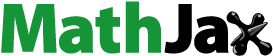
Abstract
In this article, we investigated the impact of a two electrodes argon atmospheric pressure plasma jet with a second grounded electrode (SGE) on the basil (Ocimum basilicum) seeds germination rate. The SGE enhanced the axial electric field in the direction of the treated sample. The plasma operated with two current pulses per each halve a cycle of an applied voltage. The second plasma jet current pulsed increased from 0.7 mA to 3.6 mA and the emission of the O (777.2 nm) increased 3.85 times when the jet operated with the SGE compared to that operated without SGE. The germination rate increased by eight times at 5 min of exposure using the SGE. The SEM images proved a clear etching of the basil (O. basilicum) seed surface by plasma jet with more pronounced etching and cracks by using SGE specially at higher exposure times.
1. Introduction
Plasma divided into two main types according to the thermal equilibrium between its species, i.e. thermal and non-thermal plasma. The first type of plasma is thermal plasma, in which all the plasma species have the same temperature [Citation1]. In non-thermal plasma, the electron’s temperature is higher than the other plasma species temperatures (Te << Tg), and the gas temperature can reach room temperature. Non-thermal plasma has many applications such as cancer treatments, skin treatments, sterilization, wound healing [Citation2], teeth bleaching [Citation3], cancer treatment [Citation4] nanotechnology, electrochemistry and other fields [Citation2]. Agricultural research is among the fields that used non-thermal plasma, and it played a crucial role in this field, especially in seed germination [Citation5].
Atmospheric pressure plasma jets (APPJs) used to create plasma in open space rather than confined reactors have attracted much attention over the past decade [Citation6]. The plasma jet is generated inside the tube between two electrodes and expanded through the nozzle jet by a gas flow. Currently, cold plasma researchers are increasingly focusing on many areas because of the significant benefits of plasmas: Firstly, their small dimensions and their ability to penetrate narrow gaps [Citation7,Citation8]. Secondly, Non-thermal Atmospheric Pressure Plasma Jets (NAPPJ) do not require any vacuum equipment or any complicated materials to create this type of plasma. Thirdly, cold plasmas have non-thermal effects on biological samples and heat-sensitive materials [Citation9]. Fourthly, it is a very flexible system, where it comes in the form of a pen connected to the gas cylinder and the power source. Fifthly, various operating parameters can be changed, such as the current, frequency, applied voltage, flow rate and operating gases. Finally, the plasma setup is easy and inexpensive, and all needed are a gas source, high-voltage power supply and wires and tubes [Citation10,Citation11].
The NAPPJ is one of the most common and effective systems among the other plasma systems to produce and transport reactive species to the point of interaction with the required accuracy and dosage [Citation2]. The NAPP generates charged particles, UV photons and several reactive oxygen and nitrogen species (ROS, RNS) easily generated from surrounding air [Citation12]. The plasma components play a major role in improving and modifying seed germination [Citation13]. The effect of plasma can etch the surface of the seeds by interacting with the seeds and altering the surface’s characteristics, which weakens the seed coat. The plant metabolism can be activated by the ROS generated by plasma jet, through the oxidative damage [Citation14], resulting in the oxidation of lipids of the seed coats oxidation, increasing the hydrophilicity, which increases the uptake of water by seeds [Citation15]. It has been reported that CAPPJ plays an important role in the decontamination and sterilization of seed surfaces [Citation16]. The electric and spectroscopic investigation of adding a second grounded electrode has been presented by Mohamed et al. [Citation17].
Basil (Ocimum basilicum) is an annual herb that belongs to the family Lamiaceae. Basil originates from India and other Asian regions. It is possible to use basil leaves, which contain essential oils with a distinctive aroma, fresh and dry, to season different food types. Basil plant is an industrially and economically important plant. It is usually grown in warm climatic conditions, such as Greece, India and Morocco. It can be used as flavouring agents in beverages, foods, perfumes, insecticides, fungicides, and different industrial and pharmaceutical products [Citation18]. Many areas of the world face reduced crop harvests because plants usually undergo many stresses from low water, high salinity, toxicity, decreased minerals, very extreme temperatures, drought [Citation19,Citation20] and seed-borne pathogens [Citation21].
