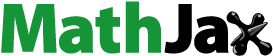
ABSTRACT
Background
The impact of heat waves and atmospheric oxidising pollutants on residential mortality within the framework of global climate change has become increasingly important.
Objective
In this research, the interactive effects of heat waves and oxidising pollutants on the risk of residential mortality in Fuzhou were examined. Methods We collected environmental, meteorological, and residential mortality data in Fuzhou from 1 January 2016, to 31 December 2021. We then applied a generalised additive model, distributed lagged nonlinear model, and bivariate three-dimensional model to investigate the effects and interactions of various atmospheric oxidising pollutants and heat waves on the risk of residential mortality.
Results
Atmospheric oxidising pollutants increased the risk of residential mortality at lower concentrations, and O3 and Ox were positively associated with a maximum risk of 2.19% (95% CI: 0.74–3.66) and 1.29% (95% CI: 0.51–2.08). The risk of residential mortality increased with increasing temperature, with a strong and long-lasting effect and a maximum cumulative lagged effect of 1.11% (95% CI: 1.01, 1.23). Furthermore, an interaction between atmospheric oxidising pollutants and heat waves may have occurred: the larger effects in the longest cumulative lag time on residential mortality per 10 µg/m3 increase in O3, NO2 and Ox during heat waves compared to non-heat waves were [−3.81% (95% CI: −14.82, 8.63)]; [−0.45% (95% CI: −2.67, 1.81)]; [67.90% (95% CI: 11.55, 152.71)]; 16.37% (95% CI: 2.43, 32.20)]; [−3.00% (95% CI: −20.80, 18.79)]; [−0.30% (95% CI: −3.53, 3.04)]. The risk on heat wave days was significantly higher than that on non-heat wave days and higher than the separate effects of oxidising pollutants and heat waves.
Conclusions
Overall, we found some evidence suggesting that heat waves increase the impact of oxidising atmospheric pollutants on residential mortality to some extent.
Responsible Editor Maria Nilsson
Introduction
Climate change and ambient air pollution are regarded as significant global health threats in the 21st century, as they cause significant excess mortality [Citation1,Citation2]. Meanwhile, heat waves and air oxidising pollution [Citation3,Citation4] have been linked to non-accidental deaths in numerous epidemiological studies with time-series analyses [Citation5–7]. Li found that exist positive and significant excess death risks due to the synergism between high temperature and air pollution (PM10, O3, NO2, and CO) in Northeast Asia [Citation6]. Studies also observed an interaction between high temperature and air pollutants (PM10, O3, NO2, and SO2) on mortality in China [Citation5,Citation7]. Heat waves and oxidising pollution have independent effects on health [Citation8,Citation9], but their potential interaction could amplify the burden of mortality. A synergistic effect may exist for these two hazards [Citation7]. However, research relating to the potential interactive effects between heat waves and oxidising pollution on mortality is limited today.
Health concerns can arise from both air pollution and extreme heat, and the risk of disease is influenced by the interplay between temperature and oxidising pollutants [Citation10]. Oxidising pollution has been linked to non-accidental deaths in numerous epidemiological studies and been observed in different countries [Citation11–14]. As heat wave events [Citation15,Citation16] have become more frequent in recent years, studies have demonstrated that excessive heat is connected with an increased risk of mortality in the population [Citation17–20]. In developing nations, the number of such studies is rather low, and the results have not yet been harmonised [Citation21].
According to some studies, the combined impacts of high temperatures and air pollution should be more detrimental to health outcomes than each factor acting alone [Citation5–7]. Although the relationship between air pollution and high temperatures has recently received increased attention [Citation22,Citation23], the results are still inconclusive. According to a study conducted in the USA [Citation24], the systemic reaction brought on by short-term ozone exposure varied with temperature and was more severe at high temperatures. Furthermore, other studies in Europe and the USA, where the air quality is good, have also shown an interaction between high temperatures and oxidising pollutants such as photochemical oxidants (such as ozone, O3), carbon monoxide (CO) and nitrogen oxides (NOx) on the risk of mortality in the population [Citation25–27].
Low levels of oxidising pollutant exposure has been shown to increase the risk of population mortality [Citation28–31], even in Europe, where oxidising pollutant concentrations are better controlled [Citation3,Citation32], while few such studies have been conducted in lightly polluted cities in southern China. Therefore, analysis of the combined effects of heat waves and low-level oxidising pollutant exposure can further inform the development of interventions designed to reduce the burden of oxidising pollutant pollution and heat wave-induced mortality.
Fuzhou is a city characterised by a humid subtropical climate and low level of air pollution in China [Citation33]. Fuzhou is one of China’s hottest provincial capitals according to data from the Chinese Meteorological Agency and has been called an ‘oven’ city because of its extremely hot summers [Citation33]. In comparison to some of the more polluted towns in northern China [Citation34,Citation35], Fuzhou has higher air quality, and many of its air pollutants are below the Chinese National Ambient Air Quality Standard (CAAQS) standard I [Citation36]; this city is considered a low-concentration control site for the National Air Pollution Health Impacts Monitoring Project. Given that China’s air quality is improving as a result of ongoing interventions and that there will be more days with high heat as a result of climate change, Fuzhou was selected as the research location to examine how heat waves and oxidising pollutants in the atmosphere interact. Future research of this kind in other southern coastal cities with hot but less polluted climates may use the findings of this study as a guide.
