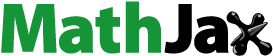
ABSTRACT
Expansive clayey soils at the subgrade level of roads and highways in different cities can affect the performance and durability of pavements. This increases the need for maintenance and conservation work, leading to higher operating costs. Traditionally, clayey soils are stabilised by adding lime or cement. However, the handling and use of these materials are restricted in some Latin American countries due to illegal drug production, and obtaining high-quality materials from distant sources can be impractical. The goal of this study is to determine the effectiveness of incorporating fibres in improving the performance of expansive clayey subgrade soils. To achieve this goal, resilient modulus tests and measurements of compacted soil expansion were performed. Two types of fibres were selected for this study: natural Ichu fibre, which is common in the Peruvian Andes, and polypropylene fibre. The soil used was a high-plasticity clay from the city of Pucallpa, to which varying percentages of fibres were incorporated (0.25%, 0.5% and 1%). The samples were prepared with a gyratory compactor to achieve their maximum dry density as obtained with the standard compaction effort. The results indicated that the incorporation of fibres improved the value of the resilient modulus while significantly reducing soil swelling.
1. Introduction
In recent years, cities across Peru have experienced significant growth, requiring road infrastructure to be built on problematic soils. Traditional construction methods involve replacing problematic soils with higher-quality materials or stabilising them with lime and cement. However, these methods are not always practical in the Amazon region, which comprises more than half of the Peruvian territory. This is due to the lack of sources for appropriate materials and restrictions on the use of lime because of its association with illegal drug production.
The city of Pucallpa, located in the Central East zone of Peru, sits on large semi-consolidated deposits of sandy, silty and mainly clayey soils of alluvial origin from the Quaternary period of the recent Holocene. Constructing any type of road infrastructure on this type of soil is an engineering challenge because the soil has a low bearing capacity and high expansion potential, which leads to cracks, fissures and damage to urban pavements.
An alternative method to improve pavement performance is the use of natural or synthetic fibres as a reinforcing element in the subgrade layer. The reinforcement of soils with different types of fibres has been studied in recent years (Prabakar and Sridhar Citation2002, Akbulut et al. Citation2007, Mohanty et al. Citation2011, Hejazi et al. Citation2012, Plé and Lê Citation2012, Nezhad et al. Citation2021, Ekinci et al. Citation2022); however, the use of subgrade layers for paving purposes is still not widespread.
Recent research has indicated that the shear strength of soil can be improved by 40% when polypropylene fibres are incorporated (Pusari and Rodriguez Citation2020, Arreluce and Solis Citation2021). In addition, polypropylene fibre reinforcement has been found to reduce the swelling potential of expansive clays (Puppala and Musenda Citation2000). Increasing the fibre content has also been found to significantly improve the tensile strength of reinforced soil in both a random and discrete distribution (He et al. Citation2021). The value of the California bearing ratio (CBR) has also been demonstrated to increase up to 25% with the incorporation of 1% polypropylene fibre (Mamani and Lazarte Citation2019). The mechanisms that improve soil resistance are controlled by adhesion and resistance to friction at the interface of the soil with the fibre (Tang et al. Citation2007). The shear strength at the interface depends on the rearrangement of soil particles, the effective contact area between the soil and the fibre, the roughness of the fibre surface, and the composition of the soil (Tang et al. Citation2010).
There are conflicting findings in the technical literature regarding the effect of reinforcing soil with fibres on the deformation modulus. Li and Ding (Citation2002) observed that the elastic modulus of reinforced soil increased with the fibre content. Similarly, Yuan et al. (Citation2019) reported that the addition of fibre to cohesive soil increased the dynamic elastic modulus by 20%, and this increase was dependent on the tension of the fibre. Hojjati and Sarkar (Citation2022) and Gupta et al. (Citation2020) demonstrated an improvement in the elastic modulus of reinforced soil of 40% to 60% compared to that of natural soil. In contrast, Tang et al. (Citation2007) reported that the addition of fibres reduced the initial stiffness modulus of the reinforced soil.
