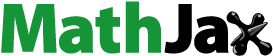
ABSTRACT
In the present research, the survival and sustainability of a Lactobacillus rhamnosus probiotic has been investigated with regard to the prebiotic impact of introducing two different kinds of onion (Allium cepa L.) peel extract to probiotic microcapsules. Effective inclusion of red and white onion peel extract featuring good prebiotic action into the microcapsules enhanced probiotic survival. The structure, distribution of size, zeta potential, and encapsulation efficacy of probiotics and substances in the extract were evaluated along with the probiotics capability to persist under simulated gastrointestinal circumstances. Fourier Transform Infrared Spectroscopy (FTIR) was employed to investigate the molecular structure and internal framework. The wall and core components possess adhesive relationships, as demonstrated by FTIR spectra. Probiotics that were free and those that were in capsules were evaluated as well in vitro in undesirable persistence performance (>90%). Probiotics with encapsulation exhibited substantially (p > .05) greater vitality compared free cells, in accordance with an in-vitro experiment. Under simulated gastrointestinal situations, free cells continued diminishing their vitality, but encapsulated cells maintained their viability count beyond the suggested level (107 cfu/g). SEM photographs indicated that probiotics have been effectively encapsulated inblends. The microcapsules were around 191 ± 2 and 176 ± 2 μm in size. These outcomes reveal that these kinds of microcapsules may encourage the probiotic L. rhamnosus prolonged viability and consistency under multiple conditions.
Introduction
The food industry has been progressively intrigued by studies focused on developing commodities that implement these microorganisms into their preparations as a potential substitute to consuming probiotic cells. Through including probiotic strains in a secondary protective layer to sustain their suitability and performance characteristics because they come into contact with risks throughout their retention period, the co-encapsulation technique helps to protect those[Citation1]. Using this approach also allows for the estimation of potential advantages that chemical constituents might offer starter bacteria. The microencapsulation methodology is employed to merge two or even more bioactive components into a single material to produce co-microcapsules.[Citation2] Probiotic granule implications of biologically active components were always underexplored. The influence of Thai plant supplements on the persistence of probiotic alginate beads were examined by.[Citation3] The researchers were successful in boosting the stability of probiotics employing cashew blossom and tea extract from green tea through combining components having massive concentrations of bioactive constituents and significant antioxidant potential. Similar to the preceding,[Citation4] studied the use of spray drying to co-encapsulate phytochemical components from blackberry juice using Lactobacillus acidophilus, also concluded that when employing whey protein as a structural component, probiotics showed good viability beyond preservation. Furthermore, additional research is required to investigate the impact of various pharmacological compounds on the probiotics capacity for development in body. The study claimed that[Citation5] onions (Allium cepa L.) are really an excellent source of plant-based components having medicinal and pharmacological advantages, such as organo-sulfur substances, thiosulfinates, phenolic acids, and flavonoids, specifically quercetin and anthocyanin’s, the last of which is mainly abundant in red and white onions. Numerous investigations have demonstrated the antifungal, antioxidant, cytotoxic, and antibacterial characteristics of the essential oils and isolated compounds of various Allium species.[Citation6] On the opposite side, preceding chemical researches have demonstrated that a plethora of components, such as steroidal, organosulfur derivatives, triterpenoidal saponins, and thiosulfinates, possess potential adverse effects.[Citation7] These substances operate through inhibiting the growth of multiple cancers, such as prostate, esophageal, pancreatic, and bladder cancers.[Citation8] The study further indicated that a variety of analysis demonstrated that onions and related may have therapeutic potential against asthma, diabetes, as well as other autoimmune reactions along with exhibiting prebiotic activities.[Citation9] The concentration of quercetin in onion outer surface powder is multiple times stronger compared to the consumable portion, and it also contains a greater proportion of quercetin to aglycone.[Citation10] Numerous pharmacological activities of the botanical flavonoid quercetin have been documented, comprising anti-atherosclerotic, cardiovascular preventative medicine, anti-inflammatory, anti-tumor, antihypertensive, and antioxidant properties.[Citation11,Citation12] Research has claimed that quercetin plays a role in maintaining the gastrointestinal microflora ecological homeostasis.[Citation13] Furthermore, it is recognized that onion peels possess greater quantities of these phytochemicals than other sections of this as well as other fruit and vegetables products.[Citation14] Moreover, despite of having substantial antioxidant capacity that defends against cell damage brought on by oxygen species that are reactive (developed by metabolic reactions and other external environmental pollutants) competent of oxidizing epithelial biomaterials (carbohydrates, proteins, lipids, enzymes, and nucleic acids), the husks are generally overlooked when handling or consuming the vegetable.[Citation15] Hence, it is necessary to contribute value to this leftover, which is particularly rich in bioactive components, minimize discarding of it by employing formulations that facilitate its implantation into food samples, and to eliminate its unpleasant sensory changes caused by its distinguish smell and taste. With the previous facts in mind, main purpose of this research was to produce microcapsules as well as evaluate the impact of co-encapsulating sodium alginate (SA) combined red onion peels and white onion skin extract on the durability and survivability of the probiotic L. rhamnosus. Among the most intriguing nutritional components used within foodstuffs is prebiotic.[Citation16] Probiotic bacteria play a part in health advantages through (1) promoting the growth of beneficial bacteria (prebiotics) in the human gut, (2) functioning as a dispersed fiber in the diet with a fat-lowering benefit, and (3) being extractable polysaccharides, including carcinomas and adenomas in the gut, and also lower the risk factors cause in colorectal disorders.[Citation17] Prebiotics can also be synthesized biochemically and are also present spontaneously in a number of products. Inulin and FOS are the most widely used prebiotics (also sometimes termed oligofructose or oligofructans). Vegetables including artichokes, asparagus, onions, garlic, and leeks have substantial amounts of inulin. Numerous plants, especially Jerusalem, onions, chicory, garlic, roots, asparagus, and artichokes, along with certain grains, particularly wheat and barley, also have high levels of FOS.[Citation18] The large number of the remaining naturally present probiotic strains and oligosaccharides, according to[Citation19] discovered in vegetables; nevertheless, several oligosaccharides, such as malt oligosaccharides, are additionally identified in decayed starch form cereals and some may be observed in plenty of other fruits and vegetables, like in crops with roots and tubers, which have previously been examined for their prebiotic action. In order to determine the concentration of prebiotics in onion peel extract and afterward to analyze the prebiotic efficacy, the research was carried out. The proliferation of a specified probiotic strain in particular substrate or in OPE isolate was employed as the foundation to assess the prebiotic effect.[Citation20] These observations might be beneficial for determining different food products that can function as prebiotic suppliers. Furthermore, this investigation may identify a more affordable technique for creating prebiotic oligosaccharides that are immediately derived from food sources, including two distinct kinds of onion peels.
Materials and methods
Materials
For this research, calcium chloride, sodium hydroxide, sodium chloride, sodium alginate, peptone and ethanol were used for this experiment. All these chemicals were purchased from Sigma-Aldrich (USA) and Merck (Germany). Probiotic culture L. rhamnosus in freeze dried form was purchased from the National Institute of Food Science and Technology (NIFSAT) and the experiment was conducted at Food Safety and Biotechnology Laboratory in Government College University Faisalabad (GCUF, Pakistan).
