.N. Tolchenov, R.A. Toth, J. Vander Auwera, P. Varanasi and G. Wagner, The HITRAN 2004 molecular spectroscopic database. J. Quant. Spectrosc. Radiat. Transf. 96, 139–204 (2005). doi:10.1016/j.jqsrt.2004.10.008.
- H. Partridge and D.W. Schwenke, The determination of an accurate isotope dependent potential energy surface for water from extensive ab initio calculations and experimental data. J. Chem. Phys. 106, 4618–4639 (1997). doi:10.1063/1.473987.
- J. Tennyson and S.N. Yurchenko, ExoMol: Molecular line lists for exoplanet and other atmospheres. MNRAS 425, 21–33 (2012). doi:10.1111/j.1365-2966.2012.21440.x.
- J. Tennyson, S.N. Yurchenko, A.F. Al-Refaie, E.J. Barton, K.L. Chubb, P.A. Coles, S. Diamantopoulou, M.N. Gorman, C. Hill, A.Z. Lam, L. Lodi, L.K. McKemmish, Y. Na, A. Owens, O.L. Polyansky, T. Rivlin, C. Sousa-Silva, D.S. Underwood, A. Yachmenev and E. Zak, The ExoMol database: Molecular line lists for exoplanet and other hot atmospheres. J. Mol. Spectrosc. 327, 73–94 (2016). doi:10.1016/j.jms.2016.05.002.
- M. Rey, A.V. Nikitin, Y.L. Babikov and V.G. Tyuterev, TheoReTS – An information system for theoretical spectra based on variational predictions from molecular potential energy and dipole moment surfaces. J. Mol. Spectrosc. 327, 138–158 (2016). doi:10.1016/j.jms.2016.04.006.
- D.W. Schwenke, Variational calculations of rovibrational energy levels and transition intensities for tetratomic molecules. J. Phys. Chem. 100, 2867–2884 (1996). Erratum: J. Phys. Chem. 1996, 100(48), 18884.doi:10.1021/jp9525447.
- S.N. Yurchenko, J. Zheng, H. Lin, P. Jensen and W. Thiel, Potential-energy surface for the electronic ground state of NH 3 up to 20000 cm −1 above equilibrium. J. Chem. Phys. 123, 134308 (2005). doi:10.1063/1.2047572.
- L.S. Rothman, I.E. Gordon, A. Barbe, D. Chris Benner, P.F. Bernath, M. Birk, V. Boudon, L.R. Brown, A. Campargue, J.-P. Champion, K. Chance, L.H. Coudert, V. Dana, V.M. Devi, S. Fally, J.-M. Flaud, R.R. Gamache, A. Goldman, D. Jacquemart, I. Kleiner, N. Lacome, W.J. Lafferty, J.-Y. Mandin, S.T. Massie, S.N. Mikhailenko, C.E. Miller, N. Moazzen-Ahmadi, O.V. Naumenko, A.V. Nikitin, J. Orphal, V.I. Perevalov, A. Perrin, A. Predoi-Cross, C.P. Rinsland, M. Rotger, M. Šimecková, M.A.H. Smith, K. Sung, S.A. Tashkun, J. Tennyson, R.A. Toth, A.C. Vandaele and J. Vander Auwera, The HITRAN 2008 molecular spectroscopic database. J. Quant. Spectrosc. Radiat. Transf. 110, 533–572 (2009). doi:10.1016/j.jqsrt.2009.02.013.
- P.A. Coles, R.I. Ovsyannikov, O.L. Polyansky, S.N. Yurchenko and J. Tennyson, Improved potential energy surface and spectral assignments for ammonia in the near-infrared region. J. Quant. Spectrosc. Radiat. Transf. 219, 199–212 (2018). doi:10.1016/j.jqsrt.2018.07.022.
- O.L. Polyansky, R.I. Ovsyannikov, A.A. Kyuberis, L. Lodi, J. Tennyson, A. Yachmenev, S.N. Yurchenko and N.F. Zobov, Calculation of rotation-vibration energy levels of the ammonia molecule based on an ab initio potential energy surface. J. Mol. Spectrosc. 327, 21–30 (2016). doi:10.1016/j.jms.2016.08.003.
