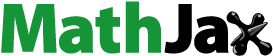
Abstract
Feline infectious peritonitis (FIP) is a potentially fatal coronavirus-driven disease of cats. Treatment with nucleoside analogue GS-441524 and or prodrug remdesivir (RDV) have produced remission in both experimentally induced and naturally occurring FIP, yet information regarding metabolism of RDV into GS-441524 in cats is scarce. This study assessed possible phase I metabolism of RDV in cats, utilising an in vitro feline microsome model with in vitro t1/2 and in vitro Clint calculated using the substrate depletion method. A previously validated high-performance liquid chromatography (HPLC) fluorescence method was utilised for detection and analysis of RDV and GS-441524. Qualitative yield of RDV and intermediate metabolite GS-441524 were determined following microsome incubation, then compared to whole blood and plasma incubations. In vitro microsome incubation resulted in rapid depletion of RDV, though it did not appear to resemble a conventional phase I-dependent reaction in cats, as it is in humans and dogs. Depletion of RDV into GS-441524 was demonstrated in whole blood in vitro, suggesting cats convert RDV to GS-441524, likely via blood esterases, as observed in mice and rats. RDV metabolism is unlikely to be impacted by impaired liver function in cats. Furthermore, as RDV depletes within minutes, whereas GS-441524 is very stable, whole blood or plasma GS-441524 concentrations, rather than plasma RDV concentrations, are more appropriate for therapeutic drug monitoring (TDM) in cats receiving RDV.
1. Introduction
Feline infectious peritonitis (FIP) is a potentially fatal coronavirus-driven disease of domestic and wild felids. Treatment with the small molecule-based nucleoside analogue GS-441524 has been a topic of global interest since remission in experimentally induced FIP was first demonstrated in 2018 (Murphy et al. Citation2018). Multiple publications have now documented remission in naturally occurring FIP using both oral and parenteral (intravenous [IV] and subcutaneous [SC]) formulations of GS-441524 (Pedersen et al. Citation2019; Krentz et al. Citation2021; Jones et al. Citation2021; Coggins et al. Citation2023; Green et al. Citation2023).
Significant inter-species variation can exist with respect to drug metabolism and resultant drug efficacy and safety profiles. Historically, many antiviral agents investigated for FIP treatment were shown to possess zero or limited therapeutic benefit, proved toxic when administered in vivo (Weiss et al. Citation1993; Hartmann and Ritz Citation2008; Pedersen Citation2014), or were not progressed beyond in vitro models (Barlough and Shacklett Citation1994; Balzarini et al. Citation2005). Nucleoside and nucleotide analogues are a powerful class of molecules with diverse therapeutic utility. Primarily, they are used as antiviral and chemotherapeutic agents, owing to their ability to influence replication and transcription of nucleic acids (Périgaud et al. Citation1992; De Clercq Citation2004; Jordheim et al. Citation2013). Nucleosides are composed of a sugar moiety and nucleobase, whereas nucleotides possess the addition of one or more phosphate groups (Yates and Katherine Citation2018).
Remdesivir (RDV) (GS-5734) is a low-molecular-weight, monophosphoramidate nucleotide prodrug (Agostini et al. Citation2018). On reaching the extravascular and interstitial compartments, RDV can diffuse across cell membranes. Once inside the cell, RDV undergoes hydrolysis to form the alanine metabolite (GS-704277), then the principal metabolite GS-441524, which is then tri-phosphorylated to form the pharmacologically active GS-443902, in humans, rhesus monkeys and mice (Warren et al. Citation2016; Sheahan et al. Citation2017; Avataneo et al. Citation2020; Humeniuk et al. Citation2020; Williamson et al. Citation2020; Eastman et al. Citation2020). GS-443902 is then incorporated into viral RNA via RNA-dependent RNA polymerase (RdRp), disrupting viral replication () (Cho et al. Citation2012; Warren et al. Citation2016; Humeniuk et al. Citation2020; Yan and Muller Citation2021; Li et al. Citation2022; VEKLURY Prescriber Information Citation2022; Gordon et al. Citation2020). In humans, RDV predominantly undergoes phase 1 hepatic metabolism facilitated by cytochrome P450 enzymes. More specifically, RDV is metabolized in humans by CYP2C8, CYP2D6, and CYP3A4 in vitro, though metabolism in vivo is also likely to be mediated by hydrolase activity (Agency and Division Citation2020). The intrinsic half-life of RDV is 1 min when incubated with human hepatic enzymes (microsomes) and has a plasma half-life (t1/2) in vivo of approximately 1 h (Humeniuk et al. Citation2020; Xie and Wang Citation2021; Tempestilli et al. Citation2020, Citation2021). In contrast, RDV metabolism in mice does not appear to be a NADPH-dependent process (Xie and Wang Citation2021; Hu et al. Citation2021), with blood esterases hypothesised to be responsible for the rapid hydrolysis of RDV to GS-441524 (Bahar et al. Citation2012). The pharmacokinetic profile of GS-441524 in a small number of healthy specific pathogen free (SPF) cats after intravenous (IV) and subcutaneous (SC) administration of 5 mg/kg has been described (Murphy et al. Citation2018). Additionally, the formation of the active triphosphate GS-443902 within peripheral blood mononuclear cells was demonstrated at concentrations 8–20 times higher than the 50% of the effective concentration (EC50) of 1 µM over 72 h (Murphy et al. Citation2018).
