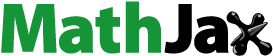
Abstract
In the present work, the influence of the nature of the plant extract on the synthesis of nanostructured ZnO via green chemistry was investigated. Two plant extracts were tested: Artemisia and Hibiscus. We noticed that Hibiscus plants prevented the growth of a film on the substrate, but resulted in nanoparticle formation. However, the Artemisia plant extract favours ZnO nanorod's growth on the substrate. We concluded that the low viscosity of Hibiscus favours homogeneous precipitation, whereas Artemisia, due to its larger viscosity, favours heterogeneous condensation on the substrate.
Highlights
The influence of the nature of the plant extract on the synthesis of nanostructured ZnO, that is, nanoparticles and nanorods, via green chemistry was investigated.
Two plant extracts were tested: Artemisia and Hibiscus.
Hibiscus plants prevented the growth of a film on the substrate but resulted in nanoparticle formation.
Artemisia plant extract favoured ZnO nanorods growth on the substrate.
This difference can be explained by the plant extract control of the condensation process in the solution.
1. Introduction
Nowadays, there is a growing interest in nanoscience and nanotechnology, and this leads to a rapid increase in the research activity in the development of the synthesis, characterization, and the application of several nanostructured materials [Citation1–8]. In this dynamic, a large variety of nanostructured semiconductors have been explored. For the synthesis of nanostructured materials, two major techniques are adopted for the starting material: (i) top-down and (ii) bottom-up. The former is expensive and requires sophisticated equipment. The second method is based mainly on chemical processes [Citation9, Citation10].
Various nanostructures with different shapes and sizes of metallic oxide materials have been synthesized using bottom-down techniques including hydrothermal, sol–gel, chemical bath, and electrodeposition. A large number of review papers on the different nanomaterial’s synthesis and applications are recently published [Citation11–17].
The green chemistry method has emerged in recent years as a simple and safe nanomaterial preparation method [Citation18, Citation19]. In this approach, plant extracts were used as the solvents. For green synthesis, a large variety of plants have been investigated. In general, plant roots, leaves, and flowers are used for plant extract preparation [Citation20]. The phytochemical radicals present in the plant extract act as capping or stabilization agents, leading to nanoparticles with morphology and size [Citation21]. They may alter the physical properties of the solution, such as viscosity and surface tension alloying, leading to better control of the nanoparticle growth [Citation22].
Zinc oxide (ZnO), is an n-type semiconductor owing to a wide direct band gap of 3.37 eV, its unique piezoelectric, and optical properties qualify it as a promising candidate semiconductor for several applications in different technological fields [Citation23–31].
ZnO nanoparticles have been synthesized using a large variety of plant leaf extracts such as China rose (Hibiscus rosa-sinensis) [Citation32], Green tea (Camellia sinensis) [Citation33], aloe leaf broth extract (Aloe barbadensis Miller) [Citation34], Calotropis gigantean [Citation35], Aloe barbadensis [Citation36], Achiella millefolium l [Citation37], Cayratia pedata leaf [Citation38] Thyme plant leaf [Citation39], and Coriandrum sativum [Citation40]. The quasi totally of these green method studies deal only with the nanoparticle synthesis. Yet, as far as we know, no study of ZnO nanorod synthesis via green chemistry was reported in the literature.
In this study, ZnO nanostructures were grown using the green chemistry method. For this purpose two plants Hibiscus and Wormwood (Artemisia absinthium) extract are investigated. A comparison between the produced nanostructures' shape is addressed.
2. Experimental details
Hibiscus and Artemisia flowers were finely cut and ground, the produced powder was washed with distilled water for impurities removal. The plant extract solution was prepared by boiling 10 g of the obtained powder in 100 ml of distilled water, at 70°C for about 30 min, until the solution changes its colour. Indeed, ZnO nanorods cannot be formed on seed-free substrates because of the lattice mismatch between the substrate and nanorods. Therefore, a seed layer is essential to produce well-aligned nanorods. Therefore, ZnO nanorods growth can be achieved via a two-step process: (a) ZnO seed layer deposition on the glass substrate and (b) ZnO nanorods arrays growth in a chemical solution. ZnO thin films were first deposited, on glass substrates at ambient temperature, by DC sputtering.