Method
Data collection
We chose Fuzhou City as the study site. The data collected everyday for this study are long time-series of six consecutive years from 1 January 2016, to 31 December 2021, in Fuzhou city; they include environmental data, meteorological data and residential mortality data.
Environmental data were obtained from seven national environmental monitoring sites at the Fuzhou City Environmental Monitoring Centre Station, including daily average concentrations of atmospheric pollutants. Meteorological data were obtained from the Fuzhou Meteorological Bureau, including temperature, barometric pressure and relative humidity. Mortality data were collected by the Fuzhou Centre for Disease Control and Prevention (CDC). Number of daily deaths used the International Classification of Disease version 10 (ICD-10) underlying cause of death codes as the criterion for classifying disease variables and collecting numbered non-accidental total deaths (ICD-10: A00-R99), including deaths due to diseases of the circulatory system, respiratory system, cardiovascular system, cerebrovascular system, etc. The research received ethical approval from Fuzhou Center for Disease Control and Prevention, Bio- and Medical Ethics Committee (No: 2022003). We obtained verbal consent to enter the households and then written informed consent was obtained in the survey to ensure the authenticity of the study.
Heat wave definition
A heat wave was defined as a consecutive period of at least 3 days during which the daily maximum temperature exceeded the 92.5th percentile of the reference period (2016 − 2021) [Citation37,Citation38]. This definition is optimal for its capacity to capture the real pattern of health effects at a national scale, which is derived from the best model fit of the exposure-response relationship [Citation38,Citation39]. Almost all heat waves occur in warm seasons [Citation9], hence, we limited our research to the period of May to October.
Data analysis
Studies of the health impacts of temperature and air pollution primarily use time-series studies and case-crossover studies; of these, generalised additive models (GAMs) in time-series research are the most common [Citation40]. A quasi-Poisson connected generalised additive model was used to analyse the effect of exposure factors on population mortality because small probability events, such as deaths roughly follow a Poisson distribution; the variance in daily death counts calculated in this study was higher than the mean.
Models for the effects of oxidising pollutants on mortality
On the basis of the GAMs, a log-linear exposure-response model was fitted using a spline function [Citation41], and the independent variables were treated non-linearly to plot exposure-response curves, with the following analytical model form:
Where: E (Yt) is the expected value of the number of deaths on day t; Zt is the concentration of atmospheric oxidising pollutant on observation day t; S is a spline function for exposure-response curve fitting; ns()-natural smoothing spline function to control for nonlinear confounding factors; df is its degree of freedom; time is the date variable; Xt is the meteorological factor, including mean temperature, mean barometric pressure and mean relative humidity; Dow is a dummy variable reflecting the day-of-week effect; holiday is a dummy variable reflecting the holiday effect; and ɑ is a constant intercept term.
A single pollutant model was used as the main model to analyse the relative risk of short-term air pollution (O3, Ox, NO2) exposure to the number of daily deaths in the population. A multi-day moving average lag01-lag07 was introduced to analyse the cumulative lag effect of pollutants to prevent underestimation of the health effects of pollutants [Citation42,Citation43]. The excess risk and 95% confidence interval (ER, 95% CI) for the risk of mortality when pollutant concentrations were increased were calculated. The specific analytical model form was as follows:
Where: β is the exposure response relationship coefficient, i.e. the increase in daily mortality per unit increase in pollutant concentration; other variables are the same as in Equationequation (1)(1)
(1) .
Models for the effect of temperature on mortality
The GAMs combined with a distributed lagged non-linear model (DLNM) was used in this study to fit the non-linear temperature-mortality relationship and lagged effects. The maximum lag days were set at 14 days [Citation44]. The analytical model form was as follows:
Where: Tempt,l is the lagged days temperature matrix obtained from DLNM; l is the maximum lagged days; pollutant is the explanatory variable that has a linear effect on the dependent variable, which is the concentration of atmospheric pollutants on observation day t; and Xt is relative humidity, which incorporates a low correlation meteorological factor with temperature [Citation45]. Other variables are the same as in Equationequation (1)(1)
(1) .
Interaction model for oxidising pollutant and heat waves
A thin-slab spline function was used to demonstrate the interaction between air temperature and atmospheric oxidising pollutants on mortality. Atmospheric oxidising pollutants and temperature were introduced into the model via a continuous equation with bivariate variables, and 3D graphs of these two variables and the ending variables were plotted for visually judging the relationship between the three variables. The model is as follows:
Where: TS stands for thin-plate regression splines; TS(PtL,Tempt) represents the interaction term between atmospheric oxidising pollutants and temperature, PtL is the moving average of atmospheric oxidising pollutants on day t for day L; and Tempt is the daily average temperature on day t. Other variables are the same as in Equationequation (1)(1)
(1) .
A GAM model was used to assess the risk of oxidising pollutants to mortality under both heat wave and non-heat wave layers. The model is as follows:
Where: β is the exposure-response coefficient; Hk denotes the dummy variable for heat wave (H1 = 0, H2 = 0) and non-heat wave days (H1 = 0, H2 = 0); and β1 and β2 represent the role of oxidising pollutants in the heat wave and non-heat wave layers, respectively. Other variables are the same as in Equationequation (1)(1)
(1) .
All data analyses were performed using ‘tsModel’, ‘dlnm’, ‘mgcv’, ‘lubridate’, ‘ggplot2’, ‘plyr’ and ‘splines’ package in statistical software R version 4.2.1 [Citation33], p = 0.05.
Results
Descriptive statistics
provides the descriptive statistics on the average number of daily non-accidental deaths and weather conditions in Fuzhou, China, 2016–2021. During the study period, the daily concentrations of PM2.5 and PM10 were on average 23.06 μg/m3 and 44.12 μg/m3, respectively, while the daily average values of NO2, SO2, CO, Ox and O3–8 h were 23.36 μg/m3, 5.13 μg/m3, 0.65 mg/m3, 66.36 μg/m3 and 88.54 μg/m3, respectively. The average barometric pressure, temperature and humidity were 1008.40 hPa, 21.60°C and 72.86%, respectively. The population experiences approximately 32 non-accidental deaths per day.
Table 1. Descriptive statistics on daily mortality and weather conditions in Fuzhou, China, 2016–2021.
Effects of oxidising pollutants on mortality
shows the curves of O3, NO2 and Ox and the risk of mortality in Fuzhou. There was a positive correlation between increasing concentrations of O3, NO2 and Ox and the relative risk of death for the population (p < 0.05). The curves for the effects of O3 and NO2 on mortality are approximately linear and increase at the smallest concentrations, O3: 70 ~ 100 µg/m3 and NO2: 15 ~ 25 µg/m3.
Figure 1. The impact of oxidising pollutants on the health of the population. (a) Exposure-response curves for O3, NO2 and Ox on the main causes of mortality in the population. (b) Impact of each 10 μg/m3 increase in O3, NO2 and Ox on the risk of excess mortality in the population (ER values and 95% CI).

O3, NO2 and Ox were introduced as linear independent variables for the single pollutant model analysis. and Supplement show the effect of each 10 μg/m3 increase in O3, NO2 and Ox concentrations on the excess mortality risk of residents in Fuzhou. The results show a more significant positive association overall between O3 and resident mortality, for which the overall O3 effect value [2.13% (0.6%, 3.68%)] is higher than that of Ox [1.29% (0.51%, 2.08%)]; a more significant negative association is found between NO2 and resident mortality, with an effect value [−1.67% (−3.23%, −0.09%)]. O3, Ox and NO2 have different effect values on mortality and show inconsistent lagging characteristics.
Effects of heat waves on mortality
There were 24 heat wave events in Fuzhou during May–October 2016–2021, with a total of 114 heat wave days and 990 non-heat wave days. Heat waves occurred as early as mid-June and as late as late-September. 2020 had the highest number of heat wave events at 6. 2020 and 2021 had the highest number of heat wave days at 27. 2017 and 2018 had the longest duration of heat wave events at 10 days.
) shows the 3D and contour plots of the effect of temperature on mortality, respectively. We can see a significant positive effect of temperature on residential mortality at high versus low temperatures, while the risk value is greater in extremely hot weather and on an upward trend.
Figure 2. The impact of temperature on the health of the population. (a) 3D maps of the effect of temperature on residential mortality. (b) Contour maps of the effect of temperature on residential mortality. (c) Cross-sectional plot of the impact of heat waves on residential mortality (RR values and 95% CI).

A daily maximum temperature above 92.5% for three consecutive days was used as a cutoff point to classify heat wave days and non-heat wave days. and Supplement Table 2 show the cut-plot and relative risk (RR) values of heat wave days on residential deaths in Fuzhou City at different lag days from 2016 to 2021. The RR of the impact of the heat wave on total non-accidental resident deaths reached a maximum on the day of the heat wave at 1.10% (1.07%, 1.14%); its impact persisted for 2–3 days and then disappeared (p < 0.05). And it also presents a higher risk at 1.11% (1.01%, 1.23%) in cumulative effects.
Effects of oxidising pollutants interacting with heat waves on mortality
shows a bivariate three-dimensional surface plot of the effects of oxidising pollutants and heat wave day temperature on the main causes of death. We found that the effects of oxidising pollutants on residential mortality were not entirely consistent at heat wave day temperature levels. The number of deaths increased with increasing concentrations of oxidising pollutants O3 and Ox and temperature; the maximum number of resident deaths was reached when both oxidising pollutant concentrations and temperature were at their maximum; NO2 had a strong effect at low pollutant concentrations, and the concentration played a major role.
Figure 3. Response to the effects of oxidising pollutants and temperature in major mortality. (a): O3, (b): Ox, (c): NO2.

