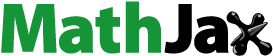
Abstract
The powder of Ocimum tenuiflorum leaves (OtLP) was modified by ZnCl2, H2C2O4, or CuS. The modified powders were characterized and applied as adsorbents for the first time to adsorb Congo red (CR). The effects of the most potent experimental variables on the CR adsorption by the chosen adsorbent were examined. Kinetic, isotherm, and thermodynamic constants were investigated. The best adsorbent was discovered to be the powder modified by ZnCl2 and CuS, which removed 97.82% of the CR and had a pore size of 1349.0 Å, pore volume of 2.1211 cm³.g−1, and a 281.16 m2.g−1 surface area. This adsorption followed models of second order and Langmuir for its kinetics and isotherms, respectively. Thermodynamic analyses proved that this adsorption is both heat-absorbing and spontaneous. The high uptake capacities (625.000, 714.286, and 769.231 mg.g−1) obtained in this study prove that this adsorbent will gain a significant attention in the field of water decontamination.
1. Introduction
Congo red (CR) is an anionic diazo dye that metabolizes into benzidine, which is recognized as carcinogenic to humans [Citation1]. Furthermore, benzidine resulting from the metabolization of CR has health risks like gastrointestinal, ocular, and skin irritation [Citation2]. Blood clotting, respiratory difficulties, and somnolence are the other side effects of benzidine [Citation2]. CR and other dyes are generally used in the paper and textile industries to dye paper and fabrics such as silk, hemp, and cotton [Citation3]. These dyes also applied in a variety of other industries, like cosmetics, plastics, and the medical industries [Citation4]. It is estimated that 10–20% of CR or any other dyes will be lost and discharged with the effluent during industrial operations [Citation5]. As a result, discharging sewage from these industries into the surrounding aquatic environment will alter the odour and colour of the water and strongly impact the photosynthetic process of the aquatic life [Citation6]. Long-term contact to sewage containing CR can affect the hematopoiesis, liver, and the circulatory system of humans, resulting in diarrhoea, nausea, breathing problems, and vomiting [Citation7]. Furthermore, wastewater containing less than 1 ppm of CR, or any dye is very visible, unwanted, and must be removed before wastewater can be released into the environment [Citation8].
Therefore, biodegrades such as polypropylene and polyurethane foams [Citation9] and white rot fungus [Citation10] have been employed for the degradation of CR contained in the effluent of those industries. Graphene oxide-zeolite and Laccase enzyme immobilized on Titania nanoparticles were also employed as a biocatalyst for degradation of Direct Red 23, 31 dyes and others [Citation11,Citation12]. The process of Fenton was used for the degradation of methylene blue and other poisonous dyes by using ions of lanthanide doped with magnesium ferrite [Citation13] and biochar doped with iron [Citation14], respectively. Moreover, nanoparticles of CoFeO [Citation15], NiO [Citation16], ZnO [Citation17] and others were applied in the catalytic degradation of CR. Due to the complicated and heterocyclic structure of this dye (CR), its bio or photo degradability has been reported to be inadequate [Citation18]. As a result, additional methods for removing CR from industrial effluent have been used, including coagulation [Citation19], electrocoagulation [Citation20], precipitation [Citation21], and filtering [Citation22]. These techniques were considered unsuccessful due to their low removal efficacy and superior cost.
On the contrary, the adsorption of CR by clay [Citation23], activated carbon of some raw materials [Citation24–26], nanoparticles of some metallic oxides like MgO [Citation27], SnO2 [Citation28], NiO [Citation29], and other adsorbents was not only the most effective but also the simplest to operate and design. As a result, the chosen method for removing CR from solutions in this investigation was adsorption. The expensive cost of manufacture has limited the use of these adsorbents, despite their great adsorptive performance. Therefore, the recent objective is to substitute these adsorbents with biosorbents that are cheap, efficient, and widely available. For this, several studies have been conducted to eliminate CR from solutions using chemically modified low-cost biosorbents like shiitake mushrooms hydrothermally treated [Citation30], pine bark [Citation31], shell powder of shrimp [Citation32], Eichhornia crassipes roots [Citation33], Egyptian water hyacinth [Citation34], soybean curd xerogels [Citation35], Teucrium polium [Citation36], and others. The results of these investigations showed that there are significant disparities in the adsorption abilities of these low-cost biosorbents when it comes to CR. As a result, more researches on CR adsorption by other various low-cost biosorbents are required.
Ocimum tenuiflorum (Ot) is considered the world’s most holy plant due to its extensive medicinal and effective disease-prevention properties [Citation37]. For example, this herb’s stems, leaves, roots, seeds, and flowers were used for several medicinal purposes in Greek and Roman times [Citation38]. Most of the anticancer, analgesic, antimetic, antiasthmatic, antifertility, antistress, diaphoretic, epatoprotective, antidiabetic, hypolipidmic, and antistress are also extracted from OT [Citation39–41]. This plant is widely cultivated all over the world. Therefore, the effective inhibitors of zinc [Citation42] and aluminium [Citation43] corrosion have been extracted from the leaves of this cheap and readily available herb (Ot). While the inhibitor extracted from the roots of Ot was used for inhibiting carbon steel corrosion [Citation44]. Ot extracts and silver nanoparticles were also found to have a potent inhibitory effect on both gram-positive and gram-negative bacteria [Citation45]. In the field of adsorption, the extract of Ot has been used for synthesis a novel silver-nanocomposites for reactive turquoise blue dye adsorption [Citation46], leaves powder of Ot was applied as an effective and cheap biosorbent for removing fluoride from drinking water [Citation47], and hexavalent chromium adsorption from solutions on Ot-based activated carbon was reported [Citation48].