Several models have been proposed to predict the resilient modulus of both cohesive and granular soils based on stress states (Seed et al. Citation1967, Moossazadeh and Witczak Citation1981, Han and Vanapalli Citation2015, Yao et al. Citation2018) or index parameters (Li and Selig Citation1994, Puppala Citation2008, Khoury and Maalouf Citation2018, Chu et al. Citation2021). In this study, the model used in the AASHTOWare Pavement ME Design software, proposed by Witczak for the NCHRP Project 1-28A, was modified to incorporate the effect of fibres on the resilient modulus.
Peru has significant potential for natural fibre, particularly the Stipa Obtusa grass species, also known as Ichu, which grows in the high Andean zone and has excellent physical and mechanical characteristics (Candiotti et al. Citation2020). Therefore, this study aimed to evaluate the effectiveness of incorporating natural Ichu fibre in improving pavement performance on expansive clayey soils. To achieve this objective, a test programme was conducted to determine the resilient modulus of soil reinforced with two different types of fibres: Ichu fibres and polypropylene fibres. Furthermore, the expansion of the fibre-reinforced soil was measured using various compacted soil samples.
2. Materials
2.1. Characteristics of Pucallpa clay
Pucallpa clay is a highly plastic clay, classified as CH in the Unified Soil Classification system (USCS). It has an average liquid limit of approximately 55% and a plastic index varying between 24% and 37% (see ). The specific gravity of Pucallpa clay is 2.57, and the void ratio is on the order of 0.754. The particle size distribution obtained from the sedimentation analysis is presented in . The percentage of particles smaller than 0.002 mm is 35% (clay fraction), and the activity of the clay is on the order of 0.85; therefore, the clay can be classified as having high expansion potential in the Van der Merwe chart (Van der Merwe Citation1964). The maximum dry density is 1650 kg/m3, and the optimum moisture content is 21.3%.
The undrained strength of the clay was determined by unconfined compression tests on undisturbed samples, resulting in an average value of 175 kPa. Direct shear tests were also conducted, which allowed estimation of the effective strength parameters of the clay, with a friction angle (φ’) of 26°. The oedometer tests indicated that Pucallpa clay is a highly compressible soil with a compression index (Cc) of 0.154 and swelling index (Cs) of 0.077 ().
Table 1. Properties of soil used in this study.
2.2. Ichu fibre characteristics
Stipa Obtusa, also known as Ichu, is a perennial grass species native to the Andean highlands. Its stems can grow between 60 and 180 cm in length, and its leaves are erect and resistant, with narrow panicles between 5 and 15 cm (see ). Stipa Obtusa can grow in steep ravines, mountain slopes, plains, hills and riverbanks, thriving in altitudes above 3,300 m above sea level and low-temperature areas.
Stipa Obtusa fibre has been the subject of recent studies to evaluate its potential in different applications. Charca et al. (Citation2015) studied the use of this fibre as a thermal insulation system for constructions in cold areas in the highlands of Peru. Mori et al. (Citation2018) presented a methodology to evaluate the Ichu fibre extraction process and its use as reinforcement in composite materials. It was observed that burning Ichu fibres produced a high level of amorphous silica, which indicates the potential for using the fibres as aggregate ash in cementitious materials. Candiotti et al. (Citation2020) evaluated the mechanical properties of Ichu fibre for its use in composite materials and found that when the fibres were subjected to cyclic deformations, an increase in the fibre modulus occurred.
The tensile strength of the fibres was investigated according to the procedure indicated in the ASTM C1557-14 and ASTM D3822-14 standards. Fifteen samples of different sections of the leaves of the plant, each 10 cm in length, were randomly selected and tested. A Testometric model X500-5027 Universal Testing Machine with a 50-N load cell was employed. The stress–strain curve of the fibre was obtained, and the elastic modulus was determined in the strain range of 0.1% to 0.3%. It should be noted that the mechanical properties of the fibre depend largely on the irregularities or defects present as well as the method chosen to measure the cross-sectional area (CSA). The procedure reported by Hu et al. (Citation2010) was selected to determine the CSA of the fibres tested. For this, a Nikon trinocular microscope and the WinTest Analysis EC6.0.23 software were used. presents the cross section of the fibres tested after failure.
presents the measurement results of the tensile strength of the Ichu fibre. The species selected in this study exhibited a tensile strength that varied between 100 and 300 MPa. The width and thickness of the fibre used in the tests were 6–8 mm and 0.6–1.2 mm, respectively. The specific gravity of the leaves was 2.8.
