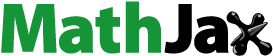
Abstract
Amorphous glasses in the system xCdO-(100-x)(yNa2O + zP2O5), (x = 0, 10, 20, 30, 40, 42.5, 45, y = z) mol% are prepared by a fast quenching technique. Addition of CdO at the expense of equal amounts of both P2O5 and Na2O causes a substantial influence on the distinctive feature of the measured Raman spectra. The concentration of nonbridging oxygen atoms (NBO) rises with increasing CdO content. The numerical data from the deconvolution of Raman spectra revealed that Q3 (branching group) and Q2 (metaphosphate group) decline while Q1 (pyrophosphate group or end group) increases. The rise in the number of NBO in terms of Q1 is supported by the change in molar volume, free volume, and packing density. This result is compatible with Raman and NMR spectroscopic observations. Increased CdO content causes a rise in the O/P ratio, which causes an increase in NBO in the glass network which lowers the glass’s hardness number.
1. Introduction
When modifier oxides are added to P2O5 [Citation1–4] or TeO2 and SiO2 [Citation5–7], non-bridged oxygen (NBO) should develop in the host glass network. To achieve the crystalline phases created by NBO in the glass network, large amounts of modifier oxides such as alkali or alkaline earth oxides are required. This activity produces many complexations that necessitate delaying or limiting the formation of simple amorphous phases in the host glass network [Citation2,Citation8]. This effect could be ascribed to the alkali oxides’ strong modifying role. To produce crystalline clusters from the alkali (Na) alone, for example, a considerable amount of the Na2O should be used as a modifier and the rest as a former, particularly at its highest concentration. These species can reduce the number of phases that lead to the formation of crystalline clusters. In such a case the concentration of NBO should be high enough in order to produce crystalline phases with a wide range of applications [Citation2–8]. Then, to get the largest concentration from the well-formed phases, NBO production must be maintained, especially in the phosphate glass network with two types of modifiers, such as Na2O and CdO. The interaction of the two modifiers (Na and Cd) can significantly increase the amount of NBO while avoiding the formation of crystalline species in different types of glass systems [Citation2,Citation5,Citation7,Citation9].
Several authors studied glassy matrix and referred to invert glasses when the properties of the studied glass matrix are more governed by the percentage of network modifier rather than the anionic skeleton [Citation10–12].
The purpose of this study is to reduce P2O5 and Na2O while increasing CdO to solve the problem of delaying the formation of amorphous phases. To produce an inverted process in the structure and characteristics, modifier oxides are introduced to the glass structure satisfying that the majority of the NBO in the glass network must be electrically balanced with Cd2+ ions rather than Na+ ions due to the inversion process [Citation2]. When the structural units are balanced with CdO, the obtained structure should limit the toxicity produced by Cd ions. Such glasses can be used in solid state Cd-batteries and in bioapplication to avoid the expected toxicity. This mechanism compels the majority of freshly added CdO to act as a modifier, which is subsequently used to produce an increasing amount of NBO [Citation2,Citation9]. The novelty in this work is considered in terms of how expanding the region of the amorphosity which can affect the building of specific inverted glass phases suitable for many applications such as Cd-batteries [Citation2,Citation6–9].
2. Experimental details
Glassy samples of the composition xCdO-(100-x)(yNa2O + zP2O5), (x = 0, 10, 20, 30, 40, 42.5, 45, y = z) mol% were synthesized using the universal melt quenching technique. Cadmium carbonate and sodium carbonate supplied by Rayasan chemicals were used as sources for cadmium and sodium oxides while ammonium dihydrogen orthophosphate supplied by Sigma Aldrich Co. was used as a source of phosphorous pentoxide. Calculated weights were mixed and melted in porcelain crucibles via a muffle furnace. Powders were dried at 350°C for an hour to ensure the complete release of carbon dioxide and ammonia. The temperature was then raised until melting to a temperature ranging between 1000 and 1100°C depending on the glass composition. The melts were swirled several times to ensure homogeneity and bubbles-free samples.
Raman spectra of the studied samples were recorded via WiTec 300 R, Raman scope (Germany) operating with a 532 excitation laser line. For each scan, multiple acquisitions were taken within the range extended from 100 to 1500 cm−1.
