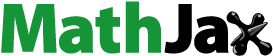
ABSTRACT
In this study, a high-calcium and high-amino acid fish frame hydrolyzate (FFH) was prepared from channel catfish (Ictalurus punetaus) through ultrasound-assisted enzymatic hydrolysis (UAEH). A 4-factor and 3-level Box-Behnken design of response surface methodology was employed to optimize the hydrolysis conditions, where the independent variables were sonication power (W), sonication time (min), enzyme activity (U) and water-substrate ratio (v/w). The degree of hydrolysis (DH) and soluble calcium content (SCC) were used as response factors. The optimal hydrolysis conditions were found to be sonication power (X1) = 492.91 W, sonication time (X2) = 15.01 min, enzyme activity (X3) = 6677.88 U, and water-to-substrate ratio (X4) = 3.56 v/w. Under these conditions, UAEH effectively disintegrated fish frame collagen and converted bound calcium to soluble calcium. Nevertheless, this study investigated the pattern of collagen transformation into short peptides and free amino acids throughout the degradation process. These findings highlight the potential of the FFH from channel catfish to serve as a foundation for creating highly nutritious food additive. The outcomes of this study further enhance the food industry’s ability to capitalize on the nutritional benefits offered by fish frame hydrolyzates. This expansion of health-focused product options and endorsement of the sustainable use of fish by-products harmonizes seamlessly with the industry’s evolving goals of waste reduction and value optimization.
Introduction
In 2020, global fish production reached a historic peak of 214 million tons, marking a growth rate exceeding 60% compared to the 1990s. This significant increase can be attributed largely to the expansion of aquaculture practices. Inland waters accounted for 62% (54 million tons) of the total aquaculture production.[Citation1] Among the freshwater aquaculture industry, channel catfish is one of the most significant species in the USA and China.[Citation2,Citation3] However, during the processing of catfish fillets, about 60% of the whole catfish is discarded as by-products, including heads, frames, skin, and viscera. Currently, only a small portion of these by-products is converted into low-value products such as animal feed and fertilizers, while the majority is disposed of at landfills, leading to the loss of valuable compounds and environmental pollution.[Citation4] Therefore, there is a clear need to valorize these catfish by-products. The circular economy approach that can be applied to the food industry emphasizes the transformation of food bio-wastes into value-added products, presenting a challenge as well as an opportunity for both the food industry and researchers.[Citation5]
The utilization of fish by-products through enzymatic hydrolysis to produce fish protein hydrolyzates has been recognized as an effective and sustainable approach to adding value to these underutilized resources,[Citation6,Citation7] while addressing environmental concerns associated with their disposal. Endopeptidases have the ability to swiftly cleave peptide chains, revealing hydrophobic amino acids that were initially embedded within the protein structure. This exposure of hydrophobic amino acids can lead to the development of bitterness in the sample, rendering it unsuitable as a potential ingredient for products aimed at enhancing flavor. Conversely, flavorzyme possesses a dual characteristic, functioning as both endopeptidases and exopeptidases. Notably, in our preliminary experiment, the degree of hydrolysis (DH) achieved by flavorzyme (approximately 25%) significantly outperformed that of various endopeptidases (DH being less than 15%), including neutral protease, alkaline protease, and papain. These comparisons were made under identical conditions of added enzyme activity (7500 U/g fish frame) (data not shown in the manuscript). Building upon our earlier research, where we employed Flavorzyme in the enzymatic hydrolysis of catfish frames to elevate flavor,[Citation8] it became evident that the resulting hydrolyzate exhibited a relatively low DH along with diminished soluble calcium content (SCC). These outcomes underscored the imperative for further enhancement of the hydrolyzate’s nutritional properties.
Ultrasonic/Ultrasound-assisted enzymatic hydrolysis (UAEH) refers to a technique that employs high-frequency sound waves to induce cavitation bubbles within a liquid combination of substrates and enzymes.[Citation9,Citation10] This process leads to the production of localized high temperatures and pressures that accelerate the enzymatic reaction rate and facilitate the conversion of complex molecules into smaller, more soluble forms. This method has been proved to speed up the enzymatic hydrolysis of proteins.[Citation11]
Calcium and collagen are combined in the fish frame through the formation of hydroxyapatite crystals, which are composed of calcium, phosphate, and hydroxyl ions and are embedded in a collagen matrix.[Citation12] The application of UAEH in the enzymatic hydrolysis of fish frames is promising due to the acceleration of the dissociation of bound state structures by cavitation bubbles generated by sonication. However, the optimal conditions for UAEH of catfish frames are yet to be determined, and identifying suitable conditions can aid in enhancing the nutritional properties of the hydrolyzate, which is a crucial step toward transitioning from experimental laboratory conditions to industrial-scale production.
Response surface methodology (RSM) allows for the efficient optimization of complex systems with multiple variables and responses, resulting in significant time and resource savings.[Citation13] Box-Behnken design (BBD) is one of the most effective experimental designs for process optimization. This method has been widely used to optimize the hydrolysis conditions of fish by-products.[Citation14–16]
The present study aimed to optimize the ultrasound-assisted enzymatic hydrolysis process of catfish fish (Ictalurus punetaus) frames using RSM. The main objective revolved around (centered on) maximizing both the DH and SCC. Additionally, the study aimed to investigate the changes in protein, peptides, and amino acids before and after hydrolysis, such that the treated hydrolyzate may be used as a base in the development of new and highly nutritious flavor offerings.
