ABSTRACT
WRKY transcription factors are critical for plant growth, development, and adaptation to stress. This paper focuses on the expression characteristic to abiotic stress and phytohormones of OsWRKY24, OsWRKY53, and OsWRKY70. Three OsWRKY TFs contained two conserved domains and there were multiple cis-elements in response to adversity stress and hormone signaling in their promoters. Real-time PCR analysis revealed their widespread expression in normal tissues during seedling and heading stages. Under various stresses such as darkness, low temperature, salt, and drought, or treatment with hormones like ABA, SA, MeJA, and GA, transcript levels of these genes had changed significantly in wild-type seedlings. The expression level of OsWRKY24 was upregulated by darkness, cold, SA, and MeJA but downregulated by salt, drought, ABA, and GA treatments. The transcripts of OsWRKY53 were induced by darkness, low-temperature, salt, drought, ABA, and JA, while inhibited by SA and GA. In addition, OsWRKY70 expression level was elevated under darkness, low-temperature, SA, and JA but suppressed with salt, drought, ABA, and GA. These findings provide valuable insights into the regulatory mechanisms by which WRKY TFs adapt to stress via plant-hormone signaling.
Introduction
WRKYs are a class of specific zinc finger-type transcription factors (TFs), which are named after the conserved WRKYGQK domain located at the N-terminal.Citation1 WRKY TFs bind to the cis-element W-box (T)TGAC(C/T) in the promoter region of target genes and regulate the expression of genes to maintain homeostasis of the internal environment in plants.Citation2 Based on the number of domains and the features of zinc fingers, WRKY TFs can be categorized into three subfamilies (group I, -II, and -III). Group I contains two WRKY domains and a zinc finger motif (C-X4–5-C-X22–23-H-X1-H). Group II includes a WRKY domain with the same zinc finger as group I. Group III harbors a domain and a zinc finger structure (C-X7-C-X23-H-X1-C).Citation1 The family evolution suggests that all WRKY genes might derive from group I.Citation3 With the discovery and utilization of more and more functional genes, regulation mechanism of genes has become one of the research focuses.
There were at least 75, 109, and 171 WRKY genes had been identified in Arabidopsis thaliana, Oryza sativa, and Triticum aestivum, respectively.Citation2,Citation4 WRKY TFs play a pivotal role in the life cycles of plants, including growth, development, senescence, and in the response to various environmental stresses.Citation5,Citation6 For instance, AtWRKY71 functioned as a positive regulator in ethylene-mediated leaf senescence by activating EIN2, ORE1, and ACS2.Citation7 WRKY1 regulated membrane transporters in Arabidopsis, which were involved in stomatal closure to retain water.Citation8 OsWRKY28 negatively regulated the transcript of JA biosynthesis genes, thereby impacting root growth.Citation9 Overexpression of OsWRKY76 decreased the resistance of rice blast (Magnaporthe oryzae).Citation10 Additionally, TaWRKY51 regulated ethylene synthesis and formation of branch roots in wheat by inhibiting the expression of ETH biosynthesis gene AC.Citation11
Recent research has shown that as a member of WRKY Group I, OsWRKY53, a negative regulator of salt stress, directly trans-regulated expressions of OsMKK10.2 and OsHKT1;5, improving salt tolerance of rice.Citation12 In addition, the mutant of OsWRKY53 enhanced cold tolerance during the booting stage, resulting in a higher yield in rice subjected to cold stress.Citation13 We found that the amino acid sequences of OsWRKY24 and OsWRKY70 were 52.43% and 62.87% identities to those of OsWRKY53, respectively. Furthermore, they also belonged to WRKY Group I.Citation14 These three TFs had been involved in regulating grain size, and they have been demonstrated to regulate defense responses against fungi, bacteria, and pests.Citation15–19 However, it was unclear whether OsWRKY24 and OsWRKY70 had the similar functions to OsWRKY53 in abiotic stress. In this study, we analyzed their conserved motifs and promoter characterization and investigated their expression patterns of tissue-specificity, abiotic stress responses, and hormone effects. These findings provide clues to further unravel the molecular basis and regulatory pathway of OsWRKY TFs under adverse circumstances.