In the second step, with the aim of ZnO nanorods synthesis, two solutions were prepared with 80 ml of a solution composed of 0.05 M anhydride zinc chloride, ZnCl2, and 0.05 M hexamethylenetetramine (HTMA C6H12N4) mixed with 20 ml of the previously prepared plant extract of Artemisia and Hibiscus. The pH of the solution was 7.6. Thereafter, seeded substrates are immersed vertically, during 2 h, in a beaker filled with the prepared solution. is a schematic drawing of the synthesis diagram used in this study.
Figure 1. Schematic representation of green synthesis process of ZnO nanostructures using the Hibiscus and Artemisia plant extract.

The obtained structure of the nanostructures is characterized by the beam of x rays diffraction (XRD), (X’pert Pro) with Cu Kα line in 2θ−θ configuration. The sample morphology was characterized using a field-emission electron microscope (JSM-7600F, JEOL) instrument equipped with an EDX spectrometer for elemental composition analysis.
3. Results and discussion
(a) represents the SEM image of the nanorods arrays formed on the glass substrate when using Artemisia plant extract. As can be seen, the ZnO NRs are grown vertically along the c-axis, they have a dense distribution with a uniform diameter. The nanorod's top edge has a conic form, a similar feature has been recently observed by Aithssi et al. [Citation41] and Tihane et al. [Citation42] in ZnO nanorods prepared by the electrodeposition technique. As shown, the nanorods were terminated by a bundle of small nanorods. A similar structure was observed by Wahid et al. [Citation43], who noticed homocentric bundling of ZnO NRs formation on the top of ZnO nanorods. The EDX sample composition ((b,c)) reveals that the synthesized is composed of 55% oxygen and 41% of Zn, the excess of oxygen is due to oxygen elements originating from the used glass substrate as well the Si and Pt signals.
Figure 2. (a) SEM image of the grown ZnO nanorods on glass substrate when using Artemisia plant extract. (b) EDX composition spectra and (c) different elements composition ratio.

In the case of the Hibiscus extract, we did not observe the formation of any deposit, except for a small agglomeration on the glass substrate. (a) is the SEM image of the glass substrate after immersion in Hibiscus plant extract. The EDX ((b,c)). The composition spectrum of the sample is composed of glass substrate elements with a small amount of zinc originating from the stacked agglomeration and the ZnO seed thin film layer, confirming thereafter, the formation of nanopowder rather than film deposition on the substrate.
Figure 3. (a) SEM image of the ZnO agglomeration and nanoparticles adsorbed on glass substrate when using the Hibiscus plant extract, (b) EDX composition spectra, and (c) different elements composition ratio.

In the case of Hibiscus, we observed nanopowder precipitation instead of film growth on the substrate. In (a,b) we have reported the SEM and TEM images of the harvested nanopowder, and it can be seen that the synthesized nanopowder is composed of well-distributed spherical nanoparticles with a diameter of 50 nm in size.
Figure 4. (a) SEM and (b) TEM images of the precipitated nanoparticles obtained with Hibiscus plant extract.

This suggests that the plant extract alters the properties of the solution and controls the species' condensation process. Artemisia plant extract favours heterogeneous condensation, that is, film formation, whereas Hibiscus plant extract favours homogeneous condensation, that is, powder formation.
shows the XRD patterns of the produced nanostructures using the different plant extracts. The patterns of the nanorods produced by using Artemesia are composed of three diffraction peaks due to the planes (002), (101), (102), and (103) diffraction assigned to the Wurtzite hexagonal structure of ZnO according to the JCPDS card no. 36-1451. The peak of the (002) plane is the most intense, suggesting that the formed nanorods grow normally to the substrate along the vertical c-axis.