Inoculum preparation
The freeze dried form of probiotic culture L. rhamnosus was activated anaerobically in 100 ml of Man, Rogosa, and Sharpe (MRS) for 20 h at 37°C. It was then centrifuged (Thermo Fisher Scientific 75,005,286 EA) at 6000 g for 10 minutes at 4°C. The obtained cells were rinsed out with saline solution (NaCl, 0.85%).
Onion peel (red & white) extract collection
Following a slightly altered version of the method[Citation21] the extracts were produced. The samples were sanitized by using 200mgL−1 sodium hypochlorite for 20 minutes after being heated in an oven for 36 hours at 50°C. The samples were then grinded in a kind of scalpel miller. To produce the extracts, white onion peel samples (6.50 g) were incorporated into 135 mL of 80% ethanol and red onion peel samples (9.20 g) had been mixed to 190 mL of 80% ethanol. The blends were then spun in an orbital shaker at 80 rpm for 4 hours at 60°C. Subsequently the resulting concentrates after that rotary evaporated, and their volume was changed by incorporating water that was distilled and then eventually used for further for applications.
Prebiotic potential
Red and white onion peel dispersion was produced in a saltwater buffer with a pH 7.2 and 0.1 M PBS to examine the prebiotic activity. Employing bacterial culture, red and white onion peel was applied to basal media to replenish the media throughout fermentation. For the investigations F1: BCOPE (1%: 0%) and F2: BCOPE (1%: 1% ROPE, 1% WOPE), following combinations were applied. Onion peel found nonexistence in the control condition F1. After 0, 6, 12, and 24 hours of fermentation in an anaerobic environment (37°C), specimens were collected.[Citation22]
Prebiotic activity score
Prebiotic potential, conferring to[Citation23] showed a substrates capability to contribute to the growth of probiotics. Hence, if substrates are absorbed by probiotic strains together with glucose but not by other gut microbes. They have a significant prebiotic activity score. The following expression was applied to estimate the prebiotic activity score:
Prebiotic activity rating is determined as follows ={(probiotic log cfu/mL on the prebiotic at 24 h – probiotic log cfu/mL on the prebiotic at 0 h)/(prebiotic log cfu/mL on glucose at 24 h – probiotic log cfu/mL on the glucose at 0 h)} - {(enteric log cfu/mL on the prebiotic at 24 h – enteric log cfu/mL on the prebiotic at 0 h)/“(‘enteric log cfu/mL on glucose 24 h – enteric log cfu/mL on the glucose at 0 h’)”}
Microcapsules production by extrusion method
Microencapsulation was done by external ionic gelatin as previously accomplished by.[Citation24] Probiotics were encapsulated by extrusion method as F1 (2% Sodium Alginate Particles + L. rhamnosus) and F2 (2% Sodium Alginate Particles + 1% Red onion Extract + 1% White onion Extract + L. rhamnosus). Briefly a blend of sodium alginate and L. rhamnosus (2 g/100 ml) in formulation 1 and in formulation 2 the onion peels extract, sodium alginate and probiotic culture (4 g/100 mL) was mixed and taken in an encapsulator and then poured drop wise in 0.1 M CaCl2 for hardening of capsules. After swirling the micro particles CaCl2 solution and then in the CaCl2 solution for 30 minutes, the CaCl2 was extracted utilizing whatman filter paper and washed with distilled water.
Morphological characterization of microcapsules
Size
A 5× optical microscope (LABOMED, LX400) used to determine the dimensions of various micro particles.[Citation25] Immediately after the preparation method and under simulated gastric circumstances, microcapsules were investigated.
Zeta potential
According to[Citation26] applied to evaluate the zeta potential of synthesized capsules, despite some minimal changes. For the dissemination of the capsules, clean water was applied. The capsules were deposited into a test sample of a zeta potentiometer after distribution (Zetasizer Nano ZSP, Malvern analytical and Malvern). The standard solution was permitted five minutes to gradually set. The prepared sample was then fixed on a zeta potentiometer for potential observations.
Encapsulation efficiency
The performance of microcapsules comprising probiotics was assessed according to the procedure given by[Citation27] with little alterations. The following calculation was applied to evaluate encapsulation performance:
The amount of cells in microcapsules (NE) and number of cells not confined (Nn). The outcomes were presented as CFU/capsule (the total amount of colonies formation).
Scanning Electron Microscopy (SEM)
The scanning electron microscopy of the microcapsules was carried out by employing a scanning electron microscopic (Emcraft cube series) offered at the department of physics Government College University Faisalabad. The pictures of the specimen were taken out on a Feld manufactured under a low vacuum with an operating distance of 12.33 mm.
Fourier Transform Infrared Spectroscopy (FTIR)
Probiotic-loaded microcapsules undergo FTIR (Fourier transform infrared spectroscopy) applying an approach established by.[Citation28] To examine the existence of multiple functional categories and their effect on the capsules, FTIR approach was applied with a spectroscope (spectrum Two-Perkin Elmer) accessible at the National Textile Research Centre NTRC spectroscopy lab National Textile University Faisalabad. For FTIR a resolution of 2–3 cm − 1 was used with 30 scans from 550 to 3000 cm−1.
Simulated study
Viability of encapsulated L. rhamnosus in simulated gastric conditions
In the study accompanied by[Citation29] the probiotics resilience in simulated intestinal situations was examined. Investigation was carried out using sterilized Falcon tubes (15 ml). Employing HCL (5 M) to stimulate stomach circumstances, L. acidophilus cells were placed in suspension in 10 ml of NaCl (0.85%) solution in addition to pepsin (3 g/L) from Sigma-Aldrich. The pH of the solution was subsequently raised to 2.0. At intervals of 0, 3, 60, 90, 120, and 150 minutes, 1 ml aliquots were eliminated during a period of incubation at 37°C with 70 rpm shaking.
Viability of encapsulated L. rhamnosus in simulated intestinal conditions
The efficacy assessment of probiotic under simulated gastrointestinal circumstances was conducted by.[Citation30] The life span of probiotic bacteria was regularly examined in medium that simulated different small intestine sections. L. rhamnosus cells were immersed with pancreatic at a concentration of approximately 2 g L−1 (Sigma) and bile salts at a concentration of 12 g L − 1 of (Sigma) after pH adjustments to 5.0 for 20 minutes (duodenum) and pH adjustments to 7.5 for 90 min (ileum). Incubation was performed out at 37°C, simulating human body temperature in an incubating shaker (Daihan Scientific WIS-20) at 150 rpm. Subsequently aliquots were removed at 110 minutes into the duodenum or 200 minutes into the ileum to figure out how long L. rhamnosus persisted free and microencapsulated.
Statistical analysis
Results were submitted to Tukey’s test evaluation for average assessments, implementing under consideration a 5% significant limit (p < .05). Results were presented as 5 mean ± standard deviation, and testing were conducted in triplicate.
