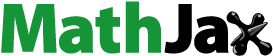
ABSTRACT
The present work focuses on the construction of a counter flow dehumidification test rig where calcium chloride liquid is used as the desiccant and is made to spray on the novel organic packing made of wood shaving with different densities and porosities. The mass flow rate of the air is varied and the performance parameters such as moisture removal rate (MRR), dehumidification effectiveness, coefficient of performance (COP), sensible heat factor (SHR) and mass transfer coefficient have been investigated. Results demonstrated an increase in the relative humidity, mass transfer coefficient and drop in the COP, MRR and dehumidification efficiency with the increase in the flow airflow rate. On increasing the CaCl2 concentration, dehumidification performance parameters such as MRR, COP and mass transfer coefficient are found to be increased. Out of the three different densities tested, 500 kg/m3 shows best performance with MRR, ΔRH and dehumidification effectiveness being 9.7%, 18.7% and 4.9% lower than the Celdek pad when measured at 5 m/s of air velocity for 30% desiccant concentration. Optimisation of the input parameters has been done and the results revealed that air velocity should be maintained within the range of 4–5 m/s to minimise energy consumption and optimum performance.
1. Introduction
The Present AC technology mainly focuses on the improvement of thermal comfort and a healthy environment with the reduction of overall operating costs (Rafique, Gandhidasan, and Bahaidarah Citation2016). It is imperative that a certain range of air humidity is maintained to achieve thermal comfort. According to ASHRAE standards, 30–60% is ideal. Deviation of relative humidity from this range causes discomfort. In the dehumidification technology, desiccant is used to remove moisture from the air. Desiccants are of two types, solid and liquid type. Solid desiccants have a high internal surface area by the unit mass. They are mainly used to maintain dry conditions in humid regions, storing food stuff, pharmaceutical industries, clean room, chemical industries, and maintaining texture and nutrients. Some the examples of solid desiccants are zeolites, activated alumina, silica gel and synthetic polymer (Sampath, Kumar, and Reddy Citation2020). They have a few drawbacks such as limited dew condensation, less dew depression, unreliable and sensitive to varying flow rates. On the other hand, the liquid desiccant dehumidification system works on the principle of absorption of water vapour (Dong et al. Citation2017). Commonly used desiccants are lithium chloride, lithium bromide, sodium chloride, potassium hydroxide, sucrose, etc. They have a few merits such as utilise less heat for regeneration, better efficiency, provide clean air and energy efficient. Conventional air conditioning system causes both cooling and dehumidification. But the system has some limitations such as pollution, higher energy consumption and maintenance. The latent heat of conventional AC systems can be reduced by using liquid desiccant based dehumidification system without having harmful effects on the environment (Abdel-Salam, Ge, and Simonson Citation2013).
When the packing is soaked with desiccant and interacts with air, dehumidification occurs (Wang et al. Citation2016). Dehumidification depends on desiccant type, concentration, packing material and configuration, wettability, flow type and regenerative system (Jain, Tripathi, and Das Citation2011). Types of packing materials which are used in conventional systems are Celdek, polypropylene, polyvinylchloride, aspen, khus, fabric, plastic, polycarbonate, hard paper, etc. Some of the configurations used are honeycomb, corrugated, S Shaped, globular, zig zag, flat porous board, slides and stack type. Celdek packing gave good wettability and stability, it is widely used in most of the dehumidification applications. But the high cost of the Celdek packing has minimised its application in household applications. In addition, difficulty in manufacturing and local unavailability have restricted its use besides higher maintenance (Salins, Reddy, and Kumar Citation2022; Suranjan Salins et al. Citation2022). Hence an alternative packing material that is biodegradable, locally available and cheap is the current need for dehumidification applications. Interaction between the air takes place along parallel, counter and cross flow directions (Seenivasan, Selladurai, and Arjunan Citation2018). After the dehumidification process, the overall concentration of the desiccant is lost and it could be regained by heating to its regeneration temperature using low grade heat such as exhaust heat, extracted heat from the boiler and solar energies (Watanabe et al. Citation2019).