Figure 5. XRD diffraction pattern of the grown ZnO nanorods with Artemisia plant extract and nanoparticles obtained with Hibiscus plant extract.

The nanostructure formed using the Hibiscus plant extract has a polycrystalline nature, as can be deduced from its diffraction pattern formed with several peaks such (100),(002), (101), (102), (110), (103), and (112) assigned to the ZnO phase nanopowder.
The crystallites size D, the strain ε, and the density of the lien are parameters that can be extracted from the diffraction peak. The crystallites size has been determined using the Scherer formula [Citation44]:
(1)
(1)
where β is the full width at the half maximum of the peak, θ is the diffraction angle of the peak, λ is the wavelength of the λCu ray (Cu) = 1,54 Å, and k is the Scherer constant, which is taken as 0.94 for the calculation.
The stain ε in the sample network can be calculated using the following formula [Citation45]:
(2)
(2)
where β is the full width at the half maximum of the peak and θ is the diffraction angle of the peak.
The dislocation density δ crystallites are calculated using the equation [Citation45]:
(3)
(3)
where D is the estimated crystallite size.
Similar methods have been adopted for thin film structural parameters extraction from XRD analysis [Citation17 Citation46, Citation47]. In we have regrouped the deduced values of the different crystallographic parameters in both synthesized ZnO nanostructures. For each sample, the intense peak is used for the calculation of the parameters. As reported in the table, the found crystallite sizes are in the nonmetric range for both samples. The whole parameters, i.e crystallite size, strain, and dislocation density are in the same order of magnitude in both samples. Nonetheless, the crystallite size is slightly larger in the nanopowder than in the grown nanorods. This might be due to the lower strain in the nanoparticles network. The preferential growth observed in the nanorods can be at the origin of the stress.
Table 1. Crystallite size D, strain, and dislocation density extracted from XRD diffraction of nanostructured ZnO synthesized via green chemistry using the two studied plant extracts.
In any synthesis process based on chemical solutions, such as chemical bath and hydrothermal two processes are at the origin of nanomaterial formation: (i) homogeneous formation of nanoparticles in the solution and (ii) heterogeneous condensation film formation on a solid surface in contact with the solution. According to this, Hibiscus extract favours homogeneous condensation, that is, powder formation, whereas Artemisia plant extract favours heterogeneous condensation, that is, film formation on a glass substrate.
Nucleation occurs at the beginning of condensation, and the nanoparticles condense from the supersaturated solutions. The first stage of growth is a collision between individual species to form embryos. Embryos grow by colliding with individual species to form the nuclei. Only nuclei with a radius r larger than the critical r* can spontaneously grow into larger particles, whereas those with r < r* dissolve in the solution. The critical radius is r* = 2γ/Gv, where γ is the surface energy of the embryo and Gv is the bulk energy [Citation48]. The surface energy of the formed nuclei is important for the synthesis of uniformly sized nanoparticles. The use of Artemisia plant extract may prevent homogeneous condensation for two reasons. (i) An increase in the embryo surface energy γ results in a larger critical radius, which limits the formation of stable nuclei on one hand. (ii) And, on the other hand, to the viscosity of the solution that may alter the species motion control hereafter, species collision, and embryo formation. It is well known that the Hibiscus plant extract has low viscosity, it depends on the concentration, at a concentration of 100 g/l at 25°C (which is close to our condition) the viscosity is 0.02 cP [Citation49]. Hibiscus is used as a viscosity modifier in the petroleum industry to reduce the viscosity of the drilling fluid and friction [Citation49]. However, Artemisia plant extract has a higher viscosity of 1.6 cP [Citation50] at ambient temperature. The large viscosity of Artemisia compared to Hibiscus plant extract hinders species collision by reducing their motion in the solution, thereby reducing the homogeneous condensation.