- E.-M. Ahrer, L. Alderson, N.M. Batalha, N.E. Batalha, J.L. Bean, T.G. Beatty, T.J. Bell, A.L. Carter, I.J.M. Crossfield, N. Espinoza, A.D. Feinstein, J.J. Fortney, N.P. Gibson, J.M. Goyal, E.M.-R. Kempton, J. Kirk, L. Kreidberg, M.R. Line, J.D. Lothringer, S.E. Moran, S. Mukherjee, K. Ohno, V. Parmentier, C. Piaulet, Z. Rustamkulov, E. Schlawin, D.K. Sing, K.B. Stevenson, H.R. Wakeford, N.H. Allen, S.M. Birkmann, J. Brande, N. Crouzet, P.E. Cubillos, M. Damiano, J.-M. Désert, P. Gao, J. Harrington, R. Hu, S. Kendrew, H.A. Knutson, P.-O. Lagage, J. Leconte, M. Lendl, R.J. MacDonald, E.M. May, Y. Miguel, K. Molaverdikhani, J.I. Moses, C.A. Murray, M. Nehring, N.K. Nikolov, D.J.M. Petit dit de la Roche, M. Radica, P.-A. Roy, K.G. Stassun, J. Taylor, W.C. Waalkes, P. Wachiraphan, L. Welbanks, P.J. Wheatley, K. Aggarwal, M.K. Alam, A. Banerjee, J.K. Barstow, J. Blecic, S.L. Casewell, Q. Changeat, K.L. Chubb, K.D. Colón, L.-P. Coulombe, T. Daylan, M. de Val-Borro, L. Decin, L.A. Dos Santos, L. Flagg, K. France, G. Fu, J.E. Gizis, A. Glidden, D. Grant, K. Heng, T. Henning, Y.-C. Hong, J. Inglis, N. Iro, T. Kataria, T.D. Komacek, J.E. Krick, E.K.H. Lee, N.K. Lewis, J. Lillo-Box, J. Lustig-Yaeger, L. Mancini, A.M. Mandell, M. Mansfield, M.S. Marley, T. Mikal-Evans, G. Morello, M.C. Nixon, K. Ortiz Ceballos, A.A.A. Piette, D. Powell, B.V. Rackham, L. Ramos-Rosado, E. Rauscher, S. Redfield, L.K. Rogers, M.T. Roman, G.M. Roudier, N. Scarsdale, E.L. Shkolnik, J. Southworth, J.J. Spake, M.E. Steinrueck, X. Tan, J.K. Teske, P. Tremblin, S.-M. Tsai, G.S. Tucker, J.D. Turner, J.A. Valenti, O. Venot, I.P. Waldmann, N.L. Wallack, X. Zhang and S. Zieba, Identification of carbon dioxide in an exoplanet atmosphere. Nature 614, 649–652 (2023). doi:10.1038/s41586-022-05590-4.
- S. Mukherjee, N.E. Batalha, J.J. Fortney and M.S. Marley, PICASO 3.0: A one-dimensional climate model for giant planets and brown dwarfs. Astrophys. J. 942, 71 (2023). doi:10.3847/1538-4357/ac9f48.
- E.-M. Ahrer, K.B. Stevenson, M. Mansfield, S.E. Moran, J. Brande, G. Morello, C.A. Murray, N.K. Nikolov, D.J.M. Petit dit de la Roche, E. Schlawin, P.J. Wheatley, S. Zieba, N.E. Batalha, M. Damiano, J.M. Goyal, M. Lendl, J.D. Lothringer, S. Mukherjee, K. Ohno, N.M. Batalha, M.P. Battley, J.L. Bean, T.G. Beatty, B.örn Benneke, Z.K. Berta-Thompson, A.L. Carter, P.E. Cubillos, T. Daylan, Néstor Espinoza, P. Gao, N.P. Gibson, S. Gill, J. Harrington, R. Hu, L. Kreidberg, N.K. Lewis, M.R. Line, M. López-Morales, V. Parmentier, D.K. Powell, D.K. Sing, S.-M. Tsai, H.R. Wakeford, L. Welbanks, M.K. Alam, L. Alderson, N.H. Allen, D.R. Anderson, J.K. Barstow, D. Bayliss, T.J. Bell, J. Blecic, E.M. Bryant, M.R. Burleigh, L. Carone, S.L. Casewell, Q. Changeat, K.L. Chubb, I.J.M. Crossfield, N. Crouzet, L. Decin, J.-M. Désert, A.D. Feinstein, L. Flagg, J.J. Fortney, J.E. Gizis, K. Heng, N. Iro, E.M.-R. Kempton, S. Kendrew, J. Kirk, H.A. Knutson, T.D. Komacek, P.-O. Lagage, J. Leconte, J. Lustig-Yaeger, R.J. MacDonald, L. Mancini, E.M. May, N.J. Mayne, Y. Miguel, T. Mikal-Evans, K. Molaverdikhani, E. Palle, C. Piaulet, B.V. Rackham, S. Redfield, L.K. Rogers, P.-A. Roy, Z. Rustamkulov, E.L. Shkolnik, K.S. Sotzen, J. Taylor, P. Tremblin, G.S. Tucker, J.D. Turner, M. de Val-Borro, O. Venot and X. Zhang, Early Release Science of the exoplanet WASP-39b with JWST NIRCam. Nature 614, 653–658 (2023). doi:10.1038/s41586-022-05590-4.
- Z. Rustamkulov, D.K. Sing, S. Mukherjee, E.M. May, J. Kirk, E. Schlawin, M.R. Line, C. Piaulet, A.L. Carter, N.E. Batalha, J.M. Goyal, M. López-Morales, J.D. Lothringer, R.J. MacDonald, S.E. Moran, K.B. Stevenson, H.R. Wakeford, N. Espinoza, J.L. Bean, N.M. Batalha, B. Benneke, Z.K. Berta-Thompson, I.J.M. Crossfield, P. Gao, L. Kreidberg, D.K. Powell, P.E. Cubillos, N.P. Gibson, J. Leconte, K. Molaverdikhani, N.K. Nikolov, V. Parmentier, P. Roy, J. Taylor, J.D. Turner, P.J. Wheatley, K. Aggarwal, E. Ahrer, M.K. Alam, L. Alderson, N.H. Allen, A. Banerjee, S. Barat, D. Barrado, J.K. Barstow, T.J. Bell, J. Blecic, J. Brande, S. Casewell, Q. Changeat, K.L. Chubb, N. Crouzet, T. Daylan, L. Decin, J. Désert, T. Mikal-Evans, A.D. Feinstein, L. Flagg, J.J. Fortney, J. Harrington, K. Heng, Y. Hong, R. Hu, N. Iro, T. Kataria, E.M.-R. Kempton, J. Krick, M. Lendl, J. Lillo-Box, A. Louca, J. Lustig-Yaeger, L. Mancini, M. Mansfield, N.J. Mayne, Y. Miguel, G. Morello, K. Ohno, E. Palle, D.J.M. Petit dit de la Roche, B.V. Rackham, M. Radica, L. Ramos-Rosado, S. Redfield, L.K. Rogers, E.L. Shkolnik, J. Southworth, J. Teske, P. Tremblin, G.S. Tucker, O. Venot, W.C. Waalkes, L. Welbanks, X. Zhang and S. Zieba, Early Release Science of the exoplanet WASP-39b with JWST NIRSpec PRISM. Nature 614, 659–663 (2023). doi:10.1038/s41586-022-05677-y.
- D. Crisp, H.R. Pollock, R. Rosenberg, L. Chapsky, R.A.M. Lee, F.A. Oyafuso, C. Frankenberg, C.W. O'Dell, C.J. Bruegge, G.B. Doran, A. Eldering, B.M. Fisher, D. Fu, M.R. Gunson, L. Mandrake, G.B. Osterman, F.M. Schwandner, K. Sun, T.E. Taylor, P.O. Wennberg and D. Wunch, The on-orbit performance of the Orbiting Carbon Observatory-2 (OCO-2) instrument and its radiometrically calibrated products. Atmos. Meas. Tech. 10, 59–81 (2017). doi:10.5194/amt-10-59-2017.