Results
Conserved motifs and proteins structure of OsWRKY24, OsWRKY53, and OsWRKY70
Oryza sativa genes WRKY24, -53, and -70 were assigned transcript accession numbers. According to the results of bioinformatics analysis, these proteins were made up of 555, 487, and 572 amino acids, respectively. Their iso-electric points (pI) ranged from 5.52 to 8.05 (). The number of coils, helix, and strand were distinct in their secondary structures. The WRKY domains which possessed two complete conserved WRKYGQK sequences primarily located in the helix and coils (). Furthermore, the tertiary structure analysis revealed that there was a certain similarity in spherical molecule and the conserved domains were positioned at the center of them (). The findings suggested that the WRKY TFs may have similar biological functions.
Figure 1. Sequence of the three OsWRKY proteins.

Figure 2. Secondary structure analysis of three OsWRKY proteins.
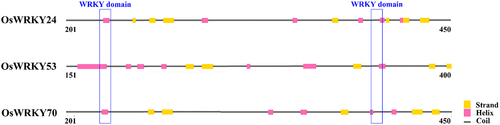
Figure 3. Tertiary structure analysis of OsWRKY proteins.
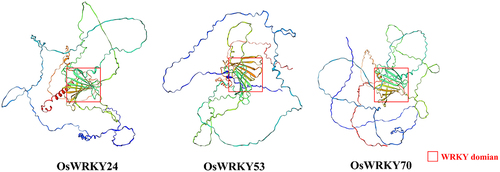
Table 1. Basic information of OsWRKY24, OsWRKY53, and OsWRKY70.
Promoter characterization of OsWRKY24, OsWRKY53, and OsWRKY70
To elucidate the function underlying the regulation of WRKY TFs, we analyzed the upstream 2000 bp region of OsWRKY24, -53, and -70. The results revealed that they contained a number of core elements which are in response to stress and hormone, such as antioxidant response element (ARE), stress-responsive element (STRE), wound responsive element (WRE3), abscisic acid-responsive element (ABRE), and salicylic acid-responsive element (as-1) (). There were 6, 2, and 4 STREs in OsWRKY24, -53, and -70, respectively. Four as-1 elements existed in OsWRKY24 and seven ABREs in OsWRKY53 (). The findings suggest that all these genes may participate in abiotic stress and hormone signaling in rice.
Figure 4. Analysis of promoter elements for OsWRKY24, OsWRKY53, and OsWRKY70.
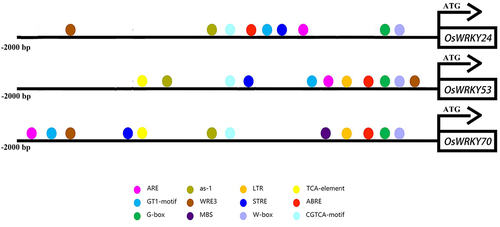
Table 2. Analysis of promoter elements for OsWRKY24, OsWRKY53, and OsWRKY70.
Tissue-specific expression pattern of OsWRKY24, OsWRKY53, and OsWRKY70
We examined the expression patterns of OsWRKY24, -53, and -70 in WT rice using fluorescence quantitative PCR. The results demonstrated that three OsWRKY genes were widely expressed under normal conditions and exhibited distinctive profiles in different tissue parts. At the seeding stage, the high expression level of OsWRKY24 and OsWRKY53 were both in leaves, while that of OsWRKY70 was in the roots which were 3.7 and 4.5 times higher than in shoots and leaves, respectively (). At the heading stage, OsWRKY24 and OsWRKY70 showed similar expression patterns in different tissues, they had high transcripts in leaf sheath and panicle, while transcript level of OsWRKY53 was low in panicle. Three OsWRKY genes had low-expression in the stem compared with other organs (). These results imply that three OsWRKY TFs which display tissue-specific expression pattern would play different roles in plant growth and development.
Figure 5. Temporal and spatial expression patterns of OsWRKY24, OsWRKY53, and OsWRKY70.