In heterogeneous nucleation, a film is formed on a solid surface through ion or subcritical embryo absorption at the substrate. The energy required to form a film on a solid substrate is typically less than that required for homogeneous nucleation. Consequently, heterogeneous nucleation is preferred. The film grew by the diffusion of species from the solution. The attractive force of an embryo or species towards the substrate surface, governed by the van der Waals interaction, is expressed as [Citation51]
where A is the Hamaker constant, d is the diameter of the species, and r is the distance between the species and substrate.
The magnitude of the Hamaker constant reflects the strength of the force between the particle and the substrate, which is dependent on the surrounding medium and the material of the particle as well. The prevention of film formation in the case of using Hibiscus plant extract may suggest that this plant extract drastically reduces the Hamaker constant limiting and the attraction of the species towards the substrate surface. In contrast to the case of Artemisia plant extract, the Hamaker constant is larger, favouring the film formation of the immersed glass substrate.
4. Conclusions
In conclusion, in the present study, we investigated the influence of the plant extract source on the precipitation process to form nanostructured ZnO. Artemisia and Hibiscus extracts were used. Artemisia plant extract yields ZnO nanorods growth on a glass substrate. While, in contrast, Hibiscus prevents the formation of any deposit but favours nanoparticle precipitation. We concluded thereafter that the plant extract controls the homogeneous and heterogeneous growth processes by modifying the solution properties. Hibiscus reduces the solution viscosity which is at the origin of the homogeneous precipitation dominance, while the larger viscosity of Artemisia favours the heterogeneous process.
Acknowledgements
This research work was funded by institutional Fund Projects under grant number (IFPRC-197-130-2020). Therefore, the authors gratefully acknowledge technical support from the Ministry of Education and King Abdulaziz University, Jeddah Saudi Arabia.
Disclosure statement
No potential conflict of interest was reported by the author(s).
Additional information
Funding
References
- Khalaf MM, Ibrahimov HG, Ismailov EH. Nanostructured materials: importance, synthesis and characterization – a review. Chem J. 2012;02:125.
- Alves AK, Bergmann CP, Berutti F. Novel synthesis and characterization of nanostructured materials. Berlin: Springer; 2013.
- Arico AS, Bruce P, Scrosati B, et al. Materials for sustainable energy. Singapore: World Scientific; 2010. p. 148.
- Wang X, Feng J, Bai Y, et al. Synthesis, properties, and applications of hollow micro-/nanostructures. Chem Rev. 2016;116:10983.
- Jones MR, Osberg KD, Macfarlane RJ, et al. Templated techniques for the synthesis and assembly of plasmonic nanostructures. Chem Rev. 2011;111:3736.
- Cauda V, Gazia R, Porro S, et al. Handbook of nanomaterial properties. Berlin, Heidelberg: B. Bhushan, Springer-Verlag; 2014.
- Wang ZL. Nanostructures of zinc oxide. Materials Today. 2004;7:26.
- Hughes WL, Wang ZL. Nanobelts as nanocantilevers. Appl Phys Lett. 2003;82:288.
- Xu L, Chen Q, Xu D. Hierarchical ZnO nanostructures obtained by electrodeposition. Phys Chem C. 2007;111:11560.
- Liu B, Chun Zeng H. Hydrothermal synthesis of ZnO nanorods in the diameter regime of 50 nm. Am Chem Soc. 2003;125:4430.
- Namra Abid A, MuhammadKhan SS, Chaudhary K, et al. Synthesis of nanomaterial’s using various top-down and bottom-up approaches, influencing factors, advantages, and disadvantages: A review. Adv Colloid Interface Sci. 2022;300:102597.
- Ashik UPM, Kudo S, Hayashi J-i. “An overview of metal oxide anostructures”. Synthesis of inorganic nanomaterials, advances and key technologies micro and nano technologies. 2018;p. p. 19–57.
- Mohammad S, Seyed R, Ziaba AM. A review of various single layer, bilayer, and multilayer TCO materials and their applications. Mater Chem Phys. 2022;292:126789.