Many types of research have been carried out on seed treatments to improve germination characteristics. As a recent technique, the cold plasma has been used during the last two decades to reduce stresses on seeds. Many studies have investigated the impact of cold plasma on seed germination. Dobrin et al. [Citation22] reported that the treatment of wheat seeds by plasma affects the seed’s early growth. Živković et al. [Citation23] found that the cold air plasma has a positive impact on modifying and improving seed growth of Paulownia tomentose. Jinkui et al. [Citation24] reported a positive impact of cold plasma on increasing alfalfa’s germination rate (Medicago sativa L.). Kitazaki et al. [Citation25] reported that oxygen plasma irradiation had a better enhancement on the germination rate of radish seed than air and argon radio-frequency (RF) plasma. Matra [Citation26] reported that the germination rate of radish seeds exposed to plasma had increased, as well as dry weight. Sarinont et al. [Citation27] investigated the impact of dielectric barrier discharge (DBD) on Raphanus sativus L. seeds using different feeding gases and analysed their seedling growth. Štěpánová et al. [Citation28] found that DBD operated in the surrounding air has the potential to reduce the spread of living micro-organisms and pathogens, leading to the accelerating and improving germination rate of the seeds and plant yields of pepper and cucumber. Jiang et al. [Citation29] found that cold plasma at 80 W could enhance wheat seed growth by significantly improving germination rate, root length, leaf area, fresh weight and plant height. Shao et al. [Citation30] reported that non-thermal plasma technology could promote alfalfa seed germination, seedling growth and water absorption ability. Sera et al. [Citation31] studied the germination rate and growth of wheat and oat seeds exposed to plasma. Stolárik et al. [Citation32] confirmed that low-temperature plasma (LTP) increased the growth of pea seeds (Pisum sativum L.) because it caused significant changes in the surface of the seed, which increases the permeability of the water absorption into seeds. Fadhlalmawla et al. [Citation33] reported that the non-equilibrium atmospheric-pressure plasma jet system enhanced and improved the fenugreek (Trigonella foenum-graecum) seeds germination rate.
The objective of this work is to investigate the seed germination of basil (O. basilicum) exposed to the non-thermal atmospheric-pressure argon plasma jet with and without using a seconded grounded electrode (SGE) as a function of exposure times. In this study, the impact of the NAPPJ on seed surface morphology was investigated by using the Scanning Electron Microscope (SEM) observation.
2. Materials and methods
2.1. Experimental setup design
The experimental set up a schematic diagram of the argon NAPPJ system is illustrated in Figure . The NAPPJ system consists of a 150 mm-long quartz-tube with 2 and 6 mm inner and outer diameters. Two copper strips separated by 35 mm are wrapped around the quartz tube, and the strip width is 7 mm. The lower strip is grounded and called the first grounded electrode (FGE), located above the tube nozzle by 25 mm. The second grounded electrode (SGE) is wrapped around a 1.5 mL Eppendorf 3810X tube containing the seed samples and located at 2 mm below the tube nozzle. A PVM500-4000 (Information Unlimited, USA) AC high-voltage power supply is connected to the upper stripe. The power supply can provide a maximum voltage of 35 kV and 30 kHz maximum frequency. The NAPPJ is generated between the two electrodes inside the quartz tube at a low argon flow rate, and it extends outside of the tube nozzle with a higher gas flow rate. The axial electric field passing through the treated samples gets enhanced by inserting the SGE. MC-5 slpm-D and MC-100 slpm-D are two mass flow metres from Alicat Scientific and measure the argon flow rate. A Pearson current probe model 6585 (PEARSON ELECTRONICS, INC, USA) is used to measure the current waveform, and a Tektronix P6015A, a high-voltage probe, is used to obtain the voltage waveform. The current and voltage waveforms were recorded using a fast oscilloscope (Tektronix DPO4104B). The variation applied plasma parameters such as the voltage, gas flow rate and the frequency are used to vary the generated jet’s length. AVT Manta G-504 colour CCD camera used to capture the plasma jet images to investigate the produced jet’s formation. The plasma jet emission spectra are recorded by a 500 mm focal, triple grating, Acton-SP-2500i spectrometer with a fast photomultiplier detector and Single-leg-fibre optic bundle (LG-455-020-3). Two gratings were used through this work; the first grating has 3600 gr/mm which is blazed at 253.65 nm and sensitive in the range 200–450 nm, the second grating has 1800 gr/mm which is blazed at 500 nm and its sensitive range is 450–850 nm. The third grating – which was not used – has 150 gr/mm and is blazed at 500 nm.
2.2. Seeds treatment with plasma
The experimental parameters applied to examine the NAPPJ effect on seeds germination were 16 kV of applied voltage, 24 kHz of applied frequency and 2 slpm argon flow rate. The seeds were placed at 2 mm below the plasma jet tube nozzle. For plasma treatment, seeds placed in 1.5 mL Eppendorf tubes 3810X. The variable parameters were the exposure times of 30 s, 1, 5, 10, 15 and 20 min. The seeds germination rate observed daily, and then the number of seeds that have germinated was recorded. Thirty seeds were selected through visual selection; healthy seeds selected without noticeable defects. After plasma treatments, seeds placed on two layers of paper filter (Whatman No. 1) inside a 90 mm diameter Petri dish; each dish contains 10 seeds. A 5 mL distilled water was added to each one and incubated in a growth chamber of 25 ± 2°C for seven days. The untreated seeds of basil (O. basilicum) served as control. The root emergence from the seed indicates the growth of the seed. After seed germination, root and shoot length of seedling were calculated with the centimetre-scale. At the end of the experiment, the fresh weight and dry weight of root and shoot were measured using a digital balance. The dry weights were estimated by splitting the seedlings into root and shoot and then dried at 75°C for 48 h in an electric oven.
