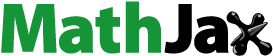
ABSTRACT
Barnyardgrass [Echinochloa crus-galli (L.) P. Beauv.] is the most difficult-to-control weed species of rice production systems worldwide. It has evolved resistance to different herbicide sites of action, including the acetyl-CoA carboxylase (ACCase)-inhibiting herbicides. Target-site mutations conferring resistance to ACCase-inhibiting herbicides are well documented; however, the role of the different ACCase genes in conferring resistance to cyhalofop-p-butyl (cyhalofop), an ACCase-inhibiting herbicide, remains poorly understood. This research assessed the contribution of gene amplification and expression of ACCase genes in a cyhalofop-resistant barnyardgrass accession. Additionally, the expression of glutathione-S-transferases (GSTs) and cytochrome P450 monooxygenases (P450s) genes as possible contributors to resistance to cyhalofop were investigated. Results demonstrated that ACCase gene amplification does not contribute to cyhalofop resistance. However, ACCase1 and ACCase3 were found to be overexpressed in the cyhalofop-resistant barnyardgrass accession. At 24 h after cyhalofop treatment, an overexpression of 2.0- and 2.8-fold was detected in ACCase1 and ACCase3, respectively. In addition, CYP81A21 (a P450 gene) was found to be 2.5-fold overexpressed compared to the susceptible accession in the same time period. These results suggest that ACCase1, ACCase3, and CYP81A21 are crucial genes in contributing cyhalofop resistance in this barnyardgrass accession.
Introduction
Barnyardgrass [Echinochloa crus-galli (L.) P. Beauv.] is a troublesome grass weed species in different cropping systems, but its presence is frequently related to rice (Oryza sativa L.) cultivation. In rice, the most prevalent weed species reported worldwide include barnyardgrass, weedy rice (Oryza sativa L.), and Cyperus weed species.Citation1 Barnyardgrass is a hexaploid (2 n = 6x = 54) weed species.Citation2,Citation3 It possesses a remarkable ability to adapt to new environments and produces an exorbitant number of seeds (ranging from 34,600 to 39,000 seeds plant−Citation1) that are easily dispersed by different means.Citation4,Citation5 In addition, barnyardgrass has allelopathic characteristics.Citation6 Yield reductions in rice due to barnyardgrass vary depending on the conditions studied; however, yield reductions can be up to 70% [reviewed by Citation6].
Control of barnyardgrass relies on the use of herbicides, and unfortunately, many accessions with resistance to different molecules have been reported worldwide.Citation7 In the US, herbicide-resistant barnyardgrass accessions exist in various states, including Arkansas, the largest rice-producing state in the US.Citation7–9 Globally, barnyardgrass has evolved resistance to many herbicide sites of action, with some specific herbicide examples being propanil (photosystem II–inhibiting herbicide),Citation10 pendimethalin (microtubule assembly-inhibiting herbicide),Citation7 mefenacet (very long-chain fatty acid synthesis-inhibiting herbicide),Citation11 quinclorac (auxin mimic-inhibiting herbicide),Citation12 cyhalofop,Citation13 penoxsulam (acetolactate synthase inhibiting-herbicide),Citation14 glyphosate [5-enolpyruvylshikimate-3-phosphate synthase-inhibiting herbicide (EPSPS)],Citation15 and clomazone (carotenoid biosynthesis-inhibiting herbicide).Citation16
The acetyl CoA carboxylase (ACCase; EC. 6.4.1.2) is a crucial enzyme that catalyzes the carboxylation of acetyl-CoA to produce malonyl-CoA.Citation17 In plants, ACCase is essential for the formation of primary fatty acids together with the buildup of long-chain fatty acids and flavonoids. ACCase-inhibiting herbicides work by blocking the formation of malonyl CoA, and, as a consequence, susceptible plants die from not having the ability to produce fatty acids.Citation17,Citation18 Necrosis of meristematic tissues, chlorosis and necrosis of apical meristem (flag leaf), and a purple leaf coloration due to the accumulation of anthocyanins are typical injury symptoms in plants treated with ACCase-inhibiting herbicides.Citation17,Citation19 Rice can safely tolerate cyhalofop due to a lack of esterase function, reduced absorption of the herbicide, and a rapid metabolism of cyhalofop acid.Citation20
In barnyardgrass, a severe cessation of growth, chlorosis, and desiccation may be observed together with new leaf growth-inhibition.Citation21 Nonetheless, resistant plants have deployed different strategies to avoid herbicide damage. Among those, plants have evolved a target-site mutation in the ACCase gene together with metabolic resistance, resulting in the herbicide being converted to nontoxic forms to surpass the herbicide toxicity.Citation17,Citation18,Citation22 Metabolic resistance of herbicides is convoluted and is normally governed by superfamilies of GSTs and P450s. However, it also includes glucosyltransferases (GTs) and aldo-keto reductase enzymes. Those superfamilies have been correlated to herbicide metabolism in different plant species.Citation22–30 In previous research, the metabolic resistance of barnyardgrass to cyhalofop herbicide was reported. The resistance in this population was attributed to a reduction in cyhalofop-acid compounds in the resistant accession compared to the susceptible standard.Citation13
Here, the contribution of ACCase genes to cyhalofop resistance has been explored. Thus, the main objective of this research was to describe the target-site resistance mechanisms involved in a cyhalofop-resistant barnyardgrass accession collected in eastern Arkansas. Gene-specific ACCase primers were designed to study their role in gene expression, gene amplification, and assess their involvement in the observed resistance to cyhalofop herbicide. Additionally, the role of GSTs and P450s genes as possible contributors to resistance to cyhalofop herbicide in barnyardgrass was examined.
Materials and methods
Plant material
A barnyardgrass accession previously characterized for resistance to cyhalofop and other aryloxyphenoxypropionate herbicides, together with a known susceptible accession were used in this research.Citation13 Under greenhouse conditions, the resistant barnyardgrass accession displayed a resistance factor of ≈ 15 compared to the susceptible standard when treated with cyhalofop.Citation13 To decrease biological variation among plants, the resistant accession followed an additional selection by treating plants with 3× cyhalofop field rate (1× = 313 g ai ha−Citation1). Treatments were carried out using a lab track sprayer calibrated to deliver 187 L ha−Citation1 using 1100067 nozzles. Further selected seeds were germinated using growing medium (Promix, LP15, Premier Horticulture Inc., PA, USA). When seedlings reached the one-leaf stage, they were transplanted to a 5 cm-diameter pot containing silt loam field soil (34% sand, 53% silt, 13% clay, and 1.5% organic matter). Plants were maintained under greenhouse conditions as described elsewhere.Citation13
ACCase-specific gene sequencing
Complementary DNA (cDNA) was extracted as described before.Citation31 To describe the presence of target-site mutations, six specific primer sets, as described in Citation32, were used to partially amplify the putative six ACCase gene copies in the cyhalofop-resistant and -susceptible barnyardgrass accessions (). Primer sets covered the region where target-site mutations have been previously associated with resistance to ACCase-inhibiting herbicides. Polymerase Chain Reactions (PCRs) were performed using OneTaq Hot Start 2× Master Mix with Standard Buffer (New England Biolabs, Ipswich, MA, USA) according to manufacturer directions and using 5 µL cDNA (five-fold dilution) as template in 25 µL reaction. To assess the best PCR conditions for ACCase amplification, gradient PCRs were carried out at different temperatures to select the optimum annealing temperature for each ACCase gene. Final PCR conditions were as follows: 94°C for 30s, 30 cycles of 94°C for 30s, 59–60°C for 30s, and 68°C for 135 s, a final extension of 68°C for 5 min, and reactions held at 10°C. After cycling, a 5 µL PCR product aliquot was loaded onto a 1.2% agarose gel to corroborate a single presence band. The remaining PCR products were cleaned using the Wizard SV Gel and PCR Clean up System (Promega Corp., Madison, WI, USA) and sent for bidirectionally Sanger sequencing (Eurofins Genomics, Louisville, KY, USA). Raw sequences were processed using BioEdit software.Citation33 At least three biological replicates for each resistant and susceptible barnyardgrass accession were sequenced.
Table 1. Primer sets used to partially amplify the ACCase gene copies in cyhalofop-resistant and -susceptible barnyardgrass accessions.Citation32
Gene expression under metabolic inhibition
Plants of resistant and susceptible accessions were managed as described in “Plant material” section. Plants were treated at the three- to four-leaf growth stage with either malathion (a P450 inhibitor) or NBD-Cl (a GST inhibitor) to assess ACCase, GSTs, and P450s gene expression under metabolic inhibition. Treatments consisted of a non-treated control (T1); cyhalofop at 313 g ai ha−Citation1 (T2); malathion at 2 kg ai ha−Citation1 + cyhalofop at 313 g ai ha−Citation1 (T3); and NBD-Cl at 80 g ha−Citation1 followed by cyhalofop at 313 g ai ha−Citation1 (T4) 48 h later. NBD-Cl was dissolved on 1% acetone + 0.1% Tween 20.Citation24 Cyhalofop treatments contained 1% v/v crop oil concentrate (Agri-dex, Helena Chemical Company, Collierville, TN, USA). Each pot had two seedlings which was considered one replicate, and each treatment had three replicates (n = 6).
RNA extraction and complementary DNA (cDNA) synthesis
RNA extraction and complementary DNA (cDNA) synthesis were performed as described before.Citation31 Fresh tissue of resistant and susceptible barnyardgrass accessions was collected, placed in 2 mL Eppendorf tubes, and immediately frozen in liquid nitrogen. Samples were stored at −80°C until RNA extraction. Monarch Total RNA Miniprep kit (New England Biolabs, Ipswich, MA, USA) was used to extract the total RNA. RNA quality and quantity were assessed spectrophotometrically using a nanodrop (Nanodrop 2000c, Thermo Scientific, Waltham, MA, USA). cDNA was obtained with 1 µg of total RNA as template using the iScript Reverse Transcription Supermix kit (Bio-Rad Laboratories Inc., Hercules, CA, USA).
ACCase gene expression
Transcripts were measured in non-treated and treated plants of resistant and susceptible barnyardgrass accessions. Thus, tissue of non-treated and treated plants was collected 24 h after treatments (HAT) for total RNA and cDNA synthesis as described earlier. Quantitative PCR (qPCR) was utilized to measure the transcript levels using cDNA according to MIQE guidelines.Citation34 Homologs of β-actin and glyceraldehyde 3-phosphate dehydrogenase (GAPDH) genes in barnyardgrass were used as reference genes to estimate the basal transcript levels in both treated and non-treated plants of resistant and susceptible accessionsCitation28,Citation35,Citation36 (). Previously research in barnyardgrass (E. crus-galli var. formosensis) resistant to cyhalofop reported the presence of six ACCase gene copies.Citation32 These six copies of ACCase genes were used in this study as target genes. Nucleotide sequences of ACCase gene copies deposited at the National Center for Biotechnology Information (NCBI) were retrieved to design a set of gene-specific primers. Additionally, a set of primers common to all six ACCase gene copies was designed based on a highly conserved region. Primers were designed using the Primer3Plus softwareCitation37 (). A CFX Connect Real-Time System instrument provided with CFX Maestro software (Bio-Rad Laboratories Inc., Hercules, CA, USA) was used to run all qPCR reactions and retrieve the quantification cycle (Cq) values, respectively. Each qPCR reaction comprised 5 µL of SsoAdvanced Universal SYBR Green Supermix (2×) (Bio-Rad Laboratories Inc., Hercules, CA, USA), 0.3 µL of each forward and reverse primer at 10 µM, 2.9 µL deionized water, and 1.5 µL of a 5-fold dilution cDNA in a 10 µL total volume ran on 96-well plates. The amplification protocol consisted of 30 sec at 98°C followed by 40 cycles of 10 sec at 98°C and 30 sec at 61°C and finalized with a standard melt curve analysis to corroborate correct amplification. No-template controls (deionized water instead of cDNA) on each primer set were included in every qPCR run. Amplicon efficiency for each primer set and sample reactions was obtained using the LinRegPCR software version 2021.2.Citation38 Fold-change expression values were calculated using the formula:
Table 2. Primer sequences of genes used to measure either transcript abundance or gene copy number in resistant and susceptible barnyardgrass accessions by qPCR.
where E corresponds to base of exponential amplification, ΔCq TG is the difference in the quantification cycles (Cq) of the target gene (TG), GeoMean represents the geometric mean of both reference genes (RG) (β-actin and GAPDH), and ΔCq RG is the difference in quantification cycles of reference genes.Citation39
To reduce biological variation among samples, equal amounts of leaf tissue from two plants were pooled to generate a single biological replicate. Subsequently, three biological replicates (n = 6) were obtained and further processed. A Student’s t-test was used to detect significant differences in fold-change expression between resistant and susceptible barnyardgrass accessions on each treatment.
P450s and GSTs gene expression
Homologs of the CYP81A21, CYP81A68, GST1, GST1a, GST2, GST2c, and GSTL3 genes in barnyardgrass were retrieved from the NCBI database. Primer design followed the same methodology as described earlier. Such genes have been reported in conferring resistance to different ACCase-inhibiting herbicides in Asia minor bluegrass (Polypogon fugax Nees ex Steud.), barnyardgrass, late watergrass [Echinochloa phyllopogon (Stapf) Koso-Pol.], and rigid ryegrass (Lolium rigidum Gaudin).Citation23,Citation26–28
ACCase gene copy number
Genomic DNA (gDNA) extracted from resistant and susceptible barnyardgrass was used to estimate the gene copy number of the ACCase genes relative to two reference genes using a qPCR approach and adhering to MIQE guidelinesCitation34 as described in the “ACCase-specific gene sequencing” section. Homologs of the cinnamoyl-CoA reductase (CCR) and peter Pan-like (PPAN) genes in barnyardgrass were used as reference genes (). Such genes have been reported as a single gene copy in different plant genomes.Citation40,Citation41 Gene copy number estimation was carried out as described before.Citation31 The gDNA was extracted from resistant and susceptible barnyardgrass accessions using the E.Z.N.A. Plant DNA kit (Omega Bio-Tek Inc., Norcross, GA, USA) and diluted to 10 ng µL−1 to use as template in subsequent qPCR reactions. Standard curves were created using 5-fold gDNA dilutions, and the efficiency (E) for each primer set was calculated as E = [10(−Citation1/slope)-1] × 100.Citation42 Each qPCR reaction included the same reagents as described earlier but 15 ng gDNA and run under the same conditions. The amplification protocol included 3 min at 98°C followed by 40 cycles of 10 sec at 98°C and 30 sec at 61°C and finalized with a standard melt curve analysis to corroborate correct amplification. Relative ACCase gene copy number was expressed as 2ΔCq as described elsewhere.Citation43,Citation44 The experiment included four plants (n = 4) per accession, and each run included two technical replicates for each gene. A Student t-test was used to identify differences between resistant and susceptible barnyardgrass accessions.
Results and discussion
ACCase-specific gene sequencing
Target-site mutations in the ACCase gene have been previously reported as responsible for conferring resistance to ACCase-inhibiting herbicides.Citation17 Those target-site mutations can be found commonly at positions 1781, 1999, 2027, 2041, 2078, 2088, and 2096 (numbered respect to that of Alopecurus myosuroides: GenBank accession AJ310767.1), all in the carboxyl transferase domain of ACCase enzyme.Citation18 This research attempted to sequence all six ACCase gene copies previously described in barnyardgrass.Citation32 Under the lab conditions, primers to amplify ACCase2, ACCase4, and ACCase6 did not yield any product amplification compared to ACCase1, ACCase3, and ACCase5 while using annealing temperatures ranging from 53 to 59°C ( Supplementary) in the barnyardgrass accessions investigated. Consequently, those were discarded for furthersequencing investigation. The lack of product amplification using the same primers could be related to genetic diversity. Echinochloa crus-galli var. crus-galli is native to Central America, East Asia, and Europe, whereas E. crus-galli var. formosensis is native to East Asia.Citation45 It is important to note that the number of ACCase genes in barnyardgrass remains unclear since some researchers have described different numbers of copies in the ACCase gene of this plant species. For instance, ref. Citation35 sequenced four copies of the ACCase gene from barnyardgrass.
Figure 1. Quantitative PCR analysis of acetyl CoA carboxylase (ACCase) genes in susceptible (S) and resistant (R) barnyardgrass accessions under different treatments. Bars ± standard deviation of the mean. A significant difference between susceptible and resistant barnyardgrass accession on each treatment is indicated by an asterisk (P ≤ .05).

In this research, analysis of the ACCase1, ACCase3, and ACCase5 between resistant and susceptible accessions did not show any nucleotide change that could lead to a target-site mutation (no further details). Similar results have been published in other barnyardgrass accessions and other ACCase-resistant weed species. A target-site mutation was shown as an unlikely resistance mechanism in cyhalofop-resistant barnyardgrass.Citation13 In another barnyardgrass population resistant to cyhalofop, no target-site mutation was reported,Citation32 and similar findings were reported by Citation46, where a target-site mutation was rejected as a possible resistance mechanism in a multiple herbicide-resistant barnyardgrass accession from Ningxia province in China. Asia minor bluegrass resistant to quizalofop-p-ethyl (quizalofop) had no target-site mutation in any of the four ACCase genes reported.Citation23 In large crabgrass [Digitaria sanguinalis (L.) Scop.] resistant to clethodim, sethoxydim, fenoxaprop-p-ethyl (fenoxaprop), fluazifop-p-butyl, and quizalofop herbicides, no target-site mutation was found in the ACCase gene.Citation47
Gene expression under metabolic inhibition
In this study, resistant and susceptible barnyardgrass plants were treated with malathion and NBD-Cl to assess ACCase, GSTs, and P450s gene expression under metabolic inhibition. Treatments included a non-treated control (T1), cyhalofop at 313 g ai ha−Citation1 (T2); malathion at 2 kg ai ha−Citation1 + cyhalofop at 313 g ai ha−Citation1 (T3); and NBD-Cl at 80 g ha−Citation1 followed by cyhalofop at 313 g ai ha−Citation1 48 h later (T4). ACCase gene-specific primers were designed to describe their role in the observed resistance of barnyardgrass to cyhalofop. Additionally, genes from the literature reported to contribute to herbicide resistance in other weed species were chosen. At 24 HAT, which was the time set up for sample collection, there were differences between resistant and susceptible accessions (P ≤ .05) (). The rationale in having selected 24 HAT for qPCR experiments is based on the production of cyhalofop-acid, thus differences were found at this time period between resistant and susceptible accessions. Resistant accession produced lower amounts of cyhalofop-acid compared to the susceptible accession and no differences were detected after 24 h time period.Citation13
Gene expression of ACCase1-6 differed between resistant and susceptible accessions in treatments T2, T3, and T4 (P ≤ .05). Fold-change expression in the resistant accession was approximately 2-times higher than the gene expression found in the susceptible one (, ACCase1-6). Ref. Citation35, reported similar results to those described here while using a common ACCase primer. At 6 h after cyhalofop treatment, there were differences in the expression level of resistant and susceptible accessions. Resistant accession was found to be 3.3-times overexpressed compared to susceptible, and the same trend was also observed in fenoxaprop- and pinoxaden-treated plants during the same time period.Citation35 However, the specific ACCase gene causing such overexpression remains unspecified.
In a quizalofop-resistant barnyardgrass accession treated with quizalofop at 60 g ai ha−Citation1, the basal gene expression in the resistant accession remained similar (≈ 1-fold) to that found in the non-treated control during the time of the study (8 days). However, in the susceptible accession, the gene expression decreased after herbicide application, and by 8 d after treatment, it was practically null.Citation48 In that research, it was concluded that gene overexpression was not involved in the resistance mechanism, but the number of ACCase genes present in such accessions was unclear.Citation48 Gene overexpression has been reported as well in johnsongrass [Sorghum halepense (L.) Pers.] resistant to sethoxydim and quizalofop, whereby the resistant accession displayed approximately 2.5-fold higher ACCase enzyme activity compared to susceptible accession.Citation49
ACCase1 also displayed differences in T2 and T3 treatments (P ≤ .05), where the resistant accession had approximately 2-fold more expression than the susceptible used for comparison (, ACCase1). Similarly, ACCase3 in the resistant barnyardgrass showed 2.8-, 2.7-, and 1.8-fold more expression (P ≤ .05) than the susceptible for T2, T3, and T4, respectively (, ACCase3). Expression levels in ACCase2, ACCase4, and ACCase5 did not differ among treatments (, ACCase2, ACCase4, and ACCase5). Surprisingly, in ACCase6, susceptible barnyardgrass had a 2.8-fold increase in expression than the resistant accession (P ≤ .05) in T2 treatment. Such overexpression would result from ACCase inhibition and stress caused by cyhalofop herbicide in this susceptible accession.Citation50 Nonetheless, it is interesting to note that the expression levels in both susceptible and resistant accessions in T3 and T4 treatments remained mostly below that of non-treated controls (T1) (, ACCase6). Interestingly, expression levels observed in the susceptible accession in ACCase1, ACCase3, and ACCase6 mainly in T3 and T4 treatments were lower than those found in the resistant accession. That could be explained by the presence of the metabolic inhibitors in those treatments that “enhanced” the efficacy of cyhalofop. Additionally, results suggest the involvement of metabolic resistance in the cyhalofop-resistant barnyardgrass. Expression levels found using cyhalofop alone versus in combination with the metabolic inhibitors decreased mainly in ACCase1 and ACCase3. Perhaps follow up gene expression experiments should explore longer harvest time points to gain more details about ACCase gene profiles. On the other hand, the detection of transcripts in ACCase2, ACCase4, and ACCase6 can be explained by the high sensitivity and specificity by qPCR technique along with the detection limits compared to a conventional PCR.Citation51,Citation52
Expression levels using the GST1 and GST1a had no differences between accessions in the treatments evaluated (, GST1, GST1a). Interestingly, GST2 was overexpressed by 2.7-, 3.0-, and 2.5-fold (P ≤ .05) in the susceptible accession in T2, T3, and T4 treatments, respectively (, GST2). A similar response was obtained with GST2c and GSTL3 genes. In GST2c, treatment T2 caused a higher expression (1.3-fold) level in the susceptible accession compared to that of the resistant (P ≤ .05) (, GST2c). In GSTL3, susceptible accession displayed 3.4-, 3.3-, and 2.5-fold higher expression levels in T2, T3, and T4 treatments, respectively compared to that found in the resistant accession (P ≤ .05) (, GSTL3). Contrary to the results found in this research, in a quizalofop-resistant accession of Asia minor bluegrass with no herbicide treatment, authors reported that GST2c and GSTL3 were constitutively expressed in the resistant accession. The authors concluded that quizalofop resistance in Asia minor bluegrass is related to non-target-site resistance mechanisms and that GST2c and GSTL3 are involved in resistance.Citation23
Figure 2. Quantitative PCR analysis of glutathione-S-transferase (GSTs) genes in susceptible (S) and resistant (R) barnyardgrass accessions under different treatments. Bars ± standard deviation of the mean. A significant difference between susceptible and resistant barnyardgrass accession on each treatment is indicated by an asterisk (P ≤ .05).

Regarding the P450s genes analyzed, gene expression using the CYP81A68 did not differ between susceptible and resistant accessions in the treatments evaluated (, CYP81A68). Nonetheless, in other barnyardgrass accessions resistant to ALS and ACCase-inhibiting-herbicides, it has been reported that the overexpression of CYP81A68 confers metabolic resistance to penoxsulam (an ALS-inhibiting herbicide) but also can confer resistance to cyhalofop and metamifop (ACCase-inhibiting herbicides).Citation28 Using the CYP81A21, the gene expression in the resistant accession showed a 2.5-fold increase in T2 treatment compared to that found in the susceptible accession (P ≤ .05) (, CYP81A21). This result suggests that CYP81A21, a P450 gene previously reported in contributing to resistance in late watergrass, is also contributing to resistance in those barnyardgrass accessions. Overexpression of CYP81A21 gene has resulted in an increase in cross-resistance to ALS and ACCase-inhibiting herbicides.Citation27 The accessions used in this research were both susceptible to the ALS-inhibiting herbicide imazethapyr-ammonium.Citation13 These results suggest that genes involved in metabolic resistance in one plant species not precisely should be involved in another one. For instance, GST2c and GSTL3 were discarded in this research as contributing to ACCase resistance. However, in Asia minor bluegrass, both genes were involved in the resistance to quizalofop.Citation23 Additionally, CYP81A68 was not associated to cyhalofop resistance in this research but it was in other barnyardgrass accessions.Citation28 Conversely, CYP81A21 was linked to cyhalofop resistance in this study but also in late watergrass.Citation27
Figure 3. Quantitative PCR analysis of CYP P450 genes in susceptible (S) and resistant (R) barnyardgrass accessions under different treatments. Bars ± standard deviation of the mean. A significant difference between susceptible and resistant barnyardgrass accession on each treatment is indicated by an asterisk (P ≤ .05).

Metabolism of ACCase-inhibiting herbicides has been reported as the non-target-site resistance mechanism in several resistant weed species, including Asia minor bluegrass resistant to quizalofop,Citation23 Chinese sprangletop [Leptochloa chinensis (L.) Nees] resistant to cyhalofop,Citation30 barnyardgrass resistant to penoxsulam, cyhalofop and metamifop,Citation25,Citation28 and rigid ryegrass resistant to multiple herbicide sites of action.Citation26 However, in ALS-resistant accessions of barnyardgrass and late watergrass, both target and non-target-site resistance mechanisms were reported. Resistance was attributed to an Ala122Asn and Trp574Leu target-site mutations in the ALS1 gene and overexpression of the ALS1 and no ALS2 or ALS3 copies.Citation53
Gene overexpression has been correlated to herbicide resistance in different herbicide-resistant weed species, which can result in an increased amount of enzyme produced by the plant to avoid herbicide damage. Thus, gene overexpression can be due to regulatory changes that increase gene transcription and/or a difference in the genomic copy number of the target gene, which also causes an increased gene transcription.Citation22 Results obtained in this research support the idea that gene overexpression of specific ACCase genes contributes to cyhalofop resistance in those barnyardgrass accessions. Rapid detection of herbicide-resistant weed species is crucial to implement the best possible weed management strategies.
ACCase gene copy number
Results displayed a low number of copies in all gene-specific ACCase compared to CCR and PPAN genes. Relative to CCR, the resistant accession showed a higher copy number (P ≤ .05) in the ACCase4 gene. However, biologically, this difference could not be related to ACCase resistance. Using PPAN, the relative gene copy number in susceptible and resistant accessions among ACCase genes, had no differences (P ≥ .05) ().
Figure 4. Relative gene copy number estimation of acetyl CoA carboxylase (ACCase) genes in susceptible (S) and resistant (R) barnyardgrass accessions. CCR and PPAN were used as reference genes to measure the relative gene copy number. Bars ± standard deviation of the mean. A significant difference between susceptible and resistant barnyardgrass accession on each gene is indicated by an asterisk (P ≤ .05).

Gene amplification of the target-site gene has conferred herbicide resistance in a diverse range of herbicide-resistant weed accessions.Citation22 Gene amplification of the EPSPS gene (target-site of glyphosate herbicide) in a diverse range of glyphosate-resistant weed species has been reported as a common target-site resistant mechanism.Citation22 Other than glyphosate, gene amplification has been found in more herbicide-resistant accessions with different herbicide sites of action. For instance, in Palmer amaranth (Amaranthus palmeri S. Watson) resistant to glufosinate, the resistant mechanism deployed was attributed to the amplification of the chloroplastic glutamine synthetase (GS) gene.Citation54 In large crabgrass accessions resistant to ACCase-inhibiting herbicides, gene amplification of the target ACCase gene was also reported.Citation47 In both cases, overexpression of the target-site gene was due to gene amplification.
In summary, this research focused on deciphering the role of gene expression and gene amplification of specific ACCase genes on cyhalofop resistance in barnyardgrass. Previously, the presence of a non-target-site resistance mechanism was documented, where a difference in cyhalofop-acid formation was detected between resistant and susceptible accessions at 24 h after cyhalofop treatment.Citation13 In this research, it has been demonstrated that ACCase1 and ACCase3 genes contribute to cyhalofop resistance. Furthermore, the overexpression observed in CYP81A21 suggests the involvement of this gene in cyhalofop resistance. Additionally, results indicate that gene amplification of ACCase genes is not contributing to resistance in this cyhalofop-resistant barnyardgrass accession.
Supplemental Material
Download JPEG Image (239.2 KB)Acknowledgments
Support given by the University of Arkansas System Division of Agriculture and the Arkansas Rice Research and Promotion Board was greatly appreciated.
Disclosure statement
No potential conflict of interest was reported by the author(s).
Supplementary material
Supplemental data for this article can be accessed online at https://doi.org/10.1080/15592324.2023.2172517
Additional information
Funding
References
- Kraehmer H, Jabran K, Mennan H, Chauhan BS. Global distribution of rice weeds – a review. Crop Prot. 2016;80:73–10.
- Aoki D, Yamaguchi H. Genetic relationship between Echinochloa crus-galli and Echinochloa oryzicola accessions inferred from internal transcribed spacer and chloroplast DNA sequences. Weed Biol Manag. 2008;8(4):233–242.
- Guo L, Qiu J, Ye C, Jin G, Mao L, Zhang H, Yang X, Peng Q, Wang Y, Jia L, et al. Echinochloa crus-galli genome analysis provides insight into its adaptation and invasiveness as a weed. Nat Commun. 2017;8(1):1031.
- Bagavathiannan MV, Norsworthy JK, Smith KL, Neve P. Seed production of barnyardgrass (Echinochloa crus-galli) in response to time of emergence in cotton and rice. J Agric Sci. 2012;150(6):717–724.
- Bosnic AC, Swanton CJ. Influence of barnyardgrass (Echinochloa crus-galli) time of emergence and density on corn (Zea mays). Weed Sci. 1997;45(2):276–282.
- Bajwa AA, Jabran K, Shahid M, Ali HH, Chauhan BS, Ehsanullah E. Eco-biology and management of Echinochloa crus-galli. Crop Prot. 2015;75:151–162.
- Heap I (2022) The international herbicide-resistant weed database. www.weedscience.org. Accessed February 24, 2022
- Riar DS, Norsworthy JK, Bond JA, Bararpour MT, Wilson MJ, Scott RC. Resistance of Echinochloa crus-galli populations to acetolactate synthase-inhibiting herbicides. Int J Agron. 2012;(2012):1–8.
- Rouse CE, Roma-Burgos N, Norsworthy JK, Tseng T-M, Starkey CE, Scott RC. Echinochloa resistance to herbicides continues to increase in Arkansas rice fields. Weed Technol. 2018;32(1):34–44.
- Carey VF, Hoagland RE, Talbert RE. Verification and distribution of propanil-resistant barnyardgrass (Echinochloa crus-galli) in Arkansas. Weed Technol. 1995;9(2):366–372.
- Cai X, Chen J, Wang X, Gao H, Xiang B, Dong L. Mefenacet resistance in multiple herbicide-resistant Echinochloa crus-galli L. Populations Pestic Biochem Physiol. 2022;182:105038.
- Lovelace ML, Talbert RE, Hoagland RE, Scherder EF. Quinclorac absorption and translocation characteristics in quinclorac- and propanil-resistant and -susceptible barnyardgrass (Echinochloa crus-galli) biotypes. Weed Technol. 2007;21(3):683–687.