Results and discussion
Prebiotic potential of onion peel (red & white)
By the prebiotic activity score, as shown in , the prebiotic ability of two variants of onion peel extract was assessed. L. rhamnosus grown on red onion peel extract (1.96) and cultured on white onion peel extract received the greatest prebiotic potential values (1.81). The cell simulated results were utilized to calculate the outputs from . The potential of white and red onion extract to improve the stability of probiotics has been evaluated. With duration, the bacterial activity among treatments demonstrated a considerable difference (8.01 ± 0.09 to 9.76 ± 0.06 CFU/mL). During 24 hours of alcoholic fermentation, the optimum survival of L. rhamnosus was reported to be (9.76 ± 0.06 CFU/mL) for (ROPE) red onion peel extract and (9.23 ± 0.05 CFU/mL) for (WOPE) white onion peel extract. Furthermore, at the initiation of treatment, ROPE and WOPE both confirmed the lowest survival (8.33 ± 0.04 CFU/mL) and (8.01 ± 0.09 CFU/mL), correspondingly. Members of the probiotic microflora in the gastrointestinal tract metabolized in a number of different ways. Similar findings were observed in the research of.[Citation23] Because of their exclusive utilization, carbohydrates have the possibility of influencing the probiotic flora in the intestinal mucosa. Subsequently, a prebiotic’s effectiveness is dependent on its ability to stimulate the establishment of particular targeted microbes and to be exclusively synthesized by those microorganisms. Onion peel extract has been employed to encourage probiotic proliferation. The percentage of viable cells substantially increased (p < .05) with higher onion peel extract concentration and incubation time. The observations are similar to those described by[Citation31] who indicated that whereas sprouted rice extract had a moderate prebiotic level (0.20) for L. acidophilus, garlic, shallot, and onion extracts showed significant prebiotic values (almost to 2.0). As prebiotics, onion peel extract enhanced the synthesis of carbohydrates, which raised the probiotics capacity for survival. The findings closely resemble those of[Citation32] who researched at the prebiotic impact of lentil flour’s water-soluble carbohydrates.
Figure 1. Prebiotic activity score of c L. rhamnosus grown on red onion peel and white onion peel extract.

Table 1. Cell density for bacterial cultures grown on onion peels extract from time 0 to time 24 h, expressed as log CFU/ml.
Morphological characterization of microcapsules
SEM (Scanning Electron Microscopy)
The technique of scanning electron microscopy was employed to evaluate the probiotic microcapsules composition (). The probiotics were encapsulated successfully, as demonstrated by SEM, and their dissemination throughout the micro particles framework was identifiable. The spherical probiotic microcapsules have a smooth texture. The structures of the microcapsules are regulated by the sodium alginate[Citation33] specify, stronger concentrations provide a smooth texture. Probiotic microcapsules prepared with 3% sodium alginate in their experiment had a smooth texture and comparatively little porosity, but probiotic microcapsules prepared with 1% sodium alginate had a rough surface with numerous pores. In our research, effective probiotic microcapsules prepared with onion peels extract have a rough texture, although probiotic microcapsules prepared with 2% sodium alginate possess an irregular (rough and smooth) appearance. Once onion peel extract is introduced to a sodium alginate composite to encapsulate L. rhamnosus that has a diversified or exceptionally rough surface, we realized that the open pores considerably grow bigger. The encapsulated microcapsules comprising L. rhamnosus prepared with SA matrix exhibit a slightly rough texture with comparatively small porous structure. According to investigations, the microbial presence and other chemicals can conflict with alginates cross-linking with calcium chloride, producing the resulting microcapsules highly permeable.[Citation34] In our instance, SEM concluded that adding onion peel extract lowered the permeability and spherical structure of micro particles because OPE comprises filaments as well as other substances. The onion peel extract – containing microcapsules were distorted and desiccated. Improved spherical patterns may be seen in the SA particles. Each composition of the micro particles had flaws. Nevertheless, according to[Citation35] micro particles specifically created of malt dextrin had a few perforations when compared to those created containing prebiotics (inulin and dextran), which contribute to enhanced probiotic safety.
Size
A micro particle must be included in this group and have sizes that range between 0.2 to 5000 μm.[Citation36] The composition, percentage of wall material, nozzle diameter and distance between the emulsifying substance and nozzle of extruder all have a serious influence on the size of particles that can be attained when applying the exterior ionic gelatin process for extrusion.[Citation37] Nonetheless, this process is recognized for synthesizing micro particles with spherical structures and precise dimensions. Reduced particle sizes enhance the products consistency and dissemination of the active component while eliminating any negative consequences on the texture of the product.[Citation38] The dimensions and form of the capsules are acknowledged to be affected by the content of the encapsulated substance.[Citation39] By employing simply alginate, its high amount expands more Ca2+ attachment points, strengthening the alginate fiber structure and providing a more uniform and strong framework Using the extrusion method, produced microcapsules of sodium alginate that coated in chitosan that expressed the probiotics such as Lactobacillus casei and Lactobacillus plantarum alone or in combination with herbal tea that is enriched with selenium. According to the observations, the beads prepared with herbal tea that contained selenium were thicker than those beads that only contained probiotics.[Citation40]
The average size of microcapsules varied between 191 ± 1.81 and 176 ± 1.56 μm for SA and OPE/SA respectively. These results are similar to those found by[Citation30] who used the external ionic gelation method to produce probiotic beads with sizes that ranged from 107 μm to 222 μm. Similar to,[Citation41] who used freeze drying method and external ionic gelation technique to produce micro particles with sizes that varied from 127.5 μm to 234.6 μm. The mean diameter of particles was also affected by the addition of the onion peels extract, since those particles were substantially smaller (p < .05) than the ones made only with alginate. By increasing the transparency, colloidal stability, and surface-to-volume ratio of the solution, a decrease in the particle size, which in turn increases the solubility and bioavailability of the bioactive molecule. The structures of the bioactive compounds and how they are organized in microcapsules often have a great impact on particle size.[Citation42] Because of the formation of weaker wall materials, the survival percentage of the bacteria encapsulated in the capsule normally decreases as the size of the capsule increases.[Citation43] It is crucial to relate the size of the particles to the product to be utilized because[Citation44] claimed that some properties, such particle size, may impact its viability and acceptability in food.
Encapsulation efficiency
The results of the investigation of efficiency of the probiotics encapsulation in microcapsules containing or not containing the extract are shown in . To establish that the active substance achieves the action site in massive amounts, the encapsulating technology must have a substantial retention rate. Although there was considerable probiotic maintenance in the capsule (>90%) in all preparations, including onion peels extract to the microcapsules had no discernible effects on the probiotics’ ability to survive the extrusion process. The encapsulation efficiency reported in this experiment may have been significantly affected by the extrusion/external ionic gelatin technique, which facilitated particle production without the requirement for organic solvents or elevated temperature. This evidence supports those of[Citation45,Citation46] both used extrusion/external ionic gelatin methods to encapsulate probiotic cells and attained encapsulation percentages of 96.75 and 99.9%, accordingly. Compared to primary information published here, several scientists did not achieve as desirable EE% values when mixing probiotics and pharmacological extracts using microencapsulation. Probiotic strains and green tea isolates were co-encapsulated by[Citation47] employing alginate to obtain an EE% of the probiotic comparable to 37.5%. The connection between both the probiotic cells and flavonoid is what prompted[Citation48] to attain minimal encapsulation efficiency such as 19.5% EE of Lactobacillus gasseri and 22.2% EE of bifidobacterium bifidum after the co-encapsulation of two types of probiotics with the prebiotic i.e. quercetin. Evaluation of the effects is essential considering these research findings that any type of extract or active ingredient that will be introduced to probiotic-containing microscopic particles because each one has special features, as well as phenolic compounds, anthocyanin, flavonoids, and antioxidant properties, all of which can have a beneficial or detrimental effect on probiotics.
Table 2. Characteristics of microparticles containing L. rhamnosus i.e. Size, encapsulation efficiency and zeta potential.
