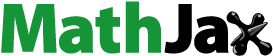
ABSTRACT
Butter is generally made from milk fat, which contains high levels of saturated fatty acids, thus providing health risks. Therefore, alternatives are needed, namely recombined butter by substituting other healthier saturated fats, namely fat rich in monoacylglycerols (MAG) and diacylglycerols (DAG) from glycerolysis of coconut stearin which is rich in medium chain fatty acids, which are then called MAG-DAG fat (MDF). This research aimed to determine the effect of MDF substitution in recombined butter, which produced the best physicochemical and sensorial properties. The substitution of MDF was carried out at concentrations 0%, 5%, 10%, 15%, and 20%. Substitution of MDF had a significant effect on physicochemical properties and sensory acceptability. Recombined butter with substitution of MDF-5% produced good physicochemical properties and was liked by the panelists. Recombined butter of MDF-5% contained MAG, DAG, and triacylglycerol (TAG) of 0.48%, 8.71%, and 90.81%, respectively, and it had a higher medium chain fatty acids (MCFAs) content, namely 10.72% compared to the MDF-0% (control) which was only 7.45%. The hardness, adhesiveness, and cohesiveness of recombined butter of MDF-5% were about 1247.44 N, −671.28 N, and 0,09 N, respectively. The crystal microstructure was in the form of small spherulites in large quantities, and polymorphism showed a mixture of β’- and β-crystals. Substitution of MDF-5% did not change the new functional groups, but increased the intensity of the hydroxyl groups. Substitution of MDF in recombined butter was suitable to produce butter with good characteristics and potentially healthier.
Introduction
Butter is an emulsion product, generally made from 80% milk fat, water, and other food additives.[Citation1,Citation2] However, milk fat contains high amounts of saturated fatty acids, especially palmitate, myristate, and stearate, so it can have a negative impact on health.[Citation3] Therefore, researchers are looking for alternatives to make recombined butter by substituting milk fat with other fat types with better health effects.[Citation4,Citation5]
Some researchers have modified butter, especially by substituting milk fat, including substitution with fish oil,[Citation6] gamma-linolenic acid,[Citation7] and sunflower oil.[Citation8] This substitution can improve the nutritional value of the recombined butter; however, substituting fat rich in unsaturated fatty acids can reduce the texture and stability of the fat crystals formed so that the butter becomes too soft and melts easily. Substitutions can also be made using saturated fats such as palm stearin or hydrogenated vegetable oil, which can maintain physicochemical properties, but saturated fats are not suitable for health.[Citation9–11] Another alternative is substituting saturated fats rich in medium-chain fatty acids (MCFAs), including coconut stearin. Coconut stearin contains MCFAs, especially in the form of laurate, reaching about 50%, where laurate has been proven to improve the immune system.[Citation12,Citation13] The positive effects of laurate and other MCFAs are even better when in the form of monoacylglycerols (MAG) and diacylglycerols (DAG) because they have better biological activity than in the form of triacylglycerols (TAG).[Citation14,Citation15]
Fats rich in MAG and DAG that contain MCFA can be obtained from coconut stearin, which is glycerolyzed to become MAG and DAG.[Citation16,Citation17] The study on the use of fats rich in MAG and DAG from coconut stearin as a substitute for recombined butter is still limited. This fat could potentially be suitable for use as a substitute for milk fat in making recombined butter because it contains MAG and DAG, which can improve physicochemical properties, act as an emulsifier, and provide sound health effects.[Citation18–20] MAG and DAG also prevent fat accumulation, obesity, and other weight-related problems.[Citation14,Citation21]
This research aimed to determine the effect of the substitution of fats rich in MAG and DAG, which was then called MAG-DAG fat (MDF), from glycerolysis of coconut stearin for the production of recombined butter. MDF is a fat rich in MAG and DAG obtained from glycerolysis of coconut stearin, which is rich in laurate; it has excellent therapeutic potential for health, especially in the form of monolaurin because it can reduce the risk of heart disease, obesity, and improve the body’s immune system. An assessment was carried out on the physicochemical and sensorial properties so that the appropriate concentration of MDF substitution was obtained to produce recombined butter with good characteristics and was liked by the panelists.
Materials and methods
Materials
Anhydrous milk fat (AMF) was obtained from NZMP (New Zealand), coconut stearin was from Mamaco PT Sari Mas Permai (Surabaya, Indonesia), skim milk powder (SMP) was from NZMP (New Zealand), lecithin, salt, and water. The chemicals such as glycerol, NaOH, citric acid, glacial acetic acid, diethyl ether, and hexane were obtained from Merck KGaA.
Preparation of MAG-DAG fat (MDF)
MDF was prepared by glycerolysis of coconut stearin, according to Subroto et al.,[Citation22] with some modifications. 50 g of coconut stearin was heated on a hotplate until it melted, and 12.5 g of glycerol was added. The catalyst of NaOH 3% was pounded using a pestle and mortar. The NaOH was mixed into the substrate while stirring, then heated at 90°C for 6 h and stirred using a magnetic stirrer. The reaction mixture was then neutralized using 20% citric acid to a pH of 7. The salt formed and residual glycerol were then separated using a centrifuge at 5000 rpm for 15 min. The top layer of MAG-DAG fat (MDF) was taken and stored in the refrigerator until used.
Preparation of recombined butter
Production of recombined butter was prepared according to Subroto et al.[Citation6] with some modifications. The fat-based materials consist of anhydrous milk fat and MDF with various concentrations of MDF (0, 5, 10, 15, and 20% w/w) were mixed, so the total fat was 80 g. The concentrations of MDF used range from 0–20% because, generally, the use of fats rich in MAG DAG was only compatible around 5–15%. Water-based materials consisting of 14 g water, 5 g skim milk powder, and 1 g NaCl were mixed until homogeneous. The water- and fat-based blend were mixed and pasteurized using a magnetic stirrer at 300 rpm, 80°C for 15 min. The mixture was then homogenized using a hand mixer in a container filled with ice water to a temperature of 5–10°C, and the recombined butter was tempered in the refrigerator at 10°C for 48 h. The number of experiments carried out in duplicate.