Despite the adsorptive effectiveness, low cost and widespread availability of the environmentally friendly Ocimum tenuiflorum in many countries, particularly in Tabuk and other parts of Saudi Arabia, no effort has been made to date to produce a new adsorbent for the removal of Congo red from wastewater using the leaf powder of Ocimum tenuiflorum (OtLP) as a raw material. As a result, the main purpose of this study is to evaluate the efficacy of CR adsorption using a new adsorbent prepared from the available and inexpensive OtLP. The leaf powders of Neem [Citation49,Citation50], Lamiaceae [Citation51], Nitraria retusa [Citation52], Ocimum basilicum [Citation53,Citation54] modified by ZnCl2 were discovered to have a significant ability for KMnO4 and methylene blue uptake. The effects of ZnCl2, C2H2O4, and CuS on the uptake performance of Teucrium polium [Citation36] and seeds of Foeniculum vulgare [Citation55] toward Congo red, and seeds of Foeniculum vulgare toward potassium permanganate [Citation56] were also studied, and it was discovered that these three chemical agents have significant effects on these adsorbents’ adsorption ability. Thus, to attain the main goal of this work, zinc chloride (ZnCl2), oxalic acid (C2H2O4), and copper sulphide (CuS) were used to modify this plant’s leaf powder. The adsorptive capabilities of CR by OtLP and the other three modified samples were compared to identify the best adsorbent. Finally, the effects of experimental circumstances as well as thermodynamic, isotherm, and kinetic constants on the CR adsorption by the best adsorbent produced and evaluated in this study were investigated.
2. Methodology
2.1. Adsorbents preparation
The leaves of Ocimum tenuiflorum that had been bought at a medicinal herb market in Tabuk, Saudi Arabia were washed four times in distilled water and then left at 115 °C in an oven for 10 h to dry. After that, the dehydrated leaves were pulverized into powder with an electric grinder and designated as OtLP.
120 g of dried powder (OtLP) was re-condensed for 4 h in 1 L of ZnCl2 (25% w/w). After that, the mixture was cooled down to room temperature. To remove any residual ZnCl2 in the solid, the solid portion of this cold mixture was filtered and rinsed once in 200 mL of 1.5 M HCl. Finally, the obtained solid was washed multiple times with distilled water, then dried in a 115 °C oven for 21 h, pulverized, and sieved before being labelled as OtLP-Zn.
The same techniques and conditions were applied to a combination of OtLP (120 g) and CuS (60 g), and the yielding adsorbent was named OtLP-ZN/Cu.
Using the same conditions and processes as previously, a combination of OtLP (120 g) and CuS (60 g) was re-condensed using 1L of H2C2O4 solution (25% w/w). The obtained adsorbent was labelled as OtLP-Ox/Cu.
120 g of OtLP were also separately modified by refluxing this powder with 500 mL (30% w/w) of NaOH, KOH, KCl, and H2SO4 solutions for 4 h.
2.2. Adsorbents characterization
The surface functional groups of the OtLP and the other three modified adsorbents before and after CR adsorption were qualitatively recognized using FT-IR (Nicolet iS5 of Thermo Scientific FT-IR, USA). The morphology of these adsorbents, on the other hand, was identified only before adsorption using SEM at a 10 kV accelerating voltage. The surface area along with the porosity of these adsorbents were then estimated using BET (NOVA-2200 Ver. 6.11) technology at 77.35 K for 22 h. In addition, five 0.05 M Na2CO3 solutions with pH starting values ranging from 2 to 10 (pHi) were prepared. In a 100 mL plastic container, 50 mL of each solution were combined with 0.4 g of the optimum adsorbent. The bottles were shaken for 23 h at 27°C and 185 rpm in shaker incubators. A pH metre was applied for measuring the pHf (final pH) of these solutions after each solution was separated by filtering. Finally, to determine the pHZPC of this adsorbent, the pHi–pHf were calculated and plotted against the pHi.
2.3. Adsorption experiments
2.3.1. Selecting the perfect adsorbent
To select the most efficient adsorbent among the four adsorbents prepared and developed in this research, a fixed mass (0.035 g) of OtLP, OtLP-Zn, OtLP-ZN/CuS, and OtLP-Ox/Cu were added separately to four amber bottles containing 10 mL of 100 mg.L−1 CR solution. Those four bottles were then sealed and agitated for 23 h at 27oC and 175 rpm in a thermal shaker. Then the solid portion of the mixture of each bottle was separated by filtration, and the CR residual in the liquid part was quantified by a Jenway 6800 UV-Vis’s spectrophotometre at 500 nm. The percent of the CR that has been eliminated from solutions (% R) by each adsorbent was computed using Equation (1).
(1)
(1)
Where C0 and Ce are the CR solution’s starting and ultimate concentrations, respectively.
2.3.2. Equilibrium and kinetic experiments
Batch adsorption experiments of 30 mL of CR solutions by OtLP-Zn/Cu as a perfect adsorbent were conducted in amber bottles (50 mL) and a thermal shaker (175 rpm) under various experimental circumstances that are listed in Table . After the selected time of each of these experiments, the adsorbents were withdrawn, and the residual CR concentration in the supernatant was determined as stated in Section 2.3.1. The quantity of CR adsorbed at time t (qt) and equilibrium (qe) were computed using Equations (2) and (3).
(2)
(2)
(3)
(3)
Where Ct stands for CR concentrations (mg.L−1) at contact time t, m for OtLP-Zn/Cu mass (g), and V for CR solution volume (L).
Table 1. Experimental conditions for uptake of Congo red by OtLP-Zn/Cu.
The effects of changing the CR concentration (20-1000 mg.L−1), mass of OtLP-Zn/Cu (0.005-0.045 g), pH (5.5-11.5), and agitation time (0–7 h) on this adsorption have been studied to determine the best experimental conditions. The impact of pH was inspected only in the range of 5.5–11.5, since at pH < 5, the CR solution colour changes to blue. The experimental data for the adsorption of 100, 200, and 300 mg.L−1of CR solutions at 27 ± 1°C by OtLP-Zn/Cu were examined using the dynamic models indicated in Table .
