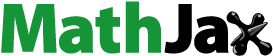
ABSTRACT
About 9 wt.% Cr steels are widely used in the design and fabrication of thick section components in combined cycle or coal-fired applications for working temperatures of 600 ~ 650°C. This family of materials possesses a martensitic microstructure stabilised by precipitates. The presence of nitrides, inclusions or evolution of second-phase particles may increase the metallurgical risk to creep. The chemical composition and microstructural evolution of 9 wt.% Cr steels contribute to thermal stability and long-term performance. In some specialist alloys, Ta is added to the composition which causes the formation of fine MX precipitates which are only present at the nanometre scale in tempered martensite, which hinders the recovery of dislocations and the migration of laths to extend creep life. However, the presence of large Ta-containing particles or inclusions in the 9 wt.% Cr steels may have a detrimental effect on its creep performance, as they may act as preferred sites for cavity nucleation. To fully appreciate the development of damage in these steels, it is necessary to link the pre- and post-test conditions, evaluate damage in the parent metal, develop procedures that provide consistency of results, and obtain statistically relevant data. The evolution of the Ta-containing phase has been tracked and quantified using a variety of correlative characterisation approaches. Utilising focused ion beam microscopy and two-dimensional electron-based microscopic characterisation, three-dimensional tomography has identified a strong relationship between creep cavities and Ta-containing phases from the early stages of creep.
Introduction
Fossil fuelled power plants have and will continue to supply a significant portion of the global electricity generation sectors as global demand for electricity rises. However, environmental concerns are growing, and stricter legislation has been enacted to reduce the release of greenhouse gases into the atmosphere. One way to reduce emissions from power plants is to improve the efficiency of the steam cycle by increasing the temperature and pressure of the steam within the boiler. Modern power plant steam cycles are routinely designed to operate at temperatures and pressures as high as 600°C and 30 MPa, respectively [Citation1]. Due to the high temperatures and pressures involved, high strength 9 wt.% Cr tempered martensitic steels are used [Citation2]. About 9 wt.% Cr steel is chosen as a structural material for fossil-fired supercritical power plants due to its favourable characteristics for hot forming and welding, as well as its promising tensile and creep properties [Citation3]. The long-term performance of high strength 9 wt.% Cr steels is well documented and linked to the formation of fine precipitates and intermetallic phases during creep [Citation4]. Therefore, the significance of accurately measuring precipitates and analysing their distribution over a representative area cannot be overemphasised.
When added to 9 wt.% Cr steels, Ta has been reported to cause the formation of fine MX precipitates [Citation5–8]. MX precipitates are only present at the nanometre scale in tempered martensite, which can hinder the recovery of dislocations and the migration of laths [Citation4]. The creep resistance of 9 wt.% Cr steels is improved due to the presence of these non-shearable MX precipitates that are resistant to coarsening and are finely dispersed [Citation9]. A novel 9 wt.% Cr steel proposed for use in ultra-supercritical boiler tube applications at 650°C is called CPJ7 [Citation10]. Detrois et al. [Citation11] reported two types of Ta-enriched precipitation in as-cast CPJ7 steel. The first being small plate-shaped Ta-containing interdendritic phase which was found at the grain boundaries. Electron probe micro-analysis (EPMA) analysis of this phase revealed partitioning of Ta and Nb, with less substantial partitioning of Si, C, and V; while also showing depletion of Fe, Cr, and Co. The second phases present were Ta2O5 inclusions. This phase is reported to show partitioning in Si, V as well, and it tends to associate with MnS particles [Citation11]. Ninety-five per cent reduction in number density of Ta2O5 was achieved after electroslag remelting (ESR) treatment [Citation11]. The influence of Ta-enriched precipitates on the creep properties of CPJ7 is not clear as a limited amount of research had been carried out on this novel material. Therefore, it has a very important research value for the study of the metallurgical creep risk factors for CPJ7 steel.
Metallographic analysis in this study revealed a strong connection between creep cavities and Ta-enriched particles after approximately 10,000 h of creep exposure, showing the harmful impact of Ta-enriched particles as the preferred sites for cavity formation. Subsequently, 2D and 3D examinations of Ta-enriched particles were carried out to document changes during creep, comparing pre-test and post-test conditions. The resulting data, including evidence of void nucleation at Ta-enriched particles in the pre-test stage, support the explanation of the harmful effect of large Ta-enriched particles in 9 wt.% Cr steels. The aim of this research is to provide improved solutions for alloy design and heat treatment and to emphasise the thermo-mechanical practices that will minimise the risk of large Ta-enriched particle formation in 9 wt.% Cr steels.