and Supplement Table 3 present the current day lagged (lag0) and continuous cumulative (lag0–7) effects of each 10 μg/m3 increase in oxidising pollutant concentrations on excess residential mortality effects during the heat wave and non-heat wave periods. Compared to non-heat wave periods, O3 and Ox had significantly increased effects on cerebrovascular disease deaths on heat wave days and interacted with the heat wave, with lag0–7 risk (ER) values of −3.81% (−14.82%, 8.63%) and −3.00% (−20.80%, 18.79%), respectively; these values were significantly higher than those on non-heat wave days, for which no statistically significant difference was found. NO2 significantly increased the risk of excess mortality in the population on both heat wave and non-heat wave days; higher risks occurred on heat wave days, with lag0–7 risk (ER) values of 67.9% (11.55%, 152.71%) and 16.37% (2.43%, 32.20%), indicating a statistically significant difference. By calculating the upper limit of O3,NO2,Ox attributable risk due to the heatwave can be up to 6.82%, 120.51%, 15.75%.
Discussion
In our study, the effects of atmospheric oxidising pollutants and heat waves on the risk of residential mortality in Fuzhou were analysed by distributed lag nonlinear models and generalised additive models. Our research found a potential interactive effect of temperature and higher oxidising pollutant concentrations on the increased risk of resident deaths for which temperature played a major role, with the risk increasing with increasing temperature.
The evidence that oxidised pollutants increase the risk of population mortality is already strong. Our study found a positive correlation between increased O3, Ox and NO2 and the relative risk of death in the population, and NO2 remained relevant even at low concentrations. Studies in the USA found a positive correlation between O3 and the relative risk of total non-accidental mortality, and this correlation persisted at background concentrations [Citation11,Citation46], which is consistent with the findings of this study. In addition, our study suggested that low concentrations of O3 and NO2 still have a significant effect on mortality, and short-term exposure to oxidising pollutants can increase the risk of mortality. The cumulative lagged effect of air oxidising pollutants on the daily number of residential deaths in this study was stronger than the daily lagged effect, which is consistent with the findings of earlier studies [Citation21,Citation47].
Currently, in addition to research on oxidising pollutants, much interest has been given to the study of non-accidental deaths from heat waves. According to this study, the risk of death increased more noticeably and gradually as a result of high temperatures. High temperatures had an impact on residents’ likelihood of dying on all lag days, with the same day and second lag days having the biggest impact. The favourable effect persisted at a total lag of 14 days, with the largest lag occurring between the first and second day of the lag. This pattern is in line with the findings of the study by Berhanu et al. [Citation48], which found that high temperatures put a greater strain on people’s health.
As the climate crisis progresses, high temperatures and air pollution are expected to increase, with a high probability of occurring simultaneously, and health impacts will synergistically worsen beyond the sum of the individual effects of temperature and air pollution [Citation49]. The bivariate 3D model revealed that the interaction between O3 and Ox concentrations and temperature during heat waves was not significant for the risk of death, but the risk of death tended to increase with increasing temperature. While a study in Germany on the relationship between daily temperature and cause-specific mortality did not find an interaction between temperature and O3 [Citation50], a time-series study in China did find a relationship between hot weather and O3 on the risk of death from cerebrovascular disease in the population [Citation6].
In addition, the interaction results showed a synergistic effect between high temperatures and oxidising pollutants, although these results were not statistically significant. This finding is consistent with a previous study [Citation49]. But from the first to the third day of the lag between the heatwave interaction and the oxidative pollutant contaminant, we found that the risk was higher than on the non-heatwave days. On heat wave days, the body’s respiratory system becomes more taxed. As a result of the increased respiratory effort required in hot weather, more pollutants are inhaled, indirectly raising the human body’s exposure level. Because the biological mechanisms behind the effects of heat and pollution on human health are similar, the combined effects of exposure to both elements may be more detrimental to health than the effects of either element alone. For instance, O3 and Ox can increase platelet activation and blood pressure, suggesting a possible mechanism by which ozone may affect cardiovascular health [Citation51]. During heat wave days, the rise in temperature causes an increase in blood viscosity and cholesterol concentration, which is manifested as an increase in blood pressure [Citation52], which then increases blood flow in the skin; this situation exacerbates suffering from cardiovascular and cerebrovascular diseases [Citation53]. Therefore, both high temperature and oxidising pollutants can create oxidising stress and vascular damage, harming cardiovascular health and increasing the chance of death.
The interaction of heat waves and NO2 is also worth discussing. The bivariate 3D model analysis findings revealed that both higher and lower NO2 concentrations increased the risk of mortality for local residents, with the effect growing with higher concentrations; as temperature increased, the risk of mortality showed a slight decrease but still had a generally positive impact on mortality risk. The impact of heat wave days was further examined, and NO2 was discovered to increase the additional mortality risk on both heat wave and non-heat wave days. This finding is consistent with the results of a survey in China [Citation54] and a meta-analysis [Citation55] that did not detect an effect of temperature on NO2-related effects. Nevertheless, Corso et al.‘s findings [Citation56] also suggest that NO2 raises only the risk of mortality and excess mortality in hot weather. The study results are varied.
