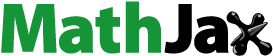
Abstract
Two types of elastomer/plastic compound-modified bitumen were developed by means of incorporating the reactive elastomeric terpolymer (RET) into the plastic (high-density polyethylene HDPE or recycled polyethylene RPE) modified bitumen and adding the wax residue (WR) into the bitumen/elastomer (crumb rubber CR or styrene–butadiene–styrene SBS) blends. The rheological properties, morphology microstructure and storage stability of these novel elastomer/plastic compound-modified binders were characterised. The results revealed that RET elastomer positively improved the high-temperature modulus, temperature insensitivity, rut resistant, elastic recovery and shear-resistance of HDPE- and RPE-modified bitumen. However, excessive RET dosage adversely influenced the cracking resistance of plastic-modified bitumen, and its optimum dosage was recommended as 1 wt%. Moreover, RET elastomer significantly strengthened the storage stability of HDPE and RPE-modified binders. The elasticity improvement effect of RET was attributed to the generated polymer network. On the other hand, adding WR limitedly deteriorated the rutting resistance and weakened the elastic recovery performance of elastomer (CR and SBS) modified bitumen. To ensure the low-temperature performance, the optimum level of WR was 2 wt%. Furthermore, the addition of WR promoted the compatibility and dispersion of CR and SBS modifiers in bitumen.
Introduction
Developing high-performance and sustainable asphalt pavement is one of the main purposes for pavement researchers because of both economic and environmental benefits (Das et al., Citation2012). Nevertheless, the neat bitumen fails to meet the standards regarding high-and-low temperatures performance for sustaining the heavy-loading and variable service conditions (synergistic effects of heat, oxygen, moisture and UV light) (Zhang et al., Citation2019). To this end, a wide variety of organic and inorganic substances were subjoined into the bitumen matrix to achieve the performance enhancement in terms of the rutting, cracking and aging resistance (Merusi & Giuliani, Citation2011).
Polymer series is the first choice to be as the modifiers of bitumen, and they are mainly classified into the plastomer, thermoplastic elastomer (TPE) and reactive polymer (RP) (Pang et al., Citation2016). Moreover, polyethylene (PE) plastics, styrene–butadiene–styrene (SBS) thermoplastic elastomer and crumb rubber (CR) elastomer are commonly utilised as bitumen modifiers owing to their convenient availability, significant efficiency and environmentally-friendly characteristics (Behnood & Gharehveran, Citation2019; Kakar et al., Citation2021). The widespread application of polyethylene-based plastic products increased research interests in PE-modified bitumen, and it was evidenced that the addition of PE-based modifiers regardless of dry and wet ways exhibited an improvement effect on high-temperature rutting and deformation resistance of bitumen (Formela et al., Citation2016; Li, Zheng, et al., Citation2021). Meanwhile, the recycled polyethylene (RPE) from plastics products in daily life was blended in bituminous materials to estimate its modification efficiency (Hesp et al., Citation2002; Huysman et al., Citation2017). It was reported that the RPE-modified bitumen technology showed advantages in cost saving, environment production and bitumen performance enhancement (Duarte & Faxina, Citation2021). Nevertheless, the principal drawbacks of PE-based plastics came from their terrible compatibility with the bitumen matrix and the lack of a polymer network generated, which resulted in a severe phase separation and insufficient elastic performance of PE-modified bitumen (Kakar et al., Citation2021; Tusar et al., Citation2022).
Different approaches have been proposed to address the issues in poor compatibility and elastic performance of polyethylene-modified binders (Domingos et al., Citation2021; Okhotnikova et al., Citation2022; Yu et al., Citation2022). It was illustrated that the molecular characteristics (molecular weight, crystallinity and branched degree) of PE polymer influenced the compatibility and storage stability of PE-modified bitumen, and the branched PE modifier with a lower crystalline and density exhibited a better compatibility (Liang et al., Citation2021). Moreover, Yan et al. (Citation2021) found that the involving of ethylene-vinyl acetate (EVA) promoted the compatibility of PE-modified bitumen accompanied with a specific chemical reaction. Similarly, the trans-polyoctenamer (TPOR) and cross-linking additive (sulphur) was beneficial in enlarging the storage stability and homogenous degree of PE-modified bitumen (Cuadri et al., Citation2016; Padhan et al., Citation2020). Recently, the lightweight oil fraction was involved in minifying the solubility parameter difference and enhancing the compatibility between PE modifiers and bitumen (Ali et al., Citation2022). Hajikarimi et al. (Citation2022) concluded that oil-treated plastics presented a higher compatibility in bitumen. In addition, compound modification technology is popularly adopted, in which various elastomers are blended in PE-based modified bitumen to replenish its elastic components. Numerous studies were performed to explore the synergistic effects of waste plastics and crumb rubber on strengthening the viscoelastic performance of bitumen in line with the significant economic and environmental efficiency (Li, Zheng, et al., Citation2012; Liang, Ren, et al., Citation2020; Wang et al., Citation2015). More sufficient high-temperature rutting and permanent deformation resistance could be achieved with the combination of RPE and CR waste polymers (Ma et al., Citation2022; Navarro et al., Citation2010; Yao et al., Citation2018). Currently, there is no agreed-upon modification mechanism for CR/PE composite modified bitumen between the physical blending with van der Waals force (Zhang et al., Citation2019) and chemo-physical processes (Ge et al., Citation2016).
However, these issues regarding the storage instability and insufficient elastic property of PE-modified binders have not been addressed completely, impeding the considerable utilisation of PE and RPE plastics in bitumen modification dominantly (Soenen et al., Citation2022; Yao et al., Citation2022). From a viewpoint of molecular structures, SBS-modified bitumen performed an excellent performance due to the simultaneous existence of viscous and elastic segments in SBS molecules, but PE-modified bitumen shows insufficient viscoelastic property due to the absence of elastic components in PE molecular structure. Thus, constructing elastic segments in PE-modifier may be an effective way to achieve this goal.
The reactive ethylene terpolymer (RET) polymers are typically on the basis of ethylene, glycidyl-methacrylate (GMA) and an ester group. They show the similar molecular characteristics with PE-based modifiers and chemical reaction capacity to bitumen components (Tauste-Martinez et al., Citation2021). The high reactivity promoted its role as a compatibiliser for different polymeric blends, such as the polyethylene, polyolefins and polyesters (Padhan et al., Citation2019). Rheo-chemical results demonstrated that the RET elastomer could react with the carboxylic groups in asphaltenes and form a polymer network structure, improving the elastic performance (Jiao et al., Citation2019) and storage stability of RET-modified bitumen (Keyf, Citation2018; Prosperi et al., Citation2022). In addition, it was suggested that the incorporation of RET had affirmative influence on permanent deformation and thermo-oxidation aging resistance (Geckil & Seloglu, Citation2018; Irfan et al., Citation2017; Shahane & Bhosale, Citation2021), as well as superior cohesion and adhesion performance to crumb rubber (Xiao et al., Citation2022). Notably, RET played a vital role in improving the compatibility between polymers (SBS (Joohari et al., Citation2022) and HDPE (Gama et al., Citation2018)) and bitumen owing to its high polarity and chemical cross-linking capability.