Various researchers have worked on different desiccants, configurations, flow types and regenerative techniques. Wen et al. (Citation2019) used mixed liquid desiccants which reduced corrosion of the system and enhanced dehumidification. Experimental investigation showed that the mixed desiccant showed 15% more dehumidification effectiveness compared to lithium chloride solution. Cho, Cheon, and Jeong (Citation2019) worked on the counter and cross flow liquid dehumidifiers. Dehumidification and enthalpy effectiveness were found to be 82% and 72% for the counterflow, and for the crossflow type they were found to be 63% and 55%. Naik and Muthu Kumar (Citation2019) studied the influence of air humidity ratio on desiccant regeneration and dehumidification performance. A correlation was developed which gave the relationship between humidity ratio and operating parameters. Experimental results were compared with the 2D theoretical model and it was found that the results were in agreement with each other. Chai et al. (Citation2019) used desiccant coating on the heat exchanger to enhance dehumidification and integrated with heat pump for the purpose of regeneration. It was found that moisture removal rate of 9.98 g/kg obtained in high humidity conditions and the COP achieved was 5.36. Ou, Cai, and He (Citation2019) built a mathematical model to predict the output parameters with the consideration of the operating conditions. Results indicated that there was a reduction in energy consumption by 12.49% as compared to that of conventional air conditioning units. Qasem and Zubair (Citation2019) coupled the humidification–dehumidification (HDH) unit with the adsorption desalination (AD) unit. Results indicated that the COP obtained for the system was 0.45. Mohamed et al. (Citation2016) conducted experiments with Gauze structured packing and the type of desiccant used was lithium chloride. Inlet parameters such as desiccant flow rates, desiccant temperatures, concentration and humidity ratio were considered. Results indicated that dehumidification effectiveness were obtained in the range of 0.67–0.87 and the maximum humidity reduction obtained was 16.4 g/kg. Kavasoğulları, Cihan, and Demir (Citation2016) used polycarbonate panels as packing material which provided high surface tension and wettability when liquid desiccants like lithium bromide (Li Br) and lithium chloride (Li-Cl) were used. Also, mass transfer obtained from this type of packing was high. Zegenhagen et al. (Citation2015) conducted experiments on solar air dehumidification unit with the use of ionic desiccant. Wu, Li, and Zhang (Citation2006) worked on the dehumidifier unit consisting of heat pump and heat exchangers. Evaporator coils were utilised for the cooling purpose and to regulate the air at desired conditions to the space. Condenser heat was used for regeneration purpose. It showed that dehumidification efficiency of 70% was obtained which was below the predicted results by 12%. Bouzenada, Frainkin, and Léonard (Citation2017) carried out regression analysis to evaluate vapour pressure of calcium chloride desiccant. Lu et al. (Citation2017) developed a mathematical model to study the performance of the crossflow dehumidification system. Performance parameters were greatly influenced by desiccant to air ratio. Research found that the humidity ratio was the highest responsible factor for the outlet air temperature. Yang, Lian, and Zhang (Citation2017) worked on an ultrasonic atomisation liquid desiccant dehumidifier with a conventional packed bed. COP obtained for the overall system was found to be 0.21. Qi, Zhi, and Zhang (Citation2019) used plastic as a packing material and calculated the wettability when the 40%. Lithium bromide was used as the solution. The above combination gave good dehumidification and the material proved to be durable. Crofoot and Harrison (Citation2012) worked on the counterflow dehumidifier test rig where lithium chloride was used as a desiccant. The system gave a COP of 0.6 and cooling capacity of 18.1 kW. Ronghui and Lin (Citation2014) used solar energy for the regeneration of liquid desiccant. The result showed that there was a saving in energy of 10% obtained compared to the conventional systems.
From the above literature, it is very clear that most of the researchers used a standard Celdek packing as a packing material, which is commercially available and of high cost. Manufacturing of Celdek packing is complex and it is difficult to get in rural areas. Alternatively, wood shaving can also be considered as a packing material for dehumidification. There are several merits of using wood shaving packing, such as free and easily available, easily disposable, biodegradable, flexible to vary the shape, structure and density. Moreover, it has good wettability characteristics which is essential for dehumidification purposes. Very limited studies have been conducted on the wood shaving packing and its different combination with any desiccant. Lithium Bromide and lithium chloride desiccants are widely used in dehumidification systems which corrode the materials on continuous use, also they are toxic in nature. Calcium chloride is one of the potential alternative salt materials that possess good hygroscopic characteristics and hence can also be used as a liquid desiccant. It has potential dehumidification characteristics such as low vapour pressure, odorless, high viscosity, high boiling point, nontoxic and chemically stable liquid moderate regeneration temperature. Literature related to the use of CaCl2 as the desiccant along with wood shaving as a packing material is very limited.
To overcome the above research gaps, the present dehumidification experimental study is carried out by using wood shaving as the pad material and calcium chloride as the liquid desiccant. In order to understand the effect of thickness of wood packing on the performance, experiments are conducted by varying the wood density and also by varying the desiccant concentration. Output performance parameters such as MRR, COP, SHR, mass transfer coefficient and change in the pressure are evaluated, which are then compared with the standard Celdek packing performance. The results have been used to optimise the wood density and operating parameters for maximising the dehumidification performance. Accordingly, the novelty of the work includes:
Due to the increased cost of the Celdek packing and unavailability in local areas, an alternative packing which is economical, cheaply available and having reasonably good performance is required and wood shaving packing having low resistance and is easily available in local areas will fulfil the above demand and is considered to one of the best alternative to Celdek packing for dehumidification applications.
Due to higher contact resistance, Celdek packing is found to be inefficient whereas wood shaving packing with configuration (density = 500 kg/m3) provides maximum contact between air and desiccant with showing reduced pressure drop and comparative performance as that of Celdek packing which is another novelty of the study.
3. Background theory
shows the typical dehumidification process which takes place when the air passes through desiccant soaked pads. The air loses its moisture and gets heated. During dehumidification latent heat is liberated due to the absorption of water vapour by the desiccant from the air which increases the temperature of both air and the desiccant solution. TA and TB are the dry bulb air temperatures and WA and WB represent the specific humidity at the inlet and outlet. Output parameters are determined using the following equations.
Sensible heat ratio (SHR) of the dehumidifier, which is defined as the ratio of sensible heat added to the air supply to the total (sensible and latent) heat energy. It is represented by Equation (1) (Salins, Reddy, and Kumar Citation2022).
(1)
(1) where QS and QL represents the sensible and latent heat, kJ/kg.
Energy consumed (EC) is defined as the total sum of energies spent to operate the system at a mass flow rate. It is given by Equation (2) (Salins, Reddy, and Kumar Citation2021).
(2)
(2) where EC, Wpump, WBlower and WElectric coil represent the power required to operate pump, blower and electrical coil respectively, kW
Coefficient of performance (COP) is defined as the ratio between the average heat transfer to the total primary energy consumption in the LDDC system. It is represented by Equation (3). Heating effect is given by the difference in the enthalpy (Salins, Reddy, and Kumar Citation2021, Citation2022).
(3)
(3) Heat transfer rate is the amount of heat energy exchange taking place due to condensation of water vapour into liquid. It is given in kW.
Dehumidifier effectiveness, ϵ, is defined as the ratio of the actual difference in specific humidity of the air in the dehumidifier to the maximum possible difference in specific humidity. It is represented by Equation (4). and
are the specific humidity at the inlet and outlet. ωeq is the specific humidity of air in the equilibrium state with the desiccant at its inlet condition, which is a function of the temperature and concentration of the desiccant at the inlet of the dehumidifier. Specific humidity is given by the unit, g/kg dry air (Salins, Reddy, and Kumar Citation2022).
(4)
(4) The amount of moisture removed from the air in a dehumidifier is assessed in the form of moisture removal rate (MRR). It is defined as the product of the mass flow rate of air and the difference in the inlet and outlet specific humidity. Moisture removal rate from the air is assessed from Equation (5) (Salins, Reddy, and Kumar Citation2021).
(5)
(5)
The average mass transfer coefficient is defined as the rate of moisture flux passing through a unit area (kg/m2-s). It can be obtained from the measured data as follows, it is given by Equation (6) (Salins, Reddy, and Kumar Citation2021, Citation2022):
(6)
(6)
(7)
(7) where K is the mass transfer coefficient, kg/m2-s, Wi, Wo, Weq and Wav be the specific humidity at inlet, outlet, equilibrium and average, g/kg of air. A be the surface area of the pad, m2.
4. Experimental test rig methodology
4.1. Experimental test rig
A schematic sketch of the dehumidification set-up with the calcium chloride as a desiccant is represented in (A). It consists of a reservoir to store the liquid desiccant, pads or packing as a carrier of the dehumidification process, duct to circulate or direct the air supplied at varying velocities from the 0.3 HP blower. The collector tank is used to accumulate the desiccant solution which is being sprayed on the pads with gravity. Pump with a capacity of 0.1 HP is used to circulate the solution throughout the cycle. The regenerator is used to bring back the concentration of the desiccant to the initial value by using an electric heater of 200-Watt capacity. An ultrasonic humidifier is used to maintain the inlet humidity to the desired level for the purpose of experimentation. Air is circulated from bottom to the top, whereas the desiccant is discharged from the top to the bottom through the packing and their interaction takes place in the counter flow direction. During the interaction, the desiccant absorbs the water vapour and hence its concentration decreases. The outlet air temperature depends on the temperature of the desiccant. The low concentration desiccant is regenerated using an electric heater to restore its original concentration. It is then passed through indirect cooling or heat exchanger to lower the temperature. Valve controls the flow rate of the desiccant and the filter removes the carryover of the desiccant from air entering the space. Air temperature and desiccant temperatures before and after the dehumidification are noted by using a digital thermometer. Air velocity is measured by using an anemometer. Relative humidity is measured by a hygrometer. Water Manometer tapings have been connected to two points before and after the packing to measure the pressure difference between entry and exit. The experimental test rig is given in (B). Specifications of the instruments used during the experimentation are given in and .
Figure 2. (A). Schematic representation of the Counter flow dehumidification test rig. (B). Dehumidification experimental test rig.

Table 1. Measuring devices to measure the parameters of dehumidification.
Table 2. Description of the desiccant solution and the packing media.
4.2. Methodology
Wood shavings of three different densities 200, 500 and 750 kg/m3 as shown in (a–c) are taken and loaded inside the packing frame, keeping the volume constant each time. They have been named as wood D1, wood D2 and wood D3, respectively. Calcium chloride desiccant concentrations of 20%, 30% and 40%, are prepared by diluting with water and made to spray onto the packing. For the purpose of comparison, Celdek packing with the wettability 632 m2/m3 is also used as the packing. The physical properties of desiccant, packing materials are given in .
Figure 3. wood shaving packing material description. (a) Wood shaving Continuous Chips (Density = 200 kg/m3), (b) Wood shaving Medium Chips (Density = 500 kg/m3), (c) Wood shaving short Chips (Density = 750 kg/m3).

Initially, experiments are performed with Celdek packing and 20% concentration of calcium chloride. Entry air temperature and relative humidity are maintained constant at 30°C and 75% each time. Operating parameters such as air velocity has been varied from 3 to 6 m/s. The experimental parameters such as relative humidity, temperature, desiccant temperature, air velocity and wet bulb temperatures are measured using measuring instruments both at the inlet and outlet. The above parameters are used to determine MRR, COP, SHR, humidification effectiveness and pressure drop across the pad section. The experiments are repeated for all the three different wood densities (wood D1, wood D2 and wood D3) and also with three different desiccant concentrations (20%, 30% and 40%).
5. Uncertainty analysis for LDDS
Experimental measured values are associated with unpredictable errors called as uncertainty. These errors in the independent terms are added together to determine the uncertainty of a dependent variable. Let R be the function of independent variables V1, V2, … … ., Vn and w1, w2 … … wn are uncertainty in the independent variables, then the uncertainty in ‘R’ will be
(8)
(8) Mass flow rate
. Uncertainty in
,
(9)
(9) Area
.
Uncertainty in area A,
(10)
(10) Moisture removal rate is given by
.
Uncertainty in moisture removal rate
(11)
(11) Heat transfer rate is given by
Uncertainty in heat transfer rate
(12)
(12) Dehumidification effectiveness
Uncertainty in dehumidification effectiveness,
(13)
(13) Coefficient of performance
Uncertainty in COP
(14)
(14) Average mass transfer coefficient
Uncertainty in K
(15)
(15) Clamp meter P = V*I
(16)
(16) where
,
,
,
,
,
,
are the uncertainty values for area, mass flow rate, air velocity, air temperature difference, specific humidity values, speed of rotation of motor shaft, voltage and current.
The uncertainty values for the independent terms like diameter, temperature, specific humidity, air velocity, voltage, current, diameter are substituted in Equations (9)–(16) and the uncertainty values for the dependent variables are calculated and are shown in .
Table 3. Uncertainty values for dependent variables.
6. Results and discussions
Dehumidification experiments have been performed using three types of wood packings by varying the operating parameters such as air flow rate from 3 to 6 m/s with increments of 1 m/s each time, and desiccant concentrations 20%, 30% and 40%. At each time, the parameters such as air temperatures and desiccant temperatures at the inlet and exit of the duct, air velocities, pressure drop across the pads are measured. Using the above details, performance parameters such as MRR, COP, dehumidification effectiveness, sensible heat factor and mass transfer coefficient are calculated and compared with the results obtained by using commercially available cellulose packing. The following section discusses the results obtained.
6.1. Relative humidity
Relative humidity variations with air velocity for the tested pads measured for 30% CaCl2 desiccant solution are represented in . When the velocity is lower, dehumidification is higher and it gradually reduces as the air velocity increases. An increase in air velocity causes reduced retention time of the air in the packing and this hinders the condensation rate of water vapour from air. Hence, dehumidification reduces and outlet RH increases or in other words the difference between the inlet and outlet RH reduces. Out of the four different types of pads tested, Celdek shows superior performance than wood shaving with slightly higher dehumidification values. Out of the three different wood densities, packing wood D2 shows higher RH drop than the other two showing better performance with density 500 kg/m3. For wood D1, shavings are loosely packed because of larger pieces thus increasing the void ratio, causing inferior performance. On the other hand, for wood D3 with density 750 kg/m3, the entire packing is filled with wood dust which opposes the air flow and hence water condensation rate is affected. Hence in either of the cases, air to desiccant interaction will be affected thereby reducing dehumidification. Due to the optimum loading of the wood chips, a comparatively higher dehumidification can be achieved by wood D2 which reveals that an optimum packing of wood shaving is essential for maximising the performance. The figure also shows the variation in RH values with three different concentrations of CaCl2 solution measured at 5 m/s of air velocity. The figure indicates a direct relationship of RH drop with the desiccant concentration. As the desiccant concentration increases, it is seen that RH values tend to drop thereby increasing the dehumidification of air. For higher concentration desiccants, vapour pressure of the desiccants will be lower. This increases the difference in vapour pressure difference between air and the wetted surface on the pads. Higher vapour pressure difference enhances the water condensation rate and thereby the outlet RH drops. Out of the three different wood packings, type wood D2 shows the best performance. Due to the optimum arrangement of wood chips, a continuous and smooth flow of the desiccant takes place along the packing and is responsible for higher dehumidification values. As noted, form the figure, the performance of wood D2 is slightly inferior to that of Celdek packing. When the concentration of CaCl2 is varied from 20% to 40%, ΔRH increases by 24% for wood D2 and that of Celdek by 26%, indicating the possible and potential use of wood packing as the dehumidification agent with increased concentrations.
6.2. Moisture removal rate (MRR)
MRR is the product of the difference in specific humidity values at the inlet and outlet with the mass flow rate of air. For lower air velocities, the mass of air entering the duct and interacting with desiccant is lower. This reduces the amount of water that is being condensed and it rapidly increases as the velocity of air is increased as shown in . Even though at higher velocities, dehumidification slightly reduces, but due to the increased mass of air results in higher moisture removed per minute from the pad surface, thus showing an improvement in the performance. Out of the four different pads, the cellulose pad shows the highest MRR followed by wood D2, wood D3 and wood D1, respectively. When the wood chips are denser, it does not allow the smooth flow of air through the packing so that interaction with the desiccant will be lower and hence the amount of water being condensed reduces. This reduces the MRR for high density wood packing wood D3 in the present study. At 3 m/s of air velocity, MRR is found to be 2.7 g/min, 4.2 and 4.9 g/min wood D1, wood D2 and wood D3 and when the air velocity is increased to 6 m/s the corresponding values are 10.9 g/min, 12.9 and 12.4 g/min, respectively. The figure also displays the variation of MRR with the desiccant concentration for a fixed air flow rate of 5 m/s. It is seen that as the concentration increases, MRR increases. Vapour pressure difference increases, which increases the capacity to absorb more moisture from the wetted surface. For any fixed air flow rate, it is seen that MRR for wood D2 is higher than wood D3 and wood D1, but lesser than the Celdek pad. At lower concentrations and lesser humidity values, the interaction between wood and the desiccant will be reduced whereas at too higher densities, air flow will be obstructed which affects the smooth flow of air and also energy exchange with the desiccant. In either case, effective dehumidification will not occur. When the concentration is increased, lower vapour pressure difference in addition to effective interaction of air with the desiccant enhance MRR for wood D2. When the concentration is increased from 20% to 40%, MRR is increased from 5.3 to11.2 g/min for wood D2 and this value is 16% lesser than the commercial Celdek packing.
6.3. Coefficient of performance (COP)
COP for dehumidification is the ratio of heat transfer from the condensate to the total energy supplied. It is seen that at lower air velocities, COP will remain lower. As the air velocity is increased, COP gradually increases and reaches a peak value at around 4.5 m/s and a further increase in the air velocity causes COP to decrease as depicted in . At higher air velocities, even though heat transferred as well as energy required increase, the rate of energy required for the blower is higher than the amount of heat transferred by the condensation of water vapour. Hence, the COP value reduces. Out of the four tested pads, COP of the Celdek packing is the highest followed by wood D2 and wood D3, respectively. Even though the density for wood D3 is higher, due to the decreased energy transfer or lesser dehumidification, COP is lower than wood D2 at any velocity of flow tested. This shows the better performance of wood with density 500 kg/m3 over 750 and 200 kg/m3. Highest COP for all pads is observed at 4.5 m/s and the values for wood D2 and wood D3 are accordingly 7.7% and 11.3% lesser than the Celdek packing measured at that air flow rate. Variation of COP with the different concentrations is shown in . It is clearly seen that as the concentration increases, COP increases. As discussed in the previous results, higher concentration improves the dehumidification rate due to the larger vapour pressure difference between desiccant on the pad surface and air. This increases the energy transfer and on the other hand energy required will remain the same. This results in higher COP. A similar trend as that of MRR can be seen here with Celdek remaining higher than wood D2 and wood D3. Upon comparing Celdek and wood D2, when the concentration is 20%, COP of the wood D2 and Celdek are found to be 1.64 and 1.98, respectively, and when the concentration is increased to 40%, these values are found to be increased by 19.5% and 15%, respectively, for wood D2 and Celdek.
6.4. Dehumidification effectiveness
Dehumidification effectiveness is the ratio of specific humidity difference between the inlet and the outlet to the difference in inlet specific humidity to the equilibrium specific humidity. It is found to be the highest for low air velocities and gradually decreases as the air velocity is increased. When the flow velocity is increased, the time available for air in contact with the desiccant over the pad surface decreases. This reduces the dehumidification values and hence efficiency drops. Out of the three wood pads tested, wood D2 shows the highest followed by D3 and D1, respectively, as indicated in . When the wood chips of optimum size and quantity have been placed inside the pad casing, it offers reduced resistance to the flow air and maintaining the increased contact and wetting between the desiccant liquid and chips better dehumidification can be observed. Hence, higher amount of moisture will be absorbed by the desiccant on the wetted wood chip surface. On the other hand, when the packings are loosely packed, exposed wood chip surface area with the desiccant drops, which reduces dehumidification. When the packing is too dense, it causes obstruction for the smooth flow of air which also affects water vapour condensation, thus reducing the dehumidification effectiveness. Results indicated that highest dehumidification effectiveness achieved for wood pads is for wood D2 and is 5.8% lower than the Celdek pad at an air velocity of 3 m/s.
Figure 7. Variation of Dehumidification effectiveness with air velocity and desiccant concentrations for various pads.

The figure also narrates the variation of dehumidification effectiveness with the concentration of the desiccant at a fixed value of air flow rate. It is seen that a higher concentration of the desiccant will reduce the equilibrium dew point temperature. This factor results in an increased value of dehumidification and hence higher efficiency will be obtained. But the use of higher concentrations is undesired as it poses the problem of crystallisation and also biological contamination. From the current result, it is seen that 40% Celdek packing shows highest efficiency. The structured arrangement of cellulose packing allows clear flow of air and desiccant which results in higher dehumidification and better efficiency. Out of the three wood packings tested, wood D2 shows the highest efficiency, marginally lesser than Celdek pad. At 20% concentration dehumidification efficiencies of wood D2 are 9.9% and 4% higher than wood D1 and wood D3 and 9.5% less than the Celdek packing. When the CaCl2 concentration is increased to 40%, dehumidification effectiveness of wood D2 is increased by 21.8% and less than Celdek packing by 4.9%. These results recommend that wood shaving with optimal arrangement of the quantity and size gives a satisfactory dehumidification effectiveness which is at par with the commercial cellulose pads.
6.5. Pressure drop (ΔP)
Pressure drop is another parameter that has been analysed during the dehumidification experiments. It decides the outlet velocity of air that is reaching the working area. If the packing is denser, it offers high resistance to the motion of air, due to which considerable pressure drop occurs and outlet air velocity and quality may be drastically reduced which may hamper the thermal comfort of the working area. In the present it is seen that wood D3 shows the highest ΔP followed by Celdek, wood D2and wood D1, respectively, as seen from . This clearly explains the disadvantage of excessive packing inserted in the given volume of the pad or in other words, in order to have best dehumidification performance, optimum density of the wood packing has to be maintained. As the flow rate is increased for all the pads, higher pressure drop is observed due to the increased frictional resistance offered due to its movement. When the air velocity is increased from 3 to 6 m/s, pressure drop for wood D2 is 16% lower than Celdek packing. As seen from the figure, pressure drop variation with the concentrations is not significant. A marginal higher drop may be experienced by the high concentration desiccant due to the slightly higher viscosity. Comparing the different pads, the Celdek pad shows highest drop followed by wood D3 and the least value is shown by wood D1. However, for any of the pads, change with the concentration is found to be minimum. From the values, it is understood that unlike the air flow rate, desiccant concentration will not have much impact on the pressure drop.
6.6. Sensible heat factor (SHF)
It is the ratio of sensible heat transfer to air to the total heat transferred due to the condensation of water vapour. Heat of condensation will be acquired by both air as well as the desiccant due to which both air temperature and desiccant temperature will increase. The fraction of heat acquired by the air to the total heat is referred to as SHF. shows the variation of SHF with air flow rate for various pads. As the air velocity is lower, dehumidification reduces, which in turn reduces the SHF. At lower air velocities, latent heat given by the condensation process will be lower and it increases as the air velocity is increased. It is intended that low sensible heat values are desired as it further reduces the cooling load in order to reach the required thermal comfort. Wood D2 shows marginal reduction in SHF compared to the Celdek packing. At 3 m/s, highest values of SHF are noticed and for wood D2, these values are 9.3% and 2.5% higher than wood D1 and wood D3, respectively, and 8.7% less than the Celdek pad. The figure also illustrates the variation of SHF for the various concentrations measured at a fixed air flow rate of 5 m/s. It indicates that as the concentration increases, SHF also increases. Higher concentration of the desiccant improves dehumidification as by water vapour being condensed increases. This in turn surge the heat of condensation and also SHF. From the figure it is seen that when the concentration is increased from 20 to 40%, SHF increases by 15.5% for wood D2.