Analysis of acylglycerol composition
Analysis of acylglycerol was carried out using thin-layer chromatography (TLC) with the modified method of Esperón-Rojas et al.[Citation23] and Fuchs et al..[Citation24] The TLC plate was activated by heating at 105°C for 30 min. The samples were then applied to the TLC plate and developed using the eluent mixture of n-hexane: diethyl ether: acetic acid glacial (8:2:0.2) as the mobile phase. The TLC plate was then subsequently dried. Iodine crystals were used to stain the samples. The area of the stain was calculated to quantify the results. The number of analyses was carried out in triplicate.
Emulsification capacity
Emulsification capacity was determined according to Cano-Medina et al..[Citation25] The vegetable oil and water were mixed in a test tube with a ratio of 1:1. Then, a 1% sample emulsifier was added. The mixture was homogenized with a vortex mixer. After homogenization, an emulsion occurred. The emulsion formed was determined by measuring the volume or height of the emulsion in a test tube, and the percentage of emulsification capacity was calculated. The number of analyses was carried out in triplicate using the following formula:
Instrumental texture profile
The texture profile was determined according to Subroto et al.[Citation6] using the Texture Analyzer TXT 32. Samples were compressed with a 50 mm diameter P/6 probe twice at a temperature of 15°C. The probe’s speed was set at 1 mm/s pretest speed, 5 mm/s test speed, 5 mm/s posttest speed, a distance of 10 mm, and a trigger force of 5 g. The hardness, adhesiveness, and cohesiveness were measured in triplicates.
Melting profiles
The melting profile was analyzed using a Shimadzu DSC-60 Plus, Japan, which was equipped with a helium gas supply as an inert gas.[Citation26] 10 mg of butter was weighed, placed in an aluminum pan, and closed tightly. Meanwhile, an empty and tightly closed aluminum pan was used as a reference. Determination of the melting profile was carried out using the DSC instrument setting, namely at a temperature of −30°C to 80°C with a temperature increase speed of 5°C/min.
Sensory evaluation
Sensory evaluation of butter was carried out using the hedonic test method. Approximately 5 grams of butter sample was served in a cup, equipped with a small spoon and small knife and accompanied by white bread as a medium for testing spreadability and drinking water as an antidote. All materials and equipment were placed on the panelists’ tables, which were separated by partitions or booths. The panelists then evaluated sensory attributes such as color, aroma, taste, spreadability, and overall acceptability. The test was carried out on 20 panelists using the hedonic scale (1 = dislike extremely to 5 = like extremely). The samples were coded using three random digit numbers.
Analysis of microstructure
Analysis of microstructure was determined according to Campos et al.[Citation27] and Kinta and Hartel[Citation28] using a polarized light microscope (PLM). The butter sample was placed on a thin glass preparation, and then observed under PLM at room temperature. Observations were made at three different points at magnifications of 10× and 40 × .
Analysis of polymorphism
Analysis of polymorphism was determined according to Le Révérend et al.[Citation29] using X-ray diffraction (XRD). The butter sample was smeared on a glass slide or sample holder, and then incubated at room temperature for 10 min. The diffraction pattern was determined using XRD at 2-theta of 3–30 deg, and then the short spacing of the diffractogram peaks was determined with the formula λ = 2d sinθ.
Analysis of functional groups
Functional groups of butter were analyzed by “Nicolet iS10 FTIR spectrometer” with a spectral of 400 to 4000 cm−1, integrated with ATR. 2 mg butter was placed in the sample holder and then pressurized for 1–2 min, and the spectra of functional groups were displayed.
Analysis of fatty acid composition
The butter was melted and then 200 μL was taken for transmethylation by adding 400 μL of NaOH-methanol complex in a closed test tube. Transmethylation was carried out by heating at 90°C for 2 h. The reaction mixture was cooled then the fatty acid methyl esters (FAMEs) fraction was extracted using hexane. The FAMEs fraction was then injected into a gas chromatograph (GC) equipped with a CP Sil 8 CB according to AOCS Ce 1–62 column.[Citation30]
Statistical analysis
The data were statistically analyzed by one-way ANOVA with PASW Statistics 18. Tukey test was then applied to detect the significant differences at a level of p values < 0.05.
Results and discussion
Characteristics of coconut stearin and MDF
The characteristics of coconut stearin and MDF, including acylglycerols composition, melting point, and emulsification capacity, can be seen in . MDF resulting from glycerolysis of coconut stearin contained MAG, DAG, and TAG of 55.79%, 34.30%, and 9.92%, respectively. The low TAG content indicated that the glycerolysis process was running well. In the glycerolysis process, 1 mol of TAG could produce 3 mol of MAG, and the formation of DAG occurred when there was no excess of glycerol.[Citation19,Citation31]
Table 1. Characteristics of coconut stearin and MDF.
Coconut stearin had a melting point (MP) of 42.23°C, and MDF had an average MP higher than coconut stearin, namely 66.7°C. MAG and DAG generally have higher melting points than TAG, depending on the type of fatty acid in it. The difference in melting points was due to the different number and strength of hydrogen bonds in carboxyl bonds.[Citation21,Citation32]
also showed that the emulsification capacity of coconut stearin was 2.8%, and MDF fat was 94.76%. This indicated that the emulsification capacity of MDF was high and was not different from other commercial emulsifiers. An emulsifier has two types of groups, such as hydrophilic and hydrophobic, so it could reduce the surface tension between two kinds of liquids to mixed.[Citation33,Citation34] This was caused by the high MAG and DAG content in MDF. MDF was then used as a substitute for recombined butter, which acted as an emulsifier while also improving functional and physicochemical properties.