Material and methods
Materials and chemicals
Catfish frames (without heads, 14.19% protein, 16.14% fat, 58.86% moisture, 10.01% ash and 3.42% Ca, wet basis) were collected during processing from Guangdong Mingji Aquatic Group Co. (Guangdong, China) and transported in a frozen state to our lab. Flavorzyme (11000 U/g) containing Aspergillus oryzae protease/peptidase was purchased from SUNSON Co., Ltd (NingXia, China). Amino acid standards were purchased from Sigma (St. Louis, MO, USA). All the other chemicals and reagents used in this study were of analytical grade and bought from Sinopharm Chemical Reagent Co., Ltd (Shanghai, China).
Preparation of fish frame hydrolyzate (FFH)
Fish frames taken from the freezer (−18°C) were thawed at room temperature, and were sequentially treated with crushing, drying, fat-removing and endogenous enzymes inactivation according to the previous method.[Citation8] A sample of 5 g of the obtained mixture was combined with a corresponding proportion of water and added to a 50-mL centrifuge tube for ultrasound-assisted hydrolysis in a Supmile KQ-600E ultrasonic bath (Kunshan, China) operated at 40 kHz with an available volume of 22.5 L. After ultrasound treatment, the mixture was maintained in a 50°C electric thermostatic water bath (Yiheng DK-8AXX, Shanghai, China) with the addition of Flavorzyme. The enzymatic hydrolysis lasted 4 hours. Then, the protease was inactivated in a water bath (95°C, 15 min). The FFH underwent filtration via a gauze to eliminate insoluble components before subsequent investigation.
DH of protein
The extent of protein hydrolysis can be quantified by DH, which is expressed as the percentage of peptide bonds cleaved.[Citation17] The total nitrogen content of FFH was determined using the Kjeldahl method[Citation18] with a conversion factor of 6.25 for fish protein. Amino nitrogen was analyzed by titration with formaldehyde[Citation19] and expressed as mg/100 g sample. DH was calculated by EquationEquation 1.(1)
(1)
Soluble calcium content (SCC)
The FFH was centrifuged at 10,000 xg for 20 min at 4 ◦C and then pre-filtered using syringe filters (0.2 µm cellulose acetate membrane, VWR International, Puerto Rico, PR, USA). A total of 1.0 mL of centrifuged sample was diluted to 10 mL with 5% HNO3 followed by Atomic Absorption Spectrometry analyses (VARIAN AA240, Varian Medical Systems, Inc., California, USA) to obtain SCC.
Sodium dodecyl sulfate-polyacrylamide gel electrophoresis (SDS-PAGE)
The operation of electrophoresis was performed by following the improved method of.[Citation20] After electrophoresis, the gels were stained with Coomassie Blue Fast Staining Solution(Beyontime P0017, Shanghai, China)for 8 hours, and subsequently destained with Ultrapure water for 2 hours.
Size-exclusion high-performance liquid chromatography (SE-HPLC)
For peptide molecular weight distribution, FFH (1 mL) was suspended in 9 mL of 5% trichloroacetic acid and stirred for 1 h at room temperature (28 ± 1°C), then centrifuged at 10,000 xg for 15 min at 4°C. The supernatant was filtered through a 0.22-μm syringe filter and analyzed on HPLC (Waters 1525EF, Waters Corporation., Milford, USA) at 220 nm with a TSK-GEL G2000 SWXL Column (7.8 × 300 mm, Tosoh Bioscience, Tokyo, Japan). The mobile phase was acetonitrile containing 0.1% (w/v) TFA (trifluoroacetic acid) and the flow rate was 0.5 mL/min. The eluted peptide was detected at 220 nm and the column temperature was set at 30 ◦C.[Citation21] Cytochrome c (12,000 Da), Bacillus enzymes (1450 Da), glycine-glycine-tyrosine-arginine (451 Da) and glycine – glycine - glycine (180 Da) from Sigma-Aldrich, Inc. (St. Louis, MO, USA) were utilized to construct the molecular weight (MW) calibration curve by determining the average retention time. The peptide content was computed by measuring the peak areas and was presented as a percentage.
Amino acid analysis
The analysis of free amino acids (FAA) in FHH followed the method described by Gao et al..[Citation8] For the determination of total amino acids (TAA), solid samples weighing 100 mg or liquid samples measuring 1 mL were mixed with 6 M HCL to obtain a total volume of 8 mL. The mixture was then subjected to hydrolysis at 120°C for 22 hours.
Experimental design
The FFH was obtained according to the experimental conditions determined by a Box-Behnken design (BBD) of response surface methodology (RSM) generated by the software Design-Expert 13 (Stat-Ease Inc, Minneapolis, USA). BBD designs involve studying process variables at three levels (1, 0, 1), which are codes for the four factors including sonication power (ultrasonic generator power), sonication time, enzyme activity and water-substrate ratio (The water-to-substrate ratio of 2, 3, 4 designed in this study corresponded to a sample volume of 10 mL, 15 mL, 20 mL). shows the independent variables, their respective levels, and the codes used in this study.