Table 2. Used kinetic models in this study.
The linear Equations (4) (Freundlich) and (5) (Langmuir) along with Equation (6) were used for analysing the isotherm practical results for the adsorption of CR solutions (20–1000 mg.L−1) by OtLP-Zn/Cu (0.035 g) at a contact time of 23 h and a variety of temperatures (27 ± 1, 42 ± 1, and 57 ± 1°C).
(4)
(4)
(5)
(5)
(6)
(6)
where qmax (mg.g−1) denotes maximum uptake, KF and KL denote Freundlich and Langmuir constants, respectively. n: constants relating to uptake intensity. Sf: a separating factor. C0 is the maximum CR starting concentration.
To assess the accuracy of the models of mathematics used to analyse the kinetic and isotherm experimental data for CR adsorption by OtLP-ZN/Cu, the fractional error function (CFEF) Equation (7) and the statistic of chi-square (x2) Equation (7) have been applied.
(7)
(7)
(8)
(8)
where n is the number of experiments, and qe,cal and qe,exp are the values of the calculated and experimental uptake capacity, respectively.
Based on Equations (7) and (8), the thermodynamical coefficients like ΔSo (entropy change), ΔHo (enthalpy change), and ΔGo (standard free energy change) were calculated from the results of the adsorption of 40, 60, 200, 500, and 700 mg.L−1 CR solutions.
(9)
(9)
(10)
(10)
R (8.314 J.K−1 mol−1) stands for the universal gases constant, and T stands for the adsorption temperature (K).
Additionally, to prevent the photodegradation of CR, all the batch adsorption tests were conducted in a dark setting utilizing amber bottles and a thermal shaker.
3. Results and discussion
3.1. Characterization of OtLP and its derived adsorbents
Figure (a) shows a typical FTIR spectrum of the four adsorbents (OtLP, OtLP-Zn/Cu, OtLP-Zn, OtLP-Ox/Cu) before adsorption of CR. The OtLP sample exhibits six primary bands that are attributed to the O–H stretching mode at 3304.51 cm−1, C–H stretching (alkyl) at 2922.63 cm−1, the N–H group at 1604.52 cm−1, strong C–H stretching at 1373.14 cm−1, C–O stretching at 1243.58 cm−1, and alkyl amine at 1024.79 cm−1 (Figure ). In the case of the three modified adsorbents (OtLP-Zn/Cu, OtLP-Zn, OtLP-Ox/Cu), some of these absorption peaks with a slight shifting appeared and some of them vanished. For instance, in the OtLP-Zn/Cu sample, the peaks at 3304.51, 1373.14, and 1243.58 cm−1 cannot be observed along with the peak at 1373.14 cm−1 in the case of the OtLP-Zn sample. Moreover, the peaks at 3304.51 and 1243.58 cm−1 disappeared from the spectrum related to the OtLP-Ox/Cu sample. Most of the functional groups discovered in these adsorbents were also present in Cinnamomum camphora, which was employed for the adsorption of some heavy metals [Citation57] and carbon produced from the powder of waste toner, which was used to degrade certain organic pollutants [Citation58].
Figure (b) demonstrates the FTIR spectra of the adsorbents scanned after adsorption of CR. It can be seen that all absorption peaks in the FTIR spectra of the adsorbents before adsorption (Figure (a)) have been shifted to other positions after adsorption (Figure (b)). The changes in peak positions observed following adsorption confirm CR adsorption onto these adsorbents. Some new peaks in the range of 1600 cm−1–800 cm−1 after CR adsorption can also be observed (Figure (b)). It can be supposed that these new peaks were brought about by the adsorbed anions of CR.
The images of a, b, c, and d demonstrated in Figure are the SEM images of OtLP, OtLP-Zn/Cu, OtLP-Zn, and OtLP-Ox/Cu, respectively. The images in this figure reveal that the structures and folds of OtLP were considerably affected by the chemical agents (ZnCl2, ZnCl2/CuS, H2C2O4/CuS) applied in this study. Where the folds and structures of OtLP were transformed to tiny and fine-scattered masses due to its modification by ZnCl2/CuS (Image b) and H2C2O4/CuS (image d). Whereas the treatment of OtLP by ZnCl2 (image c) converted it into huge masses woven together in one or two pieces.
The porosity and surface area of OtLP-Zn/Cu and OtLP-Ox/Cu are expected to be greater than that of OtLP and OtLP-Zn based on these findings.
The BET surface analyser results indicate that (315.0 Å, 0.1715 cm³.g−1, 6.05 m2.g−1), (1349.0 Å, 2.1211 cm³.g−1, 281.16 m2.g−1), (69.0 Å, 0.0855 cm³.g−1, 5.75 m2.g−1), and (581.3 Å, 1.3012 cm³.g−1, 160.07 m2.g−1) are the pore size, pore volume, and surface area of OtLP, OtLp-Zn/Cu, OtLp-Zn, and OtLp-Ox/Cu, correspondingly. This means that the porosity (pores volume and size) and surface area of OtLp-Zn < OtLP < OtLp-Ox/Cu < OtLp-Zn/Cu. The outcomes of this part are as predicted from SEM results. This demonstrates that the treatment of OtLP with ZnCl2 and CuS has a significant impact on the porosity and surface area of this new and cheap raw substance (OtLP).
The plot of the pHi against (pHi–pHf) values is shown in Figure . This ideal adsorbent’s surface will be neutrally charged at a pH of 7.4 in a solution.