Acylglycerol profiles and melting point of butter
The MAG, DAG, and TAG contents in recombined butter can be seen in . The MAG and DAG content in MDF-0% (control) was not detected, while MAG content in MDF-5%, MDF-10%, and MDF-15% butter was not significantly different (p<.05) from each other and significantly different from MDF-20. Meanwhile, the DAG content in all recombined butter treatments was not significantly different (p<.05) from each other. There was an increase in the MAG and DAG content, while a decrease in the TAG content as the increase of MDF. In the acylglycerol profile of MDF, the MAG and DAG content was 55.79% and 34.30%, respectively, so their addition to butter increased the MAG and DAG content but decreased the TAG content.
Table 2. Acylglycerols composition and melting point of recombined butter with the various concentrations of MDF.
also showed that the addition of MDF affected the melting point of the recombined butter. Recombined butter had a melting point ranging from 44.7–50.0°C with an increase in the concentration of MDF. This was caused by increased MAG and DAG content, which have a higher melting point than TAG.[Citation20,Citation35,Citation36] The melting point of recombined butter can also be affected by the fatty acid composition. MDF contained some saturated fatty acids, such as lauric and myristic acid which had a high melting point. The high melting point could affect the texture of recombined butter by increasing the hardness.
Melting profiles
Melting profiles shown by the thermogram of recombined butter with the substitution of MDF at various concentrations can be seen in . Substitution of MDF affected the melting profiles of recombined butter significantly, especially on the number of peaks formed and Tendset, which also interpreted the end point of melting. showed that in MDF-0% (control), only 2 thermogram peaks were formed, whereas recombined butter with MDF substitution had 3 thermogram peaks in all treatments. This was caused by the MDF content which had different types of fatty acids and acylglycerols (MAG and DAG) from coconut stearin, thus forming a new thermogram peak.
also showed that Tendset also shifted at higher temperatures. This showed that recombined butter which was substituted with higher levels of MDF, produced a higher melting point than the control. Butter on MDF-0% (control) started to melt at Tonset of 9.71°C, then melting finished at Tendset of 37.08°C. MDF-5% started to melt at Tonset of 10.44°C, then melting was complete at Tendset of 39.08°C. MDF-10% started to melt at Tonset of 0.75°C, then melting was complete at Tendset of 42.99°C. MDF-15% started to melt at Tonset of 0.31°C, then melting was completed at Tendset of 46.38°C. While the MDF-20% started to melt at Tonset of 0.43°C, then melting was complete at Tendset of 47.70°C. The increase in melting endpoint (Tendset) due to MDF substitution increased the MAG and DAG content in recombined butter. Generally, MAG and DAG can form stable crystal structures with higher melting points than TAG.[Citation37–40] However, a melting point that was too high in recombined butter was less favorable because it could reduce the adhesiveness and spreadability of the butter.
Texture profiles
Texture profiles of recombined butter with the various concentrations of MDF can be seen in . Based on , it can be seen that the hardness for MDF-0% (control) was not significantly different with MDF-5%. In contrast, the hardness for MDF concentrations of 10% and above was a significant difference (p<.05). The adhesiveness for MDF-0% (control) were not significantly different with MDF-5%, MDF-10%, and MDF-15%, but significantly different with MDF-20% (p<.05). Meanwhile, the cohesiveness did not differ significantly between all treatments. The higher the concentration of MDF substituted, the higher the hardness of the butter. This was due to the MDF having a higher melting point than TAG, so their addition affected the hardness of the butter. In addition, an increase in the fat content of MAG and DAG can accelerate the crystal formation process, resulting in a denser texture.[Citation41–43]
Table 3. Texture profiles of recombined butter with the various concentrations of MDF.
The substitution of MDF up to 15% did not affect the adhesiveness of the butter, while the substitution of MDF up to 20% decreased the adhesiveness and made it less adhesive. This was due to the MDF-20% having a denser texture, as shown in , that the hardness value of MDF-20% was higher than other recombined butter. The MAG and DAG could accelerate the formation of crystals, reducing butter’s stickiness.[Citation41,Citation42] Therefore, the greater MAG and DAG resulted in more and more crystals forming so that the texture became denser and the stickiness of the butter to the probe decreased, and vice versa.
also showed that the substitution of MDF had no significant effect (p<.05) on cohesiveness for all treatments. This indicated that the substitution of MDF as an emulsifier did not significantly affect the cohesiveness of the butter produced, so it can be said that MDF’s emulsification capacity was good and created mixed systems with homogeneous compactness. MDF contained hydrophilic and hydrophobic groups so they could form stable and homogeneous emulsions.[Citation44,Citation45]
Crystal microstructure
The microstructure of milk fat-based products could affect their physicochemical properties. The manufacturing process and chemical content affected the polymorphism of crystals in butter and the number and size of fat crystals, which were related to hardness.[Citation4] The microstructure of butter was complex due to the network of fat crystals interrupted by fat globules and water droplets dispersed in the liquid fat phase.[Citation46] The microstructure of recombined butter with the various concentrations of MDF can be seen in .
Figure 2. Microstructure of recombined butter with the various concentrations of MDF at magnifications of 10x and 40x.

Based on , it can be seen that only a few crystals formed in the control butter (MDF-0%). Meanwhile, in recombined butter, more crystals were formed as the concentration of MDF increased. The most abundant crystals were in MDF-15% and MDF-20%, indicating that crystal formation occurred the fastest in both samples. Overall, the MDF-0% differed significantly from the recombined butter. MDF substitution affected the microstructure of recombined butter. Butter with MDF substitution had a small spherulite, clustered, and many crystals but did not form needlelike crystals, so the crystals formed were soft and did not form a sandy texture.