Figure 1. Simplified biosynthesis pathway of RDV in humans. Remdesivir diffuses across the cell membrane and is then hydrolysed into intermediate metabolites, ultimately resulting in the formation of the active triphosphate GS-443902. This active triphosphate is efficiently incorporated into viral RNA via RNA-dependent RNA polymerase (RdRp), disrupting viral replication. Image created using adaptions from Eastman et al. (Citation2020) and images sourced from PubChem. CID 121304016 (GS-5734), CID 121313150 (GS-704277), CID 44468216 (GS-441524), and CID 56832906 (GS-443902).

Absence of veterinary licensing and progression to commercialisation of GS-441524 dictated that the only access to this compound was initially via unlicensed drug manufacturers. In late 2020, Australian veterinarians gained access to legally compounded RDV for parenteral administration. No information was available regarding whether cats could indeed metabolise RDV into GS-441524 and if so, it was unknown in which tissues or physiological compartments did this conversion take place. Regardless, given the fatal outcome of FIP, veterinarians began to utilise RDV to treat cats with naturally-occurring FIP, with 86% of treated cats now surviving this disease in Australia (Coggins et al. Citation2023).
The aims of this study were (i) to assess whether RDV undergoes classical phase I hepatic metabolism in cats, utilising an in vitro feline microsome depletion model (with dog and rat microsomes as comparative species controls); and (ii) to qualitatively determine whether cats metabolise RDV into the intermediate metabolite GS-441524 by hepatic microsomes, and or whether conversion first occurs in whole blood or plasma.
2. Methods
2.1. Chemicals
Remdesivir (GS-5734, CAS 1809249-37-3) and GS-441524 (purity 99.77%, CAS: 1191237-69-0) were purchased from Assay Matrix (Ivanhoe North, VIC, Australia). Commercially available pooled feline microsomes (3 males, S00779) were purchased from BioIVT Baltimore Maryland USA. Pooled canine microsomes (7 males, M00201) were purchased from BD Biosciences (Woburn, MA, USA) and pooled rat microsomes (male, number of individuals not specified M9066) were purchased from Sigma (Castle Hill, NSW, Australia). Microsomes were shipped on liquid nitrogen and stored at −80 °C prior to use. β-nicotinamide adenine dinucleotide phosphate sodium (NADP), glucose-6-phosphate dehydrogenase, glucose-6-phosphate, ammonium acetate, glacial acetic acid, and magnesium chloride were purchased from Sigma-Aldrich (Castle Hill, NSW, Australia). High-performance liquid chromatography (HPLC) grade acetonitrile (MeCN) and methanol (MeOH) were purchased from Thermo Fisher Scientific (Macquarie Park, NSW, Australia). Water was purified via a Milli-Q water purification system (Merk Millipore, Burlington, MA, USA).
2.2. In vitro microsome assay development
A substrate depletion method was used to assess RDV stability. This method utilises liver-derived microsomes containing cytochrome P450 enzymes, to simulate hepatic metabolism, whilst quantitating the decline of RDV over time. The addition of NADPH as a cofactor enhances physiological relevance, as NADPH serves as a crucial catalyst for phase I metabolism in vivo (Obach et al. Citation1997).