On the other hand, SBS and CR are widely-utilised elastomers for bitumen modification, but their high viscosity characteristic is a critical issue (Kim et al., Citation2022). High mixing and construction temperatures require high energy and result in a thermal degradation of elastomer polymers (Kumar et al., Citation2021). Various wax mix additives were mixed in SBS and CR-modified bitumen to ameliorate the workability, such as the Sasobit wax, polyethylene wax and chemical-based agent (Mazumder et al., Citation2016). Yue et al. (Citation2021) observed that adding Sasobit wax improved both fatigue performance and healing capacity, thus extending the service life of CR, SBS and SBS/CR composite-modified binders. Moreover, adding a warm-mix agent significantly mitigated the harmful emissions from CR-modified bitumen (Yang et al., Citation2019). However, these warm-mix agents are always commercial products and expensive, which distantly rises the construction cost of asphalt pavement. The wax residue (WR) from refinery chemical plants is a waste by-product of the Fischer–tropsch synthesis (FS) reaction (Li, Li, et al., Citation2021). In the light of its low value, WR materials are generally handled by means of landfills or combustion, which do harm to the environment and ecosystem. In addition, a high wax dosage in WR materials would increase their application possibility as a warm mix additive for elastomer-modified bitumen. Further, our previous work validated that WR could act as a good compatibiliser for remarkably improving the storage stability and microstructure homogeneous dispersion degree of RPE/CR compound modified bitumen (Liang, Sun, et al., Citation2020). Nevertheless, the application flexibility of wax residue on commonly-used elastomer (SBS and CR) modified bitumen has not been explored yet.
It is summarised that the elastic-and-plastic performance balance plays an essential role in comprehensively improving the high-and-low temperatures performance of modified bitumen. To plastic modified bitumen system, the reactive ethylene terpolymer may exhibit a positive function on strengthening the elastic properties and storage stability of plastic (PE and RPE) modified bitumen with the underlying mechanism of chemical reactions and polymer network formation. Similarly, it is also possible to utilise the wax residue waste as a plasticiser (warm-mix agent) to address the issue of unsatisfactory workability for elastomer (SBS and CR) modified bitumen.
Research objectives and methodology
From the perspective of elastoplastic performance improvement, a hypothesis was proposed that the RET elastomer would enhance the elastic properties of plastic (HDPE and RPE) modified bitumen. At the same time, the WR powders would play a role of a warm-mix agent in decreasing the viscosity and improving the workability of elastomer (CR and SBS) modified bitumen. The objective of this paper was to verify this hypothesis and systematically investigate the influence of RET and WR on rheological properties, microscopic morphology, and storage stability of plastic/elastomer-modified bitumen.
Figure illustrates the main research scheme containing two elastomer/plastic compound-modified bitumen. First, the RET elastomer was involved in HDPE- and RPE-modified bitumen to enhance the elastic property. Moreover, the WR powder was incorporated into CR- and SBS-modified binders to enhance their plastic-flow performance. Afterwards, the RET/HDPE, RET/RPE WR/CR and WR/SBS compound-modified binders were manufactured. The influence of RET and WR dosage on the rheological properties, high-temperature rutting resistance, elastic recovery, shearing flow behaviour, low-temperature cracking resistance, morphological microstructure and storage stability of modified bitumen were estimated using dynamic shear rheology (DSR), bending beam rheology (BBR) and fluorescence microscopy (FM) methods.
Materials and characterisation methods
Materials
The 70-PEN grade fresh bitumen was used to prepare elastomer/plastic compound-modified bitumen. The basic properties of virgin bitumen are listed in Table . Moreover, Table shows the physical indicators of HDPE, RPE and RET modifiers. The HDPE and RET polymers were pure products manufactured by the Dupont company. Further, the RPE particles came from Shandong Qilufa Transportation Company, which was a complex blend containing the HDPE substrate and different additives (plasticiser, enhancer, filler, anti-aging agent, etc.) (Wu & Montalvo, Citation2021).
Table 1. The physical and chemical properties of SK-70 virgin bitumen.
Table 2. Basic properties of HDPE, RPE and RET.
In addition, both physical and chemical properties of CR, SBS and WR modifiers are summarised in Table . The CR and SBS utilised in this study were the same as our previous study (Ren, Liu, Li, et al., Citation2020) to ensure the consistency of material resources. The CR powders were manufactured from ambient grinding procedure of waste truck tyres, while SBS copolymer with an S/B ratio of 30/70 and an average molecular weight of 10,000 g/mol was purchased from Yueyang Petrochemical Company. Meanwhile, the wax residue was the by-product during the Fischer–Tropsch synthesis (FTS) processing and was generally composed of the catalyst fine powder and organic wax (Liang, Sun, et al., Citation2020). The appearance of wax residue provided by Shenhua Group Corporation Ltd. was black solid powder.
Table 3. The physicochemical properties of CR, SBS and WR.
Preparation of different compound-modified binders
Table displays the material recipes of various elastomer/plastic compound-modified bitumen. Regarding the RET/HDPE and RET/RPE modified bitumen, the mass concentration of HDPE and RPE was 5 and 7 wt% in line with the literature review (Domingos et al., Citation2021; Tusar et al., Citation2022), which reported that high PE dosage resulted in the worse storage stability and low-temperature cracking performance. Meanwhile, previous studies recommended that the concentration of RET in bitumen should be lower than 3 wt% to balance the high-and-low temperature properties of modified bitumen (Gama et al., Citation2018; Geckil & Seloglu, Citation2018; Xiao et al., Citation2022). In this study, the RET dosage in compound-modified bitumen varied from 0 to 3 wt% with an interval of 1 wt%.
Table 4. The materials compositions of elastomer/plastic compound-modified bitumen.
On the other hand, the mass percentage of crumb rubber and SBS in compound-modified binders were selected as 15 and 3 wt%. To explore the influence of wax residue on the properties of CR- and SBS-modified bitumen, the mutative content of wax residue was 0, 2, 4 and 6 wt%, respectively.
Figure depicts the preparation conditions for various compound-modified binders in Table . Firstly, the virgin and modifier I (HDPE, RPE, CR, or SBS) were mixed using a high-speed shearing machine with a shear rate of 4500 rpm at 160°C for 1 h to guarantee the sufficient dispersion degree. Afterward, the modifier II (RET or WR) was incorporated into the corresponding modified bitumen to prepare the elastomer/plastic compound modified binders using the blender with the stirring speed of 800 rpm at 160°C for 30 min. It should be mentioned that a co-reactant of polyphosphoric acid (PPA) with an amount of 0.2 wt% was blended with RET to accelerate the reaction between the RET modifier and bitumen (Geckil & Seloglu, Citation2018; Xiao et al., Citation2022). Lastly, these modified bitumen samples were poured into the mold for cooling and subsequent testing.
Characterisation methods
DSR tests
The rheological parameters (complex modulus G* and phase angle δ), rutting factor (G*/sinδ), elastic recovery (R%), non-recoverable creep compliance (Jnr) and zero-shear viscosity (η0) were measured by using a strain-controlled DSR device (TA-AR1500EX, America) in accordance with AASHTO T315. Besides, the diameter and gap of parallel plates were 25 and 1 mm, respectively.
The temperature-dependent indices (G*and G*/sinδ) of all binders were counted with a temperature sweep test. The temperature region was 58–84°C with an interval of 6°C, and the loading frequency was 10 rad/s. Moreover, multiple stress creep and recovery (MSCR) and steady-state flow tests were implemented to estimate the elasticity and fluidity of various elastomer/plastic compound-modified bitumen at 60°C. In the MSCR test, the RTFO-aged bitumen sample was subjected to 1s-creep and 9s-recovery procedures at two stress levels of 0.1 and 3.2 kPa. In addition, the flow behaviours of compound binders were characterised using a steady-state shear mode with a shear rate increasing from 10−3–102 rad/s progressively.
BBR tests
On the basis of AASHTO T313, the BBR method was carried out on all RTFO-PAV aged bitumen at −12 and −18°C to probe the effects of RET and wax residue on the low-temperature properties of plastic or elastomer-modified bitumen. The creep stiffness (S) and creep rate (m-value) parameters were measured to assess the bitumen samples’ low-temperature flexibility and cracking resistance. All characterisation tests for each bitumen sample were repeated at least three times to ensure data reliability.