Material and experimental details
Materials
The material investigated in this study was a novel tempered martensitic steel called CPJ7 developed by the National Energy Technology Laboratory (NETL), using vacuum induction melting (VIM) and electroslag remelting (ESR) techniques, hot worked using steps of forging followed by hot rolling [Citation10], and creep tested by EPRI (The Electric Power Research Institute). The normalising heat treatment was carried out by heating to 1150°C and holding for 1 h before cooling the material to room temperature by forced air cooling. This was followed by a conventional tempering heat treatment which consisted of heating to 700°C for 1 h before cooling to room temperature using forced air cooling. The chemical composition of this CPJ7 steel is listed in with Fe balanced in weight percentage (wt.%).
Table 1. Chemical composition of CPJ7 steel with Fe balanced in weight percentage (wt.%).
An as-manufactured and a creep ruptured CPJ7 samples were provided by EPRI (The Electric Power Research Institute). The creep test conditions are listed in .
Table 2. Creep test and tensile test conditions of CPJ7.
Thermodynamic calculations
The equilibrium phase stability and the impact of various alloying additions in the tested steels were investigated using thermodynamic calculations using Thermocalc TCFE7 database for iron-based alloys. The computations were carried out using equilibrium circumstances and the as-manufactured CPJ7 samples’ compositions, which are shown in . Temperature ranges from 400°C to 1500°C with intervals of 5°C were used for the thermodynamic calculations.
Table 3. Chemical composition of CPJ7 steel for thermodynamic calculations with Fe balanced (wt.%).
Sample preparation
For metallographic examination, samples were mounted on electrically conductive Bakelite and polished to a fine finish. Grinding using SiC pads from 240 to 1200 grit was carried out with water as a lubricant. The diamond suspensions were then used to polish the sample to a 1 µm finish. A final chemo-mechanical polishing technique employing 0.06 µm colloidal silica suspension was then performed.
Scanning electron microscopy
A JEOL 7800F field emission gun SEM (FEGSEM) with Oxford Instruments X-max 80 silicon drift energy-dispersive X-ray spectroscopy (EDS) detector was used for scanning electron microscopy. A backscattered electron detector was used with a primary beam accelerating voltage of 10 kV, a probe current of 10 nA, and a working distance of 8 mm for 2D SEM backscattered electron imaging. For both samples, 49 individual BSE images with a horizontal field width (HFW) of 100 µm were collected and stitched together to create a large-scale montage for particle quantification. The total area analysed for each sample was 0.5 mm2. ImageJ software was used for image analysis to quantitatively analyse the various microstructural features. Fifteen kilovolt accelerating voltage with 15 nA probe current at 8 mm working distance was typically chosen for EDS analysis.
Transmission electron microscopy
An FEI Tecnai™ F20 transmission electron microscope (TEM) was utilised to investigate features that the SEM is unable to resolve. The lamellae for TEM analysis were prepared using an in-situ liftout using an FEI DualBeam™ Nova Nanolab 600 FIB-SEM. A 10 µm-long electron transparent lamella was produced; then, as Ta has an overlapping peak with Cu in the EDS spectrum, it was attached to a Mo grid. The TEM was operated at 200 kV, in scanning transmission electron microscope (STEM) mode. Both a Fischione High-Angle Annular Dark-Field (HAADF) STEM detector and a Gatan Bright Field STEM detector were used to capture images. Chemical analysis was completed with an Oxford Instruments X-Max 80 mm2 windowless EDS detector.
Serial section tomography for 3D reconstruction
An FEI Helios G4 UXe DualBeam p-FIB was used for 3D sample preparation. To select an area for analysis, subsurface pores were located using a BSE detector with an increased primary beam accelerating voltage of 30 kV. This reveals pores just below the surface which is used to avoid uncertainties arising from the mechanical polishing procedure. A platinum layer with a thickness of ~1 µm was deposited onto the identified area of interest to reduce curtaining effects. With a 2.5 µA current, rectangular cuts of 100 µm depths were made in front of and on either side of the region of interest. The front wall was polished using a subsequent cleaning current of 200 nA to provide a clean cross-sectional finish. After attaching the volume of interest to a 36° pre-tilted silicon sample holder, automated serial sectioning and image capture procedures were carried out through FEI Auto Slice and View process. The sample was polishing at 60 nA using the rocking mill feature, 50 nm cuts were made. After every two cuts, the samples were imaged with the BSE detector at 52° at 10 kV, 0.8 nA, with a resolution of 3072 pixels on the horizontal direction. After every six slices, EDS data were collected at 10 kV, 6.4 nA. The sample was sliced into 500 slices with an inter-slice distance of 50 nm, giving a total sampled volume of 8050
50 µm. Commercial software, Dragonfly, was used to process the data stack for three-dimensional reconstruction from the BSE 2D micrograph slices.