GS-441524 (1.25 µM) was incubated with 0.5 mg/mL feline microsomes (in duplicate) in 0.1 M phosphate buffer (pH 7.4) containing an NADPH regenerating system (consisting of 1 mM NADP, 0.8 U glucose-6-phosphate dehydrogenase, and 3 mM glucose-6-phosphate) and 3 mM MgCl2, in a water bath shaker at 37 °C. Stability of GS-441524 was confirmed, with the percentage remaining at 60 min determined to be 100% of the initial 1.25 µM GS-441524 (). This finding was consistent with observations in mice and humans (Xie and Wang Citation2021) and ensured GS-441524 formation would be detected if generated, when RDV was incubated with feline microsomes. A concentration of 0.5 mg/mL of microsomes was selected as this had been previously utilised for in vitro RDV metabolism assays in humans and mice (Xie and Wang Citation2021) and was utilised for all microsome incubations throughout this study.
Figure 2. Incubations (A) GS-441524 stability: Remaining percentage (%) GS-441524 when incubated with feline microsomes, NADPH and phosphate buffer. Remdesivir stability: Remaining percentage (%) RDV when incubated, NADPH and phosphate buffer. (B) Feline microsomes incubated with RDV, both with and without NADPH. (C) Rat microsomes incubated with RDV, both with and without NADPH. (D) Canine microsomes incubated with RDV, both with and without NADPH. Data are presented as mean ± SD (n = 2).

To ensure no interference with RDV from the NADPH regenerating system (NADPH) or phosphate buffer, RDV (1.25 µM) was incubated with NADHP and phosphate buffer (in duplicate) for 60 min at 37 °C and was determined to be stable in the absence of feline microsomes (remaining percentage 96.6%) ().
Initial screening of RDV microsomal stability was assessed a 1.25, 5 and 10 µM, up to 3 h, when incubated at 37 °C in the presence of feline microsomes (0.5 mg/mL final concentration). Microsome incubation timepoints were selected at 0, 1, 2, 3, 5, and 10 min, as RDV was not detectable after 10 min. Thereafter, specific incubation time points representing initial depletion rate was further identified based on the highest r2 value of the slope. Due to the similar depletion rates observed across the three concentrations (Supplementary Table 1), RDV 1.25 µM was chosen for microsome assay incubations, as it closely matched the concentration already used in human and mouse RDV depletion models (Xie and Wang Citation2021).
2.3. Drug analysis (chromatographic condition)
For detection and analysis of RDV and GS-441524, our previously validated HPLC with fluorescence detection was utilised, using a Shimadzu Nexera XR LC system (Rydalmere, NSW, Australia) (Kimble et al. Citation2023). The Waters X-Bridge C18, 5 µm, 150 × 4.6 mm (Dundas, NSW, Australia) was selected as the stationary phase with the column temperature maintained at 35 °C. Flow rate was set at 1.2 mL/min and analytes monitored with excitation and emission wavelengths of 250 nm and 475 nm, respectively. For screening of GS-704277, GS-441524 and RDV, a mixture of 20 mM of ammonium acetate (adjusted to pH 4.5 with acetic acid) with solutions of either 5% of acetonitrile (Mobile phase A) or 70% of acetonitrile (Mobile phase B) were prepared for the following gradient: 0–1 min (0–5% B); 1–2 min (5% B); 2–5 min (5–50% B); 5–10 min (50–90% B); 10–11 min (90% B); and 11–12 min (90–0%). Total run time was 15 min to equilibrate the condition; where retention times of GS-441524 and RDV were 4.78 and 9.95 min, respectively. For monitoring the RDV peak area for both in vitro t1/2 and intrinsic clearance, isocratic mobile phase (45% MeCN in 20 mM of ammonium acetate, adjusted to pH 4.5 with acetic acid) was used.
2.4. RDV incubations with hepatic microsomes
For the phase I microsome assay, RDV (1.25 μM) was preincubated in a glass tube containing: 2.0 mL of 0.1 M phosphate buffer (pH 7.4); and a NADPH regenerating system in an open-air shaking water bath at 37 °C for 3 min. Subsequently, 50 μL of hepatic microsome (20 mg/mL) from either cats, dogs or rats, was added to a prepared 2 mL solution to initiate an enzymatic reaction at the final microsome concentration of 0.5 mg/mL. During incubation, 200 μL aliquots were removed at time (t) = 0, 1, 2, 3, 5, and 10 min. Each aliquot was placed into individual 1.5 mL Eppendorf tubes containing 400 μL ice-cold MeOH to facilitate instant deactivation of enzymatic reactions. Each mixture in the Eppendorf tubes were vortexed and centrifuged at 14,000 g for 10 min, and 10 μL of supernatant injected directly into the HPLC system for analysis. Negative controls were performed assessing RDV stability in a microsome assay without the NADPH regenerating system, and with NADPH but without presence of the microsomes. All assay procedures were performed in duplicate as per the hepatic microsome assay with NADPH, however, for the negative controls the NAPDH was substituted with same volume of phosphate buffer. Remdesivir incubation with feline microsomes was also repeated at a higher concentration of 10 µM (over 2 h) to exclude the possibility that lack of GS-441524 formation seen with 1.25 µM was a dose dependent phenomenon.