FM observations
The influence of RET and wax residue on the dispersion of polymer modifiers (HDPE, RPE, CR and SBS) in the bitumen matrix was evaluated with a morphological microstructure observed using an FM device (Olympus BX53) with a magnification of 400×.
Storage stability tests
The aluminium tube tests were adopted to characterise the storage stability performance of compound-modified binders. About 50 g bitumen specimen was encased in an aluminium tube and subjected to a thermal storage measurement vertically in an oven at 163°C for 48 h. After a rapid cooling procedure, the tube specimen was equally divided into three parts, and the softening point difference between the first and third segments was tested.
Results and discussion
Influence of RET on properties of plastic-modified bitumen
Temperature sensitivity
The temperature sensitivity curves of the G* parameters of compound bitumen are plotted in Figure . As the temperature increases, the G* of all binders reduces linearly following Equation (1), where the slope value of k refers to the temperature sensitivity of virgin and modified bitumen.
(1)
(1) Compared to virgin bitumen, the absolute slope |k| values of H5 and H7 binders are slightly higher, indicating the incorporation of the HDPE modifier increases the temperature sensitivity of bitumen. In addition, the effect of HDPE dosage on the temperature susceptibility of HDPE-modified bitumen is not significant. It is noticed that the |k| values of RET/HDPE compound-modified binders are all lower than that of HDPE-modified bitumen, which gradually reduces as the increment in RET content. In particular, the |k| value of H7-3 bitumen is smaller than unmodified bitumen. It means that the RET elastomer has a positive effect on undermining the temperature sensitivity of HDPE-modified bitumen owing to the formation of polymer network.
On the contrary, the |k| values of RPE-modified binders are all lower than virgin bitumen, especially when the RPE dosage is 7 wt%. In other word, the influence of RPE on the temperature sensitivity of bitumen is opposite to HDPE, and the involvement of RPE significantly weakens the temperature dependence. It may be associated with the presence of inorganic fillers in RPE materials. Similar to HDPE-modified bitumen, the |k| value of the R5 sample reduces progressively when the RET elastomer was blended. Overall, the RET shows a positive influence on enhancing the thermal stability of PE-based modified bitumen. However, it is worth mentioning that the |k| value of the R7-3 binder is more prominent than the R7 binder, indicating that RET elastomer reversely intensifies the temperature sensitivity of RPE-modified bitumen with high RPE dosage. Hence, the impacts of RET on both complex modulus and the temperature sensitivity of RPE-modified bitumen depend on the RPE dosage dramatically.
Rutting factor and failure temperature
To probe the rutting performance of RET/HDPE and RET/RPE compound-modified bitumen, the variations of rutting factor (G*/sinδ) as a function of temperature are measured and illustrated in Figure . The bitumen with a higher G*/sinδ value would exhibit a better rutting resistance at high temperatures. As expected, the G*/sinδ parameters of virgin and modified binders present a linearly decreasing trend as the temperature gradually increases from 58 to 84°C. It can be explained that a high temperature reduces the intermolecular friction and enhances molecular mobility (Kang et al., Citation2019). That’s why asphalt roads are more susceptible to rutting in the tropics.
According to the SHPR project, rutting failure temperature (RFT) was proposed to assess the rutting potential of a binder directly efficiently. The RFT value is the temperature when the rutting factor G*/sinδ of unaged bitumen reaches 1.0 kPa. Equation (2) is utilised to fit the Lg(G*/sinδ)-T curves, where constants a and c refers to the temperature-dependence and rutting factor at 0°C.
(2)
(2) Table lists the parameters of a, c, RFT and correlation coefficient R2 of all bitumen samples. The R2 values higher than 0.99 suggests that Equation (2) can fit the Lg(G*/sinδ)-T curves well. Additionally, incorporating HDPE and RPE increases the G*/sinδ and RFT values, implying that HDPE and RPE could both enhance the rutting resistance of bitumen. In detail, the RFT values of H5 and H7 samples are 77.11°C and 83.71°C, while R5 and R7 have the RFT value of 81.07°C and 106.6°C. It means that the rutting resistance of RPE-modified bitumen is stronger than HDPE-modified binder, and RPE exhibits a more significant impact on improving the rut resistant performance of bitumen. It may be ascribed to the additional inorganic filler and the aging hardening of RPE modifier (Duarte & Faxina, Citation2021). Regarding the HDPE modified bitumen, as the RET dosage rises from 1% to 3%, the RFT value of H5 binder increases by 2.54, 5.15 and 9.29°C, respectively. In addition, the RFT parameter of H7-3 is 6.35°C higher than H7 sample. It denotes that the RET blending plays a role in enhancing the rutting resistance of HDPE-modified bitumen.
Table 5. The rutting failure temperature of RET/HDPE and RET/RPE modified bitumen.
Similarly, the impact of RET on the rutting properties of RPE-modified bitumen depends on the RPE dosage. The RFT value of the R5 decreases by 2.64°C but increases by 0.55 and 4.37°C when 1%, 2% and 3% RET are blended. Interestingly, the RFT value of R7-3 is 15.63°C lower than R7, indicating that the RET negatively influences the rutting resistance of RPE-modified bitumen with a high RPE content of 7 wt%. It may be explained that RPE with a peculiar and unique chemical structure requires a minimum dosage of RET to create a complete chemical reaction. It is summarised that incorporating RET enhances the rut resistant performance of RPE-modified bitumen but adversely affects the high-temperature performance when the RPE dosage exceeds a particular point.
Recovery percentage and non-recoverable creep compliance
The MSCR test is an efficient way to estimate the high-temperature deformation potential of bituminous materials. The recovery percentage (R%) and non-recoverable creep compliance (Jnr) are calculated using Equations (3) and (4), respectively.
(3)
(3)
(4)
(4) where τ represents the stress level; ϵ1 and ϵ10 are the strain increment of bitumen after the 1s creep and 9s recovery cycles. The R% is strongly associated with the elastic properties, while a higher Jnr value denotes the more considerable permanent deformation sensitivity.
Figure plots the R% and Jnr curves of bitumen binders at 60°C and 3.2 kPa. The R% and Jnr values of fresh bitumen are the minimum and maximum because of its dominant viscous characteristic. Compared to virgin bitumen, the R% parameter of H5, H7, R5 and R7 increases from 0.13% to 2.02%, 10.14%, 11.01% and 45.47%, respectively. Meanwhile, the corresponding Jnr value decreases from 3.47–1.38, 0.40, 0.66 and 0.13 (kPa−1). Overall, both HDPE and RPE improve the elastic properties and reduce the permanent deformation potential of bitumen.
In addition, the RET elastomer increases the R% and reduces the Jnr of HDPE- and RPE-modified bitumen. In detail, compared with the H5 sample, the R% of compound-modified bitumen H5-1, H5-2 and H5-3 increases by 5.17%, 17.19% and 30.26%, while the R% value of H7-3 is 37.62% higher than H7. However, the influence degree of RET on the Jnr parameter is remarkably smaller than R%. To the HDPE-modified bitumen, the incorporation of RET elastomer can enhance its elastic recovery capacity for both cases of 5% and 7% HDPE content.
On the other hand, the R% value of R5-1, R5-2, and R5-3 is 2.89% lower, 4.43%, and 25.45% higher than the R5 binder, while the R% increment of R7-3 is only 0.76% compared to R7. The influence degree of RET on R% and Jnr of RPE-modified bitumen is smaller than HDPE-modified binder. In summary, the RET elastomer can effectively strengthen the elastic properties and deformation resistance of HDPE and RPE-modified bitumen, but its efficacy is significant to the H5, H7 and R5 modified binders. Further, the optimum RET dosage is recommended as 3 wt% when considering the improvement of elastic properties and high-temperature deformation resistance of HDPE (5% and 7%) and RPE (5%) modified binders.