- H. Fleurbaey, H. Yi, E.M. Adkins, A.J. Fleisher and J.T. Hodges, Cavity ring-down spectroscopy of CO 2 near λ=2.06μm: Accurate transition intensities for the Orbiting Carbon Observatory-2 (OCO-2) ‘strong band’. J. Quant. Spectrosc. Radiat. Transf. 252, 107104 (2020). doi:10.1016/j.jqsrt.2020.107104.
- D.A. Long, Z.D. Reed, A.J. Fleisher, J. Mendonca, S. Roche and J.T. Hodges, High-accuracy near-infrared carbon dioxide intensity measurements to support remote sensing. Geophys. Res. Lett. 47, 2019GL086344 (2020). doi:10.1029/2019GL086344.
- M. Birk, C. Röske and G. Wagner, High accuracy CO 2 Fourier transform measurements in the range 6000–7000 cm −1. J. Quant. Spectrosc. Radiat. Transf. 272, 107791 (2021). And new experiments for 2001i and 3001i bands, private communication (2023). doi:10.1016/j.jqsrt.2021.107791.
- O.L. Polyansky, A.A. Kyuberis, N.F. Zobov, J. Tennyson, S.N. Yurchenko and L. Lodi, ExoMol molecular line lists XXX: A complete high-accuracy line list for water. MNRAS 480, 2597–2608 (2018). doi:10.1093/mnras/sty1877.
- P.A. Coles, S.N. Yurchenko and J. Tennyson, ExoMol molecular line lists – XXXV. A rotation-vibration line list for hot ammonia. MNRAS 490, 4638–4647 (2019). doi:10.1093/mnras/stz2778.
- I.E. Gordon, L.S. Rothman, C. Hill, R.V. Kochanov, Y. Tan, P.F. Bernath, M. Birk, V. Boudon, A. Campargue, K.V. Chance, B.J. Drouin, J.-M. Flaud, R.R. Gamache, J.T. Hodges, D. Jacquemart, V.I. Perevalov, A. Perrin, K.P. Shine, M.-A.H. Smith, J. Tennyson, G.C. Toon, H. Tran, V.G. Tyuterev, A. Barbe, A.G. Császár, V.M. Devi, T. Furtenbacher, J.J. Harrison, J.-M. Hartmann, A. Jolly, T.J. Johnson, T. Karman, I. Kleiner, A.A. Kyuberis, J. Loos, O.M. Lyulin, S.T. Massie, S.N. Mikhailenko, N. Moazzen-Ahmadi, H.S.P. Müller, O.V. Naumenko, A.V. Nikitin, O.L. Polyansky, M. Rey, M. Rotger, S.W. Sharpe, K. Sung, E. Starikova, S.A. Tashkun, J.V. Auwera, G. Wagner, J. Wilzewski, P. Wcislo, S. Yu and E.J. Zak, The HITRAN2016 molecular spectroscopic database. J. Quant. Spectrosc. Radiat. Transf. 203, 3–69 (2017). doi:10.1016/j.jqsrt.2017.06.038.
- S.A. Tashkun, V.I. Perevalov, R.R. Gamache and J. Lamouroux, CDSD-296, high-resolution carbon dioxide spectroscopic databank: An update. J. Quant. Spectrosc. Radiat. Transf. 228, 124–131 (2019). doi:10.1016/j.jqsrt.2019.03.001.
- L.S. Rothman, I.E. Gordon, R.J. Barber, H. Dothe, R.R. Gamache, A. Goldman, V.I. Perevalov, S.A. Tashkun and J. Tennyson, HITEMP, the high-temperature molecular spectroscopic database. J. Quant. Spectrosc. Radiat. Transf. 111, 2139–2150 (2010). doi:10.1016/j.jqsrt.2010.05.001.