- Hwang J-I, Norsworthy JK, González-Torralva F, Piveta LB, Barber LT, Butts TR. Cross-resistance of barnyardgrass [Echinochloa crus-galli (L.) P. Beauv.] to aryloxyphenoxypropionate herbicides. Pestic Biochem Physiol. 2022;184:105089.
- Fang J, Yang D, Zhao Z, Chen J, Dong L. A novel Phe‐206‐Leu mutation in acetolactate synthase confers resistance to penoxsulam in barnyardgrass (Echinochloa crus‐galli (L.) P. Beauv). Pest Manag Sci. 2022;78:2560–2570.
- Vázquez-García JG, Rojano-Delgado AM, Alcántara-de la Cruz R, Torra J, Dellaferrera I, Portugal J, De PR. Distribution of glyphosate-resistance in Echinochloa crus-galli across agriculture areas in the Iberian peninsula. Front Plant Sci. 2021;12:617040.
- Norsworthy JK, Scott RC, Banwarga S, Griffith GM, Wilson MJ, Still JA. Control of clomazone-resistant barnyardgrass in rice with preeemergence herbicides. In Norman RJ, Meullenet JF, Moldenhauer KK, editors. B. R. Wells Rice Research Studies. Fayetteville (AR): Arkansas Agricultural Experiment Station; 2008. p.190–193.
- Délye C, Zhang X-Q, Michel S, Matéjicek A, Powles SB. Molecular bases for sensitivity to acetyl-Coenzyme A carboxylase inhibitors in black-grass. Plant Physiol. 2005;137(3):794–806.
- Kaundun SS. Resistance to acetyl-CoA carboxylase-inhibiting herbicides. Pest Manag Sci. 2014;70(9):1405–1417.
- Ishikawa H, Yamada S, Hosaka H, Kawana T, Okunuki S, Kohara K. Herbicidal properties of sethoxydim for the control of gramineous weeds. J Pestic Sci. 1985;10(2):187–193.
- Ruiz-Santaella JP, Heredia A, Prado RD. Basis of selectivity of cyhalofop-butyl in Oryza sativa L. Planta. 2006;223(2):191–199.
- Kim J-S, Oh J-I, Kim T-J, Pyon JY, Cho KY. Physiological basis of differential phytotoxic activity between fenoxaprop-P-ethyl and cyhalofop-butyl-treated barnyardgrass. Weed Biol Manag. 2005;5(2):39–45.
- Gaines TA, Duke SO, Morran S, Rigon CAG, Tranel PJ, Küpper A, Dayan FE. Mechanisms of evolved herbicide resistance. J Biol Chem. 2020;295(30):10307–10330.
- Chen W, Wu L, Wang J, Yu Q, Bai L, Pan L. Quizalofop-p-ethyl resistance in Polypogon fugax involves glutathione S-transferases. Pest Manag Sci. 2020;76(11):3800–3805.
- Cummins I, Wortley DJ, Sabbadin F, He Z, Coxon CR, Straker HE, Sellars JD, Knight K, Edwards L, Hughes D, et al. Key role for a glutathione transferase in multiple-herbicide resistance in grass weeds. Proc Natl Acad Sci. 2013;110(15):5812–5817.
- Fang J, Zhang Y, Liu T, Yan B, Li J, Dong L. Target-site and metabolic resistance mechanisms to penoxsulam in barnyardgrass (Echinochloa crus-galli (L.) P. Beauv). J Agric Food Chem. 2019;67(29):8085–8095.
- Han H, Yu Q, Beffa R, González S, Maiwald F, Wang J, Powles SB. Cytochrome P450 CYP81A10v7 in Lolium rigidum confers metabolic resistance to herbicides across at least five modes of action. Plant J. 2021;105(1):79–92.
- Iwakami S, Kamidate Y, Yamaguchi T, Ishizaka M, Endo M, Suda H, Nagai K, Sunohara Y, Toki S, Uchino A, et al. CYP 81A P450s are involved in concomitant cross-resistance to acetolactate synthase and acetyl-CoA carboxylase herbicides in Echinochloa phyllopogon. New Phytol. 2019;221(4):2112–2122.
- Pan L, Guo Q, Wang J, Shi L, Yang X, Zhou Y, Yu Q, Bai L. CYP81A68 confers metabolic resistance to ALS and ACCase-inhibiting herbicides and its epigenetic regulation in Echinochloa crus-galli. J Hazard Mater. 2022;428:128225.
- Pan L, Yu Q, Han H, Mao L, Nyporko A, Fan L, Bai L, Powles S. Aldo-keto reductase metabolizes glyphosate and confers glyphosate resistance in Echinochloa colona. Plant Physiol. 2019;181(4):1519–1534.
- Zhao N, Jiang M, Li Q, Gao Q, Zhang J, Liao M, Cao H. Cyhalofop-butyl resistance conferred by a novel Trp-2027-Leu mutation of acetyl-CoA carboxylase and enhanced metabolism in Leptochloa chinensis. Pest Manag Sci. 2022;78(3):1176–1186.
- González-Torralva F, Norsworthy JK. Understanding resistance mechanisms to trifluralin in an Arkansas Palmer amaranth population. Genes. 2021;12(8):1225.
- Iwakami S, Hashimoto M, Matsushima K, Watanabe H, Hamamura K, Uchino A. Multiple-herbicide resistance in Echinochloa crus-galli var. formosensis, an allohexaploid weed species, in dry-seeded rice. Pestic Biochem Physiol. 2015;119:1–8.