3. Results and discussion
3.1. Raman spectroscopy
XRD patterns of all as-prepared samples have confirmed the amorphous structure which had been confirmed in our previous study [Citation2]. Raman spectroscopy is one of the most important techniques to determine the structure, environment, and dynamics of diatomic and polyatomic molecules of glass networks [Citation13]. The Raman spectra of cadmium sodium phosphate samples at room temperature are shown in Figure . From the Raman spectra, it is observed that the bands due to modifier vibration (Na and Cd ions) are identified in the wavelength range of 380–580 cm−1. There are two envelopes in glasses of 10, 20, and 30 CdO that are observed around 350–470 cm−1. One is due to Na-O and the other is due to Cd-O vibration as modifiers. The Raman band between 650–950 cm−1 is related to bridging and non-bridging anion vibrational modes [Citation14]. Individuals and groups of bonds are shown by each peak, which corresponds to a distinct molecular bond vibration [Citation13–16]. For instance, metaphosphate (phosphate units with two NBO, denoted as Q2) has a spectrum that is nearly identical to that of a glass containing 0 mol% CdO [Citation2,Citation9]. Because the substitution of P2O5 and Na2O with CdO has a considerable impact on the distinguishing aspects of the measured Raman spectra, the discrepancies between the Raman spectra of CdO-free glass and glasses with a suitably high concentration of CdO demonstrate that CdO plays an effective function.
The peak located around 1300 cm−1 gradually diminishes with increasing CdO concentration, as indicated in the figure. A new absorbance peak forms at 1000 cm−1, and its relative area grows until it reaches its maximum value at 45 mol% CdO. With increasing CdO concentration, the speak at 1160 cm−1 broadens and shifts to a lower wavenumber. Phosphate units having two nonbridging bonds (2NBO) per phosphate building group, known as Q2, may be responsible for the main Raman peaks at 1300 cm−1. This peak has disappeared due to an increase in the concentration of NBO atoms around phosphate units, and a new one has formed at around 1000 cm−1. Furthermore, in the majority of the studied glasses, the presence of an additional resonance peak at (1000 cm−1) implies the creation of phosphate units with NBO in the form of (Q1) [Citation2,Citation12–14]. As the amount of CdO in the structure grows, the amount of NBO in the phosphate glass structure increases [Citation2,Citation16–19].
The most to be noted from the analysis is that with increasing CdO, the peak intensity of around 1300 cm−1 is decreased and the peak at 1150 cm−1 is shifted to a lower wavenumber leading to further transformation of Q2 to Q1 . From the analysis of Raman spectra, it is noticed that as the concentration of CdO is increased from 0 to 45, the peak intensity of Q2 decreases, and Q1 is formed. All these facts are consistent with the results of the NMR and infrared spectra of the previously reported study on the same glass compositions [Citation2].
Figure shows many absorbed peaks from the investigated spectra for all analysed composition (0–45 mol% CdO). Each Qn fraction may be estimated after peak deconvolution. The fractional structural component restricting the examined glasses is referred to as Qn. The structure with the largest Q2/Q1 ratio [Citation2,Citation17,Citation18] is created in the case of sodium-rich glass since ratio Q2/Q1 drops when Na2O and P2O5 are substituted with CdO. The variations in the percentages of Q2, Q3, and Q1 with increasing CdO concentration are mostly attributable to the production of additional NBO associated with CdO addition, as evidenced by prior findings based on both FTIR and NMR spectroscopy for the same glasses [Citation2]. The decrease in phosphate units in the metaphosphate (Q2) and the increase in phosphate units in the form of Q1 as pyrophosphate group of end group structure, which includes more NBO atoms, are connected to the change in the relative area representing Qn with CdO [Citation17–19]. The results of the deconvolution findings are shown in Figure .
3.2. Molar volume, density, and microhardness
Figure shows the decrease in the observed density of the as-prepared samples. As the CdO concentration grew from 0 to 45 mol%, the density values obtained increased from 2.6 to 4 g/cm3, whereas the molar volume (Vm) fell from 38.8 to 29.5 cm3/mol. The density fluctuations of the glass samples under investigation can be explained in two ways: (1) Modification of the molecular network and changes in molecular mass. The heavier CdO has replaced the lighter Na2O and P2O5 which has the main effect in an increase in density. However, the decrease in molar volume is because increasing CdO concentration increases both the total modifier and the NBO atom. As a result, the lengthy chain of P2O5 is destroyed in this circumstance, and individual phosphate units of lower sizes are created. The measured molar volume drop was around 10 cm3/mol, which might be attributed to the phosphate unit change from a longer metaphosphate chain to shorter phosphate units [Citation2,Citation3,Citation6,Citation9]. Because oxygen contributes the most to the volume of the oxygen polyhedra in the glass network, the volume change of Cd2+ and P5+ is negligible in comparison to O2. As a result, it’s plausible to assume that as the concentration of Q1 rises at the expense of greater volume Q2 units, the molar volume drops, and the density rises (Figure ). The change in molar volume in this situation, then, could be attributed to an increase in the number of NBO in the phosphate units, which is consistent with FTIR and NMR spectroscopy results [Citation2,Citation4]. Table and Figures summarize the changes in density D, molar volume Vm, free volume Vf and the packing density Pd with changes in the glass composition.
Table 1. D, Vm, Vf, and Pd changes with CdO content.
Figure shows the changes in both free volume (Vf) and the packing density (Pd) as functions of CdO content. Vf and Pd are respectively expressed as
Figure 5. Changes of both free volume (Vf) and the packing density (Pd) as functions of CdO content.

ΣxiVi is the total volume of the ions in the glass, calculated from their ionic radii. With increasing CdO content, the value of packing density rises while the value of free volume falls. This could be due to the creation of more open and less linked bonds in the glass matrix as the CdO concentration rises. The changes of D, Mv, Vf, and pd were faster in the region between 40 and 45 mol% CdO which means that Q2 units are transformed to Q1 units of the higher free spaces. At lower concentrations, mixed phosphate structural units (Q2 and Q1) are the dominant species.
The variation in Vickers hardness (Hv) with CdO content is depicted in Figure . The results reveal that the Hv of the tested glasses is affected by the presence of CdO. The hardness number decreases as CdO increases, with hardness values dropping from around 412 to 261 kg/mm2 as CdO content rises from 0 to 45%. The decrease in the cross-linked bond of bulky glass is connected to the decrease in the hardness number. These findings corroborate the FTIR and density findings, indicating that adding CdO to phosphate units reduces the number of bridging links between them. The addition of CdO can weaken the glass network, as the more resistant P–O–P bonds are replaced with weaker non-bridging oxygen [Citation2,Citation3,Citation9]. These findings are in line with the FTIR, NMR, and density findings, which suggest that adding CdO to the glass network weakens it by replacing the more robust P–O–P bonds with the weaker one containing more non-bridging oxygen. Increased CdO content increases the O/P ratio, which raises NBO in the glass network, resulting in a less toughened and weaker glassy structure [Citation17–20].
4. Conclusion
Glasses in the system xCdO-(100-x)(yNa2O + zP2O5), (x = 0, 10, 20, 30, 40, 42.5, 45, y = z) mol% have been prepared by the fast quenched technique. The exchange of both P2O5 and Na2O with CdO has a real effect on the distinctive feature of the measured Raman spectra. The concentration of nonbridging oxygen atoms (NBO) rises with increasing CdO concentration. The results based on the deconvolution process revealed that Q3 and Q2 decline while Q1 increases. The change in molar volume, free volume, and packing density in this circumstance is compatible with Raman and NMR spectroscopic observations. Increased CdO content causes a rise in the number of nonbridging oxygen atom, which lowers the glass’s hardness number.
Disclosure statement
No potential conflict of interest was reported by the author(s).