- S.N. Yurchenko, T.M. Mellor, R.S. Freedman and J. Tennyson, ExoMol line lists – XXXIX. Ro-vibrational molecular line list for CO 2. MNRAS 496, 5282–5291 (2020). doi:10.1093/mnras/staa1874.
- S. Tashkun and V. Perevalov, CDSD-4000: High-resolution, high-temperature carbon dioxide spectroscopic databank. J. Quant. Spectrosc. Radiat. Transf. 112, 1403–1410 (2011). doi:10.1016/j.jqsrt.2011.03.005.
- J.F. Gaw, A. Willets, W.H. Green and N.C. Handy, in Advances in Molecular Vibrations and Collision Dynamics, edited by J.M. Bowman, M.A. Ratner (JAI Press, Inc.: Greenwich, Connecticut, 1991), pp. 170–185.
- P. Thaddeus, J.M. Vrtilek and C.A. Gottlieb, Laboratory and astronomical detection of cyclopropenylidene, C 3H 2. Astrophys. J. 296, L63–L66 (1985). doi:10.1086/184581.
- S.C. Madden, W.M. Irvine and H.E. Matthews, Detection of 13C-substituted C 3H 2 in astronomical sources. Astrophys. J. 311, L27–L31 (1986). doi:10.1086/184792.
- M. Gerin, H.A. Wootten, F. Combes, F. Boulanger, I.I.I Peters, P.J. Encrenaz and M. Bogey, Deuterated C 3H 2 as a clue to deuterium chemistry. Astron. Astrophys. 173, L1–L4 (1987).
- S.C. Madden, W.M. Irvine, H.E. Matthews, P. Friberg and D.A. Swade, A survey of cyclopropenylidene (C 3H 2) in galactic sources. Astronom. J. 97, 1403–1422 (1989). doi:10.1086/115081.
- I.H. Park, V. Wakelam and E. Herbst, Modeling the ortho-to-para abundance ratio of cyclic C 3H 2 in cold dense cores. Astron. Astrophys. 449, 631–639 (2006). doi:10.1051/0004-6361:20054420.
- S. Spezzano, S. Brünken, P. Schilke, P. Caselli, K.M. Menten, M.C. McCarthy, L. Bizzocchi, S.P. Trevinõ-Morales, Y. Aikawa and S. Schlemmer, Interstellar detection of c-C 3D 2. Astrophys. J. Lett. 769, 19 (2013). doi:10.1088/2041-8205/769/2/L19.
- S. Spezzano, S. Brünken, C.A. Gottlieb, P. Caselli, K.M. Menten, H.S.P. Müller, L. Bizzocchi, P. Schilke, M.C. McCarthy and S. Schlemmer, A study of the C 3H 2 isomers and isotopologues: first interstellar detection of HDCCC. Astron. Astrophys. 586, A110 (2016). doi:10.1051/0004-6361/201527460.
- R.C. Fortenberry and T.J. Lee, in Annual Reports in Computational Chemistry, edited by D. Dixon (2019), Vol. 15, pp. 173–202. “Computational vibrational spectroscopy for the detection of molecules in space”.
- J.M.L. Martin and P.R. Taylor, Accurate ab Initio quartic force field for trans-HNNH and treatment of resonance polyads. Spectrochim. Acta A 53, 1039–1050 (1997). doi:10.1016/S1386-1425(96)01869-0.
- D. Zhao, K.D. Doney and H. Linnartz, Laboratory gas-phase detection of the cyclopropenyl cation (c-C 3H 3+) Astrophys. J. Lett. 791, L28 (2014). doi:10.1088/2041-8205/791/2/L28.
- L. Bizzocchi, V. Lattanzi, J. Laas, S. Spezzano, B.M. Giuliano, D. Prudenzano, C. Endres, O. Sipilä and P. Caselli, Accurate Sub-millimetre rest frequencies for HOCO + and DOCO + Ions. Astron. Astrophys. 602, A34 (2017). doi:10.1051/0004-6361/201730638.
- B.R. Westbrook and R.C. Fortenberry, pbqff: Push-button quartic force fields. J. Chem. Theory Comput. 19, 2606–2615 (2023). doi:10.1021/acs.jctc.3c00129.