Results and discussion
Thermodynamic calculations
The results of the thermodynamic equilibrium calculations are shown in . The phase transition from austenite to ferrite is predicted to happen at ~800°C. Secondary phases such as Laves phase ((Fe,Cr)2(Mo,W,Ta)), M23C6 carbides, Z phase (Cr(V,Nb)N), MX and two types of borides, M3B2 and M2B, are predicted. The formation of Laves phase is essential to stabilise the sub-grain structure during long-term creep at temperatures where the M23C6 carbides become less stable and likely coarsen [Citation12]. MX precipitates are predicted to be close to a TaC composition with some Nb, V, and N.
Identification of phases
For CPJ7 steel in its as-manufactured state, SEM-BSE imaging and EDS analysis () were used to determine which secondary phases are present before creep testing. Laves phase and M23C6 carbides were not found in the as-manufactured condition by SEM-based techniques. The addition of Ta has been reported to cause the formation of fine nano-scale MX precipitates in 9 wt.% Cr steels [Citation5–8]. The tempering treatment contributes to a uniform dispersion of the precipitates among the microstructure [Citation13]. MX precipitates have an enhanced effect on the long-term creep strength of 9 wt.% Cr steels by stabilising the sub-grain boundaries and hindering free dislocation reactions [Citation14]. However, in CPJ7 steel studied here, some of the Ta-enriched particles associated with pores are present at the micro-scale (˃0.5 µm), which has not been reported in other 9 wt.% Cr steels. Ta is a strong carbide forming alloying element; the addition of Ta results in the precipitation of MX phase at a nano scale, which has pinning effect on the dislocations. As shown in , there are three distinct types of Ta-containing particles based on their morphology, chemical composition, and location. These are as follows: 1. large Ta-containing inclusions (˃0.5 µm) which are likely to be associated with casting pores and tend to also be associated with MnS particles; 2. nano to microscale spherical/plate-shaped Ta-containing phase (0.1–0.5 µm) which was found at the grain boundaries and in the matrix; 3. Small needle-shaped nano-scale, ~50–300 nm in length, ˂50 nm in diameter, Ta-containing phase which is found in the matrix. Type 3 Ta-enriched particles have also been examined using TEM for a more detailed analysis.
Figure 2. SEM-BSE micrograph and maps of the as-manufactured CPJ7 sample; (a) BSE micrographs, (b) EDS maps.

shows TEM BF images and EDS maps of the TEM lamella from an as-manufactured CPJ7 sample. are the TEM BF images, where are the TEM-EDS maps. The images and EDS analysis confirm the Ta-enriched particles to be rich in Ta and Nb. The phase which has high Cr in the analysis is likely to be M23C6, which could not be observed using SEM-EDS. M23C6 particles are located along the lath boundaries and with the matrix from . For further confirmation of the identity of the proposed M23C6 phase, selected area diffraction patterns and EBSD would need to be collected. The identification of M23C6 precipitates in long-term creep rupture 9% Cr steel samples has also been found by other researchers [Citation15–17]. From the top of , a Type 1 Ta-enriched inclusion is associated with a MnS inclusion within a cast void. highlights an area which contains the plate-shaped Type 3 Ta-enriched particles and M23C6 precipitates from . From the 2D results, the Ta enriched particles are found either in a needle-like elongated shape or a spherical shape. Further 3D analysis is required to confirm that either they are in different shapes or the same shape from different directions.
Figure 4. TEM micrographs and EDS maps of the as-received CPJ7 sample; (a)&(c) TEM Bright Field (BF) image, (b)&(d) EDS maps.

Large Ta-containing inclusions are also found to be associated with creep damage (). The observation of large Ta-containing inclusions in the gauge region close to the fracture surface suggests that Type 1 Ta-containing inclusions may play a role in the evolution of damage. From , all Type 1 Ta-containing inclusions are associated with creep cavities; however, not all creep cavities have inclusions/particles associated with them. Based on the limitation of 2D metallurgy techniques, this is an observation of the sample from one surface, and there may be artefacts caused by the mechanical polishing process before SEM investigation. Therefore, there is value in studying the metallurgical creep risk factors of the addition of Ta in CPJ7 steel in 3D.