The emergence of radicle from the seed indicates the growth of the seed. After seed germination, root and shoot length of seedling were calculated using a ruler. At the end of the experiment, the fresh weight and dry weight of root and shoot were measured using a digital balance. The seedlings were separated into root and shoot, then dried under 75°C for 48 h to calculate the dry weight.
The first experiment results indicated that the seed germination rate was too fast to be recorded daily interval. For this reason, in the second experiment, the seeds were observed every 8 h, and a 30-minute exposure time was added, and the 30-second exposure time was removed due to the seed germination response to the long times exposure to plasma. In experiment 2, the seconded grounded electrode (SGE) was added to the NAPPJ system to increase the axial electric field passing through the seeds. Two groups of the exposure times were used: the first one without using SGE and the second one using SGE.
2.3. Analysis of seeds exposed to plasma using SEM
A scanning electron microscope (SEM) was used to evaluate the NAPPJ impact on seed structure. The seed structure morphology imaging was used to investigate the effect of the NAPPJ. The FEI company TEM microscope with a magnification of 20–1,000,000×, Quanta 250 FEG model, used in the range of 500–5000× magnification. At 500× and 5000× magnification, the plasma effect appeared obviously.
3. Results and discussion
3.1. Plasma jet characteristics
The investigated sinusoidal voltage–current waveforms of the operational plasma jet shown in Figure . This figure shows the total current (It), which passes through the high-voltage electrode. The ground current (Ig) passes through the grounded electrode. The total current is the sum of the conduction current (Icon) and the displacement current (Idis), i.e. [It = Icon + Idis]. The conduction current found by subtracting the displacement current (when plasma off) from the total current pulse (plasma is on). The jet current (Ij) obtained by subtracting the Ig from the It [Ij = It − Ig]. The applied sinusoidal voltage–current waveforms are used to enhance the basil seeds germination rate, presented in Figure (A). This experiment’s operating plasma parameters were 16 kV of applied voltage and an applied frequency of 24 kHz at 2 slpm flow rate for various time intervals. There are two operating conditions through this study: the jet operated without and with the SGE. The current waveforms for both operating conditions showed two-current pulses per each half a cycle of the applied voltage. However, more streamers are pronounced and presented as ripples over the current waveforms when SGE is used. The current waveforms when operating a plasma jet without using the SGE showed that the first total current (It) peak to peak is much greater than the second one by 39%, and It of the first current pulse was 6.50 mA, Figure (A). Similarly, the measured second pulses (peak-to-peak) of the total and ground current pulse were 4.30 and 3.60 mA, respectively. Then, the Ij of the first and second pulse was 0.19 and 0.7 mA, respectively. Similarly, when SGE is used, the total current peak values were 6.43 and 5.52 mA for the two current discharge pulses (first and second), while Ig values for the first and second current discharge pulses were 6.40 and 2.42 mA, respectively. Therefore, the first and second Ij pulses were 0.03 and 3.1 mA, respectively. These results indicated that higher Ij current is obtained when the SGE was used, and the Ig second pulse indicated that the second Ij pulse enhanced considerably at this operating condition. The discharge ignited with a lower voltage at an earlier time, when the SGE is used, due to the axial electric field enhancement [Citation34,Citation35]. Moreover, the first pulse duration is much shorter than the second pulse, indicating the formation of the bullet and continuous operating modes per each half cycle of the applied voltage. The bullet mode could be for the first pulse, while the second current pulse is the continuous mode [Citation36].
Figure 2. The NAPPJ voltage–current waveforms at 16 kV, 2 slpm and 24 kHz, (A) total current, (B) conduction current, and (C) ground current.

Figure presents the emission spectra of the argon NAPPJ with and without using SGE ranging from 200 to 850 nm wavelength at 16 kV applied voltage, 24 kHz applied frequency, and 2 slpm argon flow rate. The fibre optic was placed under the nozzle about 2 mm. The mainly highly reactive species of plasma which can be observed Hydroxyl (OH) at 305–312 nm atomic oxygen, and
second positive system bands. The most intense of the N2 bands belonged to the vibrational transitions (0–0) at 337.1 nm, (1–0) at 315.9 nm and (0–1) at 357.7 nm [Citation37]. The band appeared in the emission spectra at ∼610–624 nm is the second order of the Hydroxyl (OH) at 305–312 nm
. The emission spectra showed a clear enhancement by 3.85 times intensifying the
O radical spectra using the SGE. These plasma species produced in the NAPPJ are effectively interacting with the surface of the seeds to play an essential role in the decontamination and sterilization of the seed coat surface [Citation16].