- Goktas S, Goktas A. A comparative study on recent progress in efficient ZnO based nanocomposite and heterojunction photocatalysts: a review. J Alloys Compd. 2021;863:158734.
- Moeen S, Ikram M, Haider A, et al. Comparative study of sonophotocatalytic, photocatalytic, and catalytic activities of magnesium and chitosan-doped Tin oxide quantum dots. ACS Omega. 2022;50:46428–46439.
- Ikram M, Haider A, Bibi ST, et al. Synthesis of Al/starch co-doped in CaO nanoparticles for enhanced catalytic and antimicrobial activities: experimental and DFT approaches. RSC Adv 2022;12:32142–32155.
- Goktas A, Aslan F, Mutlu IH. Effect of preparation technique on the selected characteristics of Zn1−xCoxO nanocrystalline thin films deposited by sol–gel and magnetron sputtering. J Alloys Compd. 2014;615:765–778.
- Anastas PT, Kirchhoff MM. Origins, current status, and future challenges of green chemistry. Acc Chem Res. 2002;35:686.
- Clark J, Macquarre D. Handbook of green chemistry and technology. London: Blackwell Science; 2002.
- Doble M, Kruthiventi AK. Green chemistry and engineering. Cambridge: Academic Press; 2007.
- Saha R, Karthik S, Balu KS, et al. Influence of the various synthesis methods on the ZnO nanoparticles property made using the bark extract of Terminalia arjuna. Mater Chem Phys. 2018;209:208.
- Thohoogi N, Diallo A, Gurib-Fakim A, et al. Nanoparticles green synthesis by Hibiscus sabdariffa flower extract: main physical properties. J Alloys and Compd. 2015;647:392.
- Galstyan V, Comini E, Baratto C, et al. Nanostructured ZnO chemical gas sensors. Ceram Int. 2015;41:14239–14244.
- Patil VL, Vanalakara SA, Patil PS, et al. Fabrication of nanostructured ZnO thin films based NO 2 gas sensor via SILAR technique. Sens Actuators, B. 2017;239:1185–1193.
- Abubakar S, Khalid N, Fatimah S, et al. Fabrication and characterization of nanostructured zinc oxide on printed microcontact electrode for piezoelectric applications. J Mater Research Technol. 2020;9:15952–15961.
- Baradaran M, Ghodsi FE, Bittencourt C, et al. The role of Al concentration on improving the photocatalytic performance of nanostructured ZnO/ZnO:Al/ZnO multilayer thin films. J Alloys Compnds. 2019;788:289.
- Mohammed AM, Ibraheem IJ, Obaid AS, et al. Nanostructured ZnO-based biosensor: DNA immobilization and hybridization. Sens Biosensing Res. 2017;15:46.
- Mahmood K, Khalid A, Mehran MT. Nanostructured ZnO electron transporting materials for hysteresis-free perovskite solar cells. Sol Energy. 2018;173:496.
- Qiu X, Jiang Y, Zhang H, et al. Lead-free mesoscopic Cs2SnI6 perovskite solar cells using different nanostructured ZnO nanorods as electron transport layers. Phys Status Solidi RRL. 2016;10:587.
- Leone F, Cataldo R, Mohamed SSY, et al. Nanostructured ZnO as multifunctional carrier for a green antibacterial drug delivery system—a feasibility study. Nanomaterials. 2019;9:407.
- Alves MJ, Grenho L, Lopes C, et al. Antibacterial effect and biocompatibility of a novel nanostructured ZnO-coated gutta-percha cone for improved endodontic treatment. Mater Sci Eng: C. 2018;92:840.
- Devi HS, Singh TD. Synthesis of copper oxide nanoparticles by a novel method and its application in the degradation of methyl orange. Adv Electron Electr Eng. 2014;4:83.