Quantification of phases in 2D
2D SEM-BSE micrographs showed that Laves phase and Ta-enriched particles appear bright, with the Ta-enriched particles brighter than Laves phase particles due to the difference in average atomic weight. Therefore, it is possible to separate Ta-enriched particles from Laves phase and quantify it by a contrast/brightness-based image analysis technique using ImageJ threshold tools. The size distribution histograms of Ta-enriched particles in both samples with a size bigger than 0.1 µm are shown in . A total of 1701 Ta-enriched particles were detected in the as-manufactured sample, with a total area of 106 µm2, which covers 0.021% of the total analysed area. In the gauge section of the creep-ruptured sample, 1578 particles were detected with a total area of 120 µm2, which covers 0.024% of the total analysed area. The total area fraction of Ta-enriched particles in the creep ruptured sample is marginally larger than that in the as-manufactured sample, whereas the number of particles is fewer. This indicates that there may be an evolution of Ta-enriched particles during creep exposure. For the size range of 0.1 µm−0.3 µm, 1316 Ta-enriched particles were detected in the as-manufactured sample, and 1098 were detected in the creep-ruptured sample at its gauge section. There were more small Ta-enriched particles in the as-manufactured sample, and particles are larger in the creep-ruptured sample which has a higher area fraction of Ta-enriched particles. In the as-manufactured sample, there were four Ta-enriched particles whose equivalent circle diameter (ECD) was larger than 1 µm, where the largest example is 1.19 µm in ECD. In the gauge of the creep ruptured sample, six Ta-enriched particles with a size larger than 1 µm ECD were found, where the largest individual had a size of 1.70 µm in ECD. It is important to quantify Ta-enriched particles, as small TaC precipitates are believed to be finely dispersed in the matrix of 9 wt.% Cr steels, acting as obstacles to dislocations and therefore contributing to the high creep resistance [Citation5–8]. Large Ta-containing inclusions are observed to be associated with creep damage in the gauge section of the creep ruptured CPJ7 sample, which may indicate that they are a preferred site for cavity nucleation.
Figure 7. Size distribution of Ta-enriched particles (a) of the as-manufactured CPJ7 sample, (b) at the gauge section of the creep ruptured CPJ7 sample.

shows the distribution size for Laves phase particles in the gauge section of the creep ruptured sample with a size bigger than 0.1 µm. A total of 15,293 Laves phase particles were identified, giving a total area of 3158 µm2, which covers 0.598% of the total analysed area. It was found that 16.7% of the analysed Laves phase particles have a size of between 0.15 and 0.2 µm in ECD. The largest individual Laves phase particle had an ECD of 1.93 µm, which was in the matrix without associating with creep cavities. Although comparing with Ta-enriched particles, there are more Laves phase particles found in the creep sample, Laves phase particles are also found to be evenly distributed in the matrix without showing any association with creep damage or other phases. Divergent opinions on the effect of Laves phase particles on the long-term creep strength of 9 wt.% Cr steels have been reported. It has been reported that Laves phase nucleation and growth rate are slow, but they grow larger than many other precipitates [Citation18]. Some researchers believe that Laves phase has a negative effect on the long-term creep strength of 9 wt.% Cr steels, suggesting that the precipitation of Laves phase removes Mo from the matrix, thereby reducing the solid solution strengthening of Mo [Citation19,Citation20]. However, it has also been reported that Laves phase particles contribute to precipitation strengthening [Citation12]. Therefore, the effect of Laves phase precipitation on the creep strength of 9 wt.% Cr steels depends on the competing outcome of these two strengthening mechanisms.
3D BSE tomography of creep cavities and second-phase particles
Subsurface creep cavities were sectioned using p-FIB and imaged using BSE and EDS to evaluate the creep damage without the potential damage and contamination caused by conventional mechanical surface preparation. shows a BSE micrograph of typical creep cavities along with an EDS spectrum illustrating the chemical composition obtained from a Ta-containing particle within the cavities of the CPJ7 sample after 10,526 h of creep exposure at 650°C. In BSE micrographs, Ta-enriched precipitates show a distinct contrast differential from the matrix with high brightness. Laves phase particles were observed in the creep rupture sample, but there was no connection between Laves phase particles and creep damage from 2D or 3D results. The Ta-enriched phase has a higher grey-scale level than Laves phase, which makes it possible to separate the phases from the matrix by grey-scale value thresholding.