2.2. Polypropylene fibre characteristics
The synthetic polypropylene fibres used in soil reinforcement had a diameter of 0.75 mm and lengths of 40, 50 and 60 mm. To evaluate the tensile strength of the fibres, 20 samples with a length of 100 mm, diameter of 0.75 mm and density of 5.10 g/cm3 were used. The test was conducted at a speed of 10 mm/min, and the results are presented in . The tensile strength of the fibres tested varied between 500 and 560 MPa. The tensile strength of polypropylene fibres exhibited less dispersion compared to that of Ichu fibre. This can be attributed to the synthetic nature of the polypropylene fibres, which were produced in a factory. In addition, the elongation of polypropylene fibres before failure ranges between 41 and 50 mm while in the case of the natural Ichu fibre it is around 1.6–3.6 mm.
3. Test equipment and procedure
In this study, unconfined compression tests (UCT), resilient modulus tests (Mr) and expansion measurements were conducted on soil samples without fibres and on samples with different percentages of natural Ichu fibres and polypropylene fibres (0.25%, 0.5% and 1%) and different fibre lengths (40, 50 and 60 mm). The samples were carefully prepared prior to testing.
3.1. Sample preparation
Clayey soil from Pucallpa was dried in an oven at a constant temperature of 60 °C for 24 h. Subsequently, lumps were carefully pulverised with a rubber hammer before proceeding with the mixing and compaction of the clay samples containing fibres. Prior to compaction, the prepared soil was mixed with the optimum moisture content (21.3%) and left for one day. The samples were compacted in a gyratory compactor until reaching the maximum dry density (1650 kg/m3) determined by the Proctor Standard test (see ). Samples with a diameter of 100 mm and height of 200 mm were prepared and compacted in three layers. Subsequently, the samples were kept in a humid chamber for 3 days to homogenise the moisture.
3.2. Test programme
3.2.1. Unconfined compression test (UCT)
A Humboldt Master Loader Elite Series HM-5030 load frame with a cell load of 50 kN was used. This device had precise stepper motor control, which enabled testing speeds ranging from 0.00001 to 50.8 mm/min. The load application speed was set at a fixed rate of 1.27 mm/min, and the vertical displacements were measured using a 25-mm range linear variable displacement transducer (LVDT) attached to the loading rod.
The soil was combined with varying percentages of natural Ichu fibres and polypropylene fibres (0%, 0.25%, 0.5% and 1%) and compacted to its maximum dry density. Two samples were tested for each fibre percentage, and the average strength was reported. In addition, three different groups of fibre lengths (40, 50 and 60 mm) were tested.
3.2.2. Resilient modulus test
The resilient modulus is an important parameter related to the structural behaviour of the road, and a higher value of this parameter results in better road performance. Mr was measured using a CONTROLS UTM-30 universal dynamic testing machine with a triaxial chamber that allowed testing samples up to 150 × 300 mm in size with a 30-KN capacity load cell. The equipment met all the requirements indicated in the AASHTO (Citation2017) T-307 standard . A total of 20 test specimens were prepared, in which three fibre lengths (40, 50 and 60 mm) and different fibre percentages (0%, 0.25%, 0.5% and 1%) were tested.
An initial conditioning stage was run by applying 1,000 repetitions of a haversine-shaped load equivalent to a cyclic axial stress of 24.8 kPa with a confining pressure of 41.4 kPa. After conditioning, 15 loading sequences with different combinations of axial and confining pressures were performed.
3.2.3. Expansion measurement
The CBR is one of the most widely used tests for pavement design and is based on the ASTM D-1883 procedure. The CBR test is intended for evaluating soil strength and the expansion of the subgrade. In this study, 30 test soil samples mixed with fibres were compacted in three layers with a standard hammer (24.4-N weight), using the Standard Proctor test (ASTM D-698) as a reference. The specimens were soaked for 4 days with a surcharge of two annular metal weights of 5 lb (2.27 kg) each, and the daily expansion was measured using a mechanical dial indicator capable of reading to 0.001 in (0.025 mm) in a range of 10 mm. The loading stage was run on a Humboldt loading frame with a penetration rate of 1.27 mm/min; however, the results of CBR tests are not discussed in this paper.
4. Results and discussion
In this section, the results of the unconfined compression, expansion and resilient modulus tests are presented and discussed.
4.1. Effect of fibres on the unconfined compressive strength (UCS)
The UCS of the samples containing fibres (qui) was normalised with respect to the resistance of the samples without fibres (qu0). The results indicated that the UCS of the soil increased as the percentage of fibres increased (see ), regardless of the type of fibre used. The length of the fibres did not significantly affect the resistance of the soil in the case of Ichu fibres, whereas it did in the case of polypropylene fibres. The maximum improvement in resistance, up to 1.8 times the initial resistance, was achieved when 1% Ichu fibres were used. However, when polypropylene fibres were added to the soil, the improvement in resistance was significantly greater, with the resistance reaching up to 5.4 times that of the soil without fibres, with a fibre length of 60 mm and fibre percentage of 1%. It should be noted that the length of the polypropylene fibres significantly affected the resistance of the soil, with greater length producing greater resistance. The fibres interact with the soil through a mechanism of adhesion and friction between the soil and the fibre surface. The roughness of the fibre has a significant influence on the strength of the reinforced soil. As seen in the microscope views, the Ichu fibre has less roughness, which explains the lower resistance gained by this fibre compared to the polypropylene fibre.
4.2. Effect of fibres on the resilient modulus
presents the results of the resilient modulus test conducted on a sample without fibres. Different combinations of confining pressure and cyclic deviator stress were used, as indicated by the AASHTO (Citation2017) T-307 standard. The results indicated that the resilient modulus decreased as the deviator stress increased. At higher levels of deviator stress, the resilient modulus tended to be constant, which is a typical behaviour observed in cohesive soils (Kim and Kim Citation2007, Zhang et al. Citation2019). However, the resilient modulus slightly increased as the confining stress was reduced, which is in contrast to the observations in the technical literature (Drumm et al. Citation1990, Lee et al. Citation1997, Zhang et al. Citation2019). This unusual behaviour indicates that some structural adjustments of the soil particles occurred during the loading cycles, influencing the soil stiffness.
displays the resilient modulus of soil samples compacted with different percentages of polypropylene and Ichu fibres. Three groups of samples with different fibre lengths were tested: 40, 50 and 60 mm. The range of modulus values obtained in tests with samples without fibres is also presented in (red dashed line). The results indicate that 40-mm polypropylene fibres did not produce a significant improvement in the resilient modulus of the soil, regardless of the percentage of fibres used. However, as the length of the fibre increased, a significant improvement in the modulus was observed, with at least a doubling of the value of the resilient modulus for a length of 60 mm (see ). An increase in the percentage of fibre for the same fibre length also produced significant improvements in the modulus value, which was more evident in the case of fibres with a length of 60 mm (see ).
Figure 11. Resilient modulus normalised to the resilient modulus of the unreinforced soil (Mr0) in relation to the length of the polypropylene fibre.

Figure 12. Resilient modulus normalised to the resilient modulus of the unreinforced soil (Mr0) in relation to the percentage of the polypropylene fibre.

Different behaviour was observed in the samples containing Ichu fibre. The test results did not indicate an increase in the resilient modulus value as the fibre length increased; on the contrary, the resilient modulus value tended to decrease (). This result indicates that stiffness is not only associated with fibre length, but also with diameter. Parameter that is not possible to control in natural fibres as it can be done with synthetic fibres. As a result, polypropylene fibres show a clear tendency to increase strength that is not observed in Ichu fibres. Regarding the percentage of fibre, the resilient modulus exhibited an increase as the percentage of Ichu fibre increased, achieving an increase of at least 50% when 1% fibre was used in comparison to the case without fibre (see ). It is observed that the percentage of fibres produces the effect of increasing the stiffness of the soil, for any of the fibres tested (Ichu or Polypropylene). Which is explained by the fact that the percentage of fibres is controlled by weight, reducing any dispersed results. Since the fibres are elements that are more rigid than the soil, the greater their quantity, the greater the rigidity of the soil.