- Maensiri S, Laokul P, Klinkaewnarong J. J Optoelectron. Indium oxide (In2O3) nanoparticles using Aloevera plant extract: Synthesis and optical properties. Adv Mater. 2008;10:161.
- Gunalan S, Sivaraj R, Rajendran V. Green synthesized ZnO nanoparticles against bacterial and fungal pathogens. Prog Nat Sci Mater Int. 2012;22:693.
- Thema FT, Manikandan E, Dhlamini MS, et al. Green synthesis of ZnO nanoparticles via Agathosma betulina natural extract. Mater Lett. 2015;161:124.
- Gnanasangeetha D, Saralathambavani D. One pot synthesis of zinc oxide nanoparticles via chemical and green method. J Chem Biol Phys Sci. 2014;4:238.
- Acar ÇA. Green synthesis of zinc oxide nanoparticles using aqueous extract of Achiella millefolium l.: in vitro anti-cancer potential on lung and colon cancer cells. Turkish J Health Sci Life. 2021;4:40–45.
- Jayachandran A, Aswathy TR, Nair AS. Green synthesis and characterization of zinc oxide nanoparticles using Cayratia pedata leaf extract. Biochem Biophys Rep. 2021;26:100995.
- Karam ST, Abdulrahman AF. Green synthesis and characterization of ZnO nanoparticles by using thyme plant leaf extract. Photonics. 2022;9:594. doi:10.3390/photonics9080594
- Ukidave VV, Ingale LT. Green synthesis of zinc oxide nanoparticles from Coriandrum sativum and their use as fertilizer on bengal gram, Turkish gram, and green gram plant growth. Int J Agron. 2022. Article ID 8310038, 14. doi:10.1155/2022/8310038
- Aithssi A, Atourki L, Labchir N, et al. Electrodeposition of oriented ZnO nanorods by two-steps potentiostatic electrolysis: effect of seed layer time. Solid State Sci. 2020;104:106207.
- Tihane A, Atourki L, Najih H, et al. Comparative study of structural, optical, and morphological properties of SILAR and electrodeposition grown ZnO and Al:ZnO nanostructures. Surf Eng Appl Electrochem. 2022;58:31–40.
- Wahid KA, Lee WY, Lee HW, et al. Effect of seed annealing temperature and growth duration on hydrothermal ZnO nanorod structures and their electrical characteristics. Appl Surf Sci. 2013;283:629.
- Debye P, Scherrer P. Interference of irregularly oriented particles in x-rays. Physik Z. 1916;17:277–283.
- Segmüller A, Murakami M. X-ray diffraction analysis of strains and stresses in thin films. Treatise Mater Sci Technol. 1988;27:143–200.
- Gencer H, Goktas A, Gunes M, et al. Electrical transport and magnetoresistance properties of La0.67Ca0.33MnO3 film coated on pyrex glass substrate. Int J Mod Phys B. 2008;22:497–506.
- Mikailzade F, Türkan H, Önal F, et al. Structural, optical and magnetic characterization of nanorod-shaped polycrystalline Zn1−xMnxO films synthesized using sol–gel technique. Appl Phys A. 2020;126, Article number: 768.
- Thanh NTK, Maclean N, Mahiddine S. Mechanisms of nucleation and growth of nanoparticles in solution. Chem Rev. 2014;114(15):7610–7630.
- Woolfe ML, Chaplid MF, Otchere G. Studies on the mucilages extracted from okra fruits (Hibiscus esculentus L.) and baobab leaves (Adansonia digitata L.). J Sci Fd Agric. 1917;28:519–529.
- Jiang P, Li D, Liu Y. Studies on the mucilages extracted from okra fruits (Hibiscus esculentus L.) and baobab leaves (Adansonia digitata L.). Appl Mech Mater 2014;448–453:98–101.
- Leite FL, Bueno CC, Da Róz AL, et al. Theoretical models for surface forces and adhesion and their measurement using atomic force microscopy. J Int J Mol Sci. 2012;13:12773–12856. doi:10.3390/ijms131012773