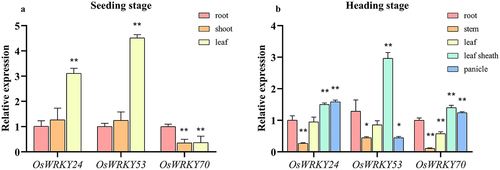
Expression profiles of OsWRKY24, OsWRKY53, and OsWRKY70 under abiotic stresses
To investigate whether OsWRKY24, -53, and -70 involved in stress responses, we conducted transcript levels of these genes under treatment with dark, cold, salt, and drought. The results showed that all three OsWRKY genes were significantly induced under dark. High-level transcript of OsWRKY24 could still be detected at 10 h, which was nearly a 5.5-fold up-regulation, while the expression levels of OsWRKY53 and OsWRKY70 reached the peak at 8 h and 6 h then decreased gradually (). Treated with 4°C, the mRNA level of OsWRKY24 was notably up-regulated nearly 20 times at 8 h. The expression of the three OsWRKY genes was clearly induced by cold stress, with a particularly significant up-regulation observed for OsWRKY24, which exhibited an approximately 18-fold increase at 8 h (). Three OsWRKY genes were also affected differentially by high salinity condition. As shown in , the salt induction of OsWRKY53 gradually increased during the early stages of stress, peaking at 10 h (22-fold). By contrast, OsWRKY24 and OsWRKY70 were constantly repressed from 0.5 h to 6 h and then rose to normal level by salt stress. The expression profiles of the three OsWRKY genes were significantly inhibited by drought stress, their mRNA levels reached the lowest level at 1 h and then increased gradually (). Taken together, these data indicate that three OsWRKY TFs might play an important role in response to different abiotic stresses.
Figure 6. Expression patterns of OsWRKY24, OsWRKY53, and OsWRKY70 under dark, cold, salt and simulated drought.
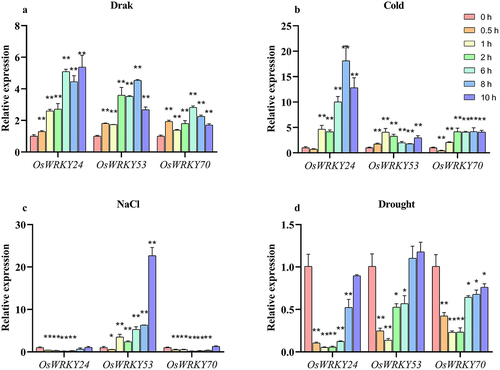
Expression profiles of OsWRKY24, OsWRKY53, and OsWRKY70 under hormone treatments
To study whether three OsWRKY genes in response to hormone, we further performed ABA, SA, MeJA, and GA on leaves of two-week-old seedings. The results showed that OsWRKY24 and OsWRKY70 were both suppressed by ABA, the latter exhibited more sensitivity to ABA, with approximately sixfold down-regulation at 6 h (). On the contrary, the expression level of OsWRKY53 was induced by ABA, which reached the peak at 9 h and returned to the normal level at 12 h (). The transcripts of OsWRKY24 and OsWRKY70 were induced by treatment of SA, with about a 3-fold and 2.5-fold upregulation at 6 h, respectively. In contrast, OsWRKY53 responded to SA slowly, which was inhibited at 12 h (). All three OsWRKY genes were induced by MeJA. Among them, OsWRKY24 was particularly sensitive to MeJA, with an over 20-fold up-regulation at 6 h (). In addition, under GA treatment, they were all repressed significantly within 6 h followed by maintenance of low transcript levels (). These results strongly suggest that these OsWRKY TFs may be involved in regulatory effects of phytohormone signaling.
Figure 7. Expression patterns of OsWRKY24, OsWRKY53, and OsWRKY70 responses to ABA, SA, MeJA and GA.