Table 3. Viability (log cfu/g) of free and encapsulated probiotic bacteria under simulated gastric and intestinal fluid.
The average findings indicate that characterization of the encapsulation capability of microcapsules using two distinct onion peel extracts is valid in . The analysis indicate that microcapsules encapsulated using onion peel extract and sodium alginate (OPE/SA, 97%) showed considerably (p ≤ .05) better encapsulation efficiency than the microcapsules prepared with sodium alginate (SA, 91%), The treatment containing high degree of efficiency property, gelling ability, and ability to create a highly configurable matrix and a better productivity was observed in OPE/SA because of the enhanced bioavailability and solubility of the bioactive components in onion peels extract. The performance of encapsulation can be affected by an extensive variety of parameters, like CaCl2 content, the kind of wall material utilized, the species of microbes that are encased, particle size, and methodology. Isolates of lactobacillus were applied by[Citation49] who enclosed the combination employing soybean meal concentrate. Their analysis demonstrates that soy protein extract promotes probiotic survivability in extremes environments and improves encapsulation output.
Zeta potential
Through using (ζ-potential) zeta potential free and encapsulated microcapsules surface electrochemical parameters were investigated. Colloids with low ζ-potential have an inclination to agglomerate or coagulate, whereas microcapsules having strong ζ-potential (positive or negative) are electronically sustained.[Citation50] The overall findings indicate that the zeta potential of sodium alginate, encapsulated micro particles comprising two distinct kinds of onion peels extract is shown in . The authors found that the zeta potential of microcapsules is substantially dependent on the sort of encapsulating components. Probiotic (L. rhamnosus) supplements with sodium alginate had a −14 mV zeta potential, but those with onion peel extract/sodium alginate had a −31 mV zeta potential. The proportion of various encapsulated elements, medium’s pH, and the ionic strength all related to the zeta potential discrepancy. Microcapsules had a positive as well as a negative zeta potential. It is a vital component of establishing electrochemical parameters.[Citation51] It is a documented fact that the solution will be persistent and less sensitive to aggregation if the zeta potential value of the samples is above +30 mV or below −30 mV, this is due to the presence of stronger repellent relations (both steric as well as electrostatic).[Citation52] The two specimens zeta potential readings were less than −40 mV, as shown in , illustrating the system’s good stability. The anionic phospholipids, like phosphatidylcholine, are accountable for the microcapsules opposite electrode in this research.
Molecular characterization of microcapsules
Fourier transforms infrared spectrometry (FTIR) of onion peels extract (red & white) and probiotics loaded microcapsules (onion peels extract/sodium alginate)
The chemical composition of the microcapsules synthesized from a combination of white and red onion peel extract, sodium alginate, and bacteria was analyzed utilizing FTIR methodology. The FTIR observations for probiotic-loaded sodium alginate microcapsules and co-encapsulated probiotic-loaded microcapsules with onion peels extract/sodium alginate was shown schematically in ) respectively.
Figure 3. The Fourier Transform Infrared Spectroscopy (FTIR) spectra of microcapsules with sodium alginate and bacterial culture (a) and onion peels extract with sodium alginate and probiotic (b).

The specific functional groups were highlighted by different bonds in the infrared spectra. Curves found evident in the FTIR spectra of SA microcapsules with L. rhamnosus at 2366 cm−1, 2335 cm−1, 1623 cm−1, 963 cm−1, 647 cm−1, 630 cm−1 and 608 cm−1. Two peaks, one at 2335 cm−1 and the other at 2366 cm−1, were identified, both of which pointed to the existence of carbon dioxide through O=C=O extending in the SA chemical composition.[Citation53] According to this, a strong peak for the amide (C=O) bending vibration was observed at 1623 cm−1. Other similar lesser sharp, strong peak appeared at 963 cm−1 indicating disubstituted (Trans) alkene (C=C) bending vibrations. The prevalence of C-Br deformation, which is characteristics of halo chemicals under the influence of IR, is associated with further higher, sharper peaks like 647 cm−1, 630 cm−1 and 608 cm−1.[Citation54,Citation55]
Once L. rhamnosus was subjected to OPE/SA combination microcapsules, there were moderate spectral adjustments observed in the spectral range. For instance, the SA microcapsules concentrations at 2366 cm−1 and 2335 cm−1 displaced to 2850 cm−1 as well as indicated a stronger absorbance peak than the SA separately. This suggests that the C-H extending in OPE improved the alkyl functional groups maximum absorption in the blended micro particles. Amplitude of the wave for the stretching mode of the amide (C=O) appeared at 1642 cm−1 in compliance with alkane, as contrary to 1623 cm−1 in the SA. The peak intensity remained unchanged substantially between the two profiles, although. Furthermore, the existence of OPE in the SA at 929 cm−1 enabled the C=C folding resonance peaks extremely prominent. Also with OPE/SA blended microcapsules comprising L. rhamnosus, the levels of peaks corresponding to C-Br extending that relate to halo components improved. Past research also indicate that sodium alginate and onion peel extract-based encapsulation spectra had experienced minor modifications.[Citation56,Citation57]
Stability of free and encapsulated L. rhamnosus under simulated gastrointestinal conditions
Probiotic capacity for existence can be seriously affected by the potential of hydrogen (pH), specifically at low levels of pH.[Citation58] A variety of these microbes must pass through the intestine and make it via the duodenum and the stomach in addition to have a positive impact on their host. The probiotic L. rhamnosus moreover, also sensitive to acidic conditions, which decreases its viability in gastrointestinal tract conditions situations, as reported by a number of research investigations.[Citation59] Probiotics are thus exposed to circumstances that imitated the esophagus/stomach, duodenal, and ileum in pure and microencapsulated administrations including (SA and OPE/SA) extracts and without (SA) in .
The free probiotic cells had a value greater than 9.40 ± 0.05 log CFU g−1 at the beginning of the analysis in synthetic gastric secretion (pH 2.0), but the probiotic encapsulated in micro particles had a level equivalent to 10.76 ± 0.12 log CFU g−1 across the OPE/SA sessions. The free probiotic observed decreases of 6.87 ± 0.03, and 2.18 ± 0.02 log CFU g−1 at 30 and 60 minutes of exposure with synthetic gastric juice (pH 2.0), correspondingly. The probiotic incorporated into the microparticles had a concentration of 9.88 ± 0.09 at 30 minutes and 8.61 ± 0.03 log CFU g−1 at 60 minutes, although, encapsulated with sodium alginate and onion peels extract + sodium alginate offered considerable resistance in the synthetic gastric secretion in . While the microcapsules in which the encapsulated probiotics had a count array from 7.39 ± 0.01 log CFU g−1, 7.02 ± 0.06 log CFU g−1 and 6.45 ± 0.17 log CFU g−1 at 90 min, 120 min, and 150 min respectively, the available probiotic had not been identified in simulated gastric secretion (pH 2.0) after 90 minutes of the evaluation, indicating that protective material is essential.
The free probiotic exhibited an even considerable decrease of functionality in the simulated duodenum (pH 5.0/20 min) compared to the stomach/esophagus. Moreover, the free probiotic in this sample sequence achieved durability below the range required for encouraging positive impacts (around 7 log CFU g−1). In the condition of 0.3% bile salts, disruption to bacterial cell wall may actually happen, causing cell fragmentation and apoptosis.[Citation60] Yet, due to the absence of complete cell liberation within the particles, the probiotics still effectively maintained by the encapsulation process and remained unchanged.