Shear-thinning behaviour and zero-shear viscosity
In the steady-state flow test, the dynamic viscosity values versus shear rate are illustrated in Figure . The Newtonian and non-Newtonian fluids are distinguished based on the shear dependence of viscosity (Ren, Liu, Wang, et al., Citation2020). For a fresh bitumen, the shear-viscosity parameter keeps constant within the shear rate region of 0.01–10 s−1. It means that fresh bitumen presents a Newtonian fluid characteristic. However, when the shear rate exceeds to 10 s−1, the viscosity of fresh bitumen drops down drastically as a non-Newtonian fluid.
From Figure , the Newtonian-fluid ranges of HDPE- and RPE-modified bitumen are more narrow than fresh bitumen, indicating the involvement of HDPE and RPE increases the shear sensitivity of bitumen. The reason may be that the mobility of polymer molecules is remarkably weaker than bitumen components, and the intermolecular friction is stronger (Kang et al., Citation2019). With an increment in HDPE and RPE dosage, the viscosity and non-Newtonian flow characteristics are intensified continuously. Further, the RPE-modified bitumen exhibits higher viscosity, shorter Newtonian-flow region, and better shear resistance performance than HDPE-modified binder.
The RET elastomer significantly affects the shear viscosity and flow behaviours of HDPE- and RPE-modified binders. As the RET content increases, the shear-thinning characteristic of HDPE-modified bitumen is more apparent. It implies that incorporating RET elastomer improves the shear resistance and sensitivity of HDPE-modified bitumen. Besides, the viscosity enhancement of RET approaches HDPE, but the RET elastomer distinctly intensifies the shear-thinning tendency of bitumen than HDPE.
In addition, a high RET dosage increases the shear viscosity and shortens the Newtonian-fluid range, which also demonstrates an inverse influence on the flow behaviour of RPE-modified bitumen with a high RET dosage. The Carreau model (shown in Equation (5)) is adopted to quantitatively describe the flow behaviour of virgin and modified binders, considering the influence of modifier type and dosage.
(5)
(5) where η and γ refer to the measured viscosity and variable shear-rate value, respectively; η0 is the zero-shear viscosity when the shear rate is zero; γc represents the critical shear rate, which behaves as the turning point from Newtonian flow to the non-Newtonian region; s is the constant and high s-value reveals the greater fluctuation level of viscosity to increasing shear-rate (Camargo et al., Citation2021).
The corresponding parameters in Carreau models of all binders are summarised in Table . Both HDPE and RPE exhibit an improvement effect on the shear resistance of bitumen, and the latter is more influential, particularly when the RPE dosage is 7%. It is depicted that the η0 value of HDPE-modified bitumen gradually increases as the RET content rises. For an RPE-modified binder with a 5 wt% RPE dosage, the improvement influence of RET on the η0 value is apparent, although 1% RET presents the opposite effect. The η0 value of R5-1, R5-2 and R5-3 is 0.86, 1.35 and 4.35 times higher than R5, respectively. Thus, the increment effect of RET on the shear-resistance property of HDPE-modified bitumen is more significant than RPE-modified binder. Further, adding 3% RET inversely reduces the viscosity and weakens the shear resistance of RPE-modified bitumen with a high RPE content of 7 wt%. In other words, the positive/negative impact of RET on η0 of the RPE-modified binder depends on the RPE content.
Table 6. The zero-shear viscosity of RET/HDPE and RET/RPE modified bitumen.
Apart from the η0, the involvement of RET elastomer remarkably influences the flow behaviours of HDPE- and RPE-modified bitumen in accordant with the variable γc and s values. The HDPE and RPE play a role in decreasing the γc parameter and increasing the non-Newtonian-fluid region of virgin bitumen. Moreover, the blending of RET elastomer further intensifies the tendency to shear-thinning behaviour of HDPE- and RPE-modified binder. Additionally, the influence of RET on the flow characteristics of modified bitumen is more considerable than HDPE but lower than RPE. The Newtonian-fluid range of R7-3 is broader than R7. It suggests that the shear-thinning potential of the RPE-modified binder with 7% RPE is reduced by adding a 3% RET elastomer. Overall, the impacts of RET on the flow properties of modified bitumen strongly depend on the plastic type and dosage.
Low-temperature cracking resistance
This study evaluated the influence of RET on the low-temperature properties (stiffness S and creep rate m-value) of HDPE- and RPE-modified bitumen. The bitumen with a lower S and a higher m-value exhibits a superior cracking resistance. Figure depicts the BBR results of HDPE-based modified bitumen before and after aging. It is observed that the S and m-value of all fresh bitumen at −12°C meet the specification requirement of 300 MPa and 0.3 (Ren, Liu, Wang, et al., Citation2020), which overstep the expected region at −18°C. Moreover, incorporating 5 and 7 wt% HDPE leads to an increment in stiffness and a reduction in m-value of bitumen, indicating that HDPE modifier adversely affects the bitumen's low-temperature cracking resistance.
The S and m-values of RET/HDPE modified bitumen depend on the RET elastomer dosage. At −12°C, adding 1% RET elastomer results in a 9.8% and 1.6% reduction of S-value of fresh and aged H5 samples, while the corresponding m-value increases by 3.5% and 6.2%, respectively. However, when the RET dosage rises to 2% and 3%, the S and m-value of RTE/HDPE modified binder increases and decreases, respectively. This is related to a large low-temperature cracking potential of H5-3 and H7-3 binders. Therefore, considering the low-temperature properties of RET/HDPE modified bitumen, the optimum dosage of RET is recommended as 1 wt%.
Figure illustrates the low-temperature indices of RPE and RET/RPE modified bitumen. The RPE-modified binder exhibits superior low-temperature cracking resistance to HDPE-modified bitumen. Besides, the stiffness and m-value of RPE-modified bitumen (5% RPE) are lower and larger than that of virgin bitumen. It means the RPE exhibits more advantages in improving the low-temperature properties of bitumen than HDPE. However, the improvement effect tends to decrease as the RPE dosage rises, and even the S and m-value of R7 binder approach to fresh bitumen. As the increment in RET dosage, the stiffness of RET/RPE-modified bitumen decreases and then increases, while the m-value presents the opposite trend. It suggests that the moderate RET content strengthens the low-temperature properties, but excessive RET strongly deteriorates the cracking resistance of RPE-modified bitumen. Overall, when the RPE dosage is 1%, the low-temperature cracking resistance of RET/RPE-modified bitumen is the best.
Compatibility and storage stability
In this study, the FM method is utilised to monitor the scattered or aggregated states of HDPE and RPE modifiers in bitumen before and after incorporating RET elastomer. Figure displays the microstructures of HDPE and RET/HDPE modified binders with different HDPE and RET dosages. It is observed that HDPE particles exist as an island state in HDPE-modified binders (H5 and H7), and the worse dispersion degree of HDPE modifiers is associated with their unsatisfactory compatibility with bitumen (Tusar et al., Citation2022). Interestingly, a significant improvement in the homogenous dispersion degree of the HDPE modifier in bitumen is detected when 1% RET elastomer is blended. As the RET dosage rises to 2% and 3%, the polymer phase in both H5 and H7 samples tends to enlarge gradually, and the polymer network is more noticeable when more RET elastomer is mixed.