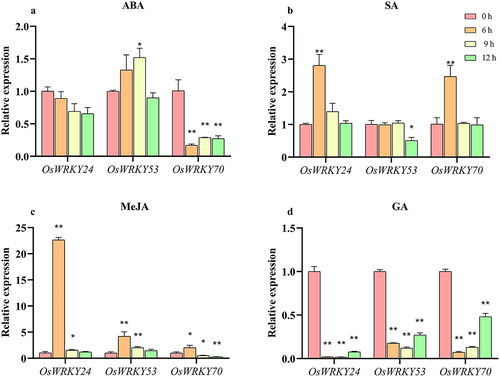
Discussion
It is well known that the structure of a protein determines its function. The diverse biological functions of protein molecules are based on their extremely complex chemical composition and spatial structure. Primary and three-dimensional structures of proteins are closely related to their specific functions. The WRKY domain is capable of specifically binding to the promoter W-box motif (T)(T)TGAC(C/T), where TGAC represents the core conserved sequence of W-box and is directly associated with the specificity of WRKY transcription factors and their downstream targets.Citation20 Two conserved WRKY domains exist in OsWRKY24, −53, and −70, in which the coding amino acid was between the 200th and the 450th (), indicating that their membership in WRKY group I. In Arabidopsis, WRKY18, WRKY40, and WRKY60 are very related in structure, which exhibit partially functional redundancy as negative regulators in plant resistance against the biotrophic bacterial pathogen P. syringae and the fungal pathogen E. cichoracearum.Citation21 AtWRKY38 and AtWRKY62, which encode two similar-type group III WRKY transcription factors, exert a regulatory role in the defense response against P. syringae.Citation22 In addition, GmWRKY58 and GmWRKY76, which belong to WRKY Group III, play a critical role in plant growth and flowering.Citation24 In recent research, it has been reported that OsWRKY24, −53, and −70 were involved in regulation of grain size.Citation18,Citation25 We speculate that the specific expression of OsWRKY24, −53, and −70 in panicles at the heading stage may be related to the regulation of grain size (). Moreover, OsWRKY24 and OsWRKY53 play an important role in the regulation of blast resistance.Citation17,Citation23 OsWRKY53 and OsWRKY70 as a specific herbivore-induced, activating the rice defense response against herbivores.Citation16,Citation26 These findings imply that WRKY proteins may display functional similarity in closely structural homologs.
Abiotic stresses, such as temperature inversion, salinity, and drought adversely affect the physiological and biochemical processes of plants.Citation27 Plants always modulate their gene expression in response to changes in the surrounding environment, which might thereby fine-tune the relevant physiological functions of proteins. Previous studies have demonstrated that WRKY TFs are involved in the regulation of plant growth and stress responses.Citation2 OsWRKY53 has been reported to play a critical role in leaf senescence,Citation28 In this paper, we found that promoter regions of OsWRKY24, -53, -70 contained 2, 6, and 2 light-responsive elements, respectively. Expressions of these genes were activated by darkness, and it seems that OsWRKY24 and OsWRKY70 could be possibly senescence-induced transcription factors. OsWRKY53 has been demonstrated to negatively regulate cold tolerance at the booting stage. OsWRKY24 is a potential candidate gene to influence the cold sensitivity of ‘Towada’ and ‘ZL31’.Citation13,Citation29,Citation30 Moreover, the analysis also showed that three OsWRKY genes were induced by low temperature (). Our experiments preliminarily found that the oswrky70 was sensitive to cold stress (no published data). The functions of OsWRKY24 and OsWRKY70 in chilling tolerance need to be further clarified. We found that OsWRKY53 was induced by salt strongly, while OsWRKY24 and OsWRKY70 were repressed (). OsWRKY53-OsMKK10.2 and OsWRKY53-OsHKT1;5 modules coordinate to regulate iron stress, which has been demonstrated.Citation12 OsWRKY53 was upregulated by the salt environment, which was consistent with the results reported above, suggesting OsWRKY24 and OsWRKY70 may play a regulatory role in the salt stress response. Regarding PEG treatment, OsWRKY24, -53, and -70 were also downregulated (), indicating their involvement in the response to drought stress. However, further investigation is needed to elucidate the specific regulatory mechanisms among these genes in the future.
Phytohormone is necessary for understanding the significance of WRKY TFs in plant development and stress. In Arabidopsis, WRKY62 could interact downstream of NPR1 and negatively regulate expression of JA-responsive genes, suggesting its involvement in the SA-mediated suppression of JA signaling.Citation31 Rice OsWRKY50 has regulated the ABA-dependent seed germination, seedling growth, and salt tolerance.Citation32 We found that the promoter of the three OsWRKY genes contained lots of cis-elements of hormone response (). Three OsWRKY genes were suppressed by GA, OsWRKY24 and OsWRKY70 was repressed by ABA, while OsWRKY53 was induced by ABA (). These three WRKY TFs have been speculated that may function in a partially redundant manner to antagonize the ABA and GA signaling pathways.Citation14 Moreover, OsWRKY53 has been proven to directly bind the promoters of OsABA8ox1 and OsABA8ox2 to repress their expression, resulting in elevated endogenous ABA contents that promoted leaf senescence.Citation28 Meanwhile, OsWRKY53 regulates the cold tolerance by suppressing the expression of gibberellin (GA) biosynthesis genes and reducing GA content in anther.Citation13 Therefore, it is worth considering whether the OsWRKY24 and OsWRKY70 are also involved in the response to abiotic stress through phytohormone signaling pathways. The conventional model of JA and SA signaling crosstalk involves antagonism between these two hormones.Citation33 OsWRKY24 and OsWRKY70 were induced by MeJA and SA, while transcript level of OsWRKY53 was suppressed by SA and increased by MeJA (), altered expression of OsWRKY70 has been found to affect the content of SA, JA, and GA.Citation16 OsWRKY24 as a blast-disease responsive transcription factor, positively regulates rice disease resistance; OsWRKY53 has also been reported to be involved in the regulation of bacterial and fungal resistance,Citation17,Citation19,Citation23,Citation34,Citation35 further investigation is required to confirm the role, if any, of OsWRKY24, −53, and −70 in the crosstalk between SA, JA, and biotic stress response in rice knockout mutants.
Plant growth, development, and responses to stress are regulated by a complex and intricate network, where different genes and hormones may exhibit synergistic or antagonistic effects. Although the three OsWRKY genes exhibit different expression patterns in response to stress and hormones, overall, they represent a self-defense mechanism employed by plants to cope with adverse environments. Continued exploration of the expression patterns of various genes under different stress conditions will provide clues for understanding their functions and underlying mechanisms.
Materials and methods
Plant materials, growth condition, and treatments
Rice seeds ‘NIP’ (Oryza sativa L. japonica) were imbibed in water in the dark at 37°C for 2 days. Germinated seeds were planted in substrate soil and grown in natural conditions and irrigated with 1/2 Yoshida culture under controlled light conditions (28°C, 16 h of light and 8 h of darkness).
To collect different parts of rice plants at different stages, including 2-week-old rice (roots, shoots, and leaves) and the heading stage (roots, stems, leaves, leaf sheaths, and panicles), 2-week-old rice seedlings were used for stress and hormone treatment. After 2 weeks, the seedlings were used for the following seven treatments. For darkness, seedlings were placed in a dark box. For cold treatments, seedlings were exposed to 4°C (16 h of light and 8 h of darkness). For salt stress and drought stress, seedlings were irrigated with 1/2 Yoshida culture containing 0.6% NaCl and 10% polyethylene glycol (PEG 6000), respectively. For ABA, JA, SA, and GA treatment, seedlings were sprayed 0.4 mmol/L ABA, 1 mmol/L SA, 100 μmol/L MeJA, and 100 μmol/L GA3, respectively. For stress treatment, the leaves of the samples were collected at 0, 0.5, 1, 2, 6, 8, and 10 h separately. For hormones treatment, samples were collected at 0, 6, 9, and 12 h separately. Each treatment was repeated three times.
Analysis of basic information and cis-elements of OsWRKY24, OsWRKY53, and OsWRKY70
The whole genome data of Oryza sativa L. japonica come from RGAP (http://rice.uga.edu/) database. Multiple sequence alignment of OsWRKY24, OsWRKY53, and OsWRKY70 identified was carried out using DNAMAN 8. PSIPRED (http://bioinf.cs.ucl.ac.uk/psipred/) and SWISS-MODEL (https://swissmodel.expasy.org/interactive) were used to analyze the secondary structure and the tertiary structure of three WRKY transcription factors. Upstream 2000-bp promoter sequences of these OsWRKY genes were obtained from NCBI (https://www.ncbi.nlm.nih.gov/) that were submitted to Plant CARE (https://bioinformatics.psb.ugent.be/webtools/plantcare/html/) for predicted cis-elements.