3.2. Adsorption experiments
3.2.1. Performance of adsorbents
The percentage values for removing CR from the experimental solutions and the quantities of the CR adsorbed by these various adsorbents were calculated using Equations (1) and (3), respectively. The obtained values were demonstrated in Figure (a and b). This figure shows that the adsorbents’ performance efficiency decreases in the following order:
Figure 4. Percentages of CR removed (a) and from solutions and the quantities of the adsorbate adsorbed (b) by the adsorbents of OtLP, OtLP-Zn, OtLP-Ox/Cu, and OtLP-Zn/Cu.

This may be explained by the fact that these adsorbents’ surface areas and pore properties both decrease in the same sequence as mentioned in section 3.1. In comparison between the efficiency of these adsorbents in terms of CR elimination, OtLP-Zn/Cu has the highest percentage removal (97.82%). Therefore, OtLP-Zn/Cu was exclusively used in this study to conduct the other adsorption trials. These findings also suggest that the surface morphological characteristics (surface area and porosity) of these adsorbents play a decisive role in the behaviour of this adsorption.
For the modification of 120 g of OtLP, 250 g of ZnCl2 and 60 g of CuS were used. 27.49 US dollars and 10 US dollars are the price of 1 kg of ZnCl2 and CuS, respectively. Therefore, modification of 120 g of OtLP will cost around 9.37 US dollars (6.87 for ZnCl2 and 2.50 for CuS). This indicates that the effective OtLP-ZN/Cu adsorbent produced and used in this work is inexpensive.
It was also found that there were no significant changes in the concentration of CR after the adsorption process in the case of OtLP modified by NaOH, KOH, KCl, and H2SO4 solutions.
3.2.2. Factors influencing adsorption
Adsorbent dosage is an important factor that influences adsorption. As a result, the effect of OtLP-Zn/Cu dose variations on the CR uptake from solution was investigated in this work to identify the optimum dosage thereafter. The outcomes of this investigation are presented in Figure (a), which demonstrates that when the mass of OtLP-Zn/Cu increased from 0.005 g to 0.035 g, the CR uptake increased from 19.06% to 94.47%. This increase was due to increasing the availability adsorption effective sites [Citation56]. Figure (a) also shows that increasing the mass of OtLP-Zn/Cu above 0.035 g has no discernible influence on CR uptake; hence, 0.035 g has been chosen as the optimal mass for the remaining experiments. The adsorption of permanganate ions by copper sulphide exhibited similar results [Citation59].
Figure 5. Factors influencing adsorption [influence the mass of OtLP- Zn/Cu (a), influence of pH (b), contact time influence (c), influence of temperature and CR concentration (d)].
![Figure 5. Factors influencing adsorption [influence the mass of OtLP- Zn/Cu (a), influence of pH (b), contact time influence (c), influence of temperature and CR concentration (d)].](/cms/asset/dc76f0e8-19e2-443b-aa41-0ab86177ce3d/tusc_a_2210973_f0005_oc.jpg)
The uptake of any adsorbate is considerably influenced by pH because the degree of adsorbate ionization, as well as the type and density of the adsorbent charge, is all dependent on the pH value of the solution [Citation60]. As a result, the impact of pH on CR uptake was also examined, and the results have been shown in Figure (b). As demonstrated, when the pH is increased from 5.5–7.5, the quantity of CR uptake decreases somewhat due to a decrease in the number of charges (+ charges) on the surface of OtLP-Zn/Cu (pHZPC = 7.4) with increasing pH in this range. As a result, the force of attraction between CR anions (pKa = 4) and the positive charges of OtLP-Zn/Cu will be decreased in the light form, resulting in a modest reduction in CR uptake. While the quantity of CR uptake was sharply decreased when pH climbed above 7.4 because of the force of repulsion between the anions of this adsorbate and the surface of OtLP-Zn/Cu, which became a negatively charged surface at a pH higher than 7.4. The same outcomes have been obtained for CR adsorption by the powdered shrimp shell [Citation32].
Figure (c) depicts the effect of agitation duration time on the uptake of CR anions from aqueous solutions (100, 200, and 300 mg.L−1) on 0.035 g of OtLP-Zn/Cu. This figure indicates that the amount of CR uptake gradually climbed with time and almost remained constant above 60 min because there is no empty site for any further adsorption after this time (60 min). Although adsorption achieved equilibrium in 1 h, the other further experiments were carried out over a period of 23 h to verify that all adsorption parameters were evaluated at equilibrium. Practically identical results were observed for CR adsorption by Teucrium polium [Citation36] and waste of tea [Citation56].
Figure (d) illustrates how the initial CR concentration influences OtLP-Zn/Cu uptake capacity (qe) at various temperatures. The preliminary concentration and temperature of the CR solution have been shown to have a favourable impact on qe. The favourable effect of the CR concentration was caused by augmenting the number of successful collisions between OtLP-Zn/Cu and CR cations [Citation61], as well as the force of driving that defeats the resistance of CR cation mass transfer between solid and liquid [Citation61,Citation62]. Since, increasing the preliminary adsorbate concentration typically increases both driving force and the successful collision rate [Citation61,Citation62]. The fact that rising temperature increases the kinetic energy of CR cations and decreases the viscosity of the CR solution explains the observed positive influence of temperature on qe [Citation63]. Moreover, the outcomes of this part reveal that the CR anion adsorption by OtPL-Zn/Cu is endothermic.
Furthermore, each of the adsorptions mentioned above resulted in crystal-clear CR solutions and the absence of any solid particles visible to the human eye, confirming the stability of this adsorbent (OtLP-Zn/Cu) in CR solutions [Citation64].