2.5. Stability of RDV in vitro in plasma and whole blood
Stability of RDV was also investigated in fresh feline whole blood and plasma. The unused excess contents of lithium heparin blood tubes were utilised from a healthy 1.5-year-old castrated male domestic medium haired cat within 30 min of collection. Approval was obtained from The University of Sydney Animal Ethics Committee, Project number: 2023/2320. Blood and plasma (0.5 mL each) were spiked with RDV (1.25 µM) and incubated in a water bath 37 °C for 8 min. During incubation, 50 µL aliquots were removed at (t) = 0, 1, 2, 3, 4, and 8 min and mixed with 100 µL of MeOH. The prepared samples were vortexed and centrifugated (14,000 g for 10 min) and 10 µL of the supernatant was injected into the HPLC.
2.6. Determination of in vitro t1/2 and in vitro Clint of RDV
In vitro Clint were calculated by the substrate depletion method utilising the in vitro t1/2 approach (Obach et al. Citation1997).
In vitro t1/2 was determined for microsome, plasma and whole blood incubations, and in vitro Clint was determined for microsome incubations. Using the area of RDV chromatogram peak (i.e. peak area [PA]) at t = 0 as 100% of substrate, PAs of other time points were converted to percentage of substrate remaining. This was plotted as natural log of remaining RDV vs. incubation time, and the slope of the regression line, represented as a constant rate (-k), was used for to calculate t1/2 (min) and in vitro Clint (μL/min/mg protein) according to the following equations:
2.7. Qualitative detection of GS-441524 in, plasma and whole blood
To detect GS-441524 formation, a higher concentration of RDV (10 µM) was incubated at 37 °C with 600 µL plasma and 600 µL blood (over 3 h). For these incubations, aliquots of 100 µL were removed at t = 0, 0.5, 1, 2 and 3 h. Formation of GS-441524 was qualitatively detected via HPLC, comparing the PA between the plasma, blood, and that of the microsome matrix when RDV 10 µM was incubated with feline microsomes.
2.8. Statistical analysis
GraphPad Prism 9.5.1 software (GraphPad Software Inc., CA, USA) was used to perform linear regression of the depletion, calculation of the coefficient of determination and for graph generation.
3. Results
3.1. RDV incubations with hepatic microsomes
In the presence of feline microsomes, rapid depletion of RDV occurred, both with and without the presence of NADPH (), with in vitro t1/2 0.35 min (SD ± 0.00) and 0.5 min (SD ± 0.04), respectively (Supplementary Table 2). Similar results were observed with rat microsome incubations (), showing rapid RDV depletion with and without NADPH, with in vitro t1/2 0.58 min (SD ± 0.00) and 1.44 min (SD ± 0.04) respectively. In contrast, dogs showed much more RDV depletion in the presence of NADPH and slower depletion without NADPH (), with in vitro t1/2 1.29 m (SD ± 0.13) and 7.28 m (SD ± 0.60), respectively.
During the 1.25 µM RDV incubations performed in the presence of feline microsomes, although rapid depletion of RDV was observed, negligible GS-441524 was generated. When incubations were repeated at 10 µM and extended over 2 h, still only small amounts of GS-441524 were detected (Supplementary Table 3). The only peak observed to form over time was suspected to be the intermediate alanine metabolite, although this peak was broad and would require additional specific assay optimisation considered beyond the scope of this investigation.
3.2. Stability of RDV in vitro in plasma and whole blood
In vitro t1/2 using 1.25 µM RDV in fresh feline whole blood and plasma were 0.39 min (SD ± 0.01) and 0.38 min (SD ± 0.01) respectively (Supplementary Table 4), and comparable to the in vitro t1/2 seen with microsome incubation. Following qualitative assessment of serial chromatograms, a GS-441524 peak formed, most notable following RDV incubation with whole blood (). Comparison of GS-441524 PA’s revealed the rate of GS-441524 formation in whole blood to be 4-fold higher than in plasma by 1 h (PA blood 10,715; PA plasma 2,643); 6-fold higher at 2 h (PA blood 32,085; PA plasma 5,071) and 8-fold higher at 3 h (PA blood 70,823; PA plasma 8,398) (Supplementary Table 3).