MAG and DAG could accelerate fat crystallization and produce a denser texture.[Citation42,Citation43] In addition, the use of MAG and DAG resulted in the formation of greater quantities of small crystal nuclei. The crystals from butter produced colors from the reflection of polarized light. Therefore, the more use of MDF, the more small crystals were formed and the resulting color spectrum. In accordance with the results of the microstructural analysis, crystal formation occurred faster when MAG and DAG were added with a large number of crystals.[Citation47,Citation48]
Sensorial properties
Sensory evaluation of recombined butter was carried out for color, aroma, taste, spreadability, and overall preference. The sensorial properties of recombined butter from various concentrations of MDF can be seen in . Based on , it can be seen that the substitution of MDF affected the panelists’ preference level. The concentration of MDF substitution above 10% decreased the preference level for all sensory attributes of recombined butter.
Table 4. Sensorial properties of recombined butter from various concentrations of MDF.
showed that color preference for MDF-0% (control) and MDF-5% were not significantly different from each other, but significantly different (p<.05) with other recombined butter. In this case, it was known that panelists liked the color of MDF-5% with a score of 4.10 and could not distinguish the effect of substitution by MDF. There was an unevenness in the butter color, which was thought to be due to the MDF having a white color, so the use of MDF at high concentrations produced a paler butter color.
also showed that the level of panelists’ preference for the taste of recombined butter decreased significantly (p<.05) along with the increasing concentration of MDF. The MDF substitution gave less taste to the butter, so the preference of panelists decreased. The less taste was suspected because MDF was produced from coconut stearin, which did not taste as good as milk fat, so the more substitution of MDF, the less savory taste of milk fat.[Citation49] The MDF substitution did not affect the level of preference for the aroma of MDF-5% with a score of about 3.65, but the concentration of MDF substitution above 10% decreased the level of panelists’ preference significantly (p<.05). Panelists liked the recombined butter with a distinctive aroma of milk.[Citation5,Citation6] The density of the milk aroma in recombined butter decreased with increasing concentrations of MDF substitutions, so this decreased the panelists’ preference level.
Spreadability is an important parameter for butter. showed that MDF-0% was not significantly different from MDF-5%, with a score of about 4.35, while the concentration of MDF substitution above 10% affected the spreadability of butter. The spreadability test was carried out using bread as a medium by applying butter to the bread. In determining the spreadability of butter, hardness was the main parameter and had a major effect on consumer preference.[Citation5,Citation50] The panelists’ preference level decreased with the increasing concentration of MDF, as well as the hardness value of the recombined butter, which increased with the increasing concentration of MDF so that the resulting texture was more plastic.[Citation49,Citation51] The high plasticity of butter made it difficult to spread due to the uneven distribution of fat.
Overall, MDF-5% had no significant effect on overall acceptability, while concentrations of MDF above 10% had a significant effect (p<.05) by reducing the overall preference level. This was due to the differences in all parameters, so the panelists were less favored. Based on the sensory evaluation results, the recombined butter of MDF-5% was the best treatment that the panelists liked. The MDF-5% was further tested for polymorphism, functional groups, and fatty acid composition compared to MDF-0% as a control.
Polymorphism
Polymorphism determines the quality of fat-based products. Based on , MDF-5% had a polymorphic profile with the highest diffraction peak value for short spacing of d = 4.5Å at 2θ of 19.96 deg, which indicated the presence of β-crystals where this type of crystal has high stability. In addition, the diffraction peaks at a short spacing of d = 4.3Å at 2θ of 20.85 deg; d = 4.2Å, 2θ = 21.69 deg; and d = 3.8Å, 2θ = 23.32 deg, that indicated the presence of β’ crystals. The β’ crystal has a crystal structure that was favored by milk fat-based products, such as butter, because it produced the desired sensory properties, such as a soft texture with good plasticity. Fat crystal polymorphism refers to the ability of acylglycerols to arrange different crystal structures.[Citation52] Various acylglycerols in recombined butter were found to produce different polymorphic forms due to the wide distribution of chain lengths, types of acylglycerol (MAG, DAG, and TAG), and different degrees of unsaturation of the fatty acids.[Citation53,Citation54] A mixture of β’ and β crystals in recombined butter could produce butter with a stable crystal shape and a soft texture. In MDF-5%, there was also present crystal formation at the 2θ of 12.50 deg, whereas this peak was not found in MDF-0%. These crystals were suspected as transition crystals because of the presence of MDF, which was easier to crystallize than TAG.
Functional groups
The FTIR spectra of butter MDF-0% (control) and recombined butter substituted with MDF-5% can be seen in . Based on , MDF-0% showed the formation of peaks at wave numbers 2924.71 cm−1 and 2853.93 cm−1, which interpreted the presence of C-H and O-H groups. The C-H group of alkanes provided absorption at wave numbers 3000–2700 cm−1, and the O-H group of carboxylic acids provided absorption at wave numbers 3300–2500 cm−1 [Citation55]. The appearance of this functional group was due to the butter containing various types of carboxylic fatty acids, such as palmitic, oleic, and stearic acids. Apart from that, other peaks were also formed at the wave number 1746.10 cm−1, which indicated the presence of the C = O group, and at the wave number 1112.31–1237.89 cm−1, one of which came from the C-O group. The C-O bond varies greatly in the absorption area, depending on the type of compound. In the FTIR spectrum graph, the absorption area at waves 1500–500 cm−1 was called the fingerprint area. This area usually produces a complex form of absorbance due to the absorption of all types of molecular bending vibrations.[Citation56,Citation57]
also showed that in MDF-5%, there was the formation of peaks at wave numbers 2924.06 cm−1 and 2853.31 cm−1, which indicated the presence of C-H and O-H groups. Apart from that, other peaks were also formed at wave number 1745.17 cm−1, which indicated the presence of the C = O group, and at wave number 1105.51–1239.08 cm−1, one of which came from the C-O group. In MDF-5%, there was a peak formation at wave number 3422.98 cm−1, which indicated the presence of an O-H group from alcohol where the peak intensity was higher than in MDF-0%. The high intensity of this functional group was due to the presence of MAGs and DAGs in coconut stearin resulting from glycerolysis on MDF-5%, where there were more O-H groups in MAGs and DAGs than TAGs.