Association of Ta enriched particles with creep cavities
The locations of large Ta2O5 inclusions are associated with the location of creep cavities in . A detailed comparison between the locations of Ta-enriched particles and creep cavities revealed that 99 Ta-enriched particles were adjacent to 35 out of 97 creep cavities observed in the analysed area of the creep-ruptured CPJ7 sample at its gauge section from the 2D results. It reveals that 36% of the creep cavities observed from the 2D SEM examination were associated with Ta-enriched particles, and some of the creep cavities are associated with more than one Ta-enriched particle. shows the size distribution histogram of Ta-enriched particles associated with creep cavities in the creep ruptured CPJ7 sample based on 2D imaging. Ninety-nine out of 1578 particles were associated with creep cavities, which is 6% in the total counts and 20% of the total Ta-enriched particle area fraction. It indicates that large particles are more likely to be the risk factor for creep cavity nucleation sites. All the large Ta-enriched particles with an ECD larger than 0.95 µm were found to be associated with creep damage.
Figure 10. Size distribution of Ta enriched particles associated with creep cavities in the creep ruptured CPJ7 sample.

Figure 11. 3D reconstructed model from BSE slices of the creep ruptured CPJ7 samples; pink = Type 1/Type 2 Ta-enriched particles; green = creep cavities.

A detailed 3D reconstructed BSE image is presented to show the association between Ta-enriched particles and creep cavities in . This figure represents a reconstructed 3D model of Type 1 and Type 2 Ta-enriched particles (both in pink/light colour) with creep cavities (in green/dark colour). Ta-enriched particles smaller than 0.5 μm are excluded from the reconstruction to show a clearer relationship between Type 1 and 2 particles and the creep damage. All 21 of the subsurface creep cavities show a connection with Type 1 Ta-enriched particles in the BSE micrographs taken from the cross-section. According to EDS and BSE results, the Type 1 Ta-enriched particles observed in all cases are inside creep cavities and have a chemical composition that is enriched in Ta and Nb. The impact of Type 1 Ta-enriched particles on the nucleation of creep damage has been underlined by this observation. The cavities were typically irregular in shape and ranged in size from 1 to 10 μm in ECD, where the Type 1 Ta-enriched particles are typically ~1–2 μm in ECD. The maximum-sized creep cavity has an ECD of 9.64 μm and a total volume of 29.85 μm3. The precipitation of Ta enriched particles in the matrix is concentrated along the boundaries of the martensitic structure. The Ta-enriched precipitates within the cavities are attached to the inner cavity walls. The Ta-enriched precipitates in the matrix are preferentially distributed along the prior austenite grain boundaries (PAGBs) and martensitic substructure boundaries.
Conclusion
CPJ7 was subjected to vacuum induction melting (VIM) and electroslag remelting (ESR) using standard industrial practices at a laboratory scale using NETL. The evolution of the Ta-containing particles in CPJ7 has been tracked and quantified using a variety of correlative characterisation approaches. Utilising focused ion beam serial sectioning and two-dimensional electron-based microscopic characterisation, three-dimensional tomography has identified a strong relationship between creep cavities and Ta-containing phases from the early stages of creep. The results from the present study highlight the importance of Ta-containing particles or inclusions in the 9 wt.% Cr steels. It has been observed that they are associated with creep damage, which may suggest that they act as preferred sites for cavity nucleation. Despite efforts to reduce the presence of Ta-enriched particles through electroslag remelting (ESR), there remains a potential risk associated with their presence in this novel 9 wt.% Cr steel. These findings underscore the importance for steel manufacturers to exercise strict control over composition, steel-making techniques, forging processes, and final heat treatment in order to achieve a desirable concentration of Ta and minimise the associated risks. This information provides valuable insights for ongoing steel development and improvement endeavours.
Acknowledgments
This work was sponsored by the EPRI, Electric Power Research Institute, Palo Alto, California, USA. The authors acknowledge the use of facilities within the Loughborough Materials Characterisation Centre including the Helios PFIB, funded by the EPSRC grant EP/P030599/1
Lastly, the authors kindly thank NETL for donating the material test samples informing this manuscript.
Disclosure statement
No potential conflict of interest was reported by the author(s).
Additional information
Funding
References
- Di Gianfrancesco A. The fossil fuel power plants technology. Elsevier; 2017. doi: 10.1016/b978-0-08-100552-1.00001-4
- Von Hagen I, Bendick W. Creep resistant ferritic steels for power plants. Niobium, Science And Technology. 2001.
- Spiegel M, Schraven P. New austenitic steels for the advanced USC power plants. Elsevier; 2017. doi: 10.1016/b978-0-08-100552-1.00011-7
- Maruyama K, Sawada K, Koike JI. Strengthening mechanisms of creep resistant tempered martensitic steel. ISIJ Inter. 2001 Jun;41(6):641–653. doi: 10.2355/isijinternational.41.641
- Di Gianfrancesco A, Blum R. A-USC programs in the European Union. Materials for Ultra-Supercritical and Advanced Ultra-Supercritical Power Plants. 2017;773–846. doi: 10.1016/b978-0-08-100552-1.00024-5
- Di Gianfrancesco A. New Japanese materials for A-USC power plants. Elsevier; 2017. doi: 10.1016/b978-0-08-100552-1.00013-0
- Zhou JH, Shen YF, Hong YY, et al. Strengthening a fine-grained low activation martensitic steel by nanosized carbides. Mater Sci Eng A. 2020;769(September 2019):138471. doi: 10.1016/j.msea.2019.138471
- Harries DR. High-chromium ferritic and martensitic steels for nuclear applications. 2001. doi: 10.1520/mono3-eb
- Caminada S. “Long term microstructural evolution of 9-12% Cr martensitic steels for advanced USC plants,” New Developments on Metallurgy And Applications of High Strength Steels: Buenos Aires 2008, vols 1 and 2, proceedings, no. Conference on New Developments on Metallurgy and Applications of High Strength Steels. Tenaris, Piazza Caduti 6 Luglio 1944 1, I-24044 Dalmine, BG, Italy, pp. 369±, 2008.
- Hawk JA, Jablonski PD. Heat-resistant advanced 9% Cr steel for fossil energy power generation. Advanced Materials - TechConnect Briefs 2017. 2017;2:152–155.
- Detrois M, Jablonski PD, Hawk JA. Evolution of tantalum content during vacuum induction melting and electroslag remelting of a novel martensitic steel. Metallurgical And Materials Transactions B: Process Metallurgy And Materials Processing Science. 2019;50(4):1686–1695. doi:10.1007/s11663-019-01614-z
- Zhu S, Yang M, Song XL, et al. A few observations on laves phase precipitation in relation to its effects on creep rupture strength of ferritic steels based on Fe–9Cr (wt%) alloys at 650 °C. Mater Sci Eng A. 2014;619:47–56. doi: 10.1016/j.msea.2014.09.059
- Foldyna V, Jakobová A, Vodárek V, et al. Chromium modified steels — metallurgical understanding. Materials For Advanced Power Engineering 1994. 1994. doi: 10.1007/978-94-011-1048-8_37
- Orlová A, Buršík J, Kuchařová K, et al. Microstructural development during high temperature creep of 9% Cr steel. Mater Sci Eng A. 1998;245(1):39–48. doi: 10.1016/S0921-5093(97)00708-9
- Pandey C, Giri A, Mahapatra MM. Evolution of phases in P91 steel in various heat treatment conditions and their effect on microstructure stability and mechanical properties. Mater Sci Eng A. 2016;664:58–74. doi: 10.1016/j.msea.2016.03.132
- Cerjak H, Hofer P, Schaffernak B. Influence of microstructural aspects on the service behaviour of advanced power plant steels. ISIJ Inter. 1999;39(9):874–888. doi: 10.2355/isijinternational.39.874
- Hong SP, Il Kim S, Ahn TY, et al. Effects of extended heat treatment on carbide evolution in Cr-mo steels. Mater Charact. 2016;115:8–13. doi: 10.1016/j.matchar.2016.03.013
- Korcakova L, Hald J, Somers MAJ. Quantification of laves phase particle size in 9CrW steel. Mater Charact. 2001;47(2):111–117. doi:10.1016/s1044-5803(01)00159-0
- Abe F. Creep rates and strengthening mechanisms in tungsten-strengthened 9Cr steels. Mater Sci Eng A-Struct Mater Proper Microstruct Process. 2001;319(12th International Conference on the Strength of Materials (ICSMA 12)):770–773. doi: 10.1016/s0921-5093(00)02002-5
- Abe F. Effect of fine precipitation and subsequent coarsening of Fe2W laves phase on the creep deformation behavior of tempered martensitic 9Cr-W steels. Metallurgical And Materials Transactions A-Physical Metallurgy And Materials Science. 2005;36A(2):321–332. doi: 10.1007/s11661-005-0305-y.