High temperatures, O3 and NO2 might truly work in concert. High temperatures, prolonged sunshine, and other meteorological factors aid the production of ozone [Citation57], the primary photochemical pollutant, which is created when atomic and molecular oxygen mix quickly. Ultraviolet radiation causes NO2 to breakdown into nitrogen oxides (NO) and atomic oxygen, a result of the photochemical production process of NO2 [Citation58]. In this situation, ozone can also react with NO to form NO2, which is another source of NO2 [Citation59]. Additionally, photochemical reactions release secondary organic aerosols that increase inflammatory responses, thus raising the risk of circulatory disease and health damage [Citation60].
In contrast to the results of previous studies of highly polluted areas [Citation34,Citation35], the results of this study reveal the interaction of heat waves and oxidising pollutants in cities with relatively low pollution levels and relatively high temperatures in China. However, there are non-significant results, may not be sufficiently persuasive, and we will continue to observe and improve our study in subsequent studies. This study may have exposure measurement errors because the analysed data, including temperature and oxidising pollutant concentrations, came from outdoor monitors, whereas people usually spend more time indoors in hot weather. A review shows proportional differences in indoor and outdoor concentrations of ozone [Citation61]. The results of the interaction effect may also be influenced by factors such as the socioeconomic level and behavioural habits of the study population because different geographical locations result in different climatic environments [Citation62,Citation63]. Therefore, interactions should be studied from as many angles as feasible.
Conclusion
In our study, exposure to heat waves and oxidising air pollutants were individually increased mortality risk; in addition, simultaneous exposure to both risk factors had a greater mortality impact than the sum of their individual effects, and that there is a stronger synergy between heat wave days and oxidative pollutants on humans than on non-heat wave days. Given that the frequency of heat waves and high pollution days is expected to increase as climate change progresses, assessing the health impacts of simultaneous exposure to air pollution and extreme heat events is urgent to better guide adaptation policies and interventions that can reduce mortality during extreme heat and oxidising pollution days.
Author contributions
N.R. and H.H. performed the main analysis and wrote the original draft. B.L., C.W., J.X., Q.Z., and S.K. contributed to the conception of the study and revised the manuscript. Y.J. and X.Z. revised the manuscript. All authors critically reviewed and accepted the final version of the manuscript. The authors read and approved the final manuscript.
Ethics and consent
The research received ethical approval from Fuzhou Center for Disease Control and Prevention, Bio- and Medical Ethics Committee (No: 2022003). We obtained verbal consent to enter the households and then written informed consent was obtained in the survey to ensure the authenticity of the study.
Paper context
As we known, oxidising pollutants and heat waves could both raise the risk of mortality. Then, we stratified temperature and found that the risk on heat wave days was significantly higher than non-heat wave days. In addition, simultaneous exposure to both risk factors had a greater mortality impact than the sum of their individual effects. The results may suggest interventions in southern coastal cities with hot but less polluted climates.
supplements_.docx
Download MS Word (25.7 KB)Acknowledgments
We sincerely thank all the participants in this study for their dedication.
Disclosure statement
No potential conflict of interest was reported by the author(s).
Data availability statement
Due to the nature of the research, due to ethical supporting data is not available.
Supplementary material
Supplemental data for this article can be accessed online at https://doi.org/10.1080/16549716.2024.2313340.
Additional information
Funding
References
- Watts N, Amann M, Arnell N, Ayeb-Karlsson S, Beagley J, Belesova K, et al. The 2020 report of the Lancet Countdown on health and climate change: responding to converging crises. Lancet. 2021;397:129–11. doi: 10.1016/S0140-6736(20)32290-X
- Lelieveld J, Pozzer A, Pöschl U, Fnais M, Haines A, Münzel T. Loss of life expectancy from air pollution compared to other risk factors: a worldwide perspective. Cardiovasc Res. 2020;116:1910–1917. doi: 10.1093/cvr/cvaa025
- Olstrup H, Johansson C, Forsberg B, Åström C. Association between mortality and short-term exposure to particles, ozone and nitrogen dioxide in Stockholm, Sweden. Int J Environ Res Public Health. 2019;16:1028. doi: 10.3390/ijerph16061028
- De Troeyer K, Bauwelinck M, Aerts R, Profer D, Berckmans J, Delcloo A, et al. Heat related mortality in the two largest Belgian urban areas: a time series analysis. Environ Res. 2020;188:109848. doi: 10.1016/j.envres.2020.109848
- Qin RX, Xiao C, Zhu Y, Li J, Yang J, Gu S, et al. The interactive effects between high temperature and air pollution on mortality: a time-series analysis in Hefei, China. Sci Total Environ. 2017;575:1530–1537. doi: 10.1016/j.scitotenv.2016.10.033
- Lee W, Choi HM, Kim D, Honda Y, Leon Guo Y-L, Kim H. Synergic effect between high temperature and air pollution on mortality in Northeast Asia. Environ Res. 2019;178:108735. doi: 10.1016/j.envres.2019.108735
- Shi W, Sun Q, Du P, Tang S, Chen C, Sun Z, et al. Modification effects of temperature on the ozone-mortality relationship: a nationwide multicounty study in China. Environ Sci Technol. 2020;54:2859–2868.