Total RNA extraction, reverse transcription, and qPCR analysis
For gene expression analysis, total RNA from the leaves of treated samples was isolated using Ultrapure RNA 200 preps Kit (Cwbio, China). cDNAs were synthesized with the HiScript III 1st Strand cDNA Synthesis Kit (+gDNA wiper) (Vazyme, China) according to the manufacturer’s protocol. Quantitative real-time PCR (RT-qPCR) was performed using ChamQ Universal SYBR qPCR Master Mix (Vazyme, China) in the CFX Connect Real-Time PCR Detection System (Bio-Rad, United States) as 95°C for 5 min, 95°C for 10 s, 60°C for 30 s, 40 cycles, and three replicates per sample. Gene-specific primers were designed using NCBI. The transcript levels of rice actin gene were used to normalize expression levels for genes (). The transcript levels of the examined genes were quantified by a relative quantitation method (2−▲▲T). Each RT-qPCR assay was biologically repeated at least twice with similar results, with each repetition having three technical replicates. Microsoft® Excel was used for data analysis. The differences were analyzed for statistical significance using two-tailed Student’s t-test.
Table 3. The list of qPCR primers used in the study.
Primers
The qPCR primers as shown in () were designed using Snapgene software and synthesized by Beijing Tsingke Biotech Co., Ltd.
Author contributions
Jiangdi Li: Conceptualization, Methodology, Writing – original draft, Data curation, Formal Analysis, Visualization. Yating Chen: Investigation. Rui Zhang: Investigation. Bin Wu: Investigation. Guiqing Xiao: Funding acquisition, Resources, Conceptualization, Methodology, Data curation, Writing – review and editing, Project administration, Supervision.
Disclosure statement
No potential conflict of interest was reported by the author(s).
Data availability statement
Data is contained within the article or supplementary material.
Additional information
Funding
References
- Chen F, Hu Y, Vannozzi A, Wu K, Cai H, Qin Y, Mullis A, Lin Z, Zhang L. The WRKY transcription factor family in model plants and crops. CRC Crit Rev Plant Sci. 2017;36(5–6):311–8. doi:10.1080/07352689.2018.1441103.
- Wani SH, Anand S, Singh B, Bohra A, Joshi R. WRKY transcription factors and plant defense responses: latest discoveries and future prospects. Plant Cell Rep. 2021;40(7):1071–1085. doi:10.1007/s00299-021-02691-8.
- Rinerson CI, Rabara RC, Tripathi P, Shen QJ, Rushton PJ. The evolution of WRKY transcription factors. BMC Plant Biol. 2015;15:66. doi:10.1186/s12870-015-0456-y.
- Ning P, Liu C, Kang J, Lv J. Genome-wide analysis of WRKY transcription factors in wheat (Triticum aestivum L.) and differential expression under water deficit condition. PeerJ. 2017;5:e3232. doi:10.7717/peerj.3232.
- Chen L, Song Y, Li S, Zhang L, Zou C, Yu D. The role of WRKY transcription factors in plant abiotic stresses. Biochim Biophys Acta. 2012;1819(2):120–128. doi:10.1016/j.bbagrm.2011.09.002.
- Rushton PJ, Somssich IE, Ringler P, Shen QJ. WRKY transcription factors. Trends Plant Sci. 2010;15(5):247–258. doi:10.1016/j.tplants.2010.02.006.
- Yu Y, Qi Y, Xu J, Dai X, Chen J, Dong CH, Xiang F. Arabidopsis WRKY71 regulates ethylene-mediated leaf senescence by directly activating EIN2, ORE1 and ACS2 genes. Plant Journal. 2021;107(6):1819–1836. doi:10.1111/tpj.15433.
- Qiao Z, Li CL, Zhang W. WRKY1 regulates stomatal movement in drought-stressed Arabidopsis thaliana. Plant Mol Biol. 2016;91(1–2):53–65. doi:10.1007/s11103-016-0441-3.