Table 1. Original and coded values of the independent variables of the hydrolysis process.
The BBD plan comprised 29 trials, each carried out in triplicate, with the average DH and SCC reported as dependent variables. To predict the optimal point, a second-order polynomial model was used to correlate the independent and dependent variables. The quadratic model was used to predict the dependent variables and is represented by the following equation:
where represents the predicted values (DH and SCC),
represents the intercept. The coefficients of the model are denoted by
for linear terms,
for quadratic terms, and
for interaction terms.
and
represent the values of two independent variables in the model, where “i” and “j” are two different variables.
Statistical analysis
Design-expert program was utilized to investigate the effects of various variables and their interactions. Mean value differences of the obtained data were evaluated by -ANOVA with Duncan’s multiple range test (P < .05). The SPSS statistic program (Version 26.0, SPSS Inc., Chicago, IL, USA) was employed for this analysis.The F-test was employed to assess the statistical significance of the regression coefficients, while the quality of fit of the polynomial model was expressed by the coefficient of determination (R2) and lack of fit. 3D response surface plots were generated using Origin 2021 software from OriginLab Corporation (Northampton, USA).
Results and discussion
A high-calcium and high-amino acid hydrolyzate was prepared from channel catfish (Ictalurus punctatus) fish frame in this study. To improve the DH and SCC of the FFH, the ultrasound-assisted method was employed in conjunction with enzymatic treatment. The hydrolysis was conducted under different levels of sonication power (420–540 W) for different periods of time (10–20 min) with different activities of the added enzyme (5000–8000 U) in different water-substrate ratios (2–4). The conditions were optimized using RSM.
Optimization of FFH conditions by BBD
Statistical analysis and model fitting
In the preliminary experiment, the optimization of ultrasound-assisted enzymatic hydrolysis (UAEH) of catfish frames identified several key design variables, including sonication power, sonication time, enzyme activity, and water-substrate ratio. Based on these findings, these variables were considered in the experimental design of the study. In the preliminary single-factor experiment, high levels of DH and SCC were obtained under the selected conditions (). presents the matrix of experimental design and responses, while Table S1 displays the ANOVA results for the DH and SCC responses.
Table 2. Box-Behnken design (BBD) with experimental values for degree of hydrolysis (DH) and soluble calcium content (SCC) of fish frame hydrolyzate (FFH).
Through fitting the data with various models and subsequent ANOVA, it was found that quadratic polynomial models were best suited to describe the relationship between the hydrolysis conditions and DH and SCC responses. The final equations in terms of coded factors are provided as follows:
The coefficients of each term in the quadratic polynomial models provide insights into the effect of independent variables on the DH and SCC responses. They indicate the expected changes in responses per unit change in factor values, assuming that the other factors are held constant. A higher absolute value of the coefficient signifies a greater significance of the corresponding independent variable, regardless of its sign.[Citation22]
Table S1 shows that the P-values for the quadratic polynomial models were very low (P < .0001), suggesting that the models were significant and suitable for the accurate prediction of DH and SCC responses within the ranges of the variables studied. Moreover, the P-values for the Lack-of-Fit test (0.4677 and 0.0896) were both greater than 0.05, indicating that the models were not significantly different from the pure error and fit well with the experimental data. The coefficient of determination (R2) values for the models were 0.9727 and 0.9242, indicating their accuracy in predicting the responses.
Diagnostics plots of model adequacy
Diagnostic plots comparing the predicted values to the actual values were used to assess the adequacy of the DH and SCC models. As shown in , the plots of predicted values versus observed values indicated a relatively linear distribution of the experimental points, suggesting good agreements between the predicted values and the experimental data. These plots also indicated that the experimental errors were normally distributed, being consistent with the findings of Bruno Siewe et al. and Schützenmeister et al..[Citation23,Citation24]
Response surface analysis of DH
DH is a direct indicator of the hydrolysis characteristics and represents the percentage of peptide bond reduction in the hydrolyzate. The results of ANOVA showed that DH was significantly associated with the linear terms of sonication power (X1), enzyme activity (X3), and water-to-substrate ratio (X4), as well as the quadratic terms (P < .05) (Table S1).
Include figures in separate files?