- Liu J, Varghese BM, Hansen A, Zhang Y, Driscoll T, Morgan G, et al. Heat exposure and cardiovascular health outcomes: a systematic review and meta-analysis. Lancet Planet Health. 2022;6:e484–e495. doi: 10.1016/S2542-5196(22)00117-6
- Vaidyanathan A, Malilay J, Schramm P, Saha S. Heat-related deaths - United States, 2004-2018. MMWR Morb Mortal Wkly Rep. 2020;69:729–734.
- Nassan FL, Kelly RS, Koutrakis P, Vokonas PS, Lasky-Su JA, Schwartz JD. Metabolomic signatures of the short-term exposure to air pollution and temperature. Environ Res. 2021;201:111553. doi: 10.1016/j.envres.2021.111553
- Di Q, Dai L, Wang Y, Zanobetti A, Choirat C, Schwartz JD, et al. Association of short-term exposure to air pollution with mortality in older adults. JAMA. 2017;318:2446–2456. doi: 10.1001/jama.2017.17923
- Crouse DL, Peters PA, Hystad P, Brook JR, van Donkelaar A, Martin RV, et al. Ambient PM2.5, O₃, and NO₂ exposures and associations with mortality over 16 years of follow-up in the Canadian Census Health and Environment Cohort (CanCHEC). Environ Health Perspect. 2015;123:1180–1186.
- Sanyal S, Rochereau T, Maesano CN, Com-Ruelle L, Annesi-Maesano I. Long-term effect of outdoor air pollution on mortality and morbidity: a 12-year follow-up study for metropolitan France. Int J Environ Res Public Health. 2018;15:2487. doi: 10.3390/ijerph15112487
- Liu Y, Pan J, Zhang H, Shi C, Li G, Peng Z, et al. Short-term exposure to ambient air pollution and asthma mortality. Am J Respir Crit Care Med. 2019;200:24–32. doi: 10.1164/rccm.201810-1823OC
- Dimitrova A, Ingole V, Basagaña X, Ranzani O, Milà C, Ballester J, et al. Association between ambient temperature and heat waves with mortality in South Asia: systematic review and meta-analysis. Environ Int. 2021;146:106170. doi: 10.1016/j.envint.2020.106170
- Marí-Dell’olmo M, Tobías A, Gómez-Gutiérrez A, Rodríguez-Sanz M, García de Olalla P, Camprubí E, et al. Social inequalities in the association between temperature and mortality in a South European context. Int J Public Health. 2019;64:27–37. doi: 10.1007/s00038-018-1094-6
- Green H, Bailey J, Schwarz L, Vanos J, Ebi K, Benmarhnia T. Impact of heat on mortality and morbidity in low and middle income countries: a review of the epidemiological evidence and considerations for future research. Environ Res. 2019;171:80–91. doi: 10.1016/j.envres.2019.01.010
- Ebi KL, Capon A, Berry P, Broderick C, de Dear R, Havenith G, et al. Hot weather and heat extremes: health risks. Lancet. 2021;398:698–708. doi: 10.1016/S0140-6736(21)01208-3
- Howe PD, Marlon JR, Wang X, Leiserowitz A. Public perceptions of the health risks of extreme heat across US states, counties, and neighborhoods. Proc Natl Acad Sci U S A. 2019;116:6743–6748. doi: 10.1073/pnas.1813145116
- Song J, Pan R, Yi W, Wei Q, Qin W, Song S, et al. Ambient high temperature exposure and global disease burden during 1990-2019: an analysis of the global burden of disease study 2019. Sci Total Environ. 2021;787:147540.
- Orellano P, Reynoso J, Quaranta N, Bardach A, Ciapponi A. Short-term exposure to particulate matter (PM10 and PM2.5), nitrogen dioxide (NO2), and ozone (O3) and all-cause and cause-specific mortality: systematic review and meta-analysis. Environ Int. 2020;142:105876. doi: 10.1016/j.envint.2020.105876
- Zhang Y, Tian Q, Feng X, Hu W, Ma P, Xin J, et al. Modification effects of ambient temperature on ozone-mortality relationships in Chengdu, China. Environ Sci Pollut Res Int. 2022;29:73011–73019. doi: 10.1007/s11356-022-20843-5
- Jhun I, Fann N, Zanobetti A, Hubbell B. Effect modification of ozone-related mortality risks by temperature in 97 US cities. Environ Int. 2014;73:128–134. doi: 10.1016/j.envint.2014.07.009
- Lim CC, Hayes RB, Ahn J, Shao Y, Silverman DT, Jones RR, et al. Long-term exposure to ozone and cause-specific mortality risk in the United States. Am J Respir Crit Care Med. 2019;200:1022–1031. doi: 10.1164/rccm.201806-1161OC
- Schwarz L, Hansen K, Alari A, Ilango SD, Bernal N, Basu R, et al. Spatial variation in the joint effect of extreme heat events and ozone on respiratory hospitalizations in California. Proc Natl Acad Sci U S A. 2021;118. doi: 10.1073/pnas.2023078118
- Grigorieva E, Lukyanets A. Combined effect of hot weather and outdoor air pollution on respiratory health: literature review. Atmosphere. 2021 Jun;12:790. doi: 10.3390/atmos12060790
- Kuźma Ł, Struniawski K, Pogorzelski S, Bachórzewska-Gajewska H, Dobrzycki S. Gender differences in association between air pollution and daily mortality in the capital of the green lungs of Poland-population-based study with 2,953,000 person-years of follow-up. J Clin Med. 2020;9(8): 2351.