3.2.3. Kinetics outcomes
The experimental results for the adsorption of 100, 200, and 300 mg.L−1 of CR solutions at 27 °C by OtLP-Zn/Cu were analysed using the linear equations of the 1st and 2nd order dynamic models provided in Table . Figures and show plots derived by using first- and second-order kinetic models, respectively. The intercepts and slopes of the plots of these two figures were used to derive the values of the rate constants and other parameters of these two kinetic models, which are reported along with values of CFEF and X2 in Table . This table shows that R2 values (correlation coefficient values) computed by the 2nd order dynamic model are equivalent to or nearly equal to one, and vice versa for the 1st order model. The experimental qe values are nearly identical to qe values estimated using the 2nd order model and considerably different from qe values computed using the 1st order model. Moreover, the values of X2 and CFEF of the 2nd order model are so much smaller than those of the 1st order model. Thus, the experimental dynamic data can be adequately described by the dynamic model of the 2nd order, confirming that CR anions were adsorbed on the OtLP-Zn/Cu surface by chemical bonds [Citation65]. This chemisorption may take place via the mechanism of electron sharing or exchange between CR anions and the surface functional groups of OtLP-Zn/Cu [Citation66]. The thermodynamic results will provide the conclusive evidence for the type of this adsorption mechanism, namely whether it is a chemical interaction such as sharing of electrons or an ionic exchange activity. For CR adsorption by the stem of papaya, nearly identical results were obtained [Citation67].
Table 3. Constants of the 1st and 2nd-order rate models for uptake of Congo red by OtLP- Zn/CuS.
3.2.4. Isotherms outcomes
Figures and illustrate the plots that were produced after applying the linear forms of the Freundlich and Langmuir isotherm models to the practical data of this adsorption. The intercepts and slopes of the plots of these two figures were used to compute the parameters and other constants of these two isotherm models, which are listed along with the values of X2 and CFEF in Table . The best isotherm model used in this study is the Langmuir model, which is proved by the linearity of the plots of these two figures, the values of R2, the values of X2 and CFEF (Table ). This demonstrates that the adsorption of CR takes place by a monolayer, the OtLP-Zn/Cu surface is a homogenous, the energies of adsorption are equivalent, and there are no interactions between the adsorbed CR cations [Citation67]. Furthermore, Sf (factor of separation) values that are below 1 (Table ) show that this adsorption is a favourable process under the experimental circumstances used in the study [Citation68]. Nearly identical results were obtained for CR adsorption by shiitake mushrooms [Citation30] and Teucrium polium [Citation36].
Table 4. Isotherm constants for the OtLP-Zn/Cu adsorption of Congo red.
The OtLP-Zn/Cu is an inexpensive and effective adsorbent for the removal of CR from the liquid phase, as evidenced by the high values of the qmax (625.000, 714.286, and 769.231 mg.g−1) in Table . This demonstrates that OtLP-Zn/Cu is an inexpensive and efficient adsorbent that will be of particular importance in the techniques used to purify wastewater and water from CR and other pollutants.
3.2.5. Thermodynamic characteristics
The calculated ln(qe/Ce) values of CR adsorption by OtLP-Zn/Cu were plotted against 1/T (K−1) (Equation (9)) as shown in Figure . The values of ΔS0 and ΔH0 were calculated, respectively, using the intercept and slope of the straight lines in Figure . ΔG0 values were computed using Equation (10) and then listed in Table along with ΔS0 and ΔH0 values. According to Table , all ΔH0 values are positive, proving that CR cations were endothermically adsorbed by the surface of OtLP-Zn/Cu [Citation68]. This is agreed well with the outcomes of the temperature impact (section 3.2.2). The mechanism of adsorption can be estimated via the ΔH0 magnitude. ΔH0 values less than 30 kJ.mol−1, kJ.mol−1, 5 kJ.mol−1, 24–43 kJ.mol−1, 2–29 kJ.mol−1, and greater than 80 kJ.mol−1, respectively, indicate hydrogen bonding, van der Waals forces, hydrophobic bond forces, ionic exchange, dipole bond forces, and chemical bond forces [Citation69]. The ΔH0 values (32.567–42.168 kJ.mol−1) found in this study demonstrate that ionic exchange and hydrogen bonding between the OtLP-Zn/Cu functional groups and anions of CR are the mechanisms via which CR adsorbs to OtLP-Zn/Cu. Increasing the randomness of the system due to adsorption of CR anions on the OtLP-Zn/Cu can be proved by the positive ΔS0 values [Citation70]. Table further reveals that values of ΔG0 are negative and become further negative with elevating temperatures, indicating that the CR anion adsorption by OtLP-Zn/Cu is spontaneous and favoured by rising temperatures [Citation6], respectively.
Table 5. Thermodynamic parameters for the OtLP-Zn/Cu adsorption of Congo red
3.3. Adsorption performance comparison
The uptake capacities of OtLP-Zn/Cu and many of the inexpensive adsorbents lately employed for CR adsorption from liquid media have been summarized in Table . This table reveals that OtLP-Zn/Cu has greater uptake capacities than the other adsorbents (625.000, 714.286, and 769.231 mg.g−1), which can be attributed to the considerable surface areas, suitable pores, and chemical activity of OtLP-Zn/Cu. The novelty and significance of this adsorbent among the various adsorbents earlier utilized for eliminating CR from effluents are therefore confirmed by the cheap cost of OtLP-Zn/Cu, its superior adsorptive performance, and the fact that it can be easily separated from the aqueous solutions after adsorption.