Figure 3. (A) Chromatogram of feline whole blood (n = 1) monitored from t = 0 to 3 h. Black arrows indicate peaks for RDV, probable alanine metabolite and GS-441524. Red circle highlights the progressive formation of GS-441524 over time. (B) Chromatogram of feline plasma (n = 1) monitored from t = 0 to 3 h. Black arrows indicate peaks for RDV, probable alanine metabolite and GS-441524. Red circle highlights the progressive formation of GS-441524 over time. Retention time (RT) of GS-441524: 4.78 min; RT of RDV: 9.95 min; RT of alanine metabolite: 2.21 min.

4. Discussion
Depletion of RDV in the presence of human microsomes without addition of NADPH has shown to be prolonged, with a half-life of t1/2 30.6 min (Xie and Wang Citation2021). In contrast, addition of NADPH and cofactors results in a rapid RDV depletion with t1/2 of 1.1 min (Xie and Wang Citation2021). This observation suggests that in man, phase 1 metabolism drives the RDV Clint. In contrast, when RDV is incubated with mouse microsomes, rapid depletion of RDV is observed both with and without NADPH (t1/2 0.8 and 1.0 m respectively) (Xie and Wang Citation2021).
In the current study, RDV was unstable in the presence of feline and rat microsomes with or without NADPH, yet remained stable in the same matrix with the microsomes removed. These results suggest that cats (and rats), may be more akin to mice, whereby RDV metabolism does not appear to be a NADPH-mediated process (Xie and Wang Citation2021). In contrast, canine microsomes resulted in far more rapid RDV depletion in the presence of NADPH than without. As NADPH is required for cytochrome activation during phase I metabolism, this suggests dogs are more like humans, where RDV metabolism is a NADPH-dependent process (Xie and Wang Citation2021).
Despite RDV being so labile in the presence of feline microsomes (RDV was undetectable within 5 min during microsome incubations both with and without NADPH), biotransformation into GS-441524 did not readily occur, with negligible amounts of GS-441524 detected following both RDV 1.25 µM and 10 µM microsome incubations over 2 h.
When the in vitro incubations were performed using 10 µM RDV incubated with feline whole blood or plasma, RDV was undetectable within minutes. GS-441524 formed progressively as a visibly larger PA in the whole blood incubation. This observation demonstrates the transformation of RDV into GS-441524 occurs within the whole blood of cats in vitro, and it seems highly likely this also occurs in vivo.
When stability of RDV was assessed in mouse plasma, RDV depleted to undetectable levels within seconds (Xie and Wang Citation2021). Our findings suggest that cats appear to be more like mice, with dephosphorylation of RDV to GS-441524 being catalysed by something in the plasma (most likely esterases). Species variations in blood and plasma esterase composition are well understood. Mice, cats, tigers, rats, rabbits and horses have been found to express high levels of carboxylesterases 1 and 2 in their plasma and blood, whereas humans do not (Li et al. Citation2005; Rudakova et al. Citation2011). In contrast, human blood cholinesterase activity appears significantly higher than mice or rats (Rudakova et al. Citation2011). This study showed significantly higher amounts of GS-441524 formation occurred with whole blood compared to plasma incubations. We hypothesise drug metabolism in cats may be mediated by red or white blood cell associated factors. Red and white blood cells have shown to possess numerous enzymes or enzyme-like properties capable of drug metabolism (Cossum Citation1988). The precise mechanism for RDV metabolism to GS-441524 was beyond the scope of this study. Furthermore, it is important to note the strength of this observation is limited, as the blood and plasma of a single cat was utilized. Further investigation using fresh blood and plasma from several normal and FIP-affected cats would provide further weight to this observation.