- Wang P, Xu X, Tang Z, Zhang W, Huang XY, Zhao FJ. OsWRKY28 regulates phosphate and arsenate accumulation, root System architecture and fertility in rice. Front Plant Sci. 2018;9:1330. doi:10.3389/fpls.2018.01330.
- Yokotani N, Sato Y, Tanabe S, Chujo T, Shimizu T, Okada K, Yamane H, Shimono M, Sugano S, Takatsuji H, et al. WRKY76 is a rice transcriptional repressor playing opposite roles in blast disease resistance and cold stress tolerance. J Exp Bot. 2013;64(16):5085–5097. doi:10.1093/jxb/ert298.
- Hu Z, Wang R, Zheng M, Liu X, Meng F, Wu H, Yao Y, Xin M, Peng H, Ni Z, et al. TaWRKY51 promotes lateral root formation through negative regulation of ethylene biosynthesis in wheat (Triticum aestivum L.). Plant Journal. 2018;96(2):372–388. doi:10.1111/tpj.14038.
- Yu J, Zhu C, Xuan W, An H, Tian Y, Wang B, Chi W, Chen G, Ge Y, Li J, et al. Genome-wide association studies identify OsWRKY53 as a key regulator of salt tolerance in rice. Nat Commun. 2023;14(1):3550. doi:10.1038/s41467-023-39167-0.
- Tang J, Tian X, Mei E, He M, Gao J, Yu J, Xu M, Liu J, Song L, Li X, et al. WRKY53 negatively regulates rice cold tolerance at the booting stage by fine-tuning anther gibberellin levels. Plant Cell. 2022;34(11):4495–4515. doi:10.1093/plcell/koac253.
- Zhang L, Gu L, Ringler P, Smith S, Rushton PJ, Shen QJ. Three WRKY transcription factors additively repress abscisic acid and gibberellin signaling in aleurone cells. Plant Sci. 2015;236:214–222. doi:10.1016/j.plantsci.2015.04.014.
- Hu L, Ye M, Li R, Zhang T, Zhou G, Wang Q, Lu J, Lou Y. The rice transcription factor WRKY53 suppresses herbivore-induced defenses by acting as a negative feedback modulator of mitogen-activated protein kinase activity. Plant Physiol. 2015;169(4):2907–2921. doi:10.1104/pp.15.01090.
- Li R, Zhang J, Li J, Zhou G, Wang Q, Bian W, Erb M, Lou Y. Prioritizing plant defence over growth through WRKY regulation facilitates infestation by non-target herbivores. Elife. 2015;4:e04805. doi:10.7554/eLife.04805.
- Yokotani N, Shikata M, Ichikawa H, Mitsuda N, Ohme-Takagi M, Minami E, Nishizawa Y. OsWRKY24, a blast-disease responsive transcription factor, positively regulates rice disease resistance. J Gen Plant Pathol. 2018;84(2):85–91. doi:10.1007/s10327-018-0768-5.
- Tang J, Mei E, He M, Bu Q, Tian X. Functions of OsWRKY24, OsWRKY70 and OsWRKY53 in regulating grain size in rice. Planta. 2022;255(4):92. doi:10.1007/s00425-022-03871-w.
- Xie W, Ke Y, Cao J, Wang S, Yuan M. Knock out of transcription factor WRKY53 thickens sclerenchyma cell walls, confers bacterial blight resistance. Plant Physiol. 2021;187(3):1746–1761. doi:10.1093/plphys/kiab400.
- Eulgem T, Rushton PJ, Robatzek S, Somssich IE. The WRKY superfamily of plant transcription factors. Trends Plant Sci. 2000;5(5):199–206. doi:10.1016/s1360-1385(00)01600-9.
- Xu X, Chen C, Fan B, Chen Z. Physical and functional interactions between pathogen-induced Arabidopsis WRKY18, WRKY40, and WRKY60 transcription factors. Plant Cell. 2006;18(5):1310–1326. doi:10.1105/tpc.105.037523.
- Kim KC, Lai Z, Fan B, Chen Z. Arabidopsis WRKY38 and WRKY62 transcription factors interact with histone deacetylase 19 in basal defense. Plant Cell. 2008;20(9):2357–2371. doi:10.1105/tpc.107.055566.