Figure 3. Argon NAPPJ emission spectra without using an SGE at 16 kV, 24 kHz and 2 slpm ranging from 200 to 850 nm. All undefined lines are argon lines.

The gas temperature is one of the most critical parameters of the NAPPJ, as it strongly affects the plasma chemistry, which plays a significant role in plasma applications. The plasma temperature stays near room temperature or slightly higher, which is essential for heat-sensitive plasma applications [Citation38]. The gas temperature was determined by comparing the simulated and experimentally measured emission spectra of the OH radical (A–X, 0–0)-band, as shown in Figure . The gas temperature was 300 ± 20 K and 330 ± 20 K without and with using an SGE, respectively, assuring a good fit between experimental and simulation spectra for five samples of temperature measurements. The gas temperature increased due to the axial electric field enhancement and the streamer formation when SGE is used.
The mechanism by which the plasma species produced can be summarized as – it has to be pointed out that water vapour molecules diffused from ambient air significantly impact OH production. In this experiment, the emission spectra were detected at 2 mm below the tube nozzle at which the highest density of OH is reported previously [Citation2,Citation39]. Electrons impact dissociation of water molecule could generate hydroxyl radicals (OH), hydrogen (H), as presented in reaction (1). OH can also be generated through water vapour reaction with excited metastable argon, which is generated by electron impact; reactions (2–4) [Citation2].
(1)
(1)
(2)
(2)
(3)
(3)
(4)
(4)
The previous mechanism of OH generation (reaction 1) has a reaction rate constant k = 2.3 × 10−12–1.8 × 10−10 m3 s−1 at Te = 1–2 eV, and that of the force reaction 4 is estimated to be k = 4.5 × 10−16 m3 s−1 [Citation40]. These reactions are possible in the investigated argon NAPPJ due to matching the electron temperature and the range of the energy needed for these reactions [Citation41].
The argon NAPPJ is generated in ambient air, which interacts with it. Therefore, Nitrogen molecules (N2) are found in the NAPPJ emission spectra. By the same means, oxygen radical’s emission spectra, (3p 5P) → (3s 5S) at 777.28 and (3p 3P) → (3s 3S) at 844.6 nm were obtained from the produced plasma jet. These oxygen radicals can be produced according to the following reactions (5–8) [Citation42].
Electron impact dissociative excitation of oxygen molecules:
(5)
(5)
Electron impact excitation of an oxygen atom in ground state:
(6)
(6)
Oxygen molecules dissociative attachment:
(7)
(7)
The oxygen excited O*(3p) decayed to the O (3s) emitted spectra lines of oxygen radicals [Citation43]:
(8)
(8)
Overall, the emission spectra from the NAPPJ enhanced considerably when the SGE was used. The shift in the electron energy distribution function towards higher values could cause the emission spectra enhancement due to the enhancement in the axial electric field. Therefore, the plasma jet-SGE provided more active plasma chemistry [Citation2,Citation35].
3.2. Plasma impact on seed germination
3.2.1 Experiment 1
The germination rate of basil (O. basilicum) seeds exposed to plasma jet at different exposure times, i.e. 30 s, 1, 5, 10, 15 and 20 min, is presented in Figure . The plasma operation parameters were constant for the results obtained in this study (applied voltage of 16 kV, 24 kHz of applied frequency and 2 slpm of argon flow rate). On the first day, there was no germination at any of the exposure time. Seeds germination rate began at day two for all NAPPJ exposure times with various intensities (positive or negative). A negative germination rate was observed at most exposure times of NAPPJ. Compared with the control seeds, there was a reduction in germination rates with 14%, 29%, 43%, 14% and 29% at 30 s, 1, 5, 10 and 15 min. No impact was recorded at 20 min on day three, and no impact on seeds germination was observed when seeds were exposed to NAPPJ at 30 s, 1, 15 and 20 min. The only exposure times where the germination rate was going down occurred at 5 and 10 min, by a decrease of 43% and 14%, respectively. An increase of almost 30% in germination rates was measured on day four at 20 min of exposure as well as, on day 5, all the treated seeds reached a peak in germination rate for almost all other exposure times.