At the time this research was conducted, in late 2020, parenteral compounded RDV was the only legally available product for treating cats with FIP in Australia. In human studies assessing RDV pharmacokinetics, the half-life of RDV (t1/2 1–1.3 h) was considered too short for measurement of this drug to be a useful marker for therapeutic drug monitoring (TDM) (Deb et al. Citation2021). The intermediate metabolite GS-441524 demonstrated a much longer plasma half-life (t1/2 24 h) (Xie and Wang Citation2021) suggesting GS-441524 is a more promising target for TDM during RDV therapy in humans (Deb et al. Citation2021). Given the demonstrated lability of RDV in cats, with similarly rapid t1/2 of < 1 m observed in microsome, blood and plasma incubations, this study suggests that, as for humans, RDV plasma levels may have poor utility for TDM. We have previously demonstrated that following administration of 15 mg/kg IV RDV in vivo to a cat with FIP, GS-441524 was the only detectable metabolite at 24 h (Kimble et al. Citation2023). Given that GS-441524 is stable when incubated in the presence of feline microsomes and persists to 24 h in the plasma, this compound may be a more helpful marker for TDM following RDV administration in vivo in cats.
5. Conclusion
For cats, in vitro microsome depletion models suggest that hydrolysis of RDV into GS-441524 is not an NADPH-dependent reaction, unlike humans and dogs. Rapid biotransformation of RDV into GS-441524 was demonstrated in whole blood in vitro, suggesting cats metabolize this drug similarly to mice and rats. Remdesivir metabolism is therefore unlikely to be impacted in cats with impaired liver metabolism. Remdesivir depletion occurs within minutes, whereas GS-441524 appears very stable, suggesting that plasma GS-441524 concentrations may prove a more valuable target for TDM of in vivo RDV treatment than plasma RDV concentrations.
Animal welfare and ethics statement
This study is a part of a broader clinical trial approved by The University of Sydney Animal Ethics Committee (2021/1874)
Supplemental Material
Download MS Word (20.5 KB)Disclosure statement
The authors declare no conflicts of interest.
Data availability statement
The data supporting the findings of this study are available from Dr. Sally Coggins ([email protected]).
Additional information
Funding
References
- Agency EM, Division HM. 2020. Remdesivir Gilead. Product No EMEA/H/K/5622/CU 03 April 2020. Summary on Compassionate Use.
- Agostini ML, Andres EL, Sims AC, Graham RL, Sheahan TP, Lu X, Smith EC, Case JB, Feng JY, Jordan R, et al. 2018. Coronavirus susceptibility to the antiviral remdesivir (GS-5734) is mediated by the viral polymerase and the proofreading exoribonuclease. mBio. 9(2):e00221–00218. doi: 10.1128/mBio.00221-18.
- Avataneo V, de Nicolò A, Cusato J, Antonucci M, Manca A, Palermiti A, Waitt C, Walimbwa S, Lamorde M, di Perri G, et al. 2020. Development and validation of a UHPLC-MS/MS method for quantification of the prodrug remdesivir and its metabolite GS-441524: a tool for clinical pharmacokinetics of SARS-CoV-2/COVID-19 and Ebola virus disease. J Antimicrob Chemother. 75(7):1772–1777. doi: 10.1093/jac/dkaa152.
- Bahar FG, Ohura K, Ogihara T, Imai T. 2012. Species difference of esterase expression and hydrolase activity in plasma. J Pharm Sci. 101(10):3979–3988. doi: 10.1002/jps.23258.
- Balzarini J, Keyaerts E, Vijgen L, Vandermeer F, Stevens M, De Clercq E, Egberink H, Van Ranst M. 2005. Pyridine N-oxide derivatives are inhibitory to the human SARS and feline infectious peritonitis coronavirus in cell culture. J Antimicrob Chemother. 57(3):472–481. doi: 10.1093/jac/dki481.
- Barlough JE, Shacklett BL. 1994. Antiviral studies of feline infectious peritonitis virus in vitro. Vet Rec. 135(8):177–179. doi: 10.1136/vr.135.8.177.
- Cho A, Saunders OL, Butler T, Zhang L, Xu J, Vela JE, Feng JY, Ray AS, Kim CU. 2012. Synthesis and antiviral activity of a series of 1′-substituted 4-aza-7,9-dideazaadenosine C-nucleosides. Bioorg Med Chem Lett. 22(8):2705–2707. doi: 10.1016/j.bmcl.2012.02.105.
- Coggins SJ, Norris JM, Malik R, Govendir M, Hall EJ, Kimble B, Thompson MF. 2023. Outcomes of treatment of cats with feline infectious peritonitis using parenterally administered remdesivir, with or without transition to orally administered GS-441524. J Vet Intern Med. 37(5):1772–1783. doi: 10.1111/jvim.16803.
- Cossum PA. 1988. Role of the red blood cell in drug metabolism. Biopharm Drug Dispos. 9(4):321–336. doi: 10.1002/bod.2510090402.
- De Clercq E. 2004. Antivirals and antiviral strategies. Nat Rev Microbiol. 2(9):704–720. doi: 10.1038/nrmicro975.
- Deb S, Reeves AA, Hopefl R, Bejusca R. 2021. ADME and pharmacokinetic properties of remdesivir: its drug interaction potential. Pharmaceuticals. 14(7):655. doi: 10.3390/ph14070655.
- Eastman RT, Roth JS, Brimacombe KR, Simeonov A, Shen M, Patnaik S, Hall MD. 2020. Remdesivir: a review of its discovery and development leading to emergency use authorization for treatment of COVID-19. ACS Cent Sci. 6(5):672–683. doi: 10.1021/acscentsci.0c00489.
- Gordon CJ, Tchesnokov EP, Woolner E, Perry JK, Feng JY, Porter DP, Götte M. 2020. Remdesivir is a direct-acting antiviral that inhibits RNA-dependent RNA polymerase from severe acute respiratory syndrome coronavirus 2 with high potency. J Biol Chem. 295(20):6785–6797. doi: 10.1074/jbc.RA120.013679.
- Green J, Syme H, Tayler S. 2023. Thirty-two cats with effusive or non-effusive feline infectious peritonitis treated with a combination of remdesivir and GS-441524. J Vet Intern Med. 37(5):1784–1793. doi: 10.1111/jvim.16804.
- Hartmann K, Ritz S. 2008. Treatment of cats with feline infectious peritonitis. Vet Immunol Immunopathol. 123(1–2):172–175. doi: 10.1016/j.vetimm.2008.01.026.
- Hu W-J, Chang L, Yang Y, Wang X, Xie Y-C, Shen J-S, Tan B, Liu J. 2021. Pharmacokinetics and tissue distribution of remdesivir and its metabolites nucleotide monophosphate, nucleotide triphosphate, and nucleoside in mice. Acta Pharmacol Sin. 42(7):1195–1200. doi: 10.1038/s41401-020-00537-9.
- Humeniuk R, Mathias A, Cao H, Osinusi A, Shen G, Chng E, Ling J, Vu A, German P. 2020. Safety, tolerability, and pharmacokinetics of remdesivir, an antiviral for treatment of COVID-19, in healthy subjects. Clin Transl Sci. 13(5):896–906. doi: 10.1111/cts.12840.
- Jones S, Novicoff W, Nadeau J, Evans S. 2021. Unlicensed GS-441524-like antiviral therapy can be effective for at-home treatment of feline infectious peritonitis. Animals. 11(8):2257. doi: 10.3390/ani11082257.
- Jordheim LP, Durantel D, Zoulim F, Dumontet C. 2013. Advances in the development of nucleoside and nucleotide analogues for cancer and viral diseases. Nat Rev Drug Discov. 12(6):447–464. doi: 10.1038/nrd4010.
- Kimble B, Coggins SJ, Norris JM, Thompson MF, Govendir M. 2023. Quantification of GS-441524 concentration in feline plasma using high performance liquid chromatography with fluorescence detection. Vet Q. 43(1):1–9. doi: 10.1080/01652176.2023.2246553.
- Krentz D, Zenger K, Alberer M, Felten S, Bergmann M, Dorsch R, Matiasek K, Kolberg L, Hofmann-Lehmann R, Meli ML, et al. 2021. Curing cats with feline infectious peritonitis with an oral multi-component drug containing GS-441524. Viruses. 13(11):2228. doi: 10.3390/v13112228.
- Li B, Sedlacek M, Manoharan I, Boopathy R, Duysen EG, Masson P, Lockridge O. 2005. Butyrylcholinesterase, paraoxonase, and albumin esterase, but not carboxylesterase, are present in human plasma. Biochem Pharmacol. 70(11):1673–1684. doi: 10.1016/j.bcp.2005.09.002.
- Li Y, Cao L, Li G, Cong F, Li Y, Sun J, Luo Y, Chen G, Li G, Wang P, et al. 2022. Remdesivir metabolite GS-441524 effectively inhibits SARS-CoV-2 infection in mouse models. J Med Chem. 65(4):2785–2793. doi: 10.1021/acs.jmedchem.0c01929.
- Murphy BG, Perron M, Murakami E, Bauer K, Park Y, Eckstrand C, Liepnieks M, Pedersen NC. 2018. The nucleoside analog GS-441524 strongly inhibits feline infectious peritonitis (FIP) virus in tissue culture and experimental cat infection studies. Vet Microbiol. 219:226–233. doi: 10.1016/j.vetmic.2018.04.026.