Fatty acid composition
The fatty acid composition of MDF-0% (control) and recombined butter substituted with MDF-5% can be seen in . Based on , there were changes in the fatty acid composition, especially an increase in the content of medium-chain fatty acids (MCFAs) on recombined butter MDF-5% compared to MDF-0% (control). Caprylic acid (C8:0) increased from 0.64% to 0.82%, capric acid (C10:0) increased from 2.36% to 2.60%, while lauric acid rose from 4.45% to 7.30%. Changes in fatty acids also occurred in unsaturated fatty acids, namely oleic acid (C18:1), which decreased from 30.70% to 27.30%. This was caused by the substitution of MDF, which came from coconut stearin, which was rich in MCFAs, especially lauric acid (C12:0). These changes in fatty acid composition contributed to physicochemical properties, such as increasing melting point, hardness, crystal microstructure, and decreasing spreadability. However, increasing the MCFA content in recombined butter had a positive impact because MCFAs were very good for health.[Citation58] Therefore, recombined butter has the potential to be healthier than conventional butter. Apart from potential health benefits, recombined butter also had the potential to have market viability and broader implications because it could meet consumer needs for better and healthier butter.
Table 5. Fatty acid composition of butter MDF-0% (control) and recombined butter (MDF-5%).
Conclusion
Substitution of MAG-DAG fat (MDF) affected the characteristics of recombined butter by increasing the MAG and DAG content, melting point, and hardness, but could decrease the adhesiveness and sensorial properties. Therefore, MDF substitution in butter must be limited to a maximum of 5%. Recombined butter substituting MDF-5% was acceptable to the panelists, with an average overall preference score of 3.80, and did not differ significantly from the MDF-0% (control). MDF-5% had MAG, DAG, and TAG content of 0.48%, 8.71%, and 90.81%, respectively. It had a higher MCFA content, namely 10.72%, compared to the control, which was only 7.45%. Small crystals were formed in large numbers, no needlelike crystals were formed, and there was a mixture of β-crystals and β’-crystals. Substitution of MDF could improve the physicochemical properties of recombined butter and had the potential to better health effects. It was recommended that in the future various applications of MDF continue to be developed in various other emulsion-based products such as chocolate, confectionary, shortening, and oleogel to improve its characteristics and health benefits.
Acknowledgement
The authors gratefully acknowledge Universitas Padjadjaran and The Ministry of Education, Culture, Research, and Technology of the Republic of Indonesia.
Disclosure statement
No potential conflict of interest was reported by the author(s).
Additional information
Funding
References
- Panchal, B.; Truong, T.; Prakash, S.; Bansal, N.; Bhandari, B. Influence of Emulsifiers and Dairy Ingredients on Manufacturing, Microstructure, and Physical Properties of Butter. Foods. 2021, 10(5), 1140. DOI: 10.3390/foods10051140.
- Dias, R. S.; Balthazar, C. F.; Cavalcanti, R. N.; Sobral, L. A.; Rodrigues, J. F.; Neto, R. P. C.; Tavares, M. I. B.; Ribeiro, A. P. B.; Grimaldi, R.; Sant’Anna, C., et al., Nutritional, Rheological and Sensory Properties of Butter Processed with Different Mixtures of Cow and Sheep Milk Cream, Food Biosci. 2022, 46, pp. 101564. DOI: 10.1016/j.fbio.2022.101564
- Hyde, P. N.; Sapper, T. N.; LaFountain, R. A.; Kackley, M. L.; Buga, A.; Fell, B.; Crabtree, C.; Phinney, S.; Miller, V.; King, S., et al. Effects of Palm Stearin versus Butter in the Context of Low-Carbohydrate/high-Fat and High-Carbohydrate/low-Fat Diets on Circulating Lipids in a Controlled Feeding Study in Healthy Humans. Nutrients. 2021, 13(6), 1944.
- Rønholt, S.; Mortensen, K.; Knudsen, J. C. The Effective Factors on the Structure of Butter and Other Milk Fat-Based Products. Compr. Rev. Food Sci. Food Saf. 2013, 12(5), 468–482. DOI: 10.1111/1541-4337.12022.
- Ziarno, M.; Derewiaka, D.; Florowska, A.; Szymańska, I. Comparison of the Spreadability of Butter and Butter Substitutes. Appl. Sci. 2023, 13(4), 2600. DOI: 10.3390/app13042600.
- Subroto, E.; Tensiska, T.; Indiarto, R.; Marta, H.; Wulan, A. S., Physicochemical and Sensorial Properties of Recombined Butter Produced from Milk Fat and Fish Oil Blend, Biosci. Res 2018, 15, pp. 3733–3740.
- Fatouh, A. E. M. Physical, Textural and Sensory Characteristics of Cold-Spreadable Recombined Butter Fortified with Chemically Interesterified Gamma-Linolenic Acid. J. Food Dairy Sci 2009, 34(6), 6467–6475. DOI: 10.21608/jfds.2009.115336.
