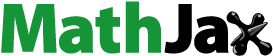
Abstract
In the present work, hematite (α-Fe2O3) nanoparticles (NPs) were synthesized by using a simple and facile green synthesis by using Hibiscus plant extract. The used solution is composed of plant extract and 0.1 M of iron chloride mixture. The nanoparticles are grown at ambient temperature. The prepared nanoparticles morphology, structure and composition were characterized by X-ray diffraction (XRD), scanning electron microscopy (SEM), energy-dispersive X-ray spectroscopy (EDS) and high-resolution transmission microscopy (HRTEM). The synthesized nanoparticles are formed by agglomeration of spherical and mono-disperse grains. XRD analysis reveals that the grains are polycrystalline with an average particle size of 20 nm. The calcinated nanoparticles contain small traces of maghemite and hydroxide. A vibrating sample magnetometer (VSM) was used for the nanoparticle magnetic properties determination. The finding reveals the superparamagnetic behaviour of the synthesized α-Fe2O3 nanoparticles owing to a saturated magnetization (Ms) of 0.96 emu/g, and low remnant magnetization Mr of 0.06 emu/g.
KEYWORDS:
1. Introduction
Iron oxide Fe2O3 oxides have been one of the most extensively studied transition metal oxides, and it has four polymorphs phases: (1) α-Fe2O3 (hematite); (2) -Fe2O3 (magnetite); (3) γ-Fe2O3 (maghemite) and (4) ϵ-Fe2O3. The -Fe2O3 and ϵ-Fe2O3 phases are generally synthesized in the laboratory while α-Fe2O3 and γ-Fe2O3 are naturally formed [Citation1]. Each polymorph possesses peculiar crystal structures and physical properties. Among them, hematite (α-Fe2O3) has many interesting properties, such as abundance low toxicity, environmental friendly nature, low-cost synthesis, chemical inertness and biocompatibility [Citation2–8]. These properties make α-Fe2O3 an interesting transition metal oxide and motivate an intense research activity on its synthesis and applications in several technological areas such as sensor [Citation9], wastewater treatment [Citation10], drug delivery [Citation11], magnetic materials coatings, [Citation12], catalysts [Citation13] and magnetic hyperthermia [Citation14], suprecapacitors [Citation15] and cancer treatment [Citation16].
Iron oxide Fe2O3 nanoparticles have been synthesized by a large number of techniques including hydrothermal methods [Citation17], sol–gel reactions [Citation18], microwave [Citation19], co-precipitation [Citation20], sonochemical [Citation21] and microemulsion [Citation22]. Generally, chemical synthesis techniques involve occasionally toxic chemicals that might produce hazardous by-products. This issue is at the origin of the interest in developing clean, simple and ecofriendly process for nanoparticle synthesis. Thereafter, various green synthesis protocols, employing plant extract and other biological products, have been investigated. The use of plant extract microorganisms might be an eco-friendly alternative to the conventional methods for metal or metal oxide nanoparticle preparation. Plant parts like leaves, seeds, fruits and root extracts contain some phytochemicals that act as both reducing agents and capping or stabilization agents. Therefore, currently, green methods of synthesizing NPs are preferred over the physical and chemical methods due to the suppression of chemical solvents and chemical products that may be hazardous to human health and the environment besides the fact that it is a simple and low-cost process.
Various herbs, spices and plants containing antioxidants are tested for iron oxide nanoparticle synthesis. These antioxidants are responsible for metal ion reduction and aggregation of metal nanoparticles prevention. They act as a capping and reducing agent, yielding to the formation of stable nanoscale nanoparticles. For example, Desalegn et al. produced iron nanoparticles, at room temperature, using Mango peel extract [Citation23]. Fruit extract from Cornelian cherry was used by Rostamizadeh et al. [Citation24] to synthesize Fe2O3 nanoparticles. Markova et al. [Citation25] synthetized iron–polyphenol nanoparticles using green tea extract. Ahmmad et al. [Citation26] succeeded in the synthesis of pure α-Fe2O3 nanoparticles by using green tea leaf extract. -Fe2O3 nanoparticles were prepared by Prasad et al. by utilizing the leaf extract of Garlic Vine [Citation27]. Phumying et al. have used Aloe Vera extract for the synthesis of Fe2O3 nanoparticles. Iron nanoparticles were prepared using Eucalyptus leaf extract [Citation28]. Venkateswarlu et al. tested plantain peel to synthesize magnetite nanoparticles. By utilizing Tridax procumbens leaves extract, Senthil and Ramesh synthesized Fe3O4 nanoparticles [Citation29]. Recently, Jamzad et al. [Citation30] synthesized nanoparticles (α-Fe2O3) nanoparticles using an extract of Laurus nobilis L. leaves.
The present work is an investigation of the iron oxide nanoparticles synthesis via green chemistry route by using, for the first time to the best of our knowledge, the Hibiscus plant extract.
2. Experimental details
Purchased Hibiscus flowers were scrupulously cleaned with tap water followed by distilled water rinsing to eliminate any contaminants and then dried in air. Flowers were cut and grinded. The Hibiscus extract was prepared by heating 10 g, at 60°C during 30 min, and the obtained flower powder in 100 ml of distilled water. The clear distilled colour changes to brown indicating the plant elements dissolution in the water.
2.1 Iron nanoparticles preparation
Iron oxide nanoparticles are prepared by mixing 10 ml of the Hibiscus extract with 90 ml FeCl3 solution 0.1 M prepared with salt dissolution in distilled water. The formation of nanoparticles was marked by the appearance of intense black precipitate. Precipitate was collected by filtration. After washing, the collected nanopowder was dried in air at 60oC during 30 min and finally calcinated at 500°C during 2 h. Figure illustrates the different steps of iron nanoparticle synthesis.
3. Results and discussion
The X-ray diffraction spectrum of the obtained nanoparticles is shown in Figure . The peaks located at 2θ = 33.16°, 35.56°, 40.87°, 49.43°, 53.96°, 62.46° and 64.02° are assigned to (104), (110), (113), (024), (116), (214) and (300) plans, respectively, originating from the rhombohedral hematite phase (JCPDS card no. 89-596.) indicating that the sample is well crystallized and mainly composed of α-Fe2O3 hematite phase. The peaks located at 29.7° and 32.12° are assigned to the (220) diffraction plane of maghemite γ-Fe2O3 and the (103) diffraction plane of α-FeOOH phase. The presence of these two peaks suggests that γ-Fe2O3 and α-FeOOH are initially formed [Citation31–33] and not transformed to α-Fe2O3 hematite phase during the calcination step at 500°C. Several authors have noticed the formation of pure hematite with increasing the heating temperature [Citation34–37]. Recently, Khan et al. [Citation18] have observed a mixture of α-Fe2O3 and ϵ-Fe2O3 in sol-gel prepared iron oxide after a heat treatment up to 600°C. Das et al. [Citation38] have reported the transition of α-Fe2O3 to Fe3O4 phases using hydrothermal reaction after thermal heating in hydrogen/argon atmosphere at 300°C.
The maghemite can be formed at low temperatures and by elevating the temperature; it transforms to α-stable phase [Citation39, Citation40]. As well, iron oxohydroxide FeOOH phase can be formed at low temperature up to 80oC [Citation41, Citation42].
It is well known that the phase transition temperature of γ-Fe2O3 to α-Fe2O3 occurs at 400°C. This transition temperature can be influenced by various parameters, including lattice defects, particle size, surface phenomena and pressure, etc. [Citation43–45].
The interspace distance dhkl, in a hexagonal structure such as the hematite phase, is given by the following relation:
(1)
(1) Knowing the relationship between dhkl and the diffraction angle θ, Equation (1) can be written as
(2)
(2)
The lattice parameter a can be then calculated using the diffraction angle 2θ = 35.56° assigned to the diffraction plane (110) the lattice constant a is then
(3)
(3) While for the determination of the lattice parameter C, we have used the diffraction angle at 33.16° assigned to the plane (104) therefore the lattice parameter c can be calculated using the relation:
(4)
(4)
The lattice parameters for the hematite and maghemite are evaluated using Equations (3) and (4), the obtained results are regrouped in Table . The crystallite size of both phases was estimated using the Debye Sheerer formula. The obtained values are also shown in Table . Table is a comparative representation of the hematite crystallite size obtained by different authors using various processes and plant extract.
Table 1. Lattice parameters, inter-plane spacing and crystallite size of the phase (the hematite bulk lattice parameter are a = b = 5.07 and c = 13.73 Å, the bulk maghemite lattice parameter are a = b = c = 8.37 Å).
Table 2. A comparative table of the reported values of the crystallite size of the hematite phase prepared by different methods and plant extract.
The Raman spectrum of the prepared nanoparticle is shown in Figure . As shown the spectrum is composed of the active optical (TO) mode vibration peaks characteristic of the hematite phase located at 227.4 and 50 cm−1 assigned to A1g modes and four peaks located at 292.8, 409, 609.8, 664.3 cm−1 attributed to Eg modes [Citation46–48].
Due to the antiferromagnetic of hematite, excited spin can have a collective vibration, forming the so-called magnon. Therefore, the intense peak at 1315 cm−1 is ascribed to two-magnon scattering [Citation46].
All peaks of the synthesized nanoparticles were found to be in accordance with the observed frequencies of α-Fe2O3 nanoparticles. The minor peak shifts originate from the variation in the size and shape in different nanoparticles. The broadness of the peak indicates the low size of the crystallite. It is commonly known, in nanoparticles, that as the particle size decreases, the peak lines become broader and shift towards lower wavenumbers [Citation49,Citation50].
This is consistent with the XRD analysis confirming the main composition of the prepared nanoparticles with α-Fe2O3. The presence of the maghemite cannot be easily detected since the peak of the Raman vibration characteristic of maghemite is 227, 289, 408 and 610 cm−1 coinciding with the hematite phase peaks position.
The SEM image and EDX composition of the synthesized nanopowder are represented in Figure (a–c). As seen in the SEM image (Figure a), an agglomeration of spherical nanoparticles is observable. Similar nanoparticles features were reported in iron oxide nanoparticles α-Fe2O3 prepared using an aqueous extract of Psoralea corylifolia seeds [Citation51], Salvadora persica aqueous extract [Citation39], Camellia sinensis (green tea) extract [Citation52] and aqueous root extract of Arisaema amurense [Citation53].
The EDX spectrum (Figure b) confirms the presence of the main elements O and Fe in the as-prepared hematite nanoparticles. The elemental mapping (Figure c) indicates the uniform dispersion of oxygen and iron elements in the nanoparticle. The nanoparticles are composed of 33% of oxygen and 67%of Fe this composition ratio indicates the lack of oxygen regarding iron.
The presence of Fe and O is also confirmed by the FTIR spectroscopy, as shown in Figure , the peak located at 592 cm−1 is assigned to stretching vibration of the Fe–O bond [Citation54].
To have an insight on nanoparticle shape and arrangement, transmission electron microscopy (TEM) and high-resolution transmission electron microscopy (HR-TEM) studies were carried out. The TEM and HRTEM of the synthesized nanoparticles are shown in Figure (a–c). The TEM image confirms the formation of nanopowder with the agglomeration of almost equal spherical nanoparticles. The grain size has a narrow distribution centred on 60 nm (Figure b). The HRTEM image (Figure c) confirms the crystalline nature of the prepared nanoparticles, the interspace associated with the (104) intense diffraction plane is visible.
Figure shows the magnetic hysteresis loop recorded in synthesized nanopowder. The prepared powder exhibits a superparamagnetic material behaviour characterized by a saturation magnetization Ms of 0.96 emu/ g, low remnant magnetization Mr of 0.06 emu/g and low coercivity Hc of 18.9 Oe. The obtained saturation in the prepared hematite is largely higher than that reported for bulk α-Fe2O3 (0.3 emu/g) [Citation55]. The presence of maghemite with hematite, as deduced from XRD analