Abstract
Objectives
Current evidence indicates that the pharmacokinetic profile of rivaroxaban is not significantly impacted by body weight. However, real-world data are needed to better assess the potential clinical benefits and risks associated with rivaroxaban in non-valvular atrial fibrillation (NVAF) patients with obesity. Thus, our objectives were to assess the real-world effectiveness and safety of rivaroxaban versus warfarin among NVAF patients with obesity in the US nationally representative commercially-insured population.
Methods
Health insurance claims data from the IQVIA PharMetrics Plus database (January 2010–September 2019) were used to identify NVAF patients with obesity (based on diagnosis codes) initiated on rivaroxaban or warfarin. Inverse probability of treatment weighting (IPTW) was used to adjust for imbalances between groups. Study outcomes of interest were evaluated up to 36 months post-treatment initiation and included the composite of stroke or systemic embolism (stroke/SE) and major bleeding. Outcomes were compared using Cox proportional hazards regression models with hazard ratios (HR) and 95% confidence intervals (CIs).
Results
A total of 10,555 patients were initiated on rivaroxaban and 5080 patients on warfarin. Following IPTW, the risk of stroke/SE was 26% lower among patients prescribed rivaroxaban relative to warfarin (HR: 0.74, 95% CI: 0.60, 0.91, p = .004) at 36 months. Rivaroxaban-initiated patients had a risk of major bleeding similar to that of warfarin-initiated patients (HR: 0.85, 95% CI: 0.71, 1.02, p = .085).
Conclusions
These results suggest that rivaroxaban is an effective and safe treatment option among NVAF patients with obesity in a commercially-insured US population.
Introduction
Thromboembolic complications, such as stroke and systemic embolism (SE), are common consequences of impaired blood flow in non-valvular atrial fibrillation (NVAF), a cardiac arrhythmia characterized by irregular and uncoordinated contractions of the heart’s atriaCitation1–3. Obesity is the second most common risk factor associated with AF and is implicated as a major driver in the increasing prevalence of this diseaseCitation4. Data from the Framingham Heart Study showed that the risk of developing AF increases by 4% for every unit (kg/m2) increase in body mass index (BMI)Citation5. Although AF is predominantly a disease of the elderly (mean age ≥70 years)Citation6–9, obesity has been associated with a 2- to 3-fold higher risk of incident AF in younger individuals (mean age ranging between 20 and 55 years across studies)Citation10–13, even in the absence of additional predisposing risk factorsCitation12.
Although pharmacological therapies are available to assist in the clinical management of AF, variations in pharmacological characteristics across different BMIs are believed to pose potential problems for obese patients, as obesity is generally known to impact the pharmacokinetic profile of several pharmacologic agentsCitation14. Treatment with direct oral anticoagulants (DOACs) has been shown to mitigate the consequences resulting from stroke/SE in patients with AFCitation15. In 2016, the International Society on Thrombosis and Haemostasis (ISTH) advised against the use of DOACs in morbidly obese patients (i.e. BMI > 40 kg/m2) due to the paucity of clinical data in this patient populationCitation16. However, since this advisement, growing evidence in support of the safety and efficacy of DOACs in obese patients has emerged. In particular, emerging evidence suggests that DOACs are associated with a similar or lower risk of stroke/SE or major bleeding relative to the vitamin K antagonist warfarin in morbidly obeseCitation17–22 and obeseCitation23 patients with AF. However, much of this evidence is based on pooled data from patients who initiated different DOACsCitation17,Citation18,Citation20, despite evidence that not all DOACs have the same pharmacokinetic profile in obese patientsCitation24. Further, the safety of DOACs among patients with extremely high body weight/BMI (body weight >150 kg or BMI ≥ 50 kg/m2) remains unclear due to a lack of pharmacokinetic and clinical data in this populationCitation25.
While much attention has been justifiably focused on the challenges of anticoagulant treatment in elderly AF patientsCitation26, the unmet treatment needs of younger populations should not be overlooked. The incidence and prevalence of AF in the US population has been shown to increase despite age-adjustmentCitation27–29, with incidence rates also increasing among younger subgroupsCitation29. Large-scale cohort studies have also observed that higher BMI is more common among younger patients compared to age-typical AF patientsCitation4,Citation30. Thus, the comparative effectiveness and safety of rivaroxaban and warfarin among younger patient populations merits consideration.
Currently, available evidence suggests that the pharmacokinetic profile of the DOAC rivaroxaban is not altered in patients with high body weightCitation31. Although there is a growing body of literature regarding the clinical benefits associated with rivaroxaban in obese patientsCitation23, additional real-world evidence is needed. Real-world evidence is derived from observational data generated during routine clinical practice (e.g. electronic health records, claims and billing activities, etc.). As such, it provides valuable insights regarding the safety and effectiveness of treatments outside the context of randomized controlled trials and is increasingly used to inform health care decisionsCitation32. To this end, the present study was conducted to assess the effectiveness (risk of stroke/SE) and safety (risk of major bleeding) of rivaroxaban as compared to warfarin in a nationally representative commercially-insured population of NVAF patients with obesity in the US.
Methods
Data source
The IQVIA PharMetricsFootnotei Plus data were used (1 January 2010–30 September 2019), which offers a diverse representation of employers, payers and providers, and geographic zones covering all 50 states in the US. It contains information on ∼40 million patients, with medical and pharmacy benefits available in any given recent year. The enrollee population in the IQVIA PharMetrics Plus data is generally representative of the less-than-65 years of age, commercially-insured population in the US with respect to both age and sexCitation33. The database contains historical information on patient demographics, plan enrollment, and claims for inpatient, outpatient, and pharmacy and their associated costs. Data are de-identified and comply with the Health Insurance Portability and Accountability Act.
Study design and study population
A retrospective, observational weighted-cohort study was conducted. The date of initiation of rivaroxaban or warfarin was defined as the index date. Patient characteristics were evaluated during the 12-month period preceding the index date (i.e. baseline period).
Given the approval of rivaroxaban in November 2011, that it may take a certain amount of time for recently approved medications to be prescribed, and that early adopters tend to be different, only patients with an index date on or after 1 January 2013 were included in the study population. Additional inclusion criteria were the following: ≥12 months of continuous health plan enrollment before the index date, ≥1 medical claim with a diagnosis of AF (International Classification of Diseases, Ninth/Tenth Revision, Clinical Modification [ICD-9-CM]: 427.31 or ICD-10-CM: I48.0x–148.2x, 148.91) during the baseline period or on the index date, ≥1 medical claim with a diagnosis of obesity or a BMI ≥30kg/m2 (see Table S1 for a complete list of BMI-related ICD diagnosis codes used to define obesity) during the baseline period or on the index date, and ≥18 years of age at the index date.
Patients were excluded from the analysis if they had any of the following: pharmacy dispensing for >1 oral anticoagulant on the index date; ≥1 pharmacy claim for an oral anticoagulant during the baseline period; ≥1 medical claim for venous thromboembolism, or knee or hip replacement surgery during the baseline period; ≥1 medical claim with a diagnosis of mitral-stenosis during the baseline period; or ≥1 medical claim for a mechanical heart-valve procedure during the baseline period.
An intention-to-treatment (ITT) approach was used to assess the effectiveness outcome, whereas an on-treatment approach was used to assess the safety outcome. Specifically, the observation (follow-up) period for the effectiveness outcome spanned from the index date until the earliest among health plan disenrollment, end of data availability, or 36 months. For the safety outcome, the follow-up period was censored upon treatment discontinuation or switch to another oral anticoagulant (i.e. to ensure patients were continuously on treatment). Treatment discontinuation was defined as a gap (i.e. grace period) in days of supply of ≥60 days between the end of an anticoagulant dispensing (based on days of supply) and the next fill, or between the end of the last dispensing and end of observation. For the effectiveness outcome, a sensitivity analysis was conducted using an on-treatment approach.
Study outcomes
The effectiveness outcome was the composite of stroke (ischemic or hemorrhagic) or SE (stroke/SE), which was defined as a primary diagnosis of stroke or SE documented in a hospitalization or emergency room visit (see Table S2 for the list of diagnosis codes used to identify stroke/SE). The effectiveness outcomes were also assessed separately (i.e. stroke, ischemic stroke, hemorrhagic stroke, and SE). The safety outcome was the occurrence of a major bleeding event, which was identified using hospitalizations with diagnoses and procedures indicating an episode of bleeding (i.e. Cunningham algorithm)Citation34. Of note, hemorrhagic stroke was also included in the definition of a major bleeding event and included intracranial hemorrhage (ICD-9-CM: 432.x; ICD-10-CM: I62.xx), intracerebral hemorrhage (ICD-9-CM: 431.x; ICD-10-CM: I61.x), and subarachnoid hemorrhage (ICD-9-CM: 430.x; ICD-10-CM: I60.xx).
Statistical analysis
Inverse probability of treatment weighting (IPTW) based on the propensity score (PS) was used to balance the rivaroxaban and warfarin cohorts, with PS defined as the conditional probability of receiving rivaroxaban based on observable covariates. The IPTW approach uses weights derived from the PS to create a pseudo-population, so that the distribution of covariates in the population is independent of treatment assignment. Patients’ weight in each cohort was equal to the inverse of their probability of receiving treatment with rivaroxaban (i.e. 1/PS for the rivaroxaban cohort and 1/[1-PS] for the warfarin cohort). Variables used in the PS calculation included age, sex, year of index date, region, type of insurance plan, morbid obesity, baseline coronary artery disease or peripheral artery disease, baseline stroke/SE, baseline major bleeding, cardiovascular-related medications, cardiovascular procedures, use of non-oral anticoagulants during baseline, number of prescription drugs used during baseline, prior cancer diagnosis and treatment, baseline healthcare resource utilization and healthcare costs, and baseline risk factors for stroke and bleeding events (with a prevalence of ≥5% in either cohort). The PS distributions are reported in Figure S1. Demographic and clinical characteristics balance (including Quan-Charlson comorbidity index score, CHA2DS2-VASc score [congestive heart failure, hypertension, age (>65 = 1 point, >75 = 2 points), diabetes, previous stroke/transient ischemic attack (2 points), vascular disease, and sex category (female)], and HAS-BLED score) was assessed using the standardized difference (std. diff.), with a threshold ≤10% considered a sufficient balanceCitation35.
Time to stroke/SE and time to major bleeding events were assessed with weighted-Kaplan–Meier (KM) survival analysis. Cumulative KM rates were reported at 12, 24, and 36 months following the index date. Study outcomes were also assessed using weighted-Cox proportional hazards regression models, with corresponding hazard ratios (HR), 95% confidence intervals (CI), and p-values. Subgroup analyses were performed stratified by age (<65 years), sex, morbid obesity (i.e. an ICD diagnosis code for morbid obesity/BMI ≥40 kg/m2; see Table S1 for list of codes), and the presence of comorbid diabetes (based on ICD-9-CM codes: 250.x, and ICD-10-CM: E10-E14).
Two sensitivity analyses were conducted to evaluate the robustness of the main analysis. First, a sensitivity analysis using IPTW with weights truncated at the 99th percentile was conducted. Second, a multivariable regression was conducted which did not utilize weights at all. A third sensitivity analysis was conducted that included only patients who were initiated on the standard 20 mg once daily (o.d.) rivaroxaban dose.
Results
After applying all selection criteria, 10,555 patients were included in the rivaroxaban cohort and 5080 patients were included in the warfarin cohort ().
Figure 1. Patient disposition. Abbreviations. AF, atrial fibrillation; BMI, body mass index; ICD, International Classification of Diseases; NVAF, non-valvular atrial fibrillation; VTE, venous thromboembolism. Notes. 1. A total of 326 patients initiated on rivaroxaban with >1 oral anticoagulant medications on the index date were excluded. 2. A total of 379 patients initiated on warfarin with >1 oral anticoagulant medications on the index date were excluded. 3. Continuous eligibility was defined as continuous health plan enrollment with medical and pharmacy coverage. 4. The baseline period was defined as the 12 months prior to the index date. 5. AF was identified with the following ICD-9-CM codes: 427.31, and ICD-10-CM: I48.x.
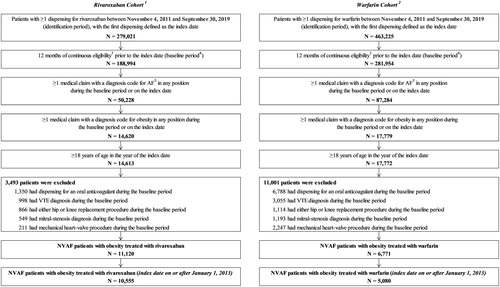
Patient characteristics
In both weighted cohorts, the mean age was 59 years, and approximately 31% of patients were female (). The Quan-Charlson Comorbidity Index was 1.8 in both cohorts. The mean CHA2DS2-VASc score was 2.7 and HAS-BLED score was 1.8 in both cohorts. Overall, approximately 40% of patients in both cohorts were morbidly obese or had evidence of diabetes ().
Table 1. Demographics and clinical characteristics of rivaroxaban and warfarin cohorts.
Risk of stroke and systemic embolism
Rivaroxaban was associated with a 26% lower risk of stroke/SE compared with warfarin over the 36-month follow-up period (HR: 0.74, 95% CI: 0.60, 0.91, p = .004; ). Rates of stroke (i.e. ischemic stroke and hemorrhagic stroke) were significantly lower among patients in the rivaroxaban cohort relative to those in the warfarin cohort at 12 months (HR: 0.64, 95% CI: 0.49, 0.85, p = .002), 24 months (HR: 0.69, 95% CI: 0.54, 0.87, p = .002), and 36 months (HR: 0.73, 95% CI: 0.59, 0.91, p = .005) as were rates of hemorrhagic stroke at 12 months (HR: 0.41, 95% CI: 0.24, 0.70, p = .001), 24 months (HR: 0.47, 95% CI: 0.30, 0.75, p = .002), and 36 months (HR: 0.55, 95% CI: 0.36, 0.84, p = .006; ). The rates of ischemic stroke were lower among patients in the rivaroxaban cohort compared to those in the warfarin cohort at 12 months (HR: 0.74, 95% CI: 0.55, 0.99, p = .042) and 24 months (HR: 0.78, 95% CI: 0.60, 1.00, p = .049; ).
Figure 2. Rates of stroke/SECitation1 among rivaroxaban vs. warfarin cohorts. Abbreviations. CI, confidence interval; SE, systemic embolism. Notes. 1. Defined as a primary diagnosis of ischemic stroke, hemorrhagic stroke, or SE during a hospitalization or emergency room visit. 2. The number of patients still observed at the specific point in time. 3. The number of stroke/SE events at 12, 24 and 36 months were 133, 189, and 224 in the rivaroxaban cohort and 99, 130, and 142 in the warfarin cohort, respectively.
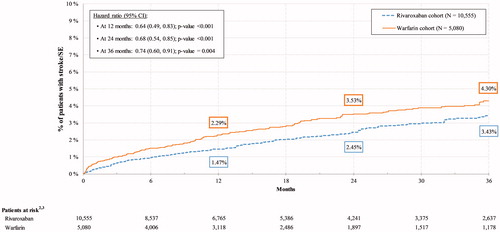
Table 2. Rates of stroke (ischemic and hemorrhagic) and SE among rivaroxaban vs. warfarin cohorts.
Results from the on-treatment sensitivity analysis were consistent with the ITT findings (Table S3). Specifically, the rates of stroke/SE were significantly lower among patients in the rivaroxaban cohort compared to those in the warfarin cohort at 12 months (HR: 0.59, 95% CI: 0.44, 0.80, p < .001), 24 months (HR: 0.64, 95% CI: 0.49, 0.85, p = .002), and 36 months (HR: 0.69, 95% CI: 0.53, 0.90, p = .005).
Risk of major bleeding
Rivaroxaban was associated with a similar risk of major bleeding up to 36 months of follow-up (HR: 0.85, 95% CI: 0.71, 1.02, p = .085). Corresponding rates were also similar for patients in the rivaroxaban cohort compared to those in the warfarin cohort at 12 months (HR: 0.92, 95% CI: 0.75, 1.14, p = .456) and 24 months (HR: 0.90, 95% CI: 0.74, 1.09, p = .266; ).
Figure 3. Rates of major bleedingCitation1,Citation2 among rivaroxaban vs. warfarin cohorts. Abbreviation. CI, confidence interval. Notes. 1. Major bleeding was identified with the Cunningham algorithm, which identifies hospitalizations with diagnoses and procedures indicating an episode of bleeding (excluding bleeding due to major trauma). 2. Evaluated on-treatment, from the index date until the earliest date between discontinuation (defined as the earliest of a gap in days of supply [i.e. ≥60 days] between the end of a dispensing [based on days of supply] and the next fill or between the end of the last dispensing and the end of data), a switch to or addition of another oral anticoagulant, 36 months, health plan disenrollment, or end of data availability. 3. The number of patients still observed at the specific point in time. 4. The number of major bleeding events at 12, 24 and 36 months were 328, 433, and 482 in the rivaroxaban cohort and 173, 225, and 258 in the warfarin cohort, respectively.
![Figure 3. Rates of major bleedingCitation1,Citation2 among rivaroxaban vs. warfarin cohorts. Abbreviation. CI, confidence interval. Notes. 1. Major bleeding was identified with the Cunningham algorithm, which identifies hospitalizations with diagnoses and procedures indicating an episode of bleeding (excluding bleeding due to major trauma). 2. Evaluated on-treatment, from the index date until the earliest date between discontinuation (defined as the earliest of a gap in days of supply [i.e. ≥60 days] between the end of a dispensing [based on days of supply] and the next fill or between the end of the last dispensing and the end of data), a switch to or addition of another oral anticoagulant, 36 months, health plan disenrollment, or end of data availability. 3. The number of patients still observed at the specific point in time. 4. The number of major bleeding events at 12, 24 and 36 months were 328, 433, and 482 in the rivaroxaban cohort and 173, 225, and 258 in the warfarin cohort, respectively.](/cms/asset/d190b991-d5da-419c-aab9-f56a62c8f16f/icmo_a_1901223_f0003_c.jpg)
For the analyses of stroke/SE and major bleeding outcomes, the proportion of patients that was censored due to health plan disenrollment up to 36 months was ∼50% in both cohorts (See Table S4).
Subgroup analyses
Results of the subgroup analyses stratified by age, sex, presence of morbid obesity, and diabetes are presented in Tables S5 and S6. The risk of stroke/SE was significantly lower among male patients in the rivaroxaban cohort compared to those in the warfarin cohort up to 36 months (HR: 0.66, 95% CI: 0.51, 0.87, p = .003). The risk of stroke/SE was significantly lower among patients in the rivaroxaban cohort compared to those in the warfarin cohort among patients <65 years of age (HR: 0.63, 95% CI: 0.48, 0.82, p < .001), morbidly obese (HR: 0.66, 95% CI: 0.48, 0.91, p = .011), and patients with diabetes (HR: 0.67, 95% CI: 0.51, 0.88, p = .003; Table S5).
The rates of major bleeding following treatment initiation were similar among males, females, those aged <65 years, and diabetic patients in the rivaroxaban cohort compared to those in the warfarin cohort (Table S6). The risk of major bleeding was significantly lower among morbidly obese patients in the rivaroxaban cohort compared to those in the warfarin cohort up to 36 months (HR: 0.72, 95% CI: 0.55, 0.95, p = .020; Table S6).
Sensitivity analyses
The sensitivity analyses with IPTW weights truncated at the 99th percentile and the multivariable analysis with no IPTW showed that the effectiveness and safety outcomes favored rivaroxaban over warfarin (Table S7). This indicates that the results obtained in the main analysis were not likely to be driven by extreme weights.
In the sensitivity analysis with IPTW and weights truncated at the 99th percentile, cohort differences in the risk of stroke/SE were similar to the main analysis, while the risk of major bleeding was more favorable for rivaroxaban. In particular, patients treated with rivaroxaban had a significantly lower risk of stroke/SE compared to those treated with warfarin across all time periods. However, the risk of major bleeding was lower among rivaroxaban-treated patients, and this difference attained statistical significance at 36 months (HR: 0.85, 95% CI: 0.73, 0.99, p = .036; Table S7).
The multivariable sensitivity analysis with no IPTW yielded a pattern of results that was similar to the main analysis. Specifically, stroke/SE outcome favored rivaroxaban-treated patients and the results remained significant across all time periods. Using the multivariable analysis, risk of major bleeding was numerically lower among patients treated with rivaroxaban compared to those treated with warfarin, although the difference did not attain statistical significance (Table S7).
In terms of dosage regimens, a total of 9647 (91.4%) rivaroxaban-treated patients were receiving the standard dose (20 mg o.d.). In a sensitivity analysis excluding patients that were not on the standard 20 mg rivaroxaban dose, the effectiveness and safety outcomes were generally consistent with those obtained in the main analysis. In particular, stroke/SE outcomes were significantly in favor of rivaroxaban across all time points (Table S7). At 36 months, the reduction in the risk of stroke/SE was more pronounced among rivaroxaban-treated patients in the sensitivity analysis compared to the main analysis (HR: 0.69, 95% CI: 0.55, 0.86, p < .001 vs. HR: 0.74, 95% CI: 0.60, 0.91, p = .004). There was no difference observed between cohorts for major bleeding events (Table S7).
Discussion
This retrospective cohort study evaluated the effectiveness and safety of rivaroxaban versus warfarin in NVAF patients with obesity in the US. Compared with NVAF patients with obesity treated with warfarin, those treated with rivaroxaban had a significantly lower risk of stroke/SE and a similar risk of major bleeding over up to 36 months of follow-up. In addition, similar findings were consistently found in various subgroups, including morbidly obese patients. These findings suggest that rivaroxaban is a safe and effective treatment option for NVAF patients with obesity and highlight its long-term incremental benefits relative to warfarin. A further strength of the present study is its focus on a commercially-insured cohort of younger patients who are distinct from the general AF population but significantly impacted by obesity as a risk factor.
The results of the present study are consistent with the growing body of evidence supporting the safety and effectiveness of rivaroxaban in NVAF patients with obesity. A post-hoc analysis of the ROCKET AF trial showed that both rivaroxaban and warfarin are effective in stroke prevention across various BMI subgroups with no significant difference among subgroups (interaction p = .537)Citation36. Subsequent observational studies, including the present study, further substantiated this evidenceCitation17–23. Costa et al. analyzed electronic health record data of NVAF patients with obesity and observed that rivaroxaban-treated patients had a 17% and 18% lower risk of stroke/SE (HR: 0.83, 95% CI: 0.73, 0.94) and major bleeding (HR: 0.82, 95% CI: 0.75, 0.89) compared with warfarin-treated patients, respectivelyCitation23. Unlike the present study population, the one analyzed by Costa et al. tended to be older (∼10 years) and encompassed a mix of commercial, Medicare, and uninsured patients rather than focusing on a population that is broadly representative of patients with commercial insurance in the US. A retrospective study by Peterson et al. found similar risks of ischemic stroke/SE (odds ratio: 0.88, 95% CI: 0.60, 1.28) and major bleeding (odds ratio: 0.80, 95% CI: 0.59, 1.08) for rivaroxaban compared to warfarin users among NVAF patients with morbid obesityCitation21. However, these findings were evaluated over a relatively short follow-up period of ∼10 months, which may explain part of the differences with the current study when evaluating patients with morbid obesity. The present study thus builds on previous findings by documenting the benefits of rivaroxaban in relatively young patients with commercial insurance plans. This is particularly relevant in light of prior evidence that obesity was associated with a 40% increase in the risk of incident AF among younger, commercially-insured patients after adjusting for other known AF risk factors (e.g. age, gender, hypertension, and diabetes)Citation37.
The safety and effectiveness of rivaroxaban in patients with obesity are consistent with the pharmacokinetic profile of this agent. Indeed, a literature review summarizing the influence of BMI on rivaroxaban concluded that the pharmacologic profile of rivaroxaban is not affected by body weight or bariatric surgeryCitation31. In contrast, the proportion of warfarin-treated patients who attain an international normalized ratio within therapeutic range was shown to decrease as BMI increasesCitation38. Taken together, these lines of evidence provide a biochemical basis for the clinical observations reported in the current study.
The findings of the present study should be considered within the context of certain limitations. First, obesity was classified based on ICD codes for high BMI and not a patient’s actual BMI value, since height and weight are not available in claims data. As a result of this, it is possible that some NVAF patients with obesity may not have been captured in this analysis. However, patients with diagnosis codes for obesity are highly likely to be obese (i.e. high positive predictive value and high specificity)Citation39–42. A study published in 2019 that assessed electronic medical records and claims data from newly treated patients with AF found that obesity diagnosis codes had high positive predictive value, high specificity, and modest sensitivityCitation42. Of note, within the obesity definition (BMI ≥ 30 kg/m2) patients with different weight or BMI categories are not differentiated. Second, the present analysis could not account for death as a competing risk factor for the study endpoints since information on mortality was not available in the data source. Prior evidence suggests that competing risk of death is an important consideration in time-to-event analyses of older patients (e.g. median age of 80 yearsCitation43), as they are at particularly high risk of near-term deathCitation43,Citation44. In contrast, we evaluated clinical outcomes in a younger population with a lower competing risk. Therefore, mortality was likely to have a lesser impact on our analysis compared to previous analyses focused on older populations.
The present study may have also been subject to general limitations inherent in administrative claims-based studies. In particular, administrative claims data depend on complete and accurate diagnosis, procedure, and drug codes. As a result, coding inaccuracies may lead to misidentification. Similarly, as a result of omissions, the data may not contain all prescription-related information, particularly medications administered in inpatient settings, and over-the-counter medications, such as aspirin. Further, despite the use of IPTW, results may be impacted by unmeasured confounders. The on-treatment analysis could have been influenced by post-baseline confounding factors since patients who discontinue treatment may have distinct characteristics compared to those who remain on treatment. These potential confounders were not accounted for in the current analysis. The proportion of patients censored at 36 months due to health plan disenrollment was approximately 50% for the rivaroxaban and warfarin cohorts. This suggests that an association between censorship and treatment exposure was unlikely to have biased the results, however, high administrative censoring generally draws associations towards the null and the true magnitude of the cohort differences in the present study may have been underestimated.
Conclusion
In this real-world study, NVAF patients with obesity who were initiated on rivaroxaban had a significantly lower risk of stroke/SE and a similar risk of major bleeding compared with those initiated on warfarin. These results from an observational study of administrative data support the accumulating evidence that rivaroxaban is a safe and effective alternative to warfarin in NVAF patients with obesity in a commercially-insured US population.
Transparency
Declaration of financial and other interest
JSB received consultancy fees from Janssen Scientific Affairs, LLC. AK and VA are employees of Janssen Scientific Affairs, LLC and may own stock or stock options. KTM is an employee of Janssen Pharmaceuticals, Inc. and may own stock or stock options. FL, DL, YJ, and PL are employees of Groupe d’analyse, Ltée, a consulting firm that received consulting fees from Janssen Scientific Affairs, LLC for the conduct of this study.
Author contribution
Conceptualization: JSB, VA, AK, KTM.
Data curation and study design: All authors.
Formal analysis: FL, DL, YJ, PL.
All authors contributed to writing and editing the completed work.
Supplemental Material
Download MS Word (104.4 KB)Acknowledgements
Medical writing support was provided by Samuel Rochette, Gloria DeWalt, and Mona Lisa Chanda, who are employees of Analysis Group, Inc. Support for this assistance was provided by Janssen Scientific Affairs, LLC.
Data availability statement
The data analyzed in this study are not publicly available due to licensing agreement with IQVIA.
Additional information
Funding
Notes
i IQVIA PharMetrics is a registered trademark of IQVIA Inc. in the United States, the European Union, and various other countries.
References
- Lip GY, Fauchier L, Freedman SB, et al. Atrial fibrillation. Nat Rev Dis Primers. 2016;2:16016.
- Center for Disease Control and Prevention. Atrial fibrillation. [cited 2020 Sep 11]. Available from: https://www.cdc.gov/heartdisease/atrial_fibrillation.htm.
- Lip GY, Banerjee A, Lagrenade I, et al. Assessing the risk of bleeding in patients with atrial fibrillation: the Loire Valley Atrial Fibrillation project. Circ Arrhythm Electrophysiol. 2012;5(5):941–948.
- Vyas V, Lambiase P. Obesity and atrial fibrillation: epidemiology, pathophysiology and novel therapeutic opportunities. Arrhythm Electrophysiol Rev. 2019;8(1):28–36.
- Wang TJ, Parise H, Levy D, et al. Obesity and the risk of new-onset atrial fibrillation. JAMA. 2004;292(20):2471–2477.
- Feinberg WM, Blackshear JL, Laupacis A, et al. Prevalence, age distribution, and gender of patients with atrial fibrillation. Analysis and implications. Arch Intern Med. 1995;155(5):469–473.
- Khairallah F, Ezzedine R, Ganz LI, et al. Epidemiology and determinants of outcome of admissions for atrial fibrillation in the United States from 1996 to 2001. Am J Cardiol. 2004;94(4):500–504.
- Lane DA, Skjoth F, Lip GYH, et al. Temporal trends in incidence, prevalence, and mortality of atrial fibrillation in primary care. J Am Heart Assoc. 2017;6(5):e005155.
- Miyasaka Y, Barnes ME, Gersh BJ, et al. Secular trends in incidence of atrial fibrillation in Olmsted County, Minnesota, 1980 to 2000, and implications on the projections for future prevalence. Circulation. 2006;114(2):119–125.
- Berkovitch A, Kivity S, Klempfner R, et al. Body mass index and the risk of new-onset atrial fibrillation in middle-aged adults. Am Heart J. 2016;173:41–48.
- Schmidt M, Botker HE, Pedersen L, et al. Comparison of the frequency of atrial fibrillation in young obese versus young nonobese men undergoing examination for fitness for military service. Am J Cardiol. 2014;113(5):822–826.
- Karasoy D, Bo Jensen T, Hansen ML, et al. Obesity is a risk factor for atrial fibrillation among fertile young women: a nationwide cohort study. Europace. 2013;15(6):781–786.
- Tedrow UB, Conen D, Ridker PM, et al. The long- and short-term impact of elevated body mass index on the risk of new atrial fibrillation the WHS (women's health study). J Am Coll Cardiol. 2010;55(21):2319–2327.
- May M, Schindler C, Engeli S. Modern pharmacological treatment of obese patients. Ther Adv Endocrinol Metab. 2020;11:2042018819897527.
- Dzeshka MS, Lip GY. Non-vitamin K oral anticoagulants in atrial fibrillation: where are we now? Trends Cardiovasc Med. 2015;25(4):315–336.
- Martin K, Beyer-Westendorf J, Davidson BL, et al. Use of the direct oral anticoagulants in obese patients: guidance from the SSC of the ISTH. J Thromb Haemost. 2016;14(6):1308–1313.
- Kalani C, Awudi E, Alexander T, et al. Evaluation of the efficacy of direct oral anticoagulants (DOACs) in comparison to warfarin in morbidly obese patients. Hosp Pract. 2019;47(4):181–185.
- Kido K, Ngorsuraches S. Comparing the efficacy and safety of direct oral anticoagulants with warfarin in the morbidly obese population with atrial fibrillation. Ann Pharmacother. 2019;53(2):165–170.
- Kushnir M, Choi Y, Eisenberg R, et al. Efficacy and safety of direct oral factor Xa inhibitors compared with warfarin in patients with morbid obesity: a single-centre, retrospective analysis of chart data. Lancet Haematol. 2019;6(7):e359–e365.
- Patil T, Lebrecht M. A single center retrospective cohort study evaluating use of direct oral anticoagulants (DOACs) in morbidly obese veteran population. Thromb Res. 2020;192:124–130.
- Peterson ED, Ashton V, Chen YW, et al. Comparative effectiveness, safety, and costs of rivaroxaban and warfarin among morbidly obese patients with atrial fibrillation. Am Heart J. 2019;212:113–119.
- Perales IJ, San Agustin K, DeAngelo J, et al. Rivaroxaban versus warfarin for stroke prevention and venous thromboembolism treatment in extreme obesity and high body weight. Ann Pharmacother. 2020;54(4):344–350.
- Costa OS, Beyer-Westendorf J, Ashton V, et al. Effectiveness and safety of rivaroxaban versus warfarin in obese nonvalvular atrial fibrillation patients: analysis of electronic health record data. Curr Med Res Opin. 2020;36(7):1081–1088.
- Sebaaly J, Kelley D. Direct oral anticoagulants in obesity: an updated literature review. Ann Pharmacother. 2020;54(11):1144–1158.
- De Caterina R, Lip GYH. The non-vitamin K antagonist oral anticoagulants (NOACs) and extremes of body weight-a systematic literature review. Clin Res Cardiol. 2017;106(8):565–572.
- Fava JP, Starr KM, Ratz D, et al. Dosing challenges with direct oral anticoagulants in the elderly: a retrospective analysis. Ther Adv Drug Saf. 2018;9(8):405–414.
- Ball J, Carrington MJ, McMurray JJ, et al. Atrial fibrillation: profile and burden of an evolving epidemic in the 21st century. Int J Cardiol. 2013;167(5):1807–1824.
- Jackson SL, Tong X, Yin X, et al. Emergency department, hospital inpatient, and mortality burden of atrial fibrillation in the United States, 2006 to 2014. Am J Cardiol. 2017;120(11):1966–1973.
- Williams BA, Chamberlain AM, Blankenship JC, et al. Trends in atrial fibrillation incidence rates within an integrated health care delivery system, 2006 to 2018. JAMA Netw Open. 2020;3(8):e2014874.
- Pandey A, Gersh BJ, McGuire DK, et al. Association of body mass index with care and outcomes in patients with atrial fibrillation: results from the ORBIT-AF Registry. JACC Clin Electrophysiol. 2016;2(3):355–363.
- Moore KT, Kroll D. Influences of obesity and bariatric surgery on the clinical and pharmacologic profile of rivaroxaban. Am J Med. 2017;130(9):1024–1032.
- United States Food and Drug Administration. Real-world data (RWD) and real-world evidence (RWE) are playing an increasing role in health care decisions. 2020. [cited 2020 Feb 11]. https://www.fda.gov/science-research/science-and-research-special-topics/real-world-evidence.
- IQVIA™ Adjudicated health plan claims user guide – September 2019. https://www.iqvia.com/library/fact-sheets/iqvia-pharmetrics-plus
- Cunningham A, Stein CM, Chung CP, et al. An automated database case definition for serious bleeding related to oral anticoagulant use. Pharmacoepidemiol Drug Saf. 2011;20(6):560–566.
- Austin PC. Using the standardized difference to compare the prevalence of a binary variable between two groups in observational research. Commun Stat Simul Comput. 2009;38(6):1228–1234.
- Patel MR, Mahaffey KW, Garg J, et al. Rivaroxaban versus warfarin in nonvalvular atrial fibrillation. N Engl J Med. 2011;365(10):883–891.
- Foy AJ, Mandrola J, Liu G, et al. Relation of obesity to new-onset atrial fibrillation and atrial flutter in adults. Am J Cardiol. 2018;121(9):1072–1075.
- Wallace JL, Reaves AB, Tolley EA, et al. Comparison of initial warfarin response in obese patients versus non-obese patients. J Thromb Thrombolysis. 2013;36(1):96–101.
- Martin BJ, Chen G, Graham M, et al. Coding of obesity in administrative hospital discharge abstract data: accuracy and impact for future research studies. BMC Health Serv Res. 2014;14:70.
- Lloyd JT, Blackwell SA, Wei II, et al. Validity of a claims-based diagnosis of obesity among Medicare beneficiaries. Eval Health Prof. 2015;38(4):508–517.
- Ammann EM, Kalsekar I, Yoo A, et al. Validation of body mass index (BMI)-related ICD-9-CM and ICD-10-CM administrative diagnosis codes recorded in US claims data. Pharmacoepidemiol Drug Saf. 2018;27(10):1092–1100.
- Jain R, Watzker A, Luo X, et al. Validation of obesity coding among newly treated nonvalvular atrial fibrillation patients using an integrated electronic medical record and claims database. Curr Med Res Opin. 2020;36(2):189–197.
- Berry SD, Ngo L, Samelson EJ, et al. Competing risk of death: an important consideration in studies of older adults. J Am Geriatr Soc. 2010;58(4):783–787.
- Abdel-Qadir H, Fang J, Lee DS, et al. Importance of considering competing risks in time-to-event analyses: application to stroke risk in a retrospective cohort study of elderly patients with atrial fibrillation. Circ Cardiovasc Qual Outcomes. 2018;11(7):e004580.