Figure 13. Resilient modulus normalised to the resilient modulus of the unreinforced soil (Mr0) in relation to the length of the Ichu fibre.

Figure 14. Resilient modulus normalised to the resilient modulus of the unreinforced soil (Mr0) in relation to the percentage of the Ichu fibre.

Based on the test results, two new linear variables were included in the model adopted by NCHRP Project 1-37A (2004), which is a variation of Witczak and Uzan (Citation1988) equation, to incorporate the effect of fibres on the resilient modulus: f, which represents the fibre percentage, and L, which represents the fibre length. The new model is expressed as follows:
(1)
(1) where k1, k2 and k3 are linear regression coefficients, Pa is the atmospheric pressure and θ is the bulk stress (σ1 + σ2 + σ3). The octahedral shear stress is defined as
in the axisymmetric condition (triaxial stress space), where σd is the deviator stress. The new coefficients a, b and c depend on the type of fibre selected and the soil used. Using the method of least squares, the coefficients for the polypropylene fibres were established, for the case studied, as a = 0.224, b = 0.057 and c = 40, while the coefficients for the Ichu fibres were established as a = 0.731, b = −0.009 and c = 60. These coefficients were used to simulate the tests conducted with both fibres. and present the results of several resilient modulus tests performed on compacted samples with several fibre percentages and the adjustment curve calculated with the proposed model. Good agreement between the model computations and experimental results was observed. The values of coefficients k1, k2 and k3 were calibrated with the data from the experiment without fibres, illustrated in .
3.3. Effect of fibres on soil expansion
The soil samples were mixed with the optimum moisture content determined by the Standard Proctor test and then compacted in CBR moulds using a standard test hammer (24.4 N) with 56, 25 and 10 blows to obtain different dry densities in each test specimen. The soil samples were mixed with fibres at different percentages (0%, 0.25%, 0.50%, 1.0%). As in the resilient modulus tests, Ichu fibres with lengths of 40, 50 and 60 mm were used. After compaction, the samples with the corresponding surcharges were immersed in water, and the expansion was measured over 4 days. The addition of Ichu fibres was found to reduce the expansion of the clayey soil sample by at least four times compared to the sample without fibres, as illustrated in . However, there was no clear trend of further reduction in soil expansion with increasing the fibre percentage or fibre length, possibly due to the dispersion of the fibre strength in Ichu fibres. Similar behaviour is expected to occur with polypropylene fibres.
4. Conclusions
This study evaluated the performance of Ichu and polypropylene fibres in improving the characteristics of a cohesive subgrade. The compressive strength, resilient modulus and soil expansion were evaluated through an extensive laboratory programme. Ichu and polypropylene fibres were used at different percentages (0%, 0.1%, 0.2% and 0.3%) and sizes and mixed with cohesive soil before being compacted to the maximum dry density with optimum moisture content. The following conclusions can be drawn based on the results of this study.
Although natural Ichu fibres have a much greater dispersion in tensile strength than polypropylene fibres, the minimum resistance tested for Ichu fibres was sufficient to improve the behaviour and resistance of the clayey soil subgrade layer used in this project.
The addition of Ichu fibres seems to considerably improve the unconfined compression resistance value of the clayey soil, reaching a value 1.8 times the initial resistance of the soil. For polypropylene fibre, a resistance of 5.4 times the initial resistance was achieved for a fibre length of 60 mm.
The results of the resilient modulus tests on the fibre-reinforced clayey soil varied depending on the confining pressure and deviator stress, following a similar behaviour pattern to the tests without fibres. The resilient moduli obtained for samples reinforced with polypropylene fibres were more stable than those obtained for samples reinforced with Ichu fibres, where greater dispersion was observed in the results. This can be explained by the fact that polypropylene fibre, being an industrial product, has greater homogeneity in its tensile strength. In contrast, greater dispersion in the results was observed for Ichu fibres. Nevertheless, the resilient moduli of Ichu-fibre-reinforced soil were at least 50% higher than non-fibre moduli when using 40-mm-long fibres and a fibre content of 1%. For polypropylene fibre, using 0.25% fibre with a length of 60 mm was sufficient to achieve at least double the resilient modulus obtained without fibre.