Ultrasound-assisted enzymatic hydrolysis (UAEH) is a process in which ultrasound is transmitted through a liquid medium, resulting in the creation of voids or cavitation bubbles due to the exceeding of the minimum molecular distance required to hold the liquid intact. The cavitation effect can cause protein denaturation, which in turn releases hydrophilic groups and facilitates enzyme-substrate binding, leading to an increased protein conversion rate. This phenomenon can enhance the overall efficiency of the enzymatic hydrolysis process.[Citation25] Analysis of the 3D plots displayed in allowed us to draw some information regarding the effect of factors on DH. The DH increased initially and then decreased slowly with increasing sonication power (X1) and sonication time (X2). As ultrasound power increases, the intensity of cavitation also increases. Higher ultrasound power leads to more intense pressure fluctuations, which in turn create larger and more numerous cavitation bubbles. The implosions of cavitation bubbles near solid surfaces can lead to erosions or surface modifications.[Citation26,Citation27] This effect potentially causes disruptions of the physical barriers, such as protein aggregates or compact structures, making the protein molecules more accessible to enzymatic attacks.[Citation28,Citation29] However, a higher sonication power (>484.45 W) or a longer sonication time (>15.24 min) caused negative effects. The accumulated energy and thermal effects of sonication damaged the active sites of the protein, leading to a decrease in the accessibility of the peptide bond to the protease.[Citation30]
Figure 2. 3D response surface plots reflecting the effects of sonication power (X1), sonication time (X2), enzyme activity (X3) and water-substrate ratio (X4) on degree of hydrolysis (DH) (a-f) and soluble calcium content (SCC) (g-l), including the interaction of X1 and X2 (a, g), X1 and X3 (b, h), X1 and X4 (c, i), X2 and X3 (d, h), X2 and X4 (e, j), and X3 and X4 (f, k).

The enzyme activity (X3) was the independent variable that mainly affected DH from FFH (EquationEq. 3(3)
(3) ) evidenced by the highest coefficient of this factor. Its importance was visually highlighted in the 3D plot. The DH gradually increased as the enzyme activity increased from 2000 U to 6500 U. As the enzyme activity continued to increase, the rate of increase leveled off, indicating that the enzyme was supersaturated with the substrate protein at this point of time.
The water-to-substrate ratio (X4) exhibited a comparable trend to X1 and X2, where the central level (X4 = 3) of water -substrate ratio was found to be optimal. DH increased gradually with the water-to-substrate ratio at the lower level. This could be attributed to the fact that a lower water-to-substrate ratio limits the mobility of enzyme molecules, which hinders their efficient and rapid binding to the protein active sites.[Citation31] Additionally, a low water-to-substrate ratio may lead to an incomplete enzymatic hydrolysis, which covers the protein active sites and hinders the enzymatic reaction. However, the DH tended to drop when the water-to-substrate ratio was greater than the optimal value, possibly due to the dilution effect that reduced the contact frequency between the enzyme and substrates.[Citation32]
Response surface analysis of SCC
The binding of collagen to calcium salts in fish frames forms a dense structure, resulting in a very low solubility of calcium. Enzymatic hydrolysis is an effective technique for converting bound calcium to soluble calcium.[Citation33,Citation34] According to EquationEq. 3(3)
(3) , the SCC response was significantly correlated (P < .05) with the linear and quadratic terms of sonication power (X1), enzyme activity (X3), and water-to-substrate ratio (X4) only, as shown in Table S1.
The 3D surface analysis in provided insights into the impact of various factors on SCC. Increasing sonication power (X1) from 420 W to 480 W was found to moderately enhance the hydrolysis rate of SCC. The cavitation effect generated by ultrasonic energy can cause disruption of the internal tissue structure of the fish frame and the loosening of protein structures, which leads to the dissolution of calcium in the fish frame.[Citation35] Additionally, the high-speed oscillation effect of sonication can disperse the fish frame more uniformly,[Citation36] improving the binding between the enzyme and the substrate.
The trend observed in the SCC response with respect to sonication time (X2) was similar to that of the DH response. Initially, SCC increased rapidly and then decreased rapidly with increasing sonication time. The longer sonication times led to the formation of insoluble protein aggregates, resulting in a decrease in the accessibility of peptide bonds to the protease.[Citation21] Moreover, the thermal effect generated by sonication led to the recombination of calcium and protein, which caused a decrease in the solubility of calcium.[Citation37]
Enzyme activity (X3) was likewise the most significant influence on SCC response. An increase in enzyme activity from 5000 U to 8000 U resulted in a gradual increase in SCC, which subsequently plateaued out. At lower levels of the water-to-substrate ratio (X4), there was a rapid increase in SCC with increasing ratio due to the difference in calcium concentration between the fish frame and the liquid. However, as the water-to-substrate ratio was further increased, the negative effect of low enzyme concentration became dominant, resulting in a rapid decrease of SCC.
Verification test under composite desirability
For multiple response values, the optimal conditions were different. In this study, optimization for multiple responses was performed using Design-Expert software to obtain optimal process conditions. The model simulation predicted the theoretical optimum conditions for the ultrasound-assisted enzymatic hydrolysis as follows: X1 = 492.91 W, X2 = 15.01 min, X3 = 6677.88 U and X4 = 3.56 v/w for sonication power, sonication time, enzyme activity and water-to-substrate ratio. Under theoretical conditions, the predictive DH and SCC were 29.39%, 17.76 mg/100 mL, respectively.
To validate the accuracy of the RSM model predictions, the optimal process conditions were applied to carry out ultrasound-assisted enzymatic hydrolysis, and the resulting DH and SCC values were measured experimentally. The obtained experimental DH and SCC values were 28.78 ± 0.56% and 17.36 ± 0.39 mg/100 mL, respectively, and showed no significant difference from the predicted values (P > .05). This close agreement between the experimental and predicted values confirms the reliability and accuracy of the RSM models in predicting the variations in DH and SCC responses.