- Obach RS, Baxter JG, Liston TE, et al. 1997. The prediction of human pharmacokinetic parameters from preclinical and in vitro metabolism data. J Pharmacol Exp Ther. 283:46–58.
- Pedersen NC, Perron M, Bannasch M, Montgomery E, Murakami E, Liepnieks M, Liu H. 2019. Efficacy and safety of the nucleoside analog GS-441524 for treatment of cats with naturally occurring feline infectious peritonitis. J Feline Med Surg. 21(4):271–281. doi: 10.1177/1098612X19825701.
- Pedersen NC. 2014. An update on feline infectious peritonitis: diagnostics and therapeutics. Vet J. 201(2):133–141. doi: 10.1016/j.tvjl.2014.04.016.
- Périgaud C, Gosselin G, Imbach JL. 1992. Nucleoside analogues as chemotherapeutic agents: a review. Nucleosides Nucleotides. 11(2–4):903–945. doi: 10.1080/07328319208021748.
- Rudakova EV, Boltneva NP, Makhaeva GF. 2011. Comparative analysis of esterase activities of human, mouse, and rat blood. Bull Exp Biol Med. 152(1):73–75. doi: 10.1007/s10517-011-1457-y.
- Sheahan TP, Sims AC, Graham RL, Menachery VD, Gralinski LE, Case JB, Leist SR, Pyrc K, Feng JY, Trantcheva I, et al. 2017. Broad-spectrum antiviral GS-5734 inhibits both epidemic and zoonotic coronaviruses. Sci Transl Med. 9(396):eaal3653. doi: 10.1126/scitranslmed.aal3653.
- Tempestilli M, Caputi P, Avataneo V, Notari S, Forini O, Scorzolini L, Marchioni L, Ascoli Bartoli T, Castilletti C, Lalle E, et al. 2020. Pharmacokinetics of remdesivir and GS-441524 in two critically ill patients who recovered from COVID-19. J Antimicrob Chemother. 75(10):2977–2980. doi: 10.1093/jac/dkaa239.
- Tempestilli M, Stazi GV, Maffongelli G, Marini MC, Ascoli Bartoli T, Ippolito G, Nicastri E, Marchioni L, Agrati C. 2021. Plasma concentrations of remdesivir metabolite in a critical COVID-19 patient needing continuous venovenous haemodialysis. Eur J Clin Pharmacol. 77(10):1583–1585. doi: 10.1007/s00228-021-03128-7.
- VEKLURY Prescriber Information. 2022. Gilead Sciences I, Foster City, CA 94404, ed. Gilead Sciences.
- Warren TK, Jordan R, Lo MK, Ray AS, Mackman RL, Soloveva V, Siegel D, Perron M, Bannister R, Hui HC, et al. 2016. Therapeutic efficacy of the small molecule GS-5734 against Ebola virus in rhesus monkeys. Nature. 531(7594):381–385. doi: 10.1038/nature17180.
- Weiss RC, Cox NR, Martinez ML. 1993. Evaluation of free or liposome-encapsulated ribavirin for antiviral therapy of experimentally induced feline infectious peritonitis. Res Vet Sci. 55(2):162–172. doi: 10.1016/0034-5288(93)90076-r.
- Williamson BN, Feldmann F, Schwarz B, Meade-White K, Porter DP, Schulz J, van Doremalen N, Leighton I, Yinda CK, Pérez-Pérez L, et al. 2020. Clinical benefit of remdesivir in rhesus macaques infected with SARS-CoV-2. Nature. 585(7824):273–276. doi: 10.1038/s41586-020-2423-5.
- Xie J, Wang Z. 2021. Can remdesivir and its parent nucleoside GS-441524 be potential oral drugs? An in vitro and in vivo DMPK assessment. Acta Pharm Sin B. 11(6):1607–1616. doi: 10.1016/j.apsb.2021.03.028.
- Yan VC, Muller FL. 2021. Why remdesivir failed: preclinical assumptions overestimate the clinical efficacy of remdesivir for COVID-19 and Ebola. Antimicrob Agents Chemother. 65(10):e01117–01121. doi: 10.1128/AAC.01117-21.
- Yates M-R, Katherine L. 2018. The evolution of nucleoside analogue antivirals: a review for chemists and non-chemists. Part 1: early structural modifications to the nucleoside scaffold. Antiviral Res. 154:66–86. doi: 10.1016/j.antiviral.2018.04.004.