The free probiotic had a score of 9.44 ± 0.06 log CFU g−1 at the beginning of the experiment under simulated intestinal environment (20 min at pH 5.0), but the probiotics encapsulate in micro particles in the OPE/SA condition had a score of 10.83 ± 0.02 log CFU g−1. The active probiotic experienced a decline of 6.87 ± 0.04, 2.18 ± 0.08 and 1.08 ± 0.03 log CFU g−1 at 30, 60, and 90 min of exposure in the existence of 0.3% bile salts (pH 5.0), respectively. The probiotic enclosed in microcapsules had a concentration of 9.91 ± 0.04 log CFU g−1 at 30 min, 8.77 ± 0.09 log CFU g−1 at 60 min and 7.65 ± 0.01 log CFU g−1 at 90 min, while, OPE/SA supplementation exhibited considerable protection in simulated gastrointestinal situations in . The probiotic that is confined in microcapsules had a count array from 7.47 ± 0.07 log CFU g−1 and 6.89 ± 0.05 log CFU g−1 at 120 min and 150 min, respectively, suggesting that protective material is essential because the available probiotic failed to be identified after 120 minutes of evaluation in simulated duodenum circumstances (pH 5.0).
Although sodium alginate is only soluble in the pH of 6.0 to 7.0 (ileum), sodium alginate microcapsules are produced precisely that the discharge of the active constituent primarily proceeds at this pH level. In this research, sodium alginate (SA) was capable of preserving probiotic persistence in spite of bile salts and a low pH level. Furthermore, extract of onion peels promoted probiotic viability under similar parameters, probably owing to its prebiotic impact. Prebiotic compounds function as precursors for these microorganisms, which has synergistic action and can enhance the lifespan of probiotic bacteria during their transit through the gastrointestinal system.[Citation61]
The microcapsules of the various treatments (SA and OPE/SA) fragmented after 120 min of assessment (pH 7.5), promote cell permeability at the specified point of action. While evaluating the probiotic L. rhamnosus reduction of survivability in encapsulated and in free states, the last one acquired at its place of action in adequate concentrations (around 7 log CFU g−1) in all micro particle combinations. Meanwhile, the other abandoned their survival in the gastrointestinal tract and was not able to access the ileum in sufficient numbers to have a positive impact, indicating that this bacterium needed a shield from any outside impacts. Different microencapsulation procedures, such as spray-drying[Citation62] emulsification[Citation63] and freeze-drying[Citation64] similarly demonstrated effectiveness in maintaining LC survivability in relation to single cells under simulated gastrointestinal situations.[Citation65] Reported that microencapsulation also had preventive properties against LC ATCC 334 and lactobacillus rhamnosus ASCC 290 lead to ice cream while experiencing simulated gastrointestinal situations.
The probiotic viability significantly enhanced when the sodium alginate and onion peel extract were complemented against micro particles containing free cells and probiotics that were encapsulating shown in . At the completions of experiment, nevertheless, OPE/SA probiotic viability remained decreased dramatically. Even though, each of the combinations achieved it to the ileum in the quantity needed. Multiple scientists have also emphasized how micro particles potentially conceal the active metabolite in the stomach and stimulate its discharge in the intestinal lumen.[Citation66,Citation67] Furthermore, phenolic components have been demonstrated to manage gut micro flora, enhance probiotic microorganisms, but also respond as prebiotics[Citation10] all of which may have made a significant contribution to enhanced probiotic viability in the simulated gastrointestinal tract circumstances investigated here.[Citation68]
Conclusion
In order to ensure the sustainability and long-term viability of probiotics in beneficial foods, the food industry must utilize microencapsulation as an appropriate substitute for the safe and effective administration of probiotics. This technology assists in guaranteeing that carrier products maintain the appropriate effective amount of probiotics (106-108 CFU/g). Non-digestible materials referred to as prebiotics contribute in the formation of probiotics. Employing encapsulation and wall material matrices, probiotic cells that have been encapsulated may exhibit a greater viability potential. The combination of sodium alginate and red and white onion peels extract were incorporated in two compositions to extrude lactobacillus rhamnosus into capsules. Red and white onion peel extract optimized the overall strength of microcapsules and elevated probiotic survival in both the food matrix and simulated gastrointestinal disorders. These extracts might possess prebiotic action that encourages development of lactobacillus probiotics. When compared to free cells, the co-encapsulation operated well under acidic and bile salt circumstances. White onion peel extract did not function either in supporting the probiotics viability as red onion peel extract did. The findings opened up the possibility for the application of onion peel extract as a wall material for probiotic encapsulation.
Informed consent
Informed consent was obtained from all individual participants included in the study.
Acknowledgments
The authors would like to thank the Researchers Supporting Project Number (RSP2023R35), King Saud University, Riyadh, Saudi Arabia.
Disclosure statement
No potential conflict of interest was reported by the author(s).
Data availability statement
Even though adequate data has been given in the form of tables and figures, all authors declare that if more data is required, the data will be provided on a request basis.
Additional information
Funding
References
- Raddatz, G. C.; Fonseca, V. R.; Cichoski, A. J.; Zepka, L. Q.; Jacob-Lopes, E.; Campagnol, P. C. B.; de Menezes, C. R.; Muller, E. I.; de Moraes Flores, E. M.; de Bona da Silva, C., et al. Viability and Stability Evaluation of Lactobacillus Casei LC03 Co-Encapsulated with Red Onion (Allium Cepa L.) Peel Extract. LWT. 2022, 153, 112434. DOI: 10.1016/j.lwt.2021.112434.
- Chen, Q.; McGillivray, D.; Wen, J.; Zhong, F.; Quek, S. Y. Co-Encapsulation of Fish Oil with Phytosterol Esters and Limonene by Milk Proteins. J. Food Eng. 2013, 117(4), 505–512. DOI: 10.1016/j.jfoodeng.2013.01.011.
- Chaikham, P. Stability of Probiotics Encapsulated with Thai Herbal Extracts in Fruit Juices and Yoghurt During Refrigerated Storage. Food. Biosci. 2015, 12, 61–66. DOI: 10.1016/j.fbio.2015.07.006.
- Colín-Cruz, M. A.; Pimentel-Gonz´ Alez, D. J.; Carrillo-Navas, H.; Alvarez-Ramírez, J.; Guadarrama-Lezama, A. Co-Encapsulation of Bioactive Compounds from Blackberry Juice and Probiotic Bacteria in Biopolymeric Matrices. LWT. 2019, 110, 94–101. DOI: 10.1016/j.lwt.2019.04.064.
- Zeng, Y.; Li, Y.; Yang, J.; Pu, X.; Du, J.; Yang, X.; Yang, T.; Yang, S. Therapeutic Role of Functional Components in Alliums for Preventive Chronic Disease in Human Being. Evid. Based Complement. Altern. Med. 2017, 2017, 1–13. DOI: 10.1155/2017/9402849.
- Rouis-Soussi, L. S.; El Ayeb-Zakhama, A. E.; Aouni Mahjoub, A.; Guido Flamini, G.; Hichem Ben Jannet, H. B.; Harzallah-Skhiri, F. Chemical Composition and Antibacterial Activity of Essential Oils from the Tunisian Allium Nigrum L. Excli. J. 2014, 13, 526–535.
- Matsuura, H. Saponins in Garlic as Modifiers of the Risk of Cardiovascular Disease. J. Nutr. 2001, 131(3), 1000S–1005S. DOI: 10.1093/jn/131.3.1000S.
- Jan, A. T.; Kamli, M. R.; Murtaza, I.; Singh, J. B.; Ali, A.; Haq, Q. M. R. Dietary Flavonoid Quercetin and Associated Health Benefits-An Overview. Food Rev. Int. 2010, 26(3), 302–317. DOI: 10.1080/87559129.2010.484285.
- Desjardins, Y. Horticultural Science Focus-Onion as a Nutraceutical and Functional Food. Chron. Hortic. 2008, 48, 8.
- Li, S.; Ma, C.; Gong, G.; Liu, Z.; Chang, C.; Xu, Z. The Impact of Onion Juice on Milk Fermentation by Lactobacillus acidophilus. LWT –Food Sci. Technol. 2016, 65, 543–548. DOI: 10.1016/j.lwt.2015.08.042.
- Tang, S. M.; Deng, X. T.; Zhou, J.; Li, Q.-P.; Ge, X.-X.; Miao, L. Pharmacological Basis and New Insights of Quercetin Action in Respect to Its Anti-Cancer Effects. BPJ. 2020, 121, 109604. DOI: 10.1016/j.biopha.2019.109604.
- Dabeek, W. M.; Marra, M. V. Dietary Quercetin and Kaempferol: Bioavailability and Potential Cardiovascular-Related Bioactivity in Humans. Nutrients. 2019, 11(10), 2288. DOI: 10.3390/nu11102288.
- Zhang, Z.; Peng, X.; Zhang, N.; Liu, L.; Wang, Y.; Ou, S. Cytotoxicity Comparison of Quercetin and Its Metabolites from in vitro Fermentation of Several Gut Bacteria. Food. Funct. 2014, 5(9), 2152. DOI: 10.1039/c4fo00418c.
- Moon, J.; Do, H. J.; Kim, O. Y.; Shin, M. J. Antiobesity Effects of Quercetin-Rich Onion Peel Extract on the Differentiation of 3T3-L1 Preadipocytes and the Adipogenesis in High Fat-Fed Rats. FCT. 2013, 58, 347–354. DOI: 10.1016/j.fct.2013.05.006.
- Prior, R. L.; Wu, X.; Schaich, K. Standardized Methods for the Determination of Antioxidant Capacity and Phenolics in Foods and Dietary Supplements. J. Agric. Food. Chem. 2005 May18, 53, 4290–4302. DOI: 10.1021/jf0502698.
- Moongngarm, A.; Trachoo, N.; Sirigungwan, N. Low Molecular Weight Carbohydrates, Prebiotic Content, and Prebiotic Activity of Selected Food Plants in Thailand. Adv. J. Food. Sci. Technol. 2011, 3(4), 269–274.
- Scholz-Ahrens, K. E.; Ade, P.; Marten, B.; Weber, P.; Timm, W.; AHil, Y.; Gluer, C. C.; Schrezenmeir, J. Prebiotics, Probiotics, and Synbiotics Affect Mineral Absorption, Bone Mineral Content, and Bone Structure. J. Nutr. 2007, 137(3), 838S–846S. DOI: 10.1093/jn/137.3.838S.
- Judprasong, K.; Tanjor, S.; Puwastien, P.; Sungpuag, P. Investigation of Thai Plants for Potential Sources of Inulin-Type Fructans. J. Food Comp. Anal. 2011, 24(4–5), 642–649. DOI: 10.1016/j.jfca.2010.12.001.
- Matsuhiro, B.; Torres, R.; Zuniga, E. A.; Aguirre, M. J.; Mendoza, L. E.; Issaacs, M. Determination of Low Molecular Weight Carbohydrates in Cabernet Sauvignon Red Wines. J. Chil. Chem. Soc. 2009, 54(4), 405–407. DOI: 10.4067/S0717-97072009000400018.
- Espinosa-Martos, I.; Rico, E.; Ruperez, P. N. Note. Low Molecular Weight Carbohydrates in Foods Usually Consumed in Spain. Food Sci. Technol. Int. 2006, 12(2), 171–175. DOI: 10.1177/1082013206063838.
- Viera, V. B.; Piovesan, N.; Rodrigues, J. B.; de O Mello, R.; Prestes, R. C.; Dos Santos, R. C.; de A Vaucher, R.; Hautrive, T. P.; Kubota, E. H. Extraction of Phenolic Compounds and Evaluation of the Antioxidant and Antimicrobial Capacity of Red Onion Skin (Allium Cepa L.). Int. Food Res. J. 2017, 24(3), 990–999.
- Hussein, H.; Awad, S.; EI-Sayed, I.; Ibrahim, A. Impact of Chickpea as Prebiotic, Antioxidant and Thickener Agent of Stirred Bio-Yoghurt. Ann. Agric. Sci. 2020, 65, 49–58. DOI: 10.1016/j.aoas.2020.03.001.
- Huebner, J.; Wehling, R. L.; Hutkins, R. W. Functional Activity of Commercial Prebiotics. Int. Dairy. J. 2007, 17(7), 770–775. DOI: 10.1016/j.idairyj.2006.10.006.
- de Araújo Etchepare, M.; Raddatz, G. C.; Cichoski, A. J.; Flores, É. M.; Barin, J. S.; Zepka, L. Q.; Jacob-Lopes, E.; Grosso, C. R.; de Menezes, C. R. Effect of Resistant Starch (Hi-Maize) on the Survival of Lactobacillus acidophilus Microencapsulated with Sodium Alginate. JFF. 2016, 21, 321–329. DOI: 10.1016/j.jff.2015.12.025.
- Krunić, T. Z.; Obradović, N. S.; Rakin, M. B. Application of Whey Protein and Whey Protein Hydrolysate as Protein Based Carrier for Probiotic Starter Culture. Food. Chem. 2019, 293, 74–82. DOI: 10.1016/j.foodchem.2019.04.062.
- Ji, Y. R.; Choi, S.; Lee, M. J.; Cho, Y.; Cho, Y. Microencapsulation of Probiotic Lactobacillus acidophilus kbl409 by Extrusion Technology to Enhance Survival Under Simulated Intestinal and Freezedrying Conditions. J. Microbiol and Biotechnol. 2019, 29(5), 721–730. DOI: 10.4014/jmb.1903.03018.
- Martin, M. J.; Lara-Villoslada, F.; Ruiz, M. A.; Morales, M. E. Effect of Unmodified Starch on Viability of Alginate-Encapsulated Lactobacillus Fermentum CECT5716. LWT- Food Sci. Technol. 2013, 53(2), 480–486. DOI: 10.1016/j.lwt.2013.03.019.
- Çanga, E. M.; Dudak, F. C. Improved Digestive Stability of Probiotics Encapsulated within Poly (Vinyl Alcohol)/Cellulose Acetate Hybrid Fbers. Carbohydr. Polym. 2021, 264, 117990. DOI: 10.1016/j.carbpol.2021.117990.
- Ribeiro, E. F.; Borreani, J.; Moraga, G.; Nicoletti, V. R.; Quiles, A.; Hernando, I. Digestibility and Bioaccessibility of Pickering Emulsions of Roasted Coffee Oil Stabilized by Chitosan and Chitosansodium Tripolyphosphate Nanoparticles. Food. Biophys. 2020, 15(2), 196–205. DOI: 10.1007/s11483-019-09614-x.