3.2.2. Experiment 2
The germination rate of basil (O. basilicum) seeds exposed to NAPPJ without using SGD at different exposure times, i.e. 1, 5, 10, 15, 20 and 30 min, is presented in Figure . There was a non-germination rate on day one, and on day two, the seeds germination began with various intensities for all exposure times. A positive impact of NAPPJ on the germination rate was obtained at all exposure times, and the increase in germination rate was 2, 4, 5, 2.5, 5 and 6 times, higher than that of control seeds, at 1, 5, 10,15, 20 and 30 min of exposure times, respectively. On day three, the germination rate of seeds was negative at 1 min, compared with the control sample, and the germination rate reduction was 25%. However, a clear increase in germination rate was observed for the other exposure times, i.e. at 5 min (75%), 10 min (75%), 15 min (175%), 20 min (50%) and 30 min (125%). On day 4, the germination rate continued to increase for all the exposure times, i.e. 5, 10, 15, 20 and 30 min by 17, 33, 83, 17 and 83%, respectively. The same pattern of NAPPJ impact on germination was observed on day 5. On day 6, all seeds treated with NAPPJ reached a peak in the germination rate at almost all other exposure times.
Figure 6. The seed germination rate of basil (Ocimum basilicum) treated by plasma without using SGE as a function of exposure times: 1, 5, 10, 15, 20 and 30 min at 16 kV, 24 kHz and 2 slpm argon flow rate.

The seed germination rate of basil (O. basilicum) was exposed to NAPPJ using SGE at different exposure times, i.e.1, 5, 10, 15, 20 and 30 min is presented in Figure . No seed germination was recorded during the first day. Seed’s germination started on day 2 for all NAPPJ exposure times with various intensities. Most of the seeds exposed to NAPPJ with SGE have more germination capacity than the control seeds. The increase in seed germination at 1, 5, 10 and 20 min-SGE was 3, 8, 3 and 1 time, respectively, while no impact was observed on seed germination rate at 15 and 30 min-SGE. On day 3, an increase in germination rate was recorded at 1 and 5 min-SGE by 25% and 125%, respectively. No effect was observed on the seed germination rate at 10 and 20 min-SGE. However, at 15 30 min-SGE, the germination rate decreased by 75% and 50%, respectively. On day 4, a slight increase was observed at 1 (17%) and 5 min-SGE (50%), while the seeds germination rate decreased at 10, 15 min-SGE, 20 and 30 min-SGE by 17%, 67%, 34%, 34%, respectively. On day 5, a significant increase in germination rate was recorded at 5 min-SGE plasma exposure, and the germination rate had peaked for almost all exposure times on day 6.
Figure 7. The seed germination rate of basil (Ocimum basilicum) treated by plasma with using SGE as a function of exposure times: 1, 5, 10, 15, 20 and 30 min at 16 kV, 24 kHz and 2 slpm argon flow rate.

Figure illustrates the impact of NAPPJ on the length of the radicle and the plumule of basil (O. basilicum). The root length increased for the exposure time of 15, 5 and 15 min-SGE by 40%, 5% and 35%, respectively, compared to the control samples. The decrease in root length growth was only for 1 min of exposure time by 27%. However, a positive plumule length response of the NAPPJ treatment affected was observed for all the exposure times. The increase was 27%, 105%, 44% and 21% at 1, 15, 5 and 15 min-SGE, respectively.
3.2.3. SEM of basil (O. basilicum) seed surface
Figure shows the SEM photographs with a magnification of 500× for basil seeds exposed to NAPPJ without using SGE at different increasing exposure times. An apparent change is observed in the seed surface morphology without significant damage to the surface of the seed coat layers. These seed surface changes are more pronounced at 10, 15, 20- and 30-min exposure times than the control seeds surface. The part of the macrosclereids on the seed coat surface had disappeared when an SGE was used in the NAPPJ, as illustrated in the images shown in Figure . The longer exposure times to plasma caused severe peeling off to the macrosclereids of the seed coats. Higher SEM magnification (5000×) showed some cracks on seeds surfaces. The creaks were observed on the SEM image for the basil seeds exposed to NAPPJ without using SGE at 5 min exposure times (Figure (B)) as well as, more pronounced cracks were observed at long exposure time 15 and 20 min when seeds treated with NAPPJ having SGE as illustrated in Figure .
Figure 9. SEM with a magnification of 500× for basil (Ocimum basilicum) seed surface treated with NAPPJ (without using SGE).

Figure 10. SEM images with a magnification of 500× for basil (Ocimum basilicum) seed surface treated with NAPPJ (with using SGE).

Figure 11. SEM with a magnification of 5000× for basil (Ocimum basilicum) seed surface treated with NAPPJ (without using SGE).

Figure 12. SEM with a magnification of 5000× for basil (Ocimum basilicum) seed surface treated with NAPPJ (with using SGE).

The effects of NAPPJ on seeds have been studied recently. NAPPJ generates reactive oxygen species such as OH and O radicals that interact with treated seeds. The radicals play an essential role in dormancy and germination. The radicals move across the cell membrane causing transient pores. Then, radicals interacted with amino acid and protein leading to the generation of long-lived reactive hydroperoxides or activation of pathways. This process modifies the intracellular radicals’ concentrations. The variation in the level of radical concentrations could be for the seed’s cells’ different responses toward identical plasma exposure [Citation44]. Radicals perform a substation role in seed reserves mobilization, endosperm weakening, programmed cell death, and protection against pathogens.