Changes of protein during enzymatic hydrolysis
Electrophoretic analysis and peptide molecular weight distribution
shows the results of electrophoretic analysis (SDS-PAGE) used to evaluate the impact of ultrasound-assisted enzymatic hydrolysis on the treated samples. In the fish frame, a prominent band was observed at > 245 kDa, which is in agreement with the characteristics of collagen. The major form of collagen present in the fish frame is type I collagen, comprising two ɑ1 chains and one ɑ2 chain, each with a molecular weight of around 100 kDa.[Citation38] Another significant band observed was actin, which has a molecular weight of approximately 45 kDa and is likely derived from the fish flesh attached to the fish frame.
Figure 3. SDS-PAGE analysis (a), molecular weight distribution of peptides (b-c), and amino acid composition (d) of fish frame(FF) and fish frame hydrolysis (FFH). M: molecular weight marker.

Compared to the fish frame, the bands in the range of 10–245 kDa were almost invisible for FFH. This indicates that the macromolecular protein in the fish frame was significantly hydrolyzed. The hydrolysis primarily occurred in the inner region of the polypeptide chain, leading to an increase in the yield of low molecular weight proteins and peptides. This hydrolysis is mainly attributed to the activity of Flavorzyme endopeptidase.[Citation39]
SE-HPLC method was used to further study the effect of UAEH on the protein, which can reflect the changes of small molecular proteins and peptides in fish frames by the particle size classification.[Citation40] It could be seen from that almost all macromolecules larger than 10 kDa were degraded. The peptides below 1,000 Da were the most abundant, reaching 92.67%. This was also consistent with the changes of protein bands in SDS-PAGE (). Usually, peptides with molecular mass of less than 5,000 Da are called low molecular weight peptides, nearly 90% of which are absorbed by the human body.[Citation41] Therefore, UAEH could be considered as an effective method to enhance the nutritional properties and absorption of fish frame hydrolyzate products.
Changes in amino acid composition
The amino acid compositions of fish frames and FFH are presented in and there were 17 amino acids measured for both samples. The amino acid composition in fish frames had the typical characteristics of collagen, with proline accounting for 19.13%.[Citation38]
Table 3. Total amino acids (TAA) of fish frame (g/kg) and free amino acids (FAA) of fish frame hydrolyzate (FHH) (mg/100 mL).
Compared to the amino acid composition of the fish frame, the amino acid composition of FFH obtained by UAEH was quite different. Seventeen free amino acids were detected in FFH, and the amino acids with high contents were Leu, Ile, Arg and Val. In terms of amino acid types, the proportion of essential amino acids (except for tryptophan) to total amino acids increased from 34.11% to 62.41%, which demonstrated that FFH can be a good source of amino acids and peptides, consistent with reports for cod and trout by-products.[Citation42,Citation43]
Free amino acids (FAA) are recognized for their ability to impact food flavor, specifically umami, sweetness, and bitterness.[Citation8] The analysis of umami and sweet amino acid proportions in FFH indicated lower levels than those found in the fish frame, whereas the proportion of bitter amino acids was notably higher. This implied that the hydrolyzate could potentially be considered as a viable raw material for the extraction of bitter amino acids or for use as food additives after the debittering process.
Conclusion
Using a Box-Behnken design, we conducted a multi-response optimization study to determine the ideal conditions for producing a high-calcium and high-amino acid fish frame hydrolyzate from channel catfish (Ictalurus punetaus) using ultrasound-assisted enzymatic hydrolysis. Our findings showed that enzyme activity had the greatest impact on the degree of hydrolysis and soluble calcium content, followed by water-to-substrate ratio and sonication power. However, the influence of sonication time was not significant, possibly due to the attenuation of sonication intensity over time. Under the optimal conditions, ultrasound-assisted enzymatic hydrolysis effectively broke down fish frame collagen and improved the nutritional properties of the hydrolyzates by increasing the concentration of peptides, free amino acids and soluble calcium. These findings highlight the potential of the FFH from channel catfish to serve as a foundation for creating highly nutritious food additive. In essence, this study’s findings contribute to the food industry’s ability to harness the nutritional benefits of fish frame hydrolyzates, thereby expanding the range of health-focused products and promoting sustainable utilization of fish by-products, aligning with the industry’s evolving goals of waste reduction and value maximization.
Author Contribution
Zhiqing Zhang: conceptualization, methodology, software, investigation, formal analysis, writing – original draft; Gao Pei: conceptualization, funding acquisition, resources, supervision, writing – review & editing; Qixing Jiang: resources, supervision; Zifeng Zhang: visualization, investigation; Xiaojing Zhang: validation; Fang Yang: project administration; Peipei Yu: project administration; Shaoquan Liu: writing – review & editing; Wenshui xia: supervision.
Supplemental Material
Download MS Word (16.7 KB)Disclosure statement
No potential conflict of interest was reported by the author(s).
Acknowledgement
We extend our sincere gratitude to our dedicated team members whose collaborative efforts and expertise were instrumental in the successful completion of this study. Your contributions have enriched every aspect of our research. We also express our appreciation to the anonymous reviewers whose insightful feedback significantly enhanced the quality and depth of this paper. Their constructive comments played a crucial role in refining our work. Our heartfelt thanks to all who contributed to the realization of this research.