- Araújo Etchepare, M.; Nunes, G. L.; Nicoloso, B. R.; Barin, J. S.; Flores, E. M. M.; de Oliveira Mello, R.; de Menezes, C. R. Improvement of the Viability of Encapsulated Probiotics Using Whey Proteins. LWT. 2020, 117, 108601. DOI: 10.1016/j.lwt.2019.108601.
- Moongngarm, A.; Saetung, N. Comparison of Chemical Compositions and Bioactive Compounds of Germinated Rough Rice and Brown Rice. Food. Chem. 2010, 122(3), 782–788. DOI: 10.1016/j.foodchem.2010.03.053.
- Portman, D.; Blanchad, C.; Mahajan, P.; Naiker, M.; Panozzo, J. F. Water-Soluble Carbohydrates During Fermentation and Baking of Composite Wheat and Lentil Flour—Implications for Enhanced Functionality. Cereal. Chem. 2019, 96, 447–455. DOI: 10.1002/cche.10144.
- Chen, K. N.; Chen, M. J.; Liu, J. R.; Lin, C. W.; Chiu, H. Y. Optimization of Incorporated Prebiotics as Coating Materials for Probiotic Microencapsulation. J. Food Sci. 2005, 70(5), M260–M266. DOI: 10.1111/j.1365-2621.2005.tb09981.x.
- Sultana, K.; Godward, G.; Reynolds, N.; Arumugaswamy, R.; Peiris, P.; Kailasapathy, K. Encapsulation of Probiotic Bacteria with Alginate-Starch and Evaluation of Survival in Simulated Gastro-Intestinal Conditions and in Yoghurt. Int. J. Food Microbiol. 2000, 62, 47–55. DOI: 10.1016/S0168-1605(00)00380-9.
- Pandey, P.; Mishra, H. N. Co-Microencapsulation of γ-Aminobutyric Acid (GABA) and Probiotic Bacteria in Thermostable and Biocompatible Exopolysaccharides Matrix. LWT–Food Sci. Technol. 2021, 136, 110293. Article DOI: 10.1016/J.LWT.2020.110293.
- Fávaro-Trindade, C. S.; Pinho, S. C. D.; Rocha, G. A. Revisão: Microencapsulação de ingredientes alimentícios. Braz. J. Food Technol. 2008, 11(2), 103–112.
- Anal, A. K.; Bhopatkar, D.; Tokura, S.; Tamura, H.; Stevens, W. F. Chitosanalginate Multilayer Beads for Gastric Passage and Controlled Intestinal Release of Protein. Drug. Dev. Ind. Pharm. 2003, 29(6), 713–724. DOI: 10.1081/DDC-120021320.
- Shaharuddin, S.; Muhamad, I. I. Microencapsulation of Alginate-Immobilized Bagasse with Lactobacillus Rhamnosus NRRL 442: Enhancement of Survivability and Themotolerance. Carbohydr. Polym. 2015, 119, 173–181. DOI: 10.1016/j.carbpol.2014.11.045.
- Sandoval-Castilla, O.; Lobato-Calleros, C.; García-Galindo, H. S.; Alvarez-Ramírez, J.; Vernon-Carter, E. J. Textural Properties of Alginate–Pectin Beads and Survivability of Entrapped Lb. Casei in Simulated Gastrointestinal Conditions and in Yoghurt. Food Res. Inter. 2010, 43, 111–117. DOI: 10.1016/j.foodres.2009.09.010.
- Vodnar, D. C.; Socaciu, C. Green Tea Increases the Survival Yield of Bifidobacteria in Simulated Gastrointestinal Environment and During Refrigerated Conditions. Chem. Cent. J. 2012, 6(1). DOI: 10.1186/1752-153X-6-61.
- Poletto, G.; Fonseca, B. D.; Raddatz, G. C.; Wagner, R.; Lopes, E. J.; Barin, J. S.; Flores, E. M. D. M.; Menezes, C. R. D. Encapsulation of Lactobacillus acidophilus and Different Prebiotic Agents by External Ionic Gelation Followed by Freeze-Drying. Cienc. Rural. 2019a, 49(2). DOI: 10.1590/0103-8478cr20180729.
- Mitrea, E.; Ott, C.; Meghea, A. New Approaches on the Synthesis of Effective Nanostructured Lipid Carriers. Rev. Chim. 2014, 65(1), 50–55.
- Capela, P.; Hay, T. K. C.; Shah, N. P. Effect of Homogenization on Bead Size and Survival of Encapsulated Probiotic Bacteria. Food Res. Inter. 2007, 40, 1261–1269. DOI: 10.1016/j.foodres.2007.08.006.
- Kim, E. S.; Lee, J. S.; Lee, H. G. Calcium-Alginate Microparticles for Sustained Release of Catechin Prepared via an Emulsion Gelation Technique. Food Sci. Biotechnol. 2016, 25(5), 1337–1343. DOI: 10.1007/s10068-016-0210-8.
- Poletto, G.; Raddatz, G. C.; Cichoski, A. J.; Zepka, L. Q.; Lopes, E. J.; Barin, J. S.; Wagner, R.; de Menezes, C. R. Study of Viability and Storage Stability of Lactobacillus Acidophillus When Encapsulated with the Prebiotics Rice Bran, Inulin and Hi-Maize. Food. Hydrocoll. 2019b, 95(95), 238–244. DOI: 10.1016/j.foodhyd.2019.04.049.
- Vaziri, A. S.; Alemzadeh, I.; Vossoughi, M.; Khorasani, A. C. Comicroencapsulation of Lactobacillus Plantarum and DHA Fatty Acid in Alginate-Pectingelatin Biocomposites. Carbohydr. Polym. 2018, 199, 266–275. DOI: 10.1016/j.carbpol.2018.07.002.
- Vodnar, D. C.; Socaciu, C. Selenium Enriched Green Tea Increase Stability of Lactobacillus Casei and Lactobacillus Plantarum in Chitosan Coated Alginate Microcapsules During Exposure to Simulated Gastrointestinal and Refrigerated Conditions. LWT- Food Sci. Technol. 2014, 57(1), 406–411. DOI: 10.1016/j.lwt.2013.12.043.
- Chavarri´, M.; Maran˜on ´, I.; Ares, R.; Ib´ Anez ˜, F. C.; Marzo, F.; Villaran ´, M. Microencapsulation of a Probiotic and Prebiotic in Alginate-Chitosan Capsules Improves Survival in Simulated Gastro-Intestinal Conditions. Int. J. Food Microbiol. 2010, 142(1–2), 185–189. DOI: 10.1016/j.ijfoodmicro.2010.06.022.
- González-Ferrero, C.; Irache, J. M.; González-Navarro, C. J. Soybean Protein-Based Microparticles for Oral Delivery of Probiotics with Improved Stability During Storage and Gut Resistance. Food. Chem. 2018, 239, 879–888. DOI: 10.1016/j.foodchem.2017.07.022.
- Isa, T.; Zakaria, Z. A. B.; Rukayadi, Y.; Mohd Hezmee, M. N.; Jaji, A. Z.; Imam, M. U.; Hammadi, N. I.; Mahmood, S. K. Antibacterial Activity of Ciprofloxacin-Encapsulated Cockle Shells Calcium Carbonate (Aragonite) Nanoparticles and Its Biocompatability in Macrophage J774A.1. Int. J. Mol. Sci. 2016, 17(5), 713. DOI: 10.3390/ijms17050713.