The cell wall loosening during plant seed germination and seedling growth by Hydroxyl radical has been reported previously by Müller et al. [Citation45]. The regulated developmental action of OH in radicals and endosperm caps can oxidize wall polysaccharides in vivo. This work shows that during the radicle elongation and endosperm weakening, both production and action of OH increased. The authors also stated a little relation between the production of OH radical sites and growth radicle. Thus, the seed cell wall loosening by OH is controlled by this type of reactive oxygen species.
The seed germination could be enhanced when water imbibition is improving by seeds which can be achieved by etching the seed coat [Citation46] or the seeds’ erosion [Citation47]. Once the water imbibition is improved, seeds’ water absorption is accelerated, which is the main factor in initiating the seed germination process. The seed surface etching by atmospheric pressure plasma could produce a tiny hole on the seed coat. The holes could facilitate seed water absorption, which enhances the seeds germination [Citation48]. Moreover, deeper penetration of the NAPPJ radiation inside the seeds is enhanced by NAPPJ-inducing degradation to the seed surface [Citation49].
The germination of sweet basil (Ocimum basilicum L.) was improved using surface dielectric barrier discharge [Citation21]. SEM pictures revealed the significant morphological changes in the seeds surface while its coat was substantially undamaged as well as most of the fungi found on the seeds were substantially damaged after plasma exposure. An apparent change in the basil (O. basilicum) surface seeds was also observed in this work. These changes improved the germination rate in the case without using the SGE. However, this improvement was limited to 10 min of exposure time when the jet operated with the SGE. This result indicated that the plasma-seeds surface etching improved the germination rate unto certain limits, behind which a negative impact of surface etching was observed, which was apparent at 15 and 20-SGE min of exposure (Figure ). The 5000× magnification reveals cracks in the seed’s surface when treated with NAPPJ using the SGE (Figure ). These cracks were not observed on any of the SEM images for the seeds treated plasma operating without the SGE except for that exposed to 5 min (Figure (B)) which could be due to some plasma instability streamers (Figure ). The second factor which improved the germination rate when jet operated with the SGE was the enhancement in the plasma emission spectra, especially O (777.2 nm) radical, 3.85 times as presented in Figure .
4. Conclusion
Argon NAPPJ was generated and characterized to use in enhancing basil (O. basilicum) seeds germination. The SGE was added to the two-electrode argon plasma jet system that has varied impact on seed germination. The addition of the SGE enhanced the axial electric field, which increased the plasma emission spectra intensity. The O-radical emission spectra (777.4 nm) increased by 3.8 times when the SGE was used compared to that without the SGE. The jet gas temperature measured 2 mm below the jet tube nozzle increased from 300 ± 20 K (without SGE) to 330 ± 20 K (with SGE) was used. The current waveform presented two current pulses per each halve a cycle of the applied voltage. The second pulse’s plasma jet current increased from 0.7 mA to 3.1 mA when the plasma jet operated with and without the SGE, respectively.
The generated NAPPJ showed an apparent effect on the seed’s germination. The results showed that the argon NAPPJ, operating without the SGE, had a clear impact on enhancing basil (O. basilicum) seeds germination for most investigated exposure times. The maximum germinations rate was 175% for the 15 min of exposure time on the third day of observation. The germination rate increased more when the SGE was used for limited exposure time up to 5 min. The germination was clearly enhanced for 1 and 5 min of NAPPJ exposure. The enhancement reached eight times for 5 min-SGE of exposure on the second day of observation. However, for higher exposure especially for 15 and 30, a negative impact of basil (O. basilicum) seeds germination was recorded. The SEM images presented cracks in the seed’s surface treated with NAPPJ operated with the SGE. These results showed the impact of NAPPJ on seed germination and this impact had limited exposure times. The SEM images showed the etching effect of argon NAPPJ on basil (O. basilicum) seed surface. The rate of surface etching was more pronounced when the SGE was used. Therefore, plasma’s maximum impact was obtained at a shorter exposure time (5 min) when the plasma operated with SGE compared to that obtained (15 min) when plasma operated without the SGE.
Disclosure statement
No potential conflict of interest was reported by the author(s).
References
- Tendero C, Tixier C, Tristant P, et al. Atmospheric pressure plasmas: a review. J Appl Phys. 2006;61:2–30.
- Lu X, Naidis G, Laroussi M, et al. Reactive species in non-equilibrium atmospheric-pressure plasmas: generation, transport, and biological effects. Phys Rep. 2016;630:1–84.
- Lee H, Nam S, Mohamed A, et al. Atmospheric pressure plasma jet composed of three electrodes: application to tooth bleaching. Plasma Process Polym. 2010;7:274–280.
- Yan D, Cui H, Zhu W, et al. The specific vulnerabilities of cancer cells to the cold atmospheric plasma-stimulated solutions. Sci Rep. 2017;7:4479.
- Šerá B, Scholtz V, Jirešová J, et al. Effects of non-thermal plasma treatment on seed germination and early growth of leguminous plants – a review. Plants (Basel). 2021;10:1616.
- Lu XP, Liu DW, Xian YB, et al. Cold atmospheric-pressure air plasma jet: physics and opportunities. Phys Plasmas. 2021;28:100501.
- Jiang N, Ji A, Cao C. Atmospheric pressure plasma jet: effect of electrode configuration, discharge behavior, and its formation mechanism. J Appl Phys. 2009;106:013308.
- Xian Y, Wu S, Wang Z, et al. Discharge dynamics and modes of an atmospheric pressure non-equilibrium air plasma jet. Plasma Process Polym. 2013;10:372–378.
- Wu S, Xu H, Lu X, et al. Effect of pulse rising time of pulse dc voltage on atmospheric pressure non-equilibrium. Plasma Plasma Process Polym. 2013;10:136–1410.
- Mohamed A-AH, Basher AH, Almarashi JQM, et al. Susceptibility of staphylococcus epidermidis to argon cold plasma Jet by oxygen admixture. Appl Sci. 2021;11:3455.
- Rupf S, Lehmann A, Hannig M, et al. Killing of adherent oral microbes by a non-thermal atmospheric plasma jet. J med Microbiol. 2010; 391:105–110.
- Lu X, Fridman A. Guest editorial the second special issue on atmospheric pressure plasma jets and their applications. IEEE Trans Plasma Sci. 2015;43:701–702.
- Judée F, Simon S, Bailly C, et al. Plasma-activation of tap water using DBD for agronomy applications: identification and quantification of long lifetime chemical species and production/consumption mechanisms. Water Res. 2018;133:47–59.
- Măgureanu M, Sîrbu R, Dobrin D, et al. Stimulation of the germination and early growth of tomato seeds by non-thermal plasma. Plasma Chem Plasma Process. 2018;38:989–1001.
- Pizá M, Prevosto L, Zilli C, et al. Effects of non–thermal plasmas on seed-borne diaporthe/phomopsis complex and germination parameters of soybean seeds. Innov Food Sci Emerg Technol. 2018;49:82–91.
- Zahoranová A, Hoppanová L, Šimončicová J, et al. Effect of cold atmospheric pressure plasma on maize seeds: enhancement of seedlings growth and surface microorganisms inactivation. Plasma Chem Plasma Process. 2018;38:969–988.
- Mohamed A-AH, Aljuhani MM, Almarashi1 JQM, et al.. The effect of a second grounded electrode on the atmospheric pressure argon plasma jet. Plasma Res Express Plasma Res Express. 2020;2:015011.
- Soni S, Patel R, et al. Technology for improving essential oil yield of Ocimum basilicum L.(sweet basil) by application of bioinoculant colonized seeds under organic field conditions. Ind Crops Prod. 2013;45:335–342.
- Randeniya L, De Groot G. Non-thermal plasma treatment of agricultural seeds for stimulation of germination, removal of surface contamination and other benefits: a review. Plasma Process Polym. 2015;12:608–623.
- Ashraf M, Akram N, Al-Qurainy F, et al. Drought tolerance: roles of organic osmolytes, growth regulators, and mineral nutrients. Adv Agron. 2011;111:249–296.
- Ambrico P, Šimek M, Morano M, et al. Reduction of microbial contamination and improvement of germination of sweet basil (Ocimum basilicum L.) seeds via surface dielectric barrier discharge. J Phys D: Appl Phys. 2017;50:305401.
- Dobrin D, Magureanu M, Mandache N, et al. The effect of non-thermal plasma treatment on wheat germination and early growth. Innov Food Sci Emerg Technol. 2015;29:255–260.
- Živković S, Puač N, Giba Z, et al. The stimulatory effect of non-equilibrium (low temperature) air plasma pretreatment on light-induced germination of Paulownia tomentosa seeds. Seed Sci Technol. 2004;32:693–701.
- Jinkui F, Decheng W, Changyong S, et al. Effects of cold plasma treatment on alfalfa seed growth under simulated drought stress. Plasma Sci Technol. 2018;20:035505.
- Kitazaki S, Koga K, Shiratani M, et al. Effects of atmospheric pressure dielectric barrier discharge plasma irradiation on yeast growth. MRS Online Proceedings Library Archive. 2012;1469:86–91.
- Matra K. Non-thermal plasma for germination enhancement of radish seeds. Procedia Comput Sci. 2016;86:132–135.
- Sarinont T, Amano T, Attri B, et al. Effects of plasma irradiation using various feeding gases on growth of Raphanus sativus L. Arch Biochem Biophys. 2016;605:129–140.
- Štěpánová V, Slavíček P, Kelar J, et al. Atmospheric pressure plasma treatment of agricultural seeds of cucumber (Cucumis sativus L.) and pepper (Capsicum annuum L.) with effect on reduction of diseases and germination improvement plasma process. Polym. 2017;15:1700076.
- Jiang JF, He X, Li L, et al. Effect of cold plasma treatment on seed germination and growth of wheat. Plasma Sci Technol. 2014;16:54–58.
- Shao C, Fang X, Tang X, et al. Effects of low-temperature plasma on seed germination characteristics of green onion. Nongye Jixie Xuebao/Transactions of the Chinese Society for Agricultural Machinery. 2013;44:201–205.
- Sera B, Spatenka P, Sery M, et al. Influence of plasma treatment on wheat and oat germination and early growth. IEEE Trans Plasma Sci. 2010;38:2963–2968.
- Stolárik T, Henselová M, Martinka M, et al. Effect of low-temperature plasma on the structure of seeds, growth and metabolism of endogenous phytohormones in pea (Pisum sativum L.). Plasma Chem Plasma Process. 2015;35:659–676.
- Fadhlalmawla S, Mohamed A, Almarashi J, et al. The impact of cold atmospheric pressure plasma jet on seed germination and seedlings growth of fenugreek (Trigonella foenum-graecum). Plasma Sci Technol. 2019;21:105503.
- Seo Y, Mohamed A, Woo K, et al. Comparative studies of atmospheric pressure plasma characteristics between He and Ar working gases for sterilization. IEEE Trans Plasma Sci. 2010;38:2954–2962.
- Walsh J, Kong M. Contrasting characteristics of linear-field and cross-field atmospheric plasma jets. Appl Phys Lett. 2008;93:111501.
- Walsh J, Iza F, Janson N, et al. Three distinct modes in a cold atmospheric pressure plasma jet. J Phys D: Appl Phys. 2010;43:075201.
- Raud J, Laan M, Jogi I. Rotational temperatures of N2 (C, 0) and OH (A, 0) as gas temperature estimates in the middle pressure Ar/O2 discharge. J Appl Phys. 2011;44:345201.
- Lu X, Laroussi M. Optimization of ultraviolet emission and chemical species generation from a pulsed dielectric barrier discharge at atmospheric pressure. J Phys D: Appl Phys. 2005;98:3023301.
- Nikiforov A, Li L, Britun N, et al. Influence of air diffusion on the OH radicals and atomic O distribution in an atmospheric Ar (bio) plasma jet. Plasma Sources Sci Technol. 2014;23:015015.
- Novicki S, Krenos J. Absolute quenching cross section for collisions between Ar (3P0,2) and H2O. J Chem Phys. 1988;89:7031–7033.
- Li L, Nikiforov A, Xiong Q, et al. Measurement of OH radicals at state X2Π in an atmospheric-pressure micro-flow dc plasma with liquid electrodes in He, Ar and N2 by means of laser-induced fluorescence spectroscopy. J Phys D: Appl Phys. 2012;45:125201.
- Bai N, Sun P, Zhou H, et al. Inactivation of Staphylococcus aureus in water by a cold, He/O2 atmospheric pressure plasma microjet. Plasma Process Polym. 2011;8:424–431.
- Pagnon D, Amorim J, Nahorny J, et al. On the use of actinometry to measure the dissociation in O2 DC glow discharges: determination of the wall recombination probability. J Phys D: Appl Phys. 1995;28:1856.
- Xu G, Liu J, Yao C, et al. Effects of atmospheric pressure plasma jet with floating electrode on murine melanoma and fibroblast cells. Phys Plasma. 2017;24:083504.
- Müller K, Linkies A, Vreeburg RA, et al. In vivo cell wall loosening by hydroxyl radicals during cress seed germination and elongation growth. Plant Physiol. 2009; 150:1855–1865.
- Ji S, Choi K, Pengkit A, et al. Effects of high voltage nanosecond pulsed plasma and micro DBD plasma on seed germination, growth development and physiological activities in spinach. Arch Biochem Biophys. 2016;605:117–128.
- Sera B, Sery M, Stranak V, et al. Does cold plasma affect breaking dormancy and seed germination? A study on seeds of lamb’s quarters (Chenopodium album agg.). Plasma Sci Technol. 2009;11:750.
- Jiayun T, Rui H, Xiaoli Z, Ruoting Z, Weiwen C, Size Y 2014 Effects of atmospheric pressure air plasma pretreatment on the seed germination and early growth of Andrographis paniculata. Plasma Sci Technol. 16:240.
- Grzegorzewski F, Rohn S, Kroh L, et al. Surface morphology and chemical composition of lamb’s lettuce (Valerianella locusta) after exposure to a low-pressure oxygen plasma. Food Chem. 2010;122:1145–1152.