- Viriato, R. L. S.; de, M.; Queirós, S.; Neves, M. I. L.; Ribeiro, A. P. B., Improvement in the Functionality of Spreads Based on Milk Fat by the Addition of Low Melting Triacylglycerols, Food. Res. Int 2019, 120, pp. 432–440. DOI: 10.1016/j.foodres.2018.10.082
- Siri-Tarino, P. W.; Sun, Q.; Hu, F. B.; Krauss, R. M. Saturated Fatty Acids and Risk of Coronary Heart Disease: Modulation by Replacement Nutrients. Curr. Atheroscler. Rep 2010, 12(6), 384–390. DOI: 10.1007/s11883-010-0131-6.
- Astrup, A.; Magkos, F.; Bier, D. M.; Brenna, J. T.; de Oliveira Otto, M. C.; Hill, J. O.; King, J. C.; Mente, A.; Ordovas, J. M.; Volek, J. S., et al., Saturated Fats and Health: A Reassessment and Proposal for Food-Based Recommendations: JACC State-Of-The-Art Rev. J. Am. Coll. Cardiol 2020, 76(7), pp. 844–857. DOI: 10.1016/j.jacc.2020.05.077
- Li, Y.; Hruby, A.; Bernstein, A. M.; Ley, S. H.; Wang, D. D.; Chiuve, S. E.; Sampson, L.; Rexrode, K. M.; Rimm, E. B.; Willett, W. C., et al., Saturated Fats Compared with Unsaturated Fats and Sources of Carbohydrates in Relation to Risk of Coronary Heart Disease: A Prospective Cohort Study, J. Am. Coll. Cardiol 2015, 66 (14), pp. 1538–1548. DOI: 10.1016/j.jacc.2015.07.055
- Masmeijer, C.; Rogge, T.; van Leenen, K.; De Cremer, L.; Deprez, P.; Cox, E.; Devriendt, B.; Pardon, B., Effects of Glycerol-Esters of Saturated Short- and Medium Chain Fatty Acids on Immune, Health and Growth Variables in Veal Calves, Prev. Vet. Med 2020, 178, pp. 104983. DOI: 10.1016/j.prevetmed.2020.104983
- Zhang, M. S.; Sandouk, A.; Houtman, J. C. D., Glycerol Monolaurate (GML) Inhibits Human T Cell Signaling and Function by Disrupting Lipid Dynamics, Sci. Rep 2016, 6(1), pp. 1–13. DOI: 10.1038/srep30225
- Subroto, E.; Indiarto, R. Bioactive Monolaurin as an Antimicrobial and Its Potential to Improve the Immune System and Against COVID-19: A Review. Food Res. 2020, 4(6), 2355–2365. DOI: 10.26656/fr.2017.4(6).324.
- Lieberman, S.; Enig, M. G.; Preuss, H. G., A Review of Monolaurin and Lauric Acid: Natural Virucidal and Bactericidal Agents, Altern. Comp. Ther 2006, 12(6), pp. 310–314. DOI: 10.1089/act.2006.12.310
- Liu, X.; Xu, W.; Wang, W.; Luo, R.; Yang, B.; Lan, D.; Wang, Y., Physicochemical Properties and Feasibility of Coconut Oil-Based Diacylglycerol As an Alternative Fat for Healthy Non-Dairy Creamer, Food Chem. 2023, 19, pp. 100749. DOI: 10.1016/j.fochx.2023.100749
- Khatoon, S.; Khan, M. I.; Jeyarani, T. Enzymatic Interesterification of Palm and Coconut Stearin Blends. Int. J. Food Sci. Technol 2012, 47(11), 2259–2265. DOI: 10.1111/j.1365-2621.2012.03096.x.
- Chen, B.; Mcclements, D. J.; Decker, E. A. Impact of Diacylglycerol and Monoacylglycerol on the Physical and Chemical Properties of Stripped Soybean Oil. Food Chem. 2014, 142, 365–372. DOI: 10.1016/j.foodchem.2013.07.070.
- Feltes, M. M. C.; de Oliveira, D.; Block, J. M.; Ninow, J. L., The Production, Benefits, and Applications of Monoacylglycerols and Diacylglycerols of Nutritional Interest, Food Bioprocess. Technol. 2013, 6 (1), pp. 17–35. DOI: 10.1007/s11947-012-0836-3
- Subroto, E. Monoacylglycerols and Diacylglycerols for Fat-Based Food Products: A Review. Food Res. 2020, 4(4), 932–943. DOI: 10.26656/fr.2017.4(4).398.
- Lo, S.-K.; Tan, C.-P.; Long, K.; Yusoff, M. S. A.; Lai, O.-M., Diacylglycerol Oil—Properties, Processes and Products: A Review, Food Bioprocess. Technol. 2008, 1 (3), pp. 223–233. DOI: 10.1007/s11947-007-0049-3
- Subroto, E.; Wisamputri, M. F.; Supriyanto, T. U.; Hidayat, C.; Hidayat, C., Enzymatic and Chemical Synthesis of High Mono- and Diacylglycerol from Palm Stearin and Olein Blend at Different Type of Reactor Stirrers, J. Saudi Soc. Agric. Sci 2020, 19(1), pp. 31–36. DOI: 10.1016/j.jssas.2018.05.003
- Esperón-Rojas, A. A.; Torres-Palacios, C.; Santos-Luna, D.; Baeza-Jiménez, R.; Cano-Sarmiento, C.; García, H. S. A Specific Thin Layer Chromatography Method for the Identification and Separation of Medium Chain Acylglycerols. J. Oleo. Sci 2018, 67(11), 1397–1403. DOI: 10.5650/jos.ess18081.
- Fuchs, B.; Süß, R.; Teuber, K.; Eibisch, M.; Schiller, J., Lipid Analysis by Thin-Layer Chromatography—A Review of the Current State, J. Chromatogr. A. 2011, 1218(19), pp. 2754–2774. DOI: 10.1016/j.chroma.2010.11.066
- Cano-Medina, A.; Jiménez-Islas, H.; Dendooven, L.; Herrera, R. P.; González-Alatorre, G.; Escamilla-Silva, E. M. Emulsifying and Foaming Capacity and Emulsion and Foam Stability of Sesame Protein Concentrates. Food. Res. Int 2011, 44(3), 684–692. DOI: 10.1016/j.foodres.2010.12.015.
- Subroto, E.; Indiarto, R.; Pangawikan, A. D.; Lembong, E.; Wulandari, E.; Selica, A.; Hartomo, J. I., Melting Behaviour of Cocoa Butter Substitutes Synthesised by Enzymatic Glycerolysis of Coconut Oil and Palm Stearin Blends, Food Res. 2023, 7 (3), pp. 194–202. DOI: 10.26656/fr.2017.7(3).872
- Campos, R.; Ollivon, M.; Marangoni, A. G. Molecular Composition Dynamics and Structure of Cocoa Butter. Cryst. Growth Des. 2010, 10(1), 205–217. DOI: 10.1021/cg900853e.
- Kinta, Y.; Hartel, R. W., Bloom Formation on Poorly-Tempered Chocolate and Effects of Seed Addition, J. Am. Oil Chem. Soc 2009 871(87), pp. 19–27. DOI: 10.1007/s11746-009-1473-5
- Le Révérend, B. J. D.; Fryer, P. J.; Coles, S.; Bakalis, S., A Method to Qualify and Quantify the Crystalline State of Cocoa Butter in Industrial Chocolate, J. Am. Oil Chem. Soc 2010, 87(3), pp. 239–246. DOI: 10.1007/s11746-009-1498-9
- AOCS. Official Methods and Recommended Practices of the American Oil Chemists’ Society, Champaign: American Oil Chemist’s Society Press, 2004.
- Phuah, E. T.; Tang, T. K.; Lee, Y. Y.; Choong, T. S. Y.; Tan, C. P.; Lai, O. M., Review on the Current State of Diacylglycerol Production Using Enzymatic Approach, Food Bioprocess. Technol. 2015, 8(6), pp. 1169–1186. DOI: 10.1007/s11947-015-1505-0
- Subroto, E.; Indiarto, R.; Pangawikan, A. D.; Lembong, E.; Hadiyanti, R., Types and Concentrations of Catalysts in Chemical Glycerolysis for the Production of Monoacylglycerols and Diacylglycerols, Adv. Sci. Technol. Eng. Syst. J 2021, 6(1), pp. 612–618. DOI: 10.25046/aj060166
- Luo, X.; Zhou, Y.; Bai, L.; Liu, F.; Zhang, R.; Zhang, Z.; Zheng, B.; Deng, Y.; McClements, D. J., Production of Highly Concentrated Oil-In-Water Emulsions Using Dual-Channel Microfluidization: Use of Individual and Mixed Natural Emulsifiers (Saponin and Lecithin), Food. Res. Int 2017, 96, pp. 103–112. DOI: 10.1016/j.foodres.2017.03.013
- Ricardo, F.; Pradilla, D.; Cruz, J. C.; Alvarez, O. Emerging Emulsifiers: Conceptual Basis for the Identification and Rational Design of Peptides with Surface Activity. Int. J. Mol. Sci. 2021, 22(9), 4615. DOI: 10.3390/ijms22094615.
- Sari, D. A.; Ningsih, D. S.; Nusantoro, B. P.; Setyaningsih, W.; Hidayat, C. Glycerolysis-Interesterification of Chicken-Palm Stearin Blend: Effect of Solvent and Chicken Stearin to Palm Stearin Ratio. IOP Conf. Ser. Earth Environ. Sci 2019, 260(1), 12094. DOI: 10.1088/1755-1315/260/1/012094.
- Latip, R. A.; Lee, Y. Y.; Tang, T. K.; Phuah, E. T.; Tan, C. P.; Lai, O. M. Physicochemical Properties and Crystallisation Behaviour of Bakery Shortening Produced from Stearin Fraction of Palm-Based Diacyglycerol Blended with Various Vegetable Oils. Food Chem 2013, 141(4), 3938–3946. DOI: 10.1016/j.foodchem.2013.05.114.
- da Silva, R. C.; Soares, F. A. S. D. M.; Maruyama, J. M.; Dagostinho, N. R.; Silva, Y. A.; Ract, J. N. R.; Gioielli, L. A., Crystallisation of Monoacylglycerols and Triacylglycerols at Different Proportions: Kinetics and Structure, Int. J. Food. Prop 2017, 20(sup1), pp. S385–S398. DOI: 10.1080/10942912.2017.1297950
- Seniorita, L.; Minami, E.; Kawamoto, H. Solid–Liquid Phase Behaviors of Binary Mixtures of Various Partial Acylglycerols by Differential Scanning Calorimetry. Eur. J. Lipid Sci. Technol 2022, 124(1), 2100092. DOI: 10.1002/ejlt.202100092.
- Saberi, A. H.; Kee, B. B.; Oi-Ming, L.; Miskandar, M. S. Physico-Chemical Properties of Various Palm-Based Diacylglycerol Oils in Comparison with Their Corresponding Palm-Based Oils. Food Chem. 2011, 127(3), 1031–1038. DOI: 10.1016/j.foodchem.2011.01.076.
- Saberi, A. H.; Lai, O. M.; Miskandar, M. S. Melting and Solidification Properties of Palm-Based Diacylglycerol, Palm Kernel Olein, and Sunflower Oil in the Preparation of Palm-Based Diacylglycerol-Enriched Soft Tub Margarine. Food Bioprocess Technol. 2012, 5(5), 1674–1685. DOI: 10.1007/s11947-010-0475-5.