- Miller MR. Oxidative stress and the cardiovascular effects of air pollution. Free Radic Biol Med. 2020 May 1;151:69–87. doi: 10.1016/j.freeradbiomed.2020.01.004
- Christidis T, Erickson AC, Pappin AJ, Crouse DL, Pinault LL, Weichenthal SA, et al. Low concentrations of fine particle air pollution and mortality in the Canadian community health survey cohort. Environ Health. 2019;18:84. doi: 10.1186/s12940-019-0518-y
- Brito J, Bernardo A, Zagalo C, Gonçalves LL. Quantitative analysis of air pollution and mortality in Portugal: Current trends and links following proposed biological pathways. Sci Total Environ. 2021;755:142473. doi: 10.1016/j.scitotenv.2020.142473
- Li M, Dong H, Wang B, Zhao W, Zare Sakhvidi MJ, Li L, et al. Association between ambient ozone pollution and mortality from a spectrum of causes in Guangzhou, China. Sci Total Environ. 2021;754:142110. doi: 10.1016/j.scitotenv.2020.142110
- Faustini A, Stafoggia M, Williams M, Davoli M, Forastiere F. The effect of short-term exposure to O3, NO2, and their combined oxidative potential on mortality in Rome. Air Qual Atmos Health. 2019;12:561–571. doi: 10.1007/s11869-019-00673-0
- Ji S, Zhou Q, Jiang Y, He C, Chen Y, Wu C, et al. The interactive effects between particulate matter and heat waves on circulatory mortality in Fuzhou, China. Int J Environ Res Public Health. 2020;17:5979. doi: 10.3390/ijerph17165979
- Gao H-Y, Liu X-L, Lu Y-K, Liu Y-H, Hu L-K, Li Y-L, et al. Short-term effects of gaseous air pollutants on outpatient visits for respiratory diseases: a case-crossover study in Baotou, China. Environ Sci Pollut Res Int. 2022;29:49937–49946. doi: 10.1007/s11356-022-19413-6
- Song X, Jiang L, Wang S, Tian J, Yang K, Wang X, et al. The impact of main air pollutants on respiratory emergency department visits and the modification effects of temperature in Beijing, China. Environ Sci Pollut Res Int. 2021;28:6990–7000. doi: 10.1007/s11356-020-10949-z
- Fu W, Chen Z, Zhu Z, Liu Q, van den Bosch CCK, Qi J, et al. Spatial and temporal variations of six criteria air pollutants in Fujian province, China. Int J Environ Res Public Health. 2018;15:2846. doi: 10.3390/ijerph15122846
- Xu Z, FitzGerald G, Guo Y, Jalaludin B, Tong S. Impact of heatwave on mortality under different heatwave definitions: a systematic review and meta-analysis. Environ Int. 2016;89-90:193–203. doi: 10.1016/j.envint.2016.02.007
- Chen H, Zhao L, Cheng L, Zhang Y, Wang H, Gu K, et al. Projections of heatwave-attributable mortality under climate change and future population scenarios in China. Lancet Reg Health West Pac. 2022;28:100582.
- Yang J, Yin P, Sun J, Wang B, Zhou M, Li M, et al. Heatwave and mortality in 31 major Chinese cities: definition, vulnerability and implications. Sci Total Environ. 2019 Feb 1;649:695–702. doi: 10.1016/j.scitotenv.2018.08.332
- Cheng Z, Wang S, Jiang J, Fu Q, Chen C, Xu B, et al. Long-term trend of haze pollution and impact of particulate matter in the Yangtze River Delta, China. Environ Pollut. 2013;182:101–110. doi: 10.1016/j.envpol.2013.06.043
- Di Q, Wang Y, Zanobetti A, Wang Y, Koutrakis P, Choirat C, et al. Air pollution and mortality in the medicare population. N Engl J Med. 2017;376:2513–2522. doi: 10.1056/NEJMoa1702747
- Lee JT, Kim H, Hong YC, Kwon HJ, Schwartz J, Christiani DC. Air pollution and daily mortality in seven major cities of Korea, 1991-1997. Environ Res. 2000;84:247–254.
- Bell ML, Samet JM, Dominici F. Time-series studies of particulate matter. Annu Rev Public Health. 2004;25:247–280. doi: 10.1146/annurev.publhealth.25.102802.124329
- Chen R, Yin P, Wang L, Liu C, Niu Y, Wang W, et al. Association between ambient temperature and mortality risk and burden: time series study in 272 main Chinese cities. BMJ. 2018;363:k4306. doi: 10.1136/bmj.k4306
- Mukaka MM. Statistics corner: a guide to appropriate use of correlation coefficient in medical research. Malawi Med J. 2012;24:69–71.