Table 6. Adsorption capacity of Congo red on OtLP-Zn/Cu and other various adsorbents
4. Conclusions
In this study, CuS, ZnCl2, and H2C2O4 were utilized to modify the raw powder of Ocimum tenuiflorum leaves (OtLP). To choose the most effective one of these four adsorbents, the raw and modified powders of this herb’s leaves were characterized and examined for their ability to adsorb Congo red (CR). It was discovered that the powder modified by a combination of CuS and ZnCl2 (OtLP-Zn/Cu) had the best percentage removal for CR (97.82%) owing to its suitable porosity (pore size of 1349.0 Å and pore volume of 2.1211 cm3.g−1) and its high surface area (281.16 m2.g−1). As a result, OtLP-Zn/Cu was identified as the best adsorbent in this study for CR adsorption from solutions. The impacts of the most potent experimental variables on this adsorption were investigated. It was demonstrated that the increasing temperature from 27 °C to 57 °C, CR concentration from 20 mg.L−1 to 1000 mg.L−1, mass of OtLP-Zn/Cu from 0.005 g to 0.035 g, and time from 2 min to 45 min increase the uptake of CR. When the mass of OtLP-Zn/Cu and adsorption time were respectively increased over 0.035 g and 45 min, the capacity of this adsorption was almost invariable. The amount of CR uptake reduced gradually as the pH increased from 5.5 to 7.5 and significantly when the pH increased above 7.4. This adsorption followed the 2nd order model and the Langmuir model for its kinetics and isotherms, respectively. Thermodynamic analyses proved that this adsorption is both heat-absorbing and spontaneous. The high uptake capacities (625.000, 714.286, and 769.231 mg.g−1) obtained in this study prove that the OtLP-Zn/Cu will be a particularly attractive adsorbent in the field of wastewater remediation. Moreover, after adsorption, this adsorbent was easily and thoroughly separated from the aqueous solutions.
Acknowledgments
The author is grateful to the Nanotechnology Research Unit, Faculty of Science, University of Tabuk, for providing him with the devices He needed to complete this research.
Disclosure statement
No potential conflict of interest was reported by the author(s).
Data availability
The data collected or investigated through this research were entirely included in the manuscript that was submitted.
References
- Mall ID, Srivastava VC, Agarwal NK, et al. Removal of Congo red from aqueous solution by bagasse fly ash and activated carbon: kinetic study and equilibrium isotherm analyses. Chemosphere. 2005;61(4):492–501.
- Mittal A, Mittal J, Malviya A, et al. Adsorptive removal of hazardous anionic dye “Congo red” from wastewater using waste materials and recovery by desorption. J Colloid Interface Sci. 2009;340:16–26.
- Dai Y, Zhang N, Xing C, et al. The adsorption, regeneration and engineering applications of biochar for removal organic pollutants: a review. Chemosphere. 2019;223:12–27.
- Du Q. Highly enhanced adsorption of Congo red onto graphene oxide/chitosan fibers by wet-chemical etching off silica nanoparticles. Chem Eng J. 2014;245:99–106.
- He Z, Song S, Zhou H, et al. Reactive Black 5 decolorization by combined sonolysis and ozonation. Ultrason Sonochem. 2007;14(3):298–304.
- Kaur S, Rani S, Mahajan RK. Adsorption kinetics for the removal of hazardous dye Congo red by biowaste materials as adsorbents. J Chem. 2013;2013:1–12. DOI:10.1155/2013/628582.
- Munagapati VS, Kim DS. Equilibrium isotherms, kinetics, and thermodynamics studies for Congo red adsorption using calcium alginate beads impregnated with nano-goethite. Ecotox Environ Safe. 2017;141:226–234.
- Vandevivere PC, Bianchi R, Verstreate W. Treatment and reuse of wastewater from the textile wet processing industry: review of emerging technologies. J Chem Technol Biotechnol. 1998;72:289–302.
- Sonwani RK, Swain G, Giri BS, et al. Biodegradation of Congo red dye in a moving bed biofilm reactor: performance evaluation and kinetic modeling. Bioresour Technol. 2020;302:122811.
- Chakraborty S, Basak B, Dutta S, et al. Decolorization and biodegradation of Congo red dye by a novel white rot fungus Alternaria alternata CMERI F6. Bioresour Technol. 2013;147:662–666.
- Mahmoodi NM, Saffar-Dastgerdi MH. Clean laccase immobilized nanobiocatalysts (graphene oxide – zeolite nanocomposites): from production to detailed biocatalytic degradation of organic pollutant. Appl Catal B. 2020;268:118443. DOI:10.1016/j.apcatb.2019.118443.
- Mohajershojaei K, Mahmoodi NM, Khosravi A. Immobilization of laccase enzyme onto titania nanoparticle and decolorization of dyes from single and binary systems. Biotechnol Bioprocess Eng. 2015;20:109–116.
- Ivanets A, Rozorovich V, Sarkisov V, et al. Effect of magnesium ferrite doping with lanthanide ions on dark-, visible- and UV-driven methylene blue degradation on heterogeneous Fenton-like catalysts. Ceram Int. 2021;47:29786–29794.
- Wang C, Sun R, Huang R. Highly dispersed iron-doped biochar derived from sawdust for Fenton-like degradation of toxic dyes. J Cleaner Prod. 2021;297:126681. DOI:10.1016/j.jclepro.2021.126681.
- Ali N, Said A, Ali F, et al. Photocatalytic degradation of Congo red dye from aqueous environment using cobalt ferrite nanostructures: development, characterization, and photocatalytic performance. Water Air Soil Pollut. 2020;231:50.
- Bhat SA, Zafar F, Mondal AH, et al. Photocatalytic degradation of carcinogenic Congo red dye in aqueous solution, antioxidant activity and bactericidal effect of NiO nanoparticles. J Iran Chem Soc. 2020;17:215–227.
- Fowsiya J, Madhumitha G, Al-Dhabi NA, et al. Photocatalytic degradation of Congo red using Carissa edulis extract capped zinc oxide nanoparticles. J Photochem Photobiol B. 2016;162:395–401.
- Velkova ZY, Kirova GK, Stoytcheva MS, et al. Biosorption of Congo red and methylene blue by pretreated waste Streptomyces fradiae biomass – equilibrium, kinetic and thermodynamic studies. J Serb Chem Soc. 2018;83:107–120.