It is summarised that adding RET elastomer is beneficial in enhancing the dispersion state of HDPE in bitumen and forming the polymer network structure. Herein, the positive influence of RET on elastic properties, high-temperature shear, rutting and permeant deformation resistance of HDPE-modified bitumen is further validated from the microstructure perspective. Moreover, a high dispersion degree of the polymer phase in RET/HDPE modified bitumen with 1% RET dosage significantly contributes to the enhanced low-temperature cracking resistance. An increment in RET content to 4% and 6% increases the polymer size, and the discontinuous polymer network is generated gradually, which increases the stress concentration/failure points and weakens the low-temperature properties of RET/HDPE compound-modified bitumen.
The morphological microstructures of RPE and RET/RPE modified binders are presented in Figure . In R5 and R7 samples, the RPE modifier is dispersed in bitumen as an irregular flake-strip pattern. Interestingly, compared to the dispersion state of HDPE in bitumen, the dispersion degree of RPE is more prominent, and its polymer phase colour is shallower, indicating the more excellent solubility and compatibility of RPE in the bitumen (Gama et al., Citation2018; Irfan et al., Citation2017). Further, blending 1% RET is beneficial to disperse the RPE modifier more homogenous.
Table lists the softening point difference of RET/HDPE and RET/RPE modified bitumen. As most studies mentioned (Domingos et al., Citation2021; Tusar et al., Citation2022; Yan et al., Citation2021), HDPE and RPE plastics present a terrible storage stability, which worsens with the increase in plastic dosage. The ΔT value of H5, H7, R5 and R7 is 10.5°C, 14.7°C, 9.2°C and 12.5°C, respectively. It suggests that the storage stability of RPE-modified bitumen is better than HDPE-modified binder, which agrees with the microstructure results. As the RET dosage rises, the ΔT values of both HDPE- and RPE-modified binders decrease gradually. In particular, when the RET content is 3 wt%, the ΔT value of plastic-modified bitumen is lower than 5°C. Hence, incorporating RET elastomer improves the storage stability of HDPE and RPE-modified bitumen. The reason may be related to the chemical reactions between RET, plastic (HDPE and RPE) and bitumen, and the formation of a 3D polymer network bitumen (Geckil & Seloglu, Citation2018; Prosperi et al., Citation2022).
Table 7. The softening point difference between RET/HDPE and RET/RPE modified bitumen.
Influence of WR on properties of elastomer-modified bitumen
The second task of this study is to estimate the application potential of wax residue (WR) as a plasticiser on elastomer (CR and SBS) modified bitumen. In this section, the influence of WR on the high-and-low temperature rheological properties and morphological microstructure of CR and SBS-modified bitumen are evaluated and discussed.
Temperature sensitivity
The impacts of WR on the G* values of CR- and SBS-modified bitumen are illustrated in Figure . The fresh bitumen exhibits the largest absolute slope value and strongest temperature sensitivity. The addition of CR and SBS reduces the slope value and weakens the temperature sensitivity of bitumen. Moreover, the temperature sensitivity of CR-modified bitumen is smaller than SBS-modified binder. The incorporation of WR increases the temperature sensitivity of modified binders, especially for the SBS-modified bitumen, which slightly depends on the WR dosage.
Rutting factor and failure temperature
The high-temperature rutting properties of WR/CR and WR/SBS modified binders are also estimated with G*/sinδ and RFT parameters, displayed in Figure and Table . Both CR- and SBS-modified binders exhibit higher G*/sinδ, RFT values, and superior rutting resistance to fresh bitumen, and the former is the best. It is depicted that the involvement of WR reduces the G*/sinδ and RFT values of modified bitumen. When the WR dosage is 2%, 4% and 6%, the RFT value of CR-modified bitumen decreases by 0.10°C, 0.42°C and 0.44°C, while SBS-modified binder shows 0.67°C, 1.52°C and 1.74°C lower RFT value, respectively. Further, it should be mentioned that the influence of WR on deteriorating the rutting resistance of modified bitumen is insignificant.
Table 8. The rutting failure temperature of WR/CR and WR/SBS modified bitumen.
Figure illustrates the R% and Jnr of WR/CR and WR/SBS modified bitumen at 60°C and 3.2 kPa. The incorporation of CR and SBS increases the R% and decreases the Jnr value of bitumen, and the elastic property of bitumen is greatly enhanced by CR and SBS modifiers (Rath et al., Citation2022). Meanwhile, the elastic behaviour of CR-modified bitumen is more significant than the SBS-modified binder based on the higher R% and smaller Jnr values.
It is demonstrated that the R% value of CR- and SBS-modified bitumen decreases distinctly due to the incorporation of WR, while the corresponding Jnr parameter increases. In addition, the MSCR parameters are more sensitive to detecting the WR influence on high-temperature properties than G*/sinδ and RFT indices. When 2%, 4% and 6% WR is mixed, the R% of CR-modified bitumen decreases from 74.28% to 73.86%, 71.51% and 70.92%, while the corresponding Jnr value increases from 0.074–0.080, 0.085 and 0.090 (kPa−1), respectively. On the other side, the R% value of WR/SBS compound modified binder is 8.02%, 31.33% and 34.48% lower than SBS modified bitumen, while the Jnr value is −0.03, 0.29 and 0.37 (kPa−1) higher, respectively. In summary, the involvement of WR displays a weakening effect on the elastic properties of both CR- and SBS-modified bitumen, and the influence on SBS-modified bitumen is more noticeable.
Shear-thinning behaviour and zero-shear viscosity
The steady-state flow tests were utilised to evaluate the influence of WR on the viscosity and flow behaviour of CR- and SBS-modified bitumen. Figure draws the flow curves fitted by the Carreau model to elaborate on the quantitative dependence of viscosity on shear rate. The zero-shear viscosity and other parameters (γc and s) of virgin and modified bitumen are summarised in Table .
Table 9. The zero-shear viscosity of WR/CR and WR/SBS modified bitumen.
Compared to virgin bitumen, the viscosity values of CR- and SBS-modified binders are more significant, and their Newtonian-fluid ranges become shorter. It implies that both CR and SBS lead to the increment of resistance to shear-deformation and a tendency to shear-thinning of bitumen. Besides, WR/CR-modified bitumen exhibits better shear resistance than WR/SBS-modified binder. From Figure , the incorporation of WR slightly reduces the viscosity of CR-modified bitumen, but its similar influence on the SBS-modified binder is more noticeable. At the same time, with the increase of WR dosage, the Newtonian-fluid range of WR/SBS modified binder tends to be more extensive. Hence, it is instructed that adding WR is beneficial in reducing the viscosity and shear sensitivity of modified bitumen.
According to the correlation coefficient R2 values higher than 0.99, the Carreau model can fit all flow curves of virgin and modified bitumen well. The zero-shear viscosity (η0) of virgin bitumen increases from 275.2 to 20498.8 and 2558.1 Pa·s when 15 wt% CR and 3 wt% SBS are blended, respectively. It is worth mentioning that the η0 value of CR- and SBS-modified bitumen decreases progressively as the WR dosage rises. In detail, the 2%, 4% and 6% WR results in the 1.2%, 2.0% and 12.0% reduction of η0 for CR modified bitumen, while η0 value of WR/SBS modified bitumen is 23.9%, 50.9% and 59.6% lower than SBS modified binder. Therefore, the involvement of WR remarkably decreases the viscosity and improves the workability of CR- and SBS-modified bitumen, especially for the latter. According to the γc and s values, the WR shows no obvious influence on the flow behaviour of CR-modified bitumen, but its effect on SBS- modified bitumen should be noticed. As the WR dosage increases, both γc and s values of WR/SBS modified binders are magnified dramatically. It indicates that the WR blending also extends the Newtonian-fluid range and intensifies the shear-thinning behaviour of modified bitumen.