Data availability statement
The authors confirm that all data included in this study are available upon request by contact with the corresponding author.
Supplemental data
Supplemental data for this article can be accessed online at https://doi.org/10.1080/10942912.2023.2281886
Additional information
Funding
References
- Food and Agriculture Organization of the United Nations (FAO). The State of World Fisheries and Aquaculture 2022; Food and Agriculture Organization of the United Nations: Rome, 2022.
- Viglia, S.; Brown, M. T.; Love, D. C.; Fry, J. P.; Scroggins, R.; Neff, R. A. Analysis of Energy and Water Use in USA Farmed Catfish: Toward a More Resilient and Sustainable Production System. J. Cleaner Prod. 2022, 379(2), 134796. DOI: 10.1016/j.jclepro.2022.134796.
- Zhou, Z.; Dai, Y. Y.; Yuan, Y.; He, Y. H.; Zhou, J. Status, Trends, and Prospects of the Channel Catfish Industry in China and the Impact of the COVID-19 Epidemic. Israeli J. Aquacult. Bamidgeh. 2021, 73, 1490779. DOI: 10.46989/001c.27636.
- Zhang, Y.; Chang, S. K. C. Protein Extraction from Catfish Byproducts and Physicochemical Properties of the Protein Isolates. J. Food Sci. 2021, 86(7), 3061–3074. DOI: 10.1111/1750-3841.15805.
- Sousa, P. M.; Moreira, M. J.; de Moura, A. P.; Lima, R. C.; Cunha, L. M. Consumer Perception of the Circular Economy Concept Applied to the Food Domain: An Exploratory Approach. Sustainability. 2021, 13(20), 11340. DOI: 10.3390/su132011340.
- Borges, S.; Odila, J.; Voss, G.; Martins, R.; Rosa, A.; Couto, J. A.; Almeida, A.; Pintado, M. Fish By-Products: A Source of Enzymes to Generate Circular Bioactive Hydrolysates. Molecules. 2023, 28(3), 1155. DOI: 10.3390/molecules28031155.
- Naghdi, S.; Rezaei, M.; Tabarsa, M.; Abdollahi, M. Fish Protein Hydrolysate from Sulfated Polysaccharides Extraction Residue of Tuna Processing By-Products with Bioactive and Functional Properties. Global Challeng. 2023, 7(4), 2200214. DOI: 10.1002/gch2.202200214.
- Gao, P.; Zhang, Z.; Ge, Y.; Cao, S.; Zhang, X.; Jiang, Q.; Xu, Y.; Xia, W.; Liu, S. Co-Inoculation of Lactiplantibacillus pentosus 1 and Saccharomyces cerevisiae 31 for a Salt-Free Fish Sauce Production from Channel Catfish (Ietalurus punetaus) Bone. Food Biosci. 2022, 50, 102137. DOI: 10.1016/j.fbio.2022.102137.
- Izadiyan, P.; Hemmateenejad, B. Multi-Response Optimization of Factors Affecting Ultrasonic Assisted Extraction from Iranian Basil Using Central Composite Design. Food Chem. 2016, 190, 864–870. DOI: 10.1016/j.foodchem.2015.06.036.
- Zhu, Y.; Zhang, M.; Law, C. L.; Wang, Y.; Liu, K. Optimization of Ultrasonic-Assisted Enzymatic Hydrolysis to Extract Soluble Substances from Edible Fungi By-Products. Food Bioprocess. Technol. 2023, 16(1), 167–184. DOI: 10.1007/s11947-022-02930-0.
- Kangsanant, S.; Murkovic, M.; Thongraung, C. Antioxidant and nitric oxide inhibitory activities of tilapia (O reochromis niloticus) protein hydrolysate: effect of ultrasonic pretreatment and ultrasonic-assisted enzymatic hydrolysis. Inter. J. Food Sci. Technol. 2014, 49(8), 1932–1938. DOI: 10.1111/ijfs.12680.
- Du, C.; Cui, F.; Zhang, W.; Feng, Q.; Zhu, X.; De Groot, K. Formation of Calcium Phosphate/Collagen Composites Through Mineralization of Collagen Matrix. J. Biomed. Mater. Res. 2000, 50(4), 518–527. DOI: 10.1002/(SICI)1097-4636(20000615)50:4<518:AID-JBM7>3.0.CO;2-W.
- Khuri, A. I.; Mukhopadhyay, S. Response Surface Methodology. Wiley Interdisciplinary Rev. 2010, 2(2), 128–149. DOI: 10.1002/wics.73.
- Ahmadkelayeh, S.; Cheema, S. K.; Hawboldt, K. Extraction of Astaxanthin from Atlantic Shrimp By-Products Using Fish Oil: Process Optimization and Operational Parameter Effects. J. Cleaner Prod. 2022, 371, 133609. DOI: 10.1016/j.jclepro.2022.133609.
- Bui, X. D.; Vo, C. T.; Bui, V. C.; Pham, T. M.; Bui, T. T. H.; Nguyen-Sy, T.; Show, P. L.; Chew, K. W.; Mukatova, M. D.; Show, P. L. Optimization of Production Parameters of Fish Protein Hydrolysate from Sardaorientalis Black Muscle (By-Product) Using Protease Enzyme. Clean Technol. Environ. Policy. 2021, 23(1), 31–40. DOI: 10.1007/s10098-020-01867-2.
- Haq, M.; Getachew, A. T.; Saravana, P. S.; Cho, Y.-J.; Park, S.-K.; Kim, M.-J.; Chun, B.-S. Effects of Process Parameters on EPA and DHA Concentrate Production from Atlantic Salmon By-Product Oil: Optimization and Characterization. Korean J. Chem. Eng. 2017, 34(8), 2255–2264. DOI: https://doi.org/10.1007/s11814-016-0362-5.
- Nielsen, P.; Petersen, D.; Dambmann, C. Improved Method for Determining Food Protein Degree of Hydrolysis. J. Food Sci. 2001, 66(5), 642–646. DOI: 10.1111/j.1365-2621.2001.tb04614.x.
- Kirk, P. L. Kjeldahl Method for Total Nitrogen. Anal. Chem. 1950, 22(2), 354–358. DOI: 10.1021/ac60038a038.
- Rutherfurd, S. M. Methodology for Determining Degree of Hydrolysis of Proteins in Hydrolysates: A Review. J. AOAC Int. 2010, 93(5), 1515–1522. DOI: 10.1093/jaoac/93.5.1515.
- Chen, N.; Jiang, Q.; Gao, P.; Yu, D.; Yang, F.; Xu, Y.; Xia, W. Gel Properties, Physicochemical Properties, and Sensory Attributes of White Leg Shrimp (Litopenaeus vannamei) Surimi Gel Treated with Sodium Chloride (NaCl) Substitutes. Int. J. Food Sci. Tech. 2023, 58(1), 22–36. DOI: https://doi.org/10.1111/ijfs.16157.
- Wang, Y.; Li, B.; Guo, Y.; Liu, C.; Liu, J.; Tan, B.; Guo, Z.; Wang, Z.; Jiang, L. Effects of Ultrasound on the Structural and Emulsifying Properties and Interfacial Properties of Oxidized Soybean Protein Aggregates. Ultrason. Sonochem. 2022, 87, 106046. DOI: 10.1016/j.ultsonch.2022.106046.
- Al-Bukhaiti, W. Q.; Al-Dalali, S.; Noman, A.; Qiu, S.; Abed, S. M.; Qiu, S.-X. Response Surface Modeling and Optimization of Enzymolysis Parameters for the In Vitro Antidiabetic Activities of Peanut Protein Hydrolysates Prepared Using Two Proteases. Foods. 2022, 11(20), 3303. DOI: 10.3390/foods11203303.
- Bruno Siewe, F.; Kudre, T. G.; Narayan, B. Optimisation of Ultrasound-Assisted Enzymatic Extraction Conditions of Umami Compounds from Fish By-Products Using the Combination of Fractional Factorial Design and Central Composite Design. Food Chem. 2021, 334, 127498. DOI: 10.1016/j.foodchem.2020.127498.
- Schützenmeister, A.; Jensen, U.; Piepho, H.-P. Checking Normality and Homoscedasticity in the General Linear Model Using Diagnostic Plots. Commun. Stat.- Simul. Comput. 2012, 41(2), 141–154. DOI: 10.1080/03610918.2011.582560.
- Chen, L.; Ettelaie, R.; Akhtar, M. Improved Enzymatic Accessibility of Peanut Protein Isolate Pre-Treated Using Thermosonication. Food Hydrocolloids. 2019, 93, 308–316. DOI: 10.1016/j.foodhyd.2019.02.050.
- Cichowska, J.; Witrowa-Rajchert, D.; Stasiak-Rozanska, L.; Figiel, A. Ultrasound-Assisted Osmotic Dehydration of Apples in Polyols and Dihydroxyacetone (DHA) Solutions. Molecules. 2019, 24(19), 3429. DOI: https://doi.org/10.3390/molecules24193429.
- Reche, C.; Rossello, C.; Umana, M. M.; Eim, V.; Simal, S. Mathematical Modelling of Ultrasound-Assisted Extraction Kinetics of Bioactive Compounds from Artichoke By-Products. Foods. 2021, 10(5), 931. DOI: 10.3390/foods10050931.
- Carreira-Casais, A.; Otero, P.; Garcia-Perez, P.; Garcia-Oliveira, P.; Pereira, A. G.; Carpena, M.; Prieto, M. A.; Simal-Gandara, J.; Prieto, M. A. Benefits and Drawbacks of Ultrasound-Assisted Extraction for the Recovery of Bioactive Compounds from Marine Algae. Int. J. Environ. Res. Public Health. 2021, 18(17), 9153. DOI: 10.3390/ijerph18179153.
- Zeng, S. I.; Wang, K.; Wu, G. Y.; Liu, X. W.; Hu, Z. Y.; Li, W. C.; Zhao, L. Time-Specific Ultrasonic Treatment of Litchi Thaumatin-Like Protein Inhibits Inflammatory Response in RAW264.7 Macrophages via NF-Κb and MAPK Transduction Pathways. Ultrason. Sonochem. 2023, 95, 106355. DOI: 10.1016/j.ultsonch.2023.106355.