The proposed model, which incorporates the variables of fibre length and percentage into the Wictzak model, enabled calculation of the results of resilient modulus tests for soils compacted with fibres using a single set of parameters.
The experiments demonstrated that the inclusion of Ichu fibres in the soil could reduce (by at least four times) the expansion of the clayey subgrade. Therefore, it is recommended to use fibres to improve the behaviour of clayey soil subgrade for improved pavement performance.
Acknowledgements
The authors also thank the staff of the Pavement Laboratory of the University of Lima, including Richard Osorio, Joao Rengifo and Fiorella Barzola, for their assistance with the execution and interpretation of the laboratory tests.
Disclosure statement
No potential conflict of interest was reported by the author(s).
Additional information
Funding
References
- AASHTO, 2017. T-307-99. Standard method of test for determining the resilient modulus of soils and aggregate materials. Washington, USA: American Association of State Highway and Transportation Officials.
- Akbulut, S., Arasan, S., and Kalkan, E., 2007. Modification of clayey soils using scrap tire rubber and synthetic fibers. Applied Clay Science, 38 (1–2), 23–32.
- Arreluce, S. and Solis, G., 2021. Incorporation of polypropylene fibers as a method of strengthening clayey soils in Palian – Huancayo. Thesys. Peruvian University of Applied Sciences (in Spanish).
- Candiotti, S., et al., 2020. Assessment of the mechanical properties of Peruvian Stipa obtusa fibers for their use as reinforcement in composite materials. Composites Part A Applied Science and Manufacturing, 135, 105950.
- Charca, S., et al., 2015. Assessment of Ichu fibers as non-expensive thermal insulation system for the Andean regions. Energy and Buildings, 108, 55–60.
- Chu, X., Dawson, A., and Thom, N., 2021. Prediction of resilient modulus with consistency index for fine-grained soils. Transportation Geotechnics, 31, 100650.
- Drumm, E.C., Boateng-Poku, Y., and Pierce, T.J., 1990. Estimation of subgrade resilient modulus from standard tests. Journal of Geotechnical and Geoenvironmental Engineering–ASCE, 116 (5), 775–789.
- Ekinci, A., Vaz Ferreira, P.M., and Rezaeian, M., 2022. The mechanical behaviour of compacted Lambeth-group clays with and without fibre reinforcement. Geotextiles and Geomembranes, 50 (1), 1–19.
- Gupta, G., Sood, H., and Gupta, P.K., 2020. Mathematical modelling of resilient modulus response of fibre reinforced clay subgrade for pavement design. Journal of Interdisciplinary Mathematics, 23 (1), 247–255. doi:10.1080/09720502.2020.1721920.
- Han, Z. and Vanapalli, S.K., 2015. Model for predicting resilient modulus of unsaturated subgrade soil using soil-water characteristic curve. Canadian Geotechnical Journal, 52 (10), 1605–1619.
- He, S., et al., 2021. Effect of fiber dispersion, content and aspect ratio on tensile strength of PP fiber reinforced soil. Journal of Materials Research and Technology, 15, 1613–1621.
- Hejazi, S.M., et al., 2012. A simple review of soil reinforcement by using natural and synthetic fibers. Web of Science. doi:10.1016/j.conbuildmat.2011.11.045.
- Hojjati, F. and Sarkar, A., 2022. Mechanical properties of soil reinforced with polypropylene fibre. Proceedings of the Institution of Civil Engineers–Construction Materials, 175 (2), 72–81. doi:10.1680/jcoma.18.00057.
- Hu, W., et al., 2010. An improved method for single fiber tensile test of natural fibers. Polymer Engineering and Science, 50 (4), 819–825.
- Khoury, N. and Maalouf, M., 2018. Prediction of resilient modulus from post-compaction moisture content and physical properties using support vector regression. Geotechnical and Geological Engineering, 36 (5), 2881–2892.
- Kim, D. and Kim, J.R., 2007. Resilient behavior of compacted subgrade soils under the repeated triaxial test. Construction and Building Materials, 21 (7), 1470–1479.
- Lee, W., et al., 1997. Resilient modulus of cohesive soils. Journal of Geotechnical and Geoenvironmental Engineering–ASCE, 123 (2), 131–136.
- Li, J. and Ding, D.W., 2002. Nonlinear elastic behavior of fiber-reinforced soil under cyclic loading. Soil Dynamics and Earthquake Engineering, 22 (9–12), 977–983. doi:10.1016/S0267-7261(02)00122-7.
- Li, D. and Selig, E.T., 1994. Resilient modulus for fine-grained subgrade soils. Journal of Geotechnical Engineering, 120 (6), 939–957.
- Mamani, F.P. and Lazarte, F.A., 2019. Synthetic fiber in roads at the level of affirmed and its effect on its mechanical properties, Puno Region. Veritas Et Scientia–UPT, 8 (1), 1091–1098 (in Spanish). doi:10.47796/ves.v8i1.113.
- Mohanty, B., Chauhan, M.S., and Mittal, S., 2011. Permanent strain of randomly oriented fiber reinforced rural road subgrade soil under repetitive triaxial loading. Geo-Frontiers 2011: Advances in Geotechnical Engineering, 646–656.
- Moossazadeh, J. and Witczak, M.W., 1981. Prediction of subgrade moduli for soil that exhibits nonlinear behavior. Transportation Research Record (810), 9–17.
- Mori, S., et al., 2018. Assessment of Ichu fibers extraction and their use as reinforcement in composite materials. Journal of Natural Fibers, 17 (5), 700–715.
- Nezhad, M.G., Tabarsa, A., and Latifi, N., 2021. Effect of natural and synthetic fibers reinforcement on California bearing ratio and tensile strength of clay. Journal of Rock Mechanics and Geotechnical Engineering, 13 (3), 626–642.
- Plé, O. and Lê, T.N.H., 2012. Effect of polypropylene fiber-reinforcement on the mechanical behavior of silty clay. Geotextiles and Geomembranes, 32, 111–116. doi:10.1016/j.geotexmem.2011.11.004.
- Prabakar, J. and Sridhar, R.S., 2002. Effect of random inclusion of sisal fibre on strength behaviour of soil. Construction and Building Materials, 16 (2), 123–131.
- Puppala, A.J., 2008. Estimating stiffness of subgrade and unbound materials for pavement design. Transportation Research Board. NCHRP Synthesis, 382.
- Puppala, J. and Musenda, C., 2000. Effects of fiber reinforcement on strength and volume change behavior of expansive soils, Transportation Research Board. In: 79th annual meeting. Washington, USA.
- Pusari, O. and Rodriguez, J., 2020. Experimental study of the improvement of the shear resistance properties of an expansive soil with recycled glass powder and polypropylene fibers in the city of Talara, department of Piura. Thesis. Peruvian University of Applied Sciences (in Spanish).
- Seed, H.B., et al., 1967. Prediction of flexible pavement deflections from laboratory repeated-load tests. NCHRP Report, 35.
- Tang, C., et al., 2007. Strength and mechanical behavior of short polypropylene fiber reinforced and cement stabilized clayey soil. Geotextiles and Geomembranes, 25 (3), 194–202. doi:10.1016/j.geotexmem.2006.11.002.
- Tang, C., Shi, B., and Zhao, L., 2010. Interfacial shear strength of fiber reinforced soil. Geotextiles and Geomembranes, 28 (1), 54–62. doi:10.1016/j.geotexmem.2009.10.001.
- Van der Merwe, D.M., 1964. The prediction of heave from the plasticity index and percentage clay fraction of soils. Civil Engineer in South Africa, 6 (6), 103–107.
- Witczak, M.W. and Uzan, J., 1988. The universal airport design system, report I of IV: granular material characterization. College Park: Department of Civil Engineering, University of Maryland.
- Yao, Y., et al., 2018. Model for predicting resilient modulus of unsaturated subgrade soils in south China. KSCE Journal of Civil Engineering, 22 (6), 2089–2098.
- Yuan, H., et al., 2019. Resilient modulus—physical parameters relationship of improved red clay by dynamic tri-axial test. Applied Sciences, 9 (6), 1155. doi:10.3390/app9061155.
- Zhang, J., et al., 2019. Prediction of resilient modulus of compacted cohesive soils in south China. International Journal of Geomechanics, 19 (7), 04019068.