References
- Möncke D, Eckert H. Review on the structural analysis of fluoride-phosphate and fluoro-phosphate glasses. J Non Cryst Solids. 2019;3:100026. DOI:10.1016/j.nocx.2019.100026
- El-Damrawi G, Zahran HM, Abdelghany AM. Cadmium invert sodium phosphate glasses: a structural peculiarities. J Taibah Univ Sci. 2021;15(1):1123–101129. DOI:10.1080/16583655.2021.2020437
- El Damrawi G, Hassan AK, Shahboub A. Chemical durability and structure of Al2O3–Ag2O–P2O5 glasses. Appl Phys A. 2020;126(4):1–7. DOI:10.1007/s00339-020-3451-6
- Brow RK. The structure of simple phosphate glasses. J Non Cryst Solids. 2000;263:1–28.
- Kaky KM, Lakshminarayana G, Baki SO, et al. Structural, thermal and optical absorption features of heavy metal oxides doped tellurite rich glasses. Results Phys. 2017;7:166–174. DOI:10.1016/j.rinp.2016.12.013
- Damrawi GE, Ramadan RM, Baiomy ME. Structural role of strontium oxide in modified silicate glasses. Silicon. 2022;14: 8733–8743. DOI:10.1007/s12633-021-01553-y.
- Nazrin SN, Halimah MK, Muhammad FD, et al. The effect of erbium oxide in physical and structural properties of zinc tellurite glass system. J Non Cryst Solids. 2018;490:35–43. DOI:10.1016/j.jnoncrysol.2018.03.017
- Asyikin AS, Halimah MK, Latif AA, et al. Physical, structural and optical properties of bio-silica borotellurite glass system doped with samarium oxide nanoparticles. J Non Cryst Solids. 2020;529:119777. DOI:10.1016/j.jnoncrysol.2019.119777
- El Damrawi G, Abdelghany M, Mnaaa E. Nuclear magnetic resonance studies on vanadium phosphate glasses. Magnet Reson Solid Electron J. 2019;21(5):19504. DOI:10.26907/mrsej-19504
- Walter G, Vogel J, Hoppe U, et al. The structure of CaO–Na2O–MgO–P2O5 invert glass. J Non Cryst Solids. 2001;296(3):212–119223. DOI:10.1016/S0022-3093(01)00912-7
- Abdelghany AM, Ghoneim NA, ElBatal HA. Characterization of invert soda lime silica glasses containing high titania content together with their glass ceramics. Silicon. 2018;10(3):1035–1043. DOI:10.1007/s12633-017-9566-5
- Abdelghany AM, Behairy A. Optical parameters, antibacterial characteristics and structure correlation of copper ions in cadmium borate glasses. J Mater Res Technol. 2020;9(5):10491–10497. DOI:10.1016/j.jmrt.2020.07.057
- Nakamoto K. Infrared & Raman spectra of inorganic & coordination compounds. 4th ed. New York: John Wiley; 1992.
- Mott NF, Davis EA. Electronic processes in non-crystalline materials. Oxford: Clarendon Press; 1971.
- Pask HM, Mildren RP, Piper JA. Solid-state Raman lasers: efficient multi-wavelength lasers for the green-yellow-red region. In Australian institute of physics national congress (16th: 2005). Sydney, Australian National University; 2005.
- Elbashar Y, Hussien S, Khaliel J, et al. Infrared spectroscopic analysis of cadmium doped sodium zinc phosphate glass matrix. Nonlin Opt Quant Opt Concept Modern Opt. 2021;54:105–114.
- Abed H, El Damrawi G, El Sherbiny M, et al. A new focus on the structure and mechanics of CuO-modified P2O5-V2O5 glasses. J Non Cryst Solids. 2021;568:120959. DOI:10.1016/j.jnoncrysol.2021.120959
- El-Damrawi G, Abou Elzahab M, Dowadair A, et al. Electron paramagnetic resonance study on phosphosilicate glasses. Magnet Resonan Solid Electron J. 2019;21(1):2–2.
- Atef N, El Damrawi G, Hassan A, et al. Dielectric Studies on CuO-Na2O-B2O3 Glasses. New J Glass Ceramic. 2020;10(4):45. DOI:10.4236/njgc.2020.104004
- Elbashar Y, Hussien S, Khaliel J, et al. Infrared spectroscopic analysis of cadmium doped sodium zinc phosphate glass matrix. Nonlin Opt Quant Opt Concept Mod Opt. 2021;54:105–114.