- Borumand, M. R. Preparation and Characterization of Sodium Alginate Nanoparticles Containing ICD-85 (Venom Derived Peptides). IJIAS. 2013, 4(3), 534–542.
- Homayoonfal, M.; Mousavi, S. M.; Kiani, H.; Askari, G.; Desobry, S.; Arab-Tehrany, E. Encapsulation of Berberis Vulgaris Anthocyanins into Nanoliposome Composed of Rapeseed Lecithin. A Comprehensive Study on Physicochemical Characteristics and Biocompatibility. Foods. 2021, 10(3), 492. DOI: 10.3390/foods10030492.
- Tian, Y.; Liu, Y.; Zhang, L.; Hua, Q.; Liu, L.; Wang, B.; Tang, J. Preparation and Characterization of Gelatin-Sodium Alginate/Paraffin Phase Change Microcapsules. Colloids Surf. A Physicochem. Eng. Aspects. 2020, 586, 124216. DOI: 10.1016/j.colsurfa.2019.124216.
- Keskin, M.; Keskin, Ş.; Kolayli, S. Preparation of Alcohol Free Propolis-Alginate Microcapsules, Characterization and Release Property. LWT. 2019, 108, 89–96. DOI: 10.1016/j.lwt.2019.03.036.
- Hui, P. C. L.; Wang, W. Y.; Kan, C. W.; Ng, F. S. F.; Zhou, C. E.; Wat, E.; Leung, P. C.; Chan, C.-L.; Lau, C. B.-S.; Leung, P.-C. Preparation and Characterization of Chitosan/Sodium Alginate (CSA) Microcapsule Containing Cortex Moutan. Colloids And Surfaces A: Physicochem And Engine. Aspects. 2013, 434, 95–101. DOI: 10.1016/j.colsurfa.2013.05.043.
- Chadorshabi, S.; Hallaj-Nezhadi, S.; Ghasempour, Z. Liposomal System Based on Lyophilization of a Monophase Solution for Stabilization of Bioactives from Red Onion Skin. LWT. 2022, 172, 114174. DOI: 10.1016/j.lwt.2022.114174.
- Kanatt, S. R.; Tari, S.; Chawla, S. P. Encapsulation of Extract Prepared from Irradiated Onion Scales in Alginate Beads: A Potential Functional Food Ingredient. J. Food Meas. Charact. 2018, 12(2), 848–858. DOI: 10.1007/s11694-017-9699-7.
- Sathyabama, S.; Ranjith Kumar, M.; Bruntha Devi, P.; Vijayabharathi, R.; Brindha Priyadharisini, V. Co-Encapsulation of Probiotics with Prebiotics on Alginate Matrix and Its Effect on Viability in Simulated Gastric Environment. LWT- Food Sci. Technol. 2014, 57(1), 419–425. DOI: 10.1016/j.lwt.2013.12.024.
- Reale, A.; Di Renzo, T.; Rossi, F.; Zotta, T.; Iacumin, L.; Preziuso, M.; Parente, E.; Sorrentino, E.; Coppola, R. Tolerance of Lactobacillus Casei, Lactobacillus Paracasei and Lactobacillus Rhamnosus Strains to Stress Factors Encountered in Food Processing and in the Gastro-Intestinal Tract. LWT- Food Sci. Technol. 2015, 60(2), 721–728. DOI: 10.1016/j.lwt.2014.10.022.
- Bron, P. A.; Marco, M.; Hoffer, S. M.; Van Mullekom, E.; De Vos, W. M.; Kleerebezem, M. Genetic Characterization of the Bile Salt Response in Lactobacillus Plantarum and Analysis of Responsive Promoters in vitro and in situ in the Gastrointestinal Tract. J. Bacteriol. Res. 2004, 186(23), 7829–7835. DOI: 10.1128/JB.186.23.7829-7835.2004.
- Ballan, R.; Battistini, C.; Xavier-Santos, D.; Saad, S. M. I. Interactions of Probiotics and Prebiotics with the Gut Microbiota. Prog. mol. biol. transl. sci. 2020. DOI: 10.1016/bs.pmbts.2020.03.008.
- Dimitrellou, D.; Kandylis, P.; Petrovi´c, T.; Dimitrijevi´c-Brankovi´c, S.; Levi´c, S.; Nedovi´c, V.; Kourkoutas, Y. Survival of Spray Dried Microencapsulated Lactobacillus Casei ATCC 393 in Simulated Gastrointestinal Conditions and Fermented Milk. LWT - Food .Sci And Technol. 2016, 71, 169–174. DOI: 10.1016/j.lwt.2016.03.007.
- Mandal, S.; Puniya, A. K.; Singh, K. Effect of Alginate Concentrations on Survival of Microencapsulated Lactobacillus Casei NCDC-298. Int. Dairy. J. 2006, 16(10), 1190–1195. DOI: 10.1016/j.idairyj.2005.10.005.
- Li, K.; Wang, B.; Wang, W.; Liu, G.; Ge, W.; Zhang, M.; Yue, B.; Kong, M. Microencapsulation of Lactobacillus Casei BNCC 134415 Under Lyophilization Enhances Cell Viability During Cold Storage and Pasteurization, and in Simulated Gastrointestinal Fluids. LWT. 2019, 116, 116. DOI: 10.1016/j.lwt.2019.108521.
- Farias, T. G. S.; de Ladislau, H. F. L.; Stamford, T. C. M.; Medeiros, J. A. C.; Soares, B. L. M.; Stamford Arnaud, T. M.; Stamford, T. L. M. Viabilities of Lactobacillus Rhamnosus ASCC 290 and Lactobacillus Casei ATCC 334 (In Free Form or Encapsulated with Calcium Alginate-Chitosan) in Yellow Mombin Ice Cream. LWT. 2019, 100, 391–396. DOI: 10.1016/j.lwt.2018.10.084.
- Raddatz, G. C.; de Souza da Fonseca, B.; Poletto, G.; Jacob-Lopes, E.; Cichoski, A. J.; Muller, E. I.; Flores, E. M. M.; de Bona da Silva, C.; Ragagnin de Menezes, C. Influence of the Prebiotics Hi-Maize, Inulin and Rice Bran on the Viability of Pectin Microparticles Containing Lactobacillus acidophilus LA-5 Obtained by Internal Gelation/Emulsification. Powder. Technol. 2020, 362, 409–415. DOI: 10.1016/j.powtec.2019.11.114.
- da Silva, T. M.; de Deus, C.; de Souza Fonseca, B.; Lopes, E. J.; Cichoski, A. J.; Esmerino, E. A.; de Bona da Silva, C.; Muller, E. I.; Moraes Flores, E. M.; de Menezes, C. R. The Effect of Enzymatic Crosslinking on the Viability of Probiotic Bacteria (Lactobacillus acidophilus) Encapsulated by Complex Coacervation. Int. Food Res. J. 2019, 125, 125. DOI: 10.1016/j.foodres.2019.108577.
- Lee, H. C.; Jenner, A. M.; Low, C. S.; Lee, Y. K. Effect of Tea Phenolics and Their Aromatic Fecal Bacterial Metabolites on Intestinal Microbiota. Res. Microbiol. 2006, 157(9), 876–884. DOI: 10.1016/j.resmic.2006.07.004.