- Chujo T, Miyamoto K, Ogawa S, Masuda Y, Shimizu, T, Kishi-Kaboshi, M, Takahashi, A, Nishizawa, Y, Minami, E, Nojiri, H et al. Overexpression of phosphomimic mutated OsWRKY53 leads to enhanced blast resistance in rice. PLoS One. 2014;9(6):e98737. doi:10.1371/journal.pone.0098737.
- Yang Y, Chi Y, Wang Z, Zhou Y, Fan B, Chen Z. Functional analysis of structurally related soybean GmWRKY58 and GmWRKY76 in plant growth and development. J Exp Bot. 2016;67(15):4727–4742. doi:10.1093/jxb/erw252.
- Tian X, Li X, Zhou W, Ren Y, Wang Z, Liu Z, Tang J, Tong H, Fang J, Bu Q. Transcription factor OsWRKY53 positively regulates brassinosteroid signaling and Plant architecture. Plant Physiol. 2017;175(3):1337–1349. doi:10.1104/pp.17.00946.
- Hu L, Ye M, Li R, Lou Y. OsWRKY53, a versatile switch in regulating herbivore-induced defense responses in rice. Plant Signal Behav. 2016;11(4):e1169357. doi:10.1080/15592324.2016.1169357.
- Jiang J, Ma S, Ye N, Jiang M, Cao J, Zhang J. WRKY transcription factors in plant responses to stresses. J Integr Plant Biol. 2017;59(2):86–101. doi:10.1111/jipb.12513.
- Xie W, Li X, Wang S, Yuan M. OsWRKY53 promotes abscisic acid accumulation to accelerate leaf senescence and inhibit seed germination by downregulating abscisic acid catabolic genes in rice. Front Plant Sci. 2021;12:816156. doi:10.3389/fpls.2021.816156.
- Fang C, Zhang P, Jian X, Chen W, Lin H, Li Y, Lin W. Overexpression of Lsi1 in cold-sensitive rice mediates transcriptional regulatory networks and enhances resistance to chilling stress. Plant Sci. 2017;262:115–126. doi:10.1016/j.plantsci.2017.06.002.
- Wu B, Chen S, Cheng S, Li C, Li S, Chen J, Zha W, Liu K, Xu H, Li P, et al. Transcriptome analysis revealed the dynamic and rapid transcriptional reprogramming involved in cold stress and related core genes in the rice seedling stage. Int J Mol Sci. 2023;24(3):1914. doi:10.3390/ijms24031914.
- Mao P, Duan M, Wei C, Li Y. WRKY62 transcription factor acts downstream of cytosolic NPR1 and negatively regulates jasmonate-responsive gene expression. Plant Cell Physiol. 2007;48(6):833–842. doi:10.1093/pcp/pcm058.
- Huang S, Hu L, Zhang S, Zhang M, Jiang W, Wu T, Du X. Rice OsWRKY50 Mediates ABA-Dependent Seed Germination and Seedling Growth, and ABA-Independent Salt Stress Tolerance. Int J Mol Sci. 2021;22(16):8625. doi:10.3390/ijms22168625.
- Khan MIR, Fatma M, Per TS, Anjum NA, Khan NA. Salicylic acid-induced abiotic stress tolerance and underlying mechanisms in plants. Front Plant Sci. 2015;6:462. doi:10.3389/fpls.2015.00462.
- Chujo T, Takai R, Akimoto-Tomiyama C, Ando S, Minami E, Nagamura Y, Kaku H, Shibuya N, Yasuda M, Nakashita H, et al. Involvement of the elicitor-induced gene OsWRKY53 in the expression of defense-related genes in rice. Biochim Biophys Acta. 2007;1769(7–8):497–505. doi:10.1016/j.bbaexp.2007.04.006.
- Chujo T, Sugioka N, Masuda Y, Shibuya N, Takemura T, Okada K, Nojiri H, Yamane H. Promoter analysis of the elicitor-induced WRKY gene OsWRKY53, which is involved in defense responses in rice. Biosci Biotechnol Biochem. 2009;73(8):1901–1904. doi:10.1271/bbb.90262.