- Liu, X.; Sun, X.; Wei, Y.; Ma, Y.; Sun, P.; Li, X. Effects of Ultrasonic Treatment on Physico-Chemical Properties and Structure of Tuna (Thunnus tonggol) Myofibrillar Proteins. J. Food Compost. Anal. 2022, 108, 104438. DOI: 10.1016/j.jfca.2022.104438.
- Jamshidi, A.; Shabanpour, B.; Pourashouri, P.; Raeisi, M. Evaluation of Different Proportions of Distilled Water to Substrate on Functional Properties, Antioxidant and Nutritional Quality of Bigeye Ilisha (Ilisha megaloptera) Protein Hydrolysate. Jorjani Biomed. J. 2018, 6(3), 24–39. DOI: 10.29252/jorjanibiomedj.6.3.24.
- Sutthiwanjampa, C.; Kim, S. M. Production and Characterisation of Hyaluronidase and Elastase Inhibitory Protein Hydrolysates from Venus Clam. Nat. Prod. Res. 2015, 29(17), 1614–1623. DOI: 10.1080/14786419.2014.990903.
- Jeon, Y.-J.; Karawita, R.; Heo, S.-J.; Kim, S.-K.; Song, C. B. Recovery of Bioavailable Calcium from Alaska Pollack (Theragra chalcogramma) Fish Backbone By-Products by Pepsinolytic Hydrolysis. Preventive Nutr. Food Sci. 2006, 11(2), 120–126. DOI: 10.3746/jfn.2006.11.2.120.
- Ling, Z.; Ming-Gui, Z.; Rong, C.; Qi, L.; Hui-Hui, S. Optimization of Preparation Technology of Cod Mince Enzymolysis for Calcium Absorption-Promoting Peptides. J. Food Saf. Qual. 2018, 9(8), 1911–1917.
- Guo, J.; Zhu, S.; Chen, H.; Zheng, Z.; Pang, J. Ultrasound-Assisted Solubilization of Calcium from Micrometer-Scale Ground Fish Bone Particles. Food Sci. Nutri. 2022, 10(3), 712–722. DOI: 10.1002/fsn3.2696.
- Asih, I.; Kemala, T.; Nurilmala, M. (2019). Halal Gelatin Extraction from Patin Fish Bone (Pangasius hypophthalmus) By-Product with Ultrasound-Assisted Extraction. IOP Conference Series: Earth and Environmental Science, 299, 012061. 10.1088/1755-1315/299/1/012061
- An, Y. Q.; Liu, Q.; Xie, Y. R.; Xiong, S. B.; Yin, T.; Liu, R. Aggregation and Conformational Changes of Silver Carp Myosin as Affected by the Ultrasound-Calcium Combination System. J. Sci. Food Agric. 2018, 98(14), 5335–5343. DOI: 10.1002/jsfa.9073.
- Nagai, T.; Suzuki, N. Preparation and Characterization of Several Fish Bone Collagens. J. Food Biochem. 2000, 24(5), 427–436. DOI: 10.1111/j.1745-4514.2000.tb00711.x.
- Xu, Y.; Galanopoulos, M.; Sismour, E.; Ren, S.; Mersha, Z.; Lynch, P.; Almutaimi, A. Effect of Enzymatic Hydrolysis Using Endo-And Exo-Proteases on Secondary Structure, Functional, and Antioxidant Properties of Chickpea Protein Hydrolysates. J. Food Meas. Charact. 2020, 14(1), 343–352. DOI: 10.1007/s11694-019-00296-0.
- Qu, J.; Zhang, M.; Hong, T.; Xu, X.; Xu, D. Improvement of Adzuki Bean Paste Quality by Flavourzyme-Mediated Enzymatic Hydrolysis. Food Biosci. 2023, 51, 102205. DOI: 10.1016/j.fbio.2022.102205.
- Yang, L.; Guo, Z.; Wei, J.; Han, L.; Yu, Q.-L.; Chen, H.; Chen, Y.; Zhang, W. Extraction of Low Molecular Weight Peptides from Bovine Bone Using Ultrasound-Assisted Double Enzyme Hydrolysis: Impact on the Antioxidant Activities of the Extracted Peptides. LWT. 2021, 146, 111470. DOI: 10.1016/j.lwt.2021.111470.
- Jafarpour, A.; Gomes, R. M.; Gregersen, S.; Sloth, J. J.; Jacobsen, C.; Sørensen, A.-D. M. Characterization of Cod (Gadus morhua) Frame Composition and Its Valorization by Enzymatic Hydrolysis. J. Food Compost. Anal. 2020, 89, 103469. DOI: 10.1016/j.jfca.2020.103469.
- Korkmaz, K.; Tokur, B. Optimization of Hydrolysis Conditions for the Production of Protein Hydrolysates from Fish Wastes Using Response Surface Methodology. Food Biosci. 2022, 45, 101312. DOI: 10.1016/j.fbio.2021.101312.