- Kristianto H, Tanuarto MY, Prasetyo S, et al. Magnetically assisted coagulation using iron oxide nanoparticles–Leucaena leucocephala seeds’ extract to treat synthetic Congo red wastewater. Int J Environ Sci Technol. 2020;17:3561–3570.
- Kristianto H, Tanuarto MY, Prasetyo S, et al. Magnetically assisted coagulation using iron oxide nanoparticles–Leucaena leucocephala seeds’ extract to treat synthetic Congo red wastewater. Int J Environ Sci Technol. 2020;17:3561–3570.
- Zhu MX, Li L, Wang HH, et al. Removal of an anionic dye by adsorption/precipitation processes using alkaline white mud. J Hazard Mater. 2007;149:735–741.
- Hou T, Guo K, Wang Z, et al. Glutaraldehyde and polyvinyl alcohol crosslinked cellulose membranes for efficient methyl orange and Congo red removal. Cellulose. 2019;26:5065–5074.
- Kausar A, et al. Dyes adsorption using clay and modified clay: a review. J Mol Liq. 2018;256:395–407.
- Ikhazuangbe P, Adama KK, Akintoye GI. Adsorption of Congo red dye onto activated carbon from periwinkle shell. Niger J Eng Sci Res. 2020;3:63–75.
- Lafi R, Montasser I, Hafiane A. Adsorption of Congo red dye from aqueous solutions by prepared activated carbon with oxygen-containing functional groups and its regeneration. Adsorp Sci Technol. 2019;37:160–181.
- Khaniabadi YO, Mohammadi MJ, Shegerd M, et al. Removal of Congo red dye from aqueous solutions by a low-cost adsorbent: activated carbon prepared from Aloe vera leaves shell. Environ Eng Manag J. 2017;4:29–35.
- Priyadarshini B, Patra T, Sahoo TR. An efficient and comparative adsorption of Congo red and trypan blue dyes on MgO nanoparticles: kinetics, thermodynamics and isotherm studies. J Magnes Alloy. 2020;16:53–63.
- Abdelkader E, Nadjia L, Noelle VR. Adsorption of Congo red azo dye on nanosized SnO2 derived from sol-gel method. Int J Ind Chem. 2016;7:53–70.
- Darwish AAA, Rashad M, Al-Aoh HA. Methyl orange adsorption comparison on nanoparticles: isotherm, kinetics, and thermodynamic studies. Dyes Pigm. 2019;160:563–571.
- Yang K, Li Y, Zheng H, et al. Adsorption of Congo red with hydrothermal treated shiitake mushroom. Mater Res Express. 2020;7:015103.
- Litefti K, Freire MS, Stitou M, et al. Adsorption of an anionic dye (Congo red) from aqueous solutions by pine bark. Sci Rep. 2019;2019(9):16530.
- Zhou Y, Ge L, Fan N. Adsorption of Congo red from aqueous solution onto shrimp shell powder. Adsorp Sci Technol. 2018;36:1310–1330.
- Wanyonyi WC, Onyari JM, Shiundu PM. Adsorption of Congo Red Dye from aqueous solutions using roots of Eichhornia crassipes: kinetic and equilibrium studies. Energy Proc. 2014;50:862–869.
- Salahuddin N, Abdelwahab MA, Akelah A, et al. Adsorption of Congo red and crystal violet dyes onto cellulose extracted from Egyptian water hyacinth. Nat Hazards. 2021;105:1375–1394.
- Zhang Z, Li Y, Du Q, et al. Adsorption of Congo red from aqueous solutions by porous soybean curd xerogels. Pol J Chem. 2018;20:95–102.
- Alamrani NA, Al-Aoh HA. Elimination of Congo Red Dye from industrial wastewater using Teucrium polium L. as a low-cost local adsorbent. Adsorp Sci Technol. 2021;2021:1–12.
- Farooqi AA, Sreeramu BS, Sreeramu BS. Cultivation of medicinal and aromatic crops, Universities Press (India) Pvt. Ltd, India, 2, Hyderabad, 2004;529–534.
- Pingale SP, Firke NP, Markandeya AG. Therapeutic activities of Ocimum tenuiflorum accounted in last decade: a review. J Pharm Res. 2012;5:2215–2220.
- Prakash P, Gupta N. Therapeutic use of Ocimum sanctum Linn (Tulsi) with note on eugenol and its pharmacological action, a short review. Indian J Physiol Pharmacol. 2005;49:125–131.
- Singh S, Taneja M, Majumdar DK. Biological activities of Ocimum sanctum L. fixed oil – an overview. Indian J Exp Biol. 2007;45:403–412.
- Singh S, Majumdar DK. Evaluation of the gastric antiulcer activity of fixed oil of Ocimum sanctum (Holy Basil). J Ethnopharmacol. 1999;65:13–19.
- Sharma SK, Mudhoo A, Jain G, et al. Inhibitory effect of Ocimum tenuiflorum (TULSI) on the corrosion of zinc in sulphuric acid: a green approach. Rasayan J Chem. 2009;2:332–339.
- Sharma A, Nair RK, Sharma A, et al. Combating aluminium alloy dissolution by employing Ocimum tenuiflorum leaves extract. Int J Adv Sci Tech Res. 2012;6:713–729.
- Ugi BU, Obeten ME, Ikeuba AI. Inhibition efficiency of eco-friendly green inhibitors (Ocimum tenuiflorum phytocompounds) on corrosion of high carbon steel in HCl environment using thermometric and electrochemical methods. J Appl Electrochem. 2018;4:158–161.
- Bagur H, Poojari CC, Melappa G, et al. Biogenically synthesized silver nanoparticles using endophyte fungal extract of Ocimum tenuiflorum and evaluation of biomedical properties. J Clust Sci. 2020;31:1241–1255.
- Banerjee P, Sau S, Das P, et al. Green synthesis of silver-nanocomposite for treatment of textile dye page 2 of 6. Nanosci Technol. 2014;1:1–6.
- Kumar M, Chayadevi A, Rao P, et al. Studies on the de-fluoridation efficacy of chrysopogon zizaniodides, Ocimum tenuiflorum and their combinational use. Int J Adv Res Publ. 2017;1:106–112.
- Sharmil SR, Venugopal T, Kannan K. Adsorption of hexavalent chromium from aqeous solution onto chemically activated Ocimum tenuiflorum stem – a kinetic, linear equilibrium and thermodynamical studies. Asian J Res Soc Sci Human. 2017;7:208–237.
- Al-Aoh HA. Equilibrium, thermodynamic and kinetic study for potassium permanganate adsorption by neem leaves powder. Desalin Water Treat. 2019;170:101–110.
- Mustafa SK, Al-Aoh HA, Bani-Atta SA, et al. Enhance the adsorption behavior of methylene blue from wastewater by using ZnCl2 modified neem (Azadirachta indica) leaves powder. Desalin Water Treat. 2021;209:367–378.
- Aljohani MMH, Almizraq JMJ, Albalawi AM, et al. Efficient dye discoloration of modified Lamiaceae leaves. Mater Res Express. 2021;8:035503. DOI:10.1088/2053-1591/abeb8f.
- Al-Aoh HA, Aljohani MMH, Darwish AAA, et al. A potentially low-cost adsorbent for methylene blue removal from synthetic wastewater. Desalin Water Treat. 2021;213:431–440.
- Bani-Atta SA, Al-Aoh HA, Aljohani MMH, et al. Methylene blue sorption by the chemically modified Ocimum basilicum leaves powder. Desalin Water Treat. 2021;222:237–245.
- Alamrani NA, Al-Aoh HA, Aljohani MMH, et al. Wastewater purification from permanganate ions by sorption on the Ocimum basilicum leaves powder modified by zinc chloride. J Chem. 2021: 1–10. DOI:10.1155/2021/5561829.
- Al-Aoh HA. Removal of the pigment Congo red from synthetic wastewater with a novel and inexpensive adsorbent generated from powdered Foeniculum vulgare seeds. Processes. 2023;11:446. DOI:10.3390/pr11020446.
- Bani-Atta SA. Potassium permanganate dye removal from synthetic wastewater using a novel, low–cost adsorbent, modified from the powder of Foeniculum vulgare seeds. Sci Rep. 2022;12:4547. DOI:10.1038/s41598-022-08543-z.
- Wang C, Wang H. Carboxyl functionalized Cinnamomum camphora for removal of heavy metals from synthetic wastewater-contribution to sustainability in agroforestry. J Cleaner Prod. 2018;184:921–928.
- Huang R, Yang J, Cao Y, et al. Peroxymonosulfate catalytic degradation of persistent organic pollutants by engineered catalyst of self-doped iron/carbon nanocomposite derived from waste toner powder. Sep Pur Technol. 2022;291:120963. DOI:10.1016/j.seppur.2022.120963.
- Aljohani MMH, Al-Aoh HA. Adsorptive removal of permanganate anions from synthetic wastewater using copper sulfide nanoparticles. Mater Res Express. 2021;8:035012.
- Basiri H, Nourmoradi H, Moghadam FM, et al. Removal of aniline as a health-toxic substance from polluted water by aloe vera waste-based activated carbon. Der Pharma Chem. 2015;7:149–155.
- Aksu Z, Tezer S. Biosorption of reactive dyes on the green alga Chlorella vulgaris. Process Biochem. 2005;40:1347–1361.
- Almeida CAP, Debacher NA, Downs AJ, et al. Removal of methylene blue from colored effluents by adsorption on montmorillonite clay. J Colloid Interface Sci. 2009;332(1):46–53.
- Hameed BH, Ahmad AA. Batch adsorption of methylene blue from aqueous solution by garlic peel, an agricultural waste biomass. J Hazard Mater. 2009;164:870–875.
- Azha SF, Ismail S. Stability and durability studies of zwitterionic adsorbent coating for the removal of organic pollutants: chemical and thermal tolerance. Mater Sci Eng. 2020;796:012054. DOI:10.1088/1757-899X/796/1/012054.
- Wanyonyi WC, Onyari JM, Shiundu PM. Adsorption of Congo red dye from aqueous solutions using roots of Eichhornia crassipes: kinetic and equilibrium studies. Energy Proc. 2014;50:862–869.
- Zhang J, Ping Q, Niu M, et al. Kinetics and equilibrium studies from the methylene blue adsorption on diatomite treated with sodium hydroxide. Appl Clay Sci. 2013;83:121–116.
- Krishni RR, Foo KY, Hameed BH. Adsorption of cationic dye using a low-cost biowaste adsorbent: equilibrium, kinetic, and thermodynamic study. Desalin Water Treat. 2014;52:6088–6095.
- Ceglowski M, Schroeder G. Removal of heavy metal ions with the use of chelating polymers obtained by grafting pyridine–pyrazole ligands onto polymethyl hydrosiloxane. Chem Eng J. 2015;259:885–893.
- Cardoso NF, Lima EC, Royer B, et al. Comparison of Spirulina platensis microalgae and commercial activated carbon as adsorbents for the removal of reactive Red 120 dye from aqueous effluents. J Hazard Mater. 2012;241–242:146–153. DOI:10.1016/j.jhazmat.2012.09.026.23040660.
- Hou HJ, Zhou RH, Wu P, et al. Removal of Congo red dye from aqueous solution with hydroxyapatite/chitosan composite. Chem Eng J. 2012;211:336–342.