6.7 Mass transfer coefficient
It is another important factor influencing the dehumidification performance and is calculated by using Equation (6). It mainly depends on the values of MRR, exposed surface area and vapour pressure difference between desiccant surface and air. It is seen that as the air flow rate and desiccant concentration are increased, the mass transfer coefficient also increases as MRR increases with the increase in both these factors. From , it is seen that out of the different pads, Celdek is having higher values followed by wood D2, wood D3 and wood D1 having the lowermost. On comparing the three different concentrations, it is seen that higher concentration of 40% gave the highest mass transfer coefficient for the Celdek pad followed by wood D2. Higher mass transfer coefficients will increase the MRR from the pad surface. Hence, the above results will reveal that out of the three different wood densities, 500 kg/m3 is ideal for dehumidification purposes. When the air velocity is increased from 3 to 6 m/s, the mass transfer coefficient of wood D2 is increased by 77%. Similarly, when the concentration is increased from 20 to 40%, it is increased by 42%.
7. Energy saving potential
Energy saving potential of the dehumidification and cooling with wood packing (wood D2 with 30% concentration) is compared with the conventional air conditioning systems taking the thermal comfort conditions as 25°C and relative humidity within the range of 55–62%. The inlet and outlet parameters obtained from the dehumidification experiments have been considered and the energy analysis is carried out. It is observed that dehumidification using the liquid desiccants has witnessed a saving in energy requirements to achieve the required thermal comfort inside the building for all the flow rates of air considered. The values will reveal that when the air velocity is increased from 3 to 6 m/s, saving in terms of energy varied from 30% to 45% as shown in . This clearly signifies the advantage of using a liquid-based dehumidification system especially with biomass-based wood shaving as a packing material over the conventional air conditioning system for cooling with dehumidification.
8. Validation
To validate the results obtained by the experimentation, the performance parameters such as dehumidification effectiveness, pressure drop and COP are compared with the results obtained by the various researchers. Type of desiccant, packing and flow configuration are selected as comparing parameters. gives a comparative study. It is observed that experimental results of wood shaving packing undertaken in the current study gave comparable results with the literature values. Trends of the important parameters considered are in line with the literature values and even some of the parameters are better than the reported literature values. These results will reveal that wood shaving can become one of the alternative biomass-based materials for dehumidification in buildings.
Table 4. Validation of the experimental results.
9. Optimisation of the results
Even though variation of several parameters has been discussed in the tested range for the counter flow dehumidification with wood packing, it is necessary to obtain the optimum design parameters in order to increase the performance with the consumption of minimum possible energy. Even though, several performance parameters have been analysed, important parameters such as change in relative humidity, energy consumption, pressure drop and dehumidification effectiveness are considered for optimisation. Optimisation of output parameters is carried out by considering the variation with input operating parameter air velocity and are represented by .
depicts change in relative humidity and the energy consumption plotted against air velocity. For the range of air velocity 4–5 m/s, the ΔRH is found to be 13% and the energy consumption is 410 W. In dehumidification effectiveness and energy consumption are plotted along the vertical axis and the air velocity along the x axis. The best value of dehumidification effectiveness is found to be 47%. In change in the pressure value is estimated from the curve by considering air velocity along the x axis and dehumidification effectiveness along the y axis. Value of ΔP obtained is 47 Pa. graph validates the value of ΔRH and ΔP when the graph is plotted against air velocity values. The counterflow dehumidifier should operate in the optimum values to provide the maximum performance with less power usage and reduced pressure drop. Optimised air velocity and corresponding optimised values of energy consumption rate, ΔRH, pressure drop and dehumidification effectiveness are given in .
Figure 13. (A) Variation of Dehumidification effectiveness and Energy Consumption with the air velocity. (B) Variation of Dehumidification effectiveness and change in the pressure with the air velocity.

Table 5. Optimum design conditions for counterflow dehumidifier.
Cost comparison is carried out between commercially available Celdek packing, Aspen packing and locally available wood shaving packing. It was found that for 1 m3 of the packing, the Celdek packing costs 25 AED, Aspen pads costs 21 AED and wood shaving packing is totally free. It is freely available as it is one of the by-products left with any woodwork. Wood shaving with a density of 500 kg/m3 shows a comparative performance with that of commercially available Celdek packing. Therefore, wood shaving packing with the density of 500 kg/m3 is an ideal replacement for the standard Celdek or Aspen packing.
10. Conclusions
An experimental investigation on dehumidification has been carried out using wood shaving as the pad material with CaCl2 as the desiccant. Analysis has been carried out by varying the air velocity and desiccant concentrations. The results obtained can be summarised as follows:
Wood shaving as a pad material shows good dehumidification characteristics whose performance is at par with that of commercially used Celdek packing.
Out of the three different densities tested, 500 kg/m3 shows best performance with MMR, ΔRH and dehumidification effectiveness being 9.7%, 18.7% and 4.9% lower than the Celdek pad when measured at 5 m/s of air velocity for 30% desiccant concentration.
Performance parameters such as MRR, COP and mass transfer coefficient are found to increase with an increase in air velocity as well as the desiccant concentration. When the air velocity is increased from 3 to 6 m/s these parameters increased by 133%, 5.29% and 78%, respectively, for wood D2.