- Hall TA. BioEdit: a user-friendly biological sequence alignment editor and analysis program for Windows 95/98/NT. Nucleic Acids Symp Ser. 1999;41:95–98.
- Bustin SA, Benes V, Garson JA, Hellemans J, Huggett J, Kubista M, Mueller R, Nolan T, Pfaffl MW, Shipley GL, et al. The MIQE guidelines: minimum information for publication of quantitative real-time PCR experiments. Clin Chem. 2009;55(4):611–622.
- Fang J, He Z, Liu T, Li J, Dong L. A novel mutation Asp-2078-Glu in ACCase confers resistance to ACCase herbicides in barnyardgrass (Echinochloa crus-galli). Pestic Biochem Physiol. 2020;168:104634.
- Gao Y, Pan L, Sun Y, Zhang T, Dong L, Li J. Resistance to quinclorac caused by the enhanced ability to detoxify cyanide and its molecular mechanism in Echinochloa crus-galli var. zelayensis. Pestic Biochem Physiol. 2017;143:231–238.
- Untergasser A, Nijveen H, Rao X, Bisseling T, Geurts R, Leunissen JAM. Primer3Plus, an enhanced web interface to Primer3. Nucleic Acids Res. 2007;35(Web Server):W71–W74.
- Ruijter JM, Ramakers C, Hoogaars WMH, Karlen Y, Bakker O, van den Hoff MJB, Moorman AFM. Amplification efficiency: linking baseline and bias in the analysis of quantitative PCR data. Nucleic Acids Res. 2009;37(6):e45–e45.
- Vandesompele J, De Preter K, Pattyn F, Poppe B, Van Roy N, De Paepe A, Speleman F. Accurate normalization of real-time quantitative RT-PCR data by geometric averaging of multiple internal control genes. Genome Biol. 2002;3(7):research0034.1.
- McInnes R, Lidgett A, Lynch D, Huxley H, Jones E, Mahoney N, Spangenberg G. Isolation and characterization of a cinnamoyl-CoA reductase gene from perennial ryegrass (Lolium perenne). J Plant Physiol. 2002;159(4):415–422.
- The Arabidopsis Information Resource (TAIR). [Online] 2021. [Accessed 2021 Mar 27]. https://www.arabidopsis.org
- Radstrom P, Lofstrom C, Lovenklev M. Strategies for overcoming PCR inhibition. In: Dieffenbach C, Dveksler G, editors. PCR primer: a laboratory manual. Cold Spring Harbor: Cold Spring Harbor Laboratory Press; 2003. p. 149–161.
- Gaines TA, Zhang W, Wang D, Bukun B, Chisholm ST, Shaner DL, Nissen SJ, Patzoldt WL, Tranel PJ, Culpepper AS, et al. Gene amplification confers glyphosate resistance in Amaranthus palmeri. Proc Natl Acad Sci U S A. 2010;107(3):1029–1034.
- Livak KJ, Schmittgen TD. Analysis of relative gene expression data using real-time quantitative PCR and the 2−ΔΔCT method. Methods. 2001;25(4):402–408.
- Randall RP. A global compendium of weeds. 3rd ed. Perth (Western Australia): RP Randall; 2017.
- Yang Q, Yang X, Zhang Z, Wang J, Fu W, Li Y. Investigating the resistance levels and mechanisms to penoxsulam and cyhalofop-butyl in barnyardgrass (Echinochloa crus-galli) from Ningxia Province, China. Weed Sci. 2021;69(4):422–429.
- Laforest M, Soufiane B, Simard M-J, Obeid K, Page E, Nurse RE. Acetyl-CoA carboxylase overexpression in herbicide-resistant large crabgrass (Digitaria sanguinalis). Pest Manag Sci. 2017;73(11):2227–2235.
- Huan Z, Xu Z, Lv D, Wang J. Determination of ACCase sensitivity and gene expression in quizalofop–ethyl-resistant and -susceptible barnyardgrass (Echinochloa crus-galli) biotypes. Weed Sci. 2013;61(4):537–542.
- Bradley KW, Wu J, Hatzios KK, Hagood ES. The mechanism of resistance to aryloxyphenoxypropionate and cyclohexanedione herbicides in a johnsongrass biotype. Weed Sci. 2001;49(4):477–484.
- González-Torralva F, Brown AP, Chivasa S. Comparative proteomic analysis of horseweed (Conyza canadensis) biotypes identifies candidate proteins for glyphosate resistance. Sci Rep. 2017;7(1):42565.
- Forootan A, Sjöback R, Björkman J, Sjögreen B, Linz L, Kubista M. Methods to determine limit of detection and limit of quantification in quantitative real-time PCR (qPCR). Biomolecular Detection and Quantification. 2017;12:1–6.
- Navarro E, Serrano-Heras G, Castaño MJ, Solera J. Real-time PCR detection chemistry. Clinica Chimica Acta. 2015;439:231–250.
- Panozzo S, Mascanzoni E, Scarabel L, Milani A, Dalazen G, Merotto AJ, Tranel PJ, Sattin M. Target-site mutations and expression of ALS gene copies vary according to Echinochloa species. Genes. 2021;12(11):1841.
- Carvalho-Moore P, Norsworthy JK, González-Torralva F, Hwang J-I, Patel JD, Barber LT, Butts TR, McElroy JS. Unraveling the mechanism of resistance in a glufosinate-resistant Palmer amaranth (Amaranthus palmeri) accession. Weed Sci. 2022;70(4):370–379.