Low-temperature cracking resistance
The BBR tests were utilised to investigate the influence of WR on the low-temperature properties of CR- and SBS-modified bitumen. Figure illustrates the stiffness S and m-value of virgin bitumen, CR, and WR/CR modified binders at two temperature levels of −12°C and −18°C. As the testing temperature decreases, all binders have higher stiffness and lower m-value, related to weakened molecular mobility and smaller free volume (Kang et al., Citation2019). As expected, the low-temperature properties of all bitumen deteriorate after the PAV-aging protocol. Compared to virgin bitumen, the S and m-value of CR-modified bitumen show a significant reduction and increment, indicating that the CR positively influences the bitumen's low-temperature cracking resistance. Furthermore, it is found that the stiffness of CR-modified bitumen declines first and then increases as the WR dosage increases, while the corresponding m-values show the inverse trend.
Moreover, the S and m-value of CR-modified bitumen approach the minimum and maximum point, regardless of the testing temperature and aging degree. In detail, when 2 wt% WR is supplemented, the S-value at −12°C of fresh and aged CR-modified bitumen decreases by 15.1% and 17.7%, while the m-value increases by 0.002 and 0.005, respectively. At −18°C, the S value of fresh and aged WR/CR modified bitumen with 2% WR dosage is 15.4% and 3.3% lower than CR modified binder, while the corresponding m-value is 0.03 and 0.006 larger, respectively. Hence, the incorporation of 2% WR is beneficial for enhancing the low-temperature cracking resistance of CR-modified bitumen.
However, when the WR is more than 2 wt%, the S and m-value of WR/CR modified binder rises and decreases distinctly, which is even higher and lower than virgin bitumen, respectively. It suggests that high WR dosage shows an adverse effect on deteriorating the low-temperature properties of CR-modified bitumen. This phenomenon is firmly ascribed to the high crystallisation potential of a wax component in WR (Li, Zheng, et al., Citation2021). Therefore, the WR dosage should be controlled to ensure the low-temperature performance of CR-modified bitumen, and the optimum level from this study output is 2 wt%.
The influence of WR on the S and m-value of SBS-modified bitumen is shown in Figure . The SBS modifier enhances the low-temperature cracking performance of bitumen, and SBSMB exhibit better cracking resistance than CRMB based on the lower S and larger m-values. It is worth mentioning that the overall impact of WR on the S and m parameters of SBSMB is similar to CRMB. It is denoted that 2% WR positively reduces the S parameter and increases the m-value of SBS-modified bitumen. In comparison, the 4% and 6% WR both remarkably increase the S and decrease the m-value of the WR/SBS modified binder. However, the S and m-value of WR/SBS modified bitumen with a 6 wt% WR dosage is still smaller and larger than that of virgin bitumen. It means that the high WR content would deteriorate the low-temperature property of WR/SBS modified, but it is still greater than pure bitumen. Further, the influence of WR on SBS-modified bitumen is less than the CR-modified binder.
Compatibility and storage stability
The morphological microstructures of WR/CR and WR/SBS modified binders are detected to further understand the underlying modification mechanism of WR on CR and SBS modified bitumen. Figure illustrates the microscopic morphology of WR/CR and WR/SBS modified binders with the WR dosage of 2%, 4% and 6%. It can be found that the crumb rubber phase (black strips) is dispersed as blocks in the bitumen matrix unevenly, while the SBS modifier exists in the form of homogeneous spots. The area of black blocks in CR-modified bitumen is zoomed out as the increase of WR dosage, while the particle size of SBS in bitumen becomes smaller. It is evidenced that the incorporation of WR could promote the dispersion and dissolving of crumb rubber and SBS in bitumen. The involvement of WR supplements the lightweight fractions to bitumen, which are absorbed by CR and SBS polymers and reinforce their compatibility with the bitumen matrix. The microscopic microstructure results further explain why the WR exhibits a significant influence on increasing the viscous component, decreasing the viscosity, and improving the workability as well as long-term aging resistance of CRMB and SBSMB binders.
Table shows the softening point difference of WR/CR and WR/SBS modified binders. Compared to the aforementioned plastic-modified bitumen, the storage stability performance of CRMB and SBSMB are more excellent. The ΔT value of CR- and SBS-modified binder without WR additive is 5.8°C and 3.9°C, respectively. The high ΔT of CR-modified bitumen is related to the incompatibility of crumb rubber powder with bitumen. Moreover, the unsatisfactory storage stability of the SBS-modified binder is mainly due to the lack of cross-linking agent (sulphur). With the increase of WR dosage, the ΔT values of WR/CR and WR/SBS-modified bitumen are down to approximately half-point, indicating that the involvement of the WR agent is beneficial in enhancing the storage stability of CRMB and SBSMB to some extent. This finding is consistent with previous studies (Yue et al., Citation2021), which demonstrated that adding Sasobit warm-mix additive weakened the crystallisation influence and accelerated the swelling degree of CR and SBS modifiers in bitumen. Thus, it raised the potential of fatigue failure resistance and the healing capacity of corresponding modified binders.
Table 10. The softening point difference of WR/CR and WR/SBS modified bitumen.
Conclusions and recommendations
Main findings from this study
In this study, we proposed improving the elasticity of recycled polyethylene-modified bitumen with RET and enhancing the plasticity of CR- or SBS-modified bitumen with WR. The main findings can be drawn as follows:
Incorporating RET elastomer improved the elastic properties of HDPE- and RPE-modified bitumen but showed a softening effect on RPE-modified binder with high RPE dosage.
The rut resistant, elastic recovery, and deformation resistance of HDPE- and RPE-modified bitumen were enhanced by adding RET. Moreover, the RET improved their shear resistance, particularly for HDPE-modified bitumen.
The moderate RET content (1%) enhanced the low-temperature cracking resistance of plastic-modified bitumen. Considering the low-temperature properties of compound-modified binders, the optimum content of RET was recommended as 1 wt%. Moreover, a significant improvement in the storage stability and compatibility between HDPE and RPE plastics with bitumen was detected when elastomer was blended.
The involving WR significantly increased the temperature sensitivity but showed a limited deterioration influence on the rutting resistance of modified binders.
The incorporation of WR weakened the elastic recovery but reduced the viscosity and shear sensitivity of CR- and SBS-modified bitumen. To ensure the low-temperature performance of modified bitumen, the optimum level of WR from this study output was 2 wt%. Meanwhile, the compatibility and dispersion of both CR and SBS particles in bitumen were promoted by adding WR.
Recommendations for future works
There are still lots of meaningful and essential works to be further studied. Firstly, more chemical tests and morphological microstructure should be conducted to further explore the underlying modification mechanism. Secondly, the durability-related fatigue and self-healing performance of these elastomer/plastic compound-modified binders considering the improvement of elastic or plastic properties with the utilisation of household and industrial wastes. Lastly, the asphalt mixtures require further investigation to validate the potential and feasibility of elastomer/plastic compound-modified bitumen incorporating recycled polyethylene, crumb rubber powder and wax residue waste materials.
Disclosure statement
No potential conflict of interest was reported by the author(s).
Additional information
Funding
References
- Ali, B., Soudani, K., & Haddadi, S. (2022). Effect of waste plastic and crumb rubber on the thermal oxidative aging of modified bitumen. Road Materials and Pavement Design, 23(1), 222–233. https://doi.org/10.1080/14680629.2020.1820893
- Behnood, A., & Gharehveran, M. M. (2019). Morphology: Rheology, and physical properties of polymer-modified asphalt binders. European Polymer Journal, 112, 766–791. https://doi.org/10.1016/j.eurpolymj.2018.10.049
- Camargo, I., Dhia, T., Loulizi, A., Hofko, B., & Mirwald, J. (2021). Anti-aging additives: Proposed evaluation process based on literature review. Road Materials and Pavement Design, 22(1), S134–S153. https://doi.org/10.1080/14680629.2021.1906738
- Cuadri, A. A., Roman, C., Garcia-Morales, M., Guisado, F., Moreno, E., & Partal, P. (2016). Formulation and processing of recycled-low-density-polyethylene-modified bitumen emulsions for reduced-temperature asphalt technologies. Chemical Engineering Science, 156, 197–205. https://doi.org/10.1016/j.ces.2016.09.018
- Das, P. K., Tasdemir, Y., & Birgisson, B. (2012). Evaluation of fracture and moisture damage performance of wax modified asphalt mixtures. Road Materials and Pavement Design, 13(1), 142–155. https://doi.org/10.1080/14680629.2011.644120
- Domingos, M., Faxina, A., & Bernucci, L. (2021). Modelling and permanent deformation analysis of low-density polyethylene (PE)-modified bitumens and asphalts. Road Materials and Pavement Design, 22(8), 1860–1880. https://doi.org/10.1080/14680629.2020.1732446
- Duarte, G. M., & Faxina, A. L. (2021). Asphalt concrete mixtures modified with polymeric waste by the wet and dry processes: A literature review. Construction and Building Materials, 312, 125408.
- Formela, K., Sulkowski, M., Saeb, M. R., Colom, X., & Haponiuk, J. T. (2016). Assessment of microstructure,: physical and thermal properties of bitumen modified with LDPE/GTR/elastomer ternary blends. Construction and Building Materials, 106, 160–167. https://doi.org/10.1016/j.conbuildmat.2015.12.108
- Gama, D. A., Yan, Y., Rodrigues, J. K. G., & Roque, R. (2018). Optimizing the use of reactive terpolymer, polyphosphoric acid and high-density polyethylene to achieve asphalt binders with superior performance. Construction and Building Materials, 169, 522–529. https://doi.org/10.1016/j.conbuildmat.2018.02.206
- Ge, D., Yan, K., You, Z., & Xu, H. (2016). Modification mechanism of asphalt binder with waste tire rubber and recycled polyethylene. Construction and Building Materials, 126, 66–76. https://doi.org/10.1016/j.conbuildmat.2016.09.014
- Geckil, T., & Seloglu, M. (2018). Performance properties of asphalt modified with reactive terpolymer. Construction and Building Materials, 173, 262–271. https://doi.org/10.1016/j.conbuildmat.2018.04.036
- Hajikarimi, P., Hosseini, A. S., & Fini, E. H. (2022). Evaluation of the compatibility of waste plastics and bitumen using micromechanical modeling. Construction and Building Materials, 317, 126107.
- Hesp, S. A. M., Hoare, T. R., & Roy, S. D. (2002). Low-temperature fracture in reactive-ethylene-terpolymer-modified asphalt binders. International Journal of Pavement Engineering, 3(3), 153–159. https://doi.org/10.1080/1029843021000067809
- Huysman, S., Schaepmeester, J. D., Ragaert, K., Dewulf, J., & Meester, S. D. (2017). Performance indicators for a circular economy: A case study on post-industrial plastic waste. Resources, Conversation and Recycling, 120, 46–54.
- Irfan, M., Saeed, M., Ahmed, S., & Ali, Y. (2017). Performance evaluation of Elvaloy as a fuel-resistant polymer in asphaltic concrete airfield pavements. Journal of Materials in Civil Engineering, 29(10), 04017163. https://doi.org/10.1061/(ASCE)MT.1943-5533.0002018
- Jiao, Y., Zhang, Y., Fu, L., Guo, M., & Zhang, L. (2019). Influence of crumb rubber and tafpack super on performances of SBS modified porous asphalt mixtures. Road Materials and Pavement Design, 20(1), S196–S216. https://doi.org/10.1080/14680629.2019.1590223
- Joohari, I. B., Maniam, S., & Giustozzi, F. (2022). Enhancing the storage stability of SBS-plastic waste modified bitumen using reactive elastomeric terpolymer. International Journal of Pavement Research and Technology, https://doi.org/10.1007/s42947-021-00132-z
- Kakar, M. R., Mikhailenko, P., Piao, Z., Bueno, M., & Poulikakos, L. (2021). Analysis of waste polyethylene (PE) and its by-products in asphalt binder. Construction and Building Materials, 280, 122492. https://doi.org/10.1016/j.conbuildmat.2021.122492
- Kang, Y., Zhou, D., Wu, Q., Liang, R., Shangguan, S., Liao, Z., & Wei, N. (2019). Molecular dynamics study on the glass forming process of asphalt. Construction and Building Materials, 214, 430–440. https://doi.org/10.1016/j.conbuildmat.2019.04.138
- Keyf, S. (2018). The modification of bitumen with styrene-butadiene-styrene,: ethylene vinyl acetate and varying the amount of reactive ethylene terpolymer. Journal of Elastomers & Plastics, 50(3), 241–255. https://doi.org/10.1177/0095244317708590
- Kim, J., Jang, H., & Kim, N. (2022). Evaluation of potential applicability of modified solvent deasphalted residue as an asphalt crack sealant. Road Materials and Pavement Design, 23(3), 725–734. https://doi.org/10.1080/14680629.2020.1845784
- Kumar, A., Choudhary, R., & Kumar, A. (2021). Characterisation of asphalt binder modified with ethylene-propylene-diene-monomer (EPDM) rubber waste from automobile industry. Road Materials and Pavement Design, 22(9), 2044–2068. https://doi.org/10.1080/14680629.2020.1740772
- Li, L., Zheng, Y., Xu, B., Xu, Y., & Liu, Z. (2021). Wax separated effectively from fischer-tropsch wax residue by solvent desorption: Thermodynamic and kinetic analysis. Applied Sciences, 11(16), 7745. https://doi.org/10.3390/app11167745
- Li, X., Li, J., Wang, J., Yuan, J., Jiang, F., Yu, X., & Xiao, F. (2021). Recent applications and developments of polyurethane materials in pavement engineering. Construction and Building Materials, 304, 124639.
- Liang, M., Ren, S., Sun, C., Zhang, J., Jiang, H., & Yao, Z. (2020). Extruded tire crumb-rubber recycled polyethylene melt blend as asphalt composite additive for enhancing the performance of binder. Journal of Materials in Civil Engineering, 32(3), 04019373. https://doi.org/10.1061/(ASCE)MT.1943-5533.0003044
- Liang, M., Sun, C., Yao, Z., Jiang, H., Zhang, J., & Ren, S. (2020). Utilization of wax residue as compatibilizer for asphalt with ground tire rubber/recycled polyethylene blends. Construction and Building Materials, 230, 116966.
- Liang, M., Xin, X., Fan, W., Zhang, J., Jiang, H., & Yao, Z. (2021). Comparison of rheological properties and compatibility of asphalt modified with various polyethylene. International Journal of Pavement Engineering, 22(1), 11–20. https://doi.org/10.1080/10298436.2019.1575968
- Ma, Y., Wang, S., Zhou, H., Hu, W., Polaczyk, P., & Huang, B. (2022). Recycled polyethylene and crumb rubber composites modified asphalt with improved aging resistance and thermal stability. Journal of Cleaner Production, https://doi.org/10.1016/j.jclepro.2021.130102
- Mazumder, M., Kim, H., & Lee, S. (2016). Performance properties of polymer modified asphalt binders containing wax additives. International Journal of Pavement Research and Technology, 9(2), 128–139. https://doi.org/10.1016/j.ijprt.2016.03.004
- Merusi, F., & Giuliani, F. (2011). Rheological characterization of wax-modified asphalt binders at high service temperatures. Materials and Structures, 44(10), 1809–1820. https://doi.org/10.1617/s11527-011-9739-4
- Navarro, F. J., Partal, P., Martinez-Boza, F. J., & Gallegos, C. (2010). Novel recycled polyethylene/ground tire rubber/bitumen blends for use in roofing applications: Thermo-mechanical properties. Polymer Testing, 29(5), 588–595. https://doi.org/10.1016/j.polymertesting.2010.03.010
- Okhotnikova, E. S., Ganeeva, Y. M., Frolov, I. N., Yusupova, T. N., & Fazylzyanova, G. R. (2022). Structural characterization and application of bitumen modified by recycled polyethylenes. Construction and Building Materials, 316, 126118.
- Ouyang, C., Gao, Q., Shi, Y., & Shan, X. (2012). Compatibilizer in waste tire powder and low-density polyethylene blends and the blends modified asphalt. Journal of Applied Polymer Science, 123(1), 485–492. https://doi.org/10.1002/app.34634
- Padhan, R., Gupta, A., & Sreeram, A. (2019). Effect of cross-linking agent on ethylene vinyl acetate/polyoctenamer modified bitumen. Road Materials and Pavement Design, 20(7), 1615–1623. https://doi.org/10.1080/14680629.2018.1467335
- Padhan, R., Sreeram, A., & Gupta, A. (2020). Evaluation of trans-polyoctenamer and cross-linking agents on the performance of waste polystyrene modified asphalt. Road Materials and Pavement Design, 21(4), 1170–1182. https://doi.org/10.1080/14680629.2018.1533490
- Pang, J., Du, S., Chang, R., & Pei, Q. (2016). The properties of SBS-modified asphalt binder in the presence of dithiodimorpholine and tetraethyl thiuram disulphide. Road Materials and Pavement Design, 17(2), 466–476. https://doi.org/10.1080/14680629.2015.1082928
- Prosperi, E., Bocci, E., & Bocci, M. (2022). Evaluation of the rejuvenating effect of different additives on bituminous mixtures including hot-recycled RA as a function of the production temperature. Road Materials and Pavement Design, 23(12), 2798–2817. https://doi.org/10.1080/14680629.2021.2002179
- Rath, P., Gettu, N., Chen, S., & Buttlar, W. G. (2022). Investigation of cracking mechanisms in rubber-modified asphalt through fracture testing of mastic specimens. Road Materials and Pavement Design, 23(7), 1544–1563. https://doi.org/10.1080/14680629.2021.1905696
- Ren, S., Liu, X., Fan, W., Qian, C., Nan, G., & Erkens, S. (2021). Investigating the effects of waste oil and styrene-butadiene rubber on restoring and improving the viscoelastic,: compatibility, and aging properties of aged asphalt. Construction and Building Materials, 269, 121338 .
- Ren, S., Liu, X., Li, M., Fan, W., Xu, J., & Erkens, S. (2020). Experimental characterization of viscoelastic behaviors,: microstructure and thermal stability of CR/SBS modified asphalt with TOR. Construction and Building Materials, 261, 120524.
- Ren, S., Liu, X., Wang, H., Fan, W., & Erkens, S. (2020). Evaluation of rheological behaviors and anti-aging properties of recycled asphalts using low-viscosity asphalt and polymers. Journal of Cleaner Production, 253, 120048.
- Shahane, H., & Bhosale, S. (2021). E-Waste plastic powder modified bitumen: Rheological properties and performance study of bituminous concrete. Road Materials and Pavement Design, 22(3), 682–702. https://doi.org/10.1080/14680629.2019.1642944
- Soenen, H., Carbonneau, X., Lu, X., Robertus, C., & Tapin, B. (2022). Rheological and chemical properties of field aged binders and their variation within the wearing course. Road Materials and Pavement Design, 23(1), 36–54. https://doi.org/10.1080/14680629.2021.1994450
- Tauste-Martinez, R., Moreno-Navarro, F., Sol-Sanchez, M., & Rubio-Gamez, M. (2021). Multiscale evaluation of the effect of recycled polymers on the long-term performance of bituminous materials. Road Materials and Pavement Design, 22(1), S99–S116. https://doi.org/10.1080/14680629.2021.1906737
- Tusar, M., Kakar, M. R., Poulikakos, L. D., Pasquini, E., Baliello, A., Pasetto, M., Porot, L., Wang, D., Falchetto, A. C., Dalmazzo, D., Presti, D. L., Giancontieri, G., Varveri, A., Veropalumbo, R., Viscione, N., Vasconcelos, K., & Carter, A. (2022). RILEM TC 279 WMR round robin study on waste polyethylene modified bituminous binders: Advantages and challenges. Road Materials and Pavement Design, DOI: 10.1080/14680629.2021.2017330
- Wang, S., Wang, Q., Wu, X., & Zhang, Y. (2015). Asphalt modified by thermoplastic elastomer based on recycled rubber. Construction and Building Materials, 93, 678–684. https://doi.org/10.1016/j.conbuildmat.2015.06.047
- Wu, S., & Montalvo, L. (2021). Repurposing waste plastics into cleaner asphalt pavement materials: A critical literature review. Journal of Cleaner Production, 280(2), 124355. https://doi.org/10.1016/j.jclepro.2020.124355
- Xiao, F., Zong, Q., Wang, J., Chen, J., & Liu, J. (2022). Storage stability characterization and improvement of SBS and crumb rubber composite modified asphalt. Road Materials and Pavement Design, 23(3), 509–526. https://doi.org/10.1080/14680629.2020.1830151
- Yan, K., Hong, Z., You, L., Ou, J., & Miljkovic, M. (2021). Influence of ethylene-vinyl acetate on the performance improvements of low-density polyethylene-modified bitumen. Journal of Cleaner Production, 278, 123865.
- Yang, X., You, Z., Perram, D., Hand, D., Ahmed, Z., Wei, W., & Luo, S. (2019). Emission analysis of recycled tire rubber modified asphalt in hot and warm mix conditions. Journal of Hazardous Materials, 365, 942–951. https://doi.org/10.1016/j.jhazmat.2018.11.080
- Yao, L., Leng, Z., Lan, J., Chen, R., & Jiang, J. (2022). Environmental and economic assessment of collective recycling waste plastic and reclaimed asphalt pavement into pavement construction: A case study in Hong Kong. Journal of Cleaner Production, 336, 130405.
- Yao, Z., Zhang, J., Gao, F., Liu, S., & Yu, T. (2018). Integrated utilization of recycled crumb rubber and polyethylene for enhancing the performance of modified bitumen. Construction and Building Materials, 170, 217–224. https://doi.org/10.1016/j.conbuildmat.2018.03.080
- Yu, L., Lyu, L., Li, R., Du, Y., & Pei, J. (2022). Microscopic mechanism of direct-input waste plastic modified asphalt. J. Transp. Eng. Part B: Pavements, 148(2), 04022003.
- Yue, M., Yue, J., Wang, R., & Xiong, Y. (2021). Evaluating the fatigue characteristics and healing potential of asphalt binder modified with Sasobit and polymers using linear amplitude sweep test. Construction and Building Materials, 289, 123054.
- Zhang, J., Yao, Z., Yu, T., Liu, S., & Jiang, H. (2019). Experimental evaluation of crumb rubber and polyethylene integrated modified asphalt mixture upon related properties. Road Materials and Pavement Design, 20(6), 1413–1428. https://doi.org/10.1080/14680629.2018.1447505
- Zhang, S., Cui, Y., & Wei, W. (2021). Low-temperature characteristics and microstructure of asphalt under complex aging conditions. Construction and Building Materials, 303, 124408.