Parameters such as ΔRH, SHF and dehumidification effectivenss are found to decrease with increasing air velocity and increase when the desiccant concentration is increased. A corresponding decrease in those parameters are 35%, 37% and 48% when the velocity increased from 3 to 6 m/s. When the concentration is increased from 20 to 40%, the corresponding increase are 25%, 16% and 21%, respectively.
Optimisation of the operating parameters had been conducted and it is revealed that for the optimum performance air velocity should be maintained between 4 and 5 m/s. Corresponding values of energy consumption, pressure drop dehumidification effectiveness and ΔRH are 410 W, 55 Pa, 65% and 13% respectively.
When the air velocity is increased from 3 to 6 m/s, energy saving obtained as compared to the conventional air condition system ranges from 30% to 45%.
Wood shaving packing gave good durability or life because of its configuration or the arrangement of the wood shave layers (for density = 500 kg/m3). It provides maximum contact between air and desiccant and also it allowed the fluids to pass through it without desiccant debris accumulation. After numerous tests for a period of three months, it was found that wood shaving packing gave consistent results without distorting the packing material or configuration.
From the current study, it can be concluded that wood shaving can used as an alternative biomass based dehumidification pad to the Celdek pack as it shows comparable performance, with added advantages such as biomass based and locally available, cheaper, renewable, better life, less maintenance, easy disposable and replacement. Hence, it certainly adds value to sustainable energy source as it saves energy required for conventional dehumidification equipment.
Nomenclature |
Abbreviations | ||
LDDS | = | Liquid desiccant dehumidification system |
MRR | = | Moisture removal rate |
COP | = | Coefficient of performance |
SHR | = | Sensible heat ratio |
HDH | = | Humidification-dehumidification |
AD | = | Adsorption desalination |
EC | = | Energy Consumed |
RH | = | Relative Humidity |
DBT | = | Dry bulb temperature |
WBT | = | Wet bulb temperature |
Chemical formulas | ||
LI BR | = | Lithium Bromide |
LI CL | = | Lithium Chloride |
CA CL2 | = | Calcium Chloride |
Variables/parameters | ||
QS | = | Sensible heat, kJ/kg |
QL | = | latent heat, kJ/kg |
EC | = | Energy Consumed, Watts |
WPUMP | = | Rate of Work done by pump, Watts |
WBLOWER | = | Rate of work done by blower, Watts |
WELECTRIC COIL | = | Rate of work done by electric heating, Watts |
HE | = | Heating effect, Watts |
WI | = | Specific Humidity at the inlet, g/kg |
WO | = | Specific Humidity at the outlet, g/kg |
WEQ | = | Specific Humidity of air in equilibrium, g/kg |
E | = | Dehumidification effectiveness or efficiency, % |
= | Mass flow rate of air, kg/s | |
K | = | Mass transfer rate, kg/m2-s |
ΔP | = | Rise in pressure, N/m2 |
TI | = | Inlet temperature, °C |
TO | = | Outlet temperature, °C |
ΔT | = | Change in temperature, °C |
ΔRH | = | Change in relative humidity, % |
D1 | = | Wood with density 200 kg/m3 |
D2 | = | Wood with density 500 kg/m3 |
D3 | = | Wood with density 750 kg/m3 |
Disclosure statement
No potential conflict of interest was reported by the author(s).
Data availability statement
Data can be given only on request by the required person or body.
References
- Abdel-Salam, A. H., G. Ge, and C. J. Simonson. 2013. “Performance Analysis of a Membrane Liquid Desiccant air-Conditioning System.” Energy and Buildings 62: 559–569. https://doi.org/10.1016/j.enbuild.2013.03.028.
- Bouzenada, S., L. Frainkin, and A. Léonard. 2017. “Experimental Investigation on Vapor Pressure of Desiccant for air Conditioning Application.” Procedia Computer Science 109: 817–824. https://doi.org/10.1016/j.procs.2017.05.338.
- Chai, S., X. Sun, Y. Zhao, and Y. Dai. 2019. “Experimental Investigation on a Fresh air Dehumidification System Using Heat Pump with Desiccant Coated Heat Exchanger.” Energy 171: 306–314. https://doi.org/10.1016/j.energy.2019.01.023.
- Cho, H. J., S. Y. Cheon, and J. W. Jeong. 2019. “Experimental Analysis of Dehumidification Performance of Counter and Cross-Flow Liquid Desiccant Dehumidifiers.” Applied Thermal Engineering 150: 210–223. https://doi.org/10.1016/j.applthermaleng.2019.01.006.
- Crofoot, L., and S. Harrison. 2012. “Performance Evaluation of a Liquid Desiccant Solar air Conditioning System.” Energy Procedia 30: 542–550. https://doi.org/10.1016/j.egypro.2012.11.064.
- Dong, C., R. Qi, L. Lu, Y. Wang, and L. Wang. 2017. “Comparative Performance Study on Liquid Desiccant Dehumidification with Different Packing Types for Built Environment.” Science and Technology for the Built Environment 23 (1): 116–126. https://doi.org/10.1080/23744731.2016.1215691.
- Jain, S., S. Tripathi, and R. S. Das. 2011. “Experimental Performance of a Liquid Desiccant Dehumidification System Under Tropical Climates.” Energy Conversion and Management 52 (6): 2461–2466. https://doi.org/10.1016/j.enconman.2010.12.052.
- Kavasoğulları, B., E. Cihan, and H. Demir. 2016. “Novel Packing Materials for Open Liquid Desiccant System.” Energy Procedia 91: 785–791. https://doi.org/10.1016/j.egypro.2016.06.244.
- Lu, J., M. Wang, Y. Li, and L. Yang. 2017. “Numerical Study on Dehumidification Performance of a Cross-Flow Liquid Desiccant air Dehumidifier.” Procedia Engineering 205: 3630–3637. https://doi.org/10.1016/j.proeng.2017.10.221.
- Mohamed, A. S. A., M. S. Ahmed, A. A. M. Hassan, and M. S. Hassan. 2016. “Performance Evaluation of Gauze Packing for Liquid Desiccant Dehumidification System.” Case Studies in Thermal Engineering 8: 260–276. https://doi.org/10.1016/j.csite.2016.08.005.
- Naik, B. K., and P. Muthukumar. 2019. “Experimental Investigation and Parametric Studies on Structured Packing Chamber Based Liquid Desiccant Dehumidification and Regeneration Systems.” Building and Environment 149: 330–348. https://doi.org/10.1016/j.buildenv.2018.12.028.
- Ou, X., W. Cai, and X. He. 2019. “Model-based Optimization Strategy for a Liquid Desiccant Cooling and Dehumidification System.” Energy and Buildings 194: 21–32. https://doi.org/10.1016/j.enbuild.2019.04.019.
- Qasem, N. A., and S. M. Zubair. 2019. “Performance Evaluation of a Novel Hybrid Humidification-Dehumidification (air-Heated) System with an Adsorption Desalination System.” Desalination 461: 37–54. https://doi.org/10.1016/j.desal.2019.03.011.
- Qi, R., J. Zhi, and L. Z. Zhang. 2019. “Wetting Improvement of Plastic Working Plate for Liquid Desiccant Dehumidification Systems.” Energy Procedia 158: 3076–3081. https://doi.org/10.1016/j.egypro.2019.01.994.
- Rafique, M. M., P. Gandhidasan, and H. M. Bahaidarah. 2016. “Liquid Desiccant Materials and Dehumidifiers – A Review.” Renewable and Sustainable Energy Reviews 56: 179–195. https://doi.org/10.1016/j.rser.2015.11.061.
- Ronghui, Q., and L. Lin. 2014. “Energy Consumption of Solar-Assisted Internally Cooled/Heated Liquid Desiccant air-Conditioning System in Hong Kong.” Energy Procedia 61: 2686–2689. https://doi.org/10.1016/j.egypro.2014.12.277.
- Salins, S. S., S. K. Reddy, and S. Kumar. 2021. “Effect of Climatic Conditions on the Performance of a Multistage Dynamic Dehumidifier Test rig.” Building and Environment 205: 108245. https://doi.org/10.1016/j.buildenv.2021.108245.
- Salins, S. S., S. K. Reddy, and S. Kumar. 2022. “Assessment of Process Parameters in a Dehumidification Process Using Biomass-Based Wood Shaving as a Packing Material.” Indoor and Built Environment 31 (2): 496–509. https://doi.org/10.1177/1420326X211008509.
- Sampath, S. S., S. Kumar, and S. K. Reddy. 2020. “Influence of Different Desiccants, Flow Type and Packings on the Liquid Desiccant Dehumidification System: A Review.” International Journal of Air-Conditioning and Refrigeration 28 (01): 2030002. https://doi.org/10.1142/S2010132520300025.
- Seenivasan, D., V. Selladurai, and T. V. Arjunan. 2018. “Experimental Studies on the Performance of Dehumidifier Using Calcium Chloride as a Liquid Desiccant.” International Journal of Energy Technology and Policy 14 (1): 49–63. https://doi.org/10.1504/IJETP.2018.088287.
- Suranjan Salins, S., S. K. Reddy, S. Kumar, and S. S. Kumar. 2022. “Investigation on the Performance of LDDS Using Biomass-Based Wood Shaving as the Packing Material.” International Journal of Sustainable Energy 41 (7): 731–750. https://doi.org/10.1080/14786451.2021.1976180.
- Wang, L., F. Xiao, X. Zhang, and R. Kumar. 2016. “An Experimental Study on the Dehumidification Performance of a Counter Flow Liquid Desiccant Dehumidifier.” International Journal of Refrigeration 70: 289–301. https://doi.org/10.1016/j.ijrefrig.2016.06.005.
- Watanabe, H., T. Komura, R. Matsumoto, K. Ito, H. Nakayama, T. Nokami, and T. Itoh. 2019. “Design of Ionic Liquids as Liquid Desiccant for an air Conditioning System.” Green Energy & Environment 4 (2): 139–145. https://doi.org/10.1016/j.gee.2018.12.005.
- Wen, T., L. Lu, Y. Nie, and H. Zhong. 2019. “Development and Investigation on the Dehumidification and Corrosion Resistance Performance of a new Mixed Liquid Desiccant.” International Journal of Heat and Mass Transfer 130: 72–82. https://doi.org/10.1016/j.ijheatmasstransfer.2018.10.066.
- Wu, A., C. Li, and H. Zhang. 2006. The Primary Research on Liquid Desiccant Dehumidifier with Cooling Capacity Using Compression Heat Pump System.
- Yang, Z., Z. Lian, and K. Zhang. 2017. “Study on the Operational Economy of the Ultrasonic Atomization Liquid Desiccant Dehumidification System.” Procedia Engineering 205: 2879–2886. https://doi.org/10.1016/j.proeng.2017.09.917.
- Zegenhagen, M. T., C. Ricart, T. Meyer, R. Kühn, and F. Ziegler. 2015. Experimental Investigation of a Liquid Desiccant System for Air Dehumidification Working with Ionic Liquids.