- Bell ML, Peng RD, Dominici F. The exposure-response curve for ozone and risk of mortality and the adequacy of current ozone regulations. Environ Health Perspect. 2006;114:532–536.
- Chen C, Li T, Sun Q, Shi W, He MZ, Wang J, et al. Short-term exposure to ozone and cause-specific mortality risks and thresholds in China: evidence from nationally representative data, 2013-2018. Environ Int. 2023;171:107666. doi: 10.1016/j.envint.2022.107666
- Wondmagegn BY, Xiang J, Dear K, Williams S, Hansen A, Pisaniello D, et al. Increasing impacts of temperature on hospital admissions, length of stay, and related healthcare costs in the context of climate change in Adelaide, South Australia. Sci Total Environ. 2021;773:145656. doi: 10.1016/j.scitotenv.2021.145656
- Rahman MM, McConnell R, Schlaerth H, Ko J, Silva S, Lurmann FW, et al. The effects of coexposure to extremes of heat and particulate air pollution on mortality in California: implications for climate change. Am J Respir Crit Care Med. 2022;206:1117–1127. doi: 10.1164/rccm.202204-0657OC
- Breitner S, Wolf K, Devlin RB, Diaz-Sanchez D, Peters A, Schneider A. Short-term effects of air temperature on mortality and effect modification by air pollution in three cities of Bavaria, Germany: a time-series analysis. Sci Total Environ. 2014;485-486:49–61. doi: 10.1016/j.scitotenv.2014.03.048
- Day DB, Xiang J, Mo J, Li F, Chung M, Gong J, et al. Association of ozone exposure with cardiorespiratory pathophysiologic mechanisms in healthy adults. JAMA Intern Med. 2017;177:1344–1353. doi: 10.1001/jamainternmed.2017.2842
- Haines A, Patz JA. Health effects of climate change. JAMA. 2004;291(1):99-103.
- Ren C, O’Neill MS, Park SK, Sparrow D, Vokonas P, Schwartz J. Ambient temperature, air pollution, and heart rate variability in an aging population. Am J Epidemiol. 2011;173:1013–1021. doi: 10.1093/aje/kwq477
- Luo K, Li R, Wang Z, Zhang R, Xu Q. Effect modification of the association between temperature variability and daily cardiovascular mortality by air pollutants in three Chinese cities. Environ Pollut. 2017;230:989–999. doi: 10.1016/j.envpol.2017.07.045
- Song X, Wang S, Hu Y, Yue M, Zhang T, Liu Y, et al. Impact of ambient temperature on morbidity and mortality: an overview of reviews. Sci Total Environ. 2017;586:241–254. doi: 10.1016/j.scitotenv.2017.01.212
- Corso M, Blanchard M, Medina S, Wagner V. Short-term associations of nitrogen dioxide (NO2) on mortality in 18 French cities, 2010-2014. Atmosphere. 2020 Nov;11(11),1198.
- Bai L, Feng J, Li Z, Han C, Yan F, Ding Y. Spatiotemporal dynamics of surface ozone and its relationship with meteorological factors over the Beijing-Tianjin-Tangshan region, China, from 2016 to 2019. Sens (Basel). 2022;22(13):4854.
- Areal AT, Zhao Q, Wigmann C, Schneider A, Schikowski T. The effect of air pollution when modified by temperature on respiratory health outcomes: a systematic review and meta-analysis. Sci Total Environ. 2022;811:152336. doi: 10.1016/j.scitotenv.2021.152336
- Raff JD, Njegic B, Chang WL, Gordon MS, Dabdub D, Gerber RB, et al. Chlorine activation indoors and outdoors via surface-mediated reactions of nitrogen oxides with hydrogen chloride. Proc Natl Acad Sci USA. 2009;106:13647–13654. doi: 10.1073/pnas.0904195106
- Diaz EA, Chung Y, Papapostolou V, Lawrence J, Long MS, Hatakeyama V, et al. Effects of fresh and aged vehicular exhaust emissions on breathing pattern and cellular responses–pilot single vehicle study. Inhal Toxicol. 2012;24:288–295. doi: 10.3109/08958378.2012.668572
- Nazaroff WW, Weschler CJ. Indoor ozone: concentrations and influencing factors. Indoor Air. 2022 Jan;32:e12942. doi: 10.1111/ina.12942
- Khajavi A, Khalili D, Azizi F, Hadaegh F. Impact of temperature and air pollution on cardiovascular disease and death in Iran: a 15-year follow-up of Tehran lipid and glucose study. Sci Total Environ. 2019;661:243–250. doi: 10.1016/j.scitotenv.2019.01.182
- Luo K, Li R, Li W, Wang Z, Ma X, Zhang R, et al. Acute effects of nitrogen dioxide on cardiovascular mortality in Beijing: an exploration of spatial heterogeneity and the district-specific predictors. Sci Rep. 2016;6:38328. doi: 10.1038/srep38328