- Zhang, Y.; Xu, J.; Tang, C.; Li, Y. Crystallization Behavior and Physical Properties of Monoglycerides-Based Oleogels as Function of Oleogelator Concentration. Foods 2023, 12(2), 345. DOI: 10.3390/foods12020345.
- Saberi, A. H.; Chin-Ping, T.; Oi-Ming, L., Phase Behavior of Palm Oil in Blends with Palm-Based Diacylglycerol, J. Am. Oil Chem. Soc 2011, 88(12), pp. 1857–1865. DOI: 10.1007/s11746-011-1860-6
- Basso, R. C.; Ribeiro, A. P. B.; Masuchi, M. H.; Gioielli, L. A.; Gonçalves, L. A. G.; dos Santos, A. O.; Cardoso, L. P.; Grimaldi, R., Tripalmitin and Monoacylglycerols As Modifiers in the Crystallisation of Palm Oil, Food Chem. 2010, 122(4), pp. 1185–1192. DOI: 10.1016/j.foodchem.2010.03.113
- Devi, B. L. A. P.; Zhang, H.; Damstrup, M. L.; Guo, Z.; Zhang, L.; Lue, B. M.; Xu, X., Enzymatic Synthesis of Designer Lipids, OCL - Ol. Corps Gras Lipides 2003, 15(3), pp. 189–195. DOI: 10.1051/ocl.2008.0194
- Zhong, N.; Li, L.; Xu, X.; Cheong, L. Z.; Xu, Z.; Li, B. High Yield of Monoacylglycerols Production Through Low-Temperature Chemical and Enzymatic Glycerolysis. Eur. J. Lipid Sci. Technol 2013, 115(6), 684–690. DOI: 10.1002/ejlt.201200377.
- Rønholt, S.; Buldo, P.; Mortensen, K.; Andersen, U.; Knudsen, J. C.; Wiking, L. The Effect of Butter Grains on Physical Properties of Butter-Like Emulsions. J. Dairy. Sci 2014, 97(4), 1929–1938. DOI: 10.3168/jds.2013-7337.
- Qiu, C.; Wang, S.; Wang, Y.; Lee, W. J.; Fu, J.; Binks, B. P.; Wang, Y., Stabilisation of Oleofoams by Lauric Acid and Its Glycerol Esters, Food Chem. 2022, 386, pp. 132776. DOI: 10.1016/j.foodchem.2022.132776
- Normah, I.; Cheow, C. S.; Chong, C. L., Crystal Habit During Crystallization of RBDPO with the Addition of Diacylglycerol (DAG): Effect of Time and Percentage of DAG Added, Int. Food Res. J 2014, 21, pp. 2045–2049.
- Krause, A. J.; Miracle, R. E.; Sanders, T. H.; Dean, L. L.; Drake, M. A. The Effect of Refrigerated and Frozen Storage on Butter Flavor and Texture. J. Dairy. Sci 2008, 91(2), 455–465. DOI: 10.3168/jds.2007-0717.
- Chudy, S. M.; Cais-Sokolińska, D.; Tomaszewska-Gras, J. Physicochemical Characteristics and Consumers’ Preferences for Milk Fat Products. Appl. Sci. 2022, 12(23), 11986. DOI: 10.3390/app122311986.
- Garvey, E. C.; Sander, T.; O’Callaghan, T. F.; Drake, M.; Fox, S.; O’Sullivan, M. G.; Kerry, J. P.; Kilcawley, K. N., A Cross-Cultural Evaluation of Liking and Perception of Salted Butter Produced from Different Feed Systems, Foods 2020, 9(12), 1767. DOI: 10.3390/foods9121767
- Sato, K. Crystallization Behaviour of Fats and Lipids — a Review. Chem. Eng. Sci 2001, 56(7), 2255–2265. DOI: 10.1016/S0009-2509(00)00458-9.
- Cholakova, D.; Tcholakova, S.; Denkov, N. Polymorphic Phase Transitions in Bulk Triglyceride Mixtures. Cryst. Growth Des 2023, 23(4), 2075–2091. DOI: 10.1021/acs.cgd.2c01021.
- Rønholt, S.; Kirkensgaard, J. J. K.; Pedersen, T. B.; Mortensen, K.; Knudsen, J. C., Polymorphism, Microstructure and Rheology of Butter. Effects of Cream Heat Treatment, Food Chem. 2012, 135(3), pp. 1730–1739. DOI: 10.1016/j.foodchem.2012.05.087
- Jayasheela, K.; Nagabalasubramanian, P. B.; Periandy, S. Conformational & Spectroscopic Characterization, Charge Analysis and Molecular Docking Profiles of Chromone-3-Carboxylic Acid Using a Quantum Hybrid Computational Method. Heliyon 2020, 6(10), e04775. DOI: 10.1016/j.heliyon.2020.e04775.
- Mukai, Y.; Okamoto, R.; Takeuchi, S. Quantum Fourier-transform infrared spectroscopy in the fingerprint region. Opt. Express 2022, 30(13), 22624–22636. DOI: 10.1364/OE.455718.
- Nandiyanto, A. B. D.; Oktiani, R.; Ragadhita, R., How to Read and Interpret Ftir Spectroscope of Organic Material, Indones. J. Sci. Technol 2019, 4(1), pp. 97–118. 1 DOI: 10.17509/ijost.v4i1.15806
- Roopashree, P. G.; Shetty, S. S.; Suchetha Kumari, N. Effect of Medium Chain Fatty Acid in Human Health and Disease. J. Funct. Foods 2021, 87, 104724. DOI: 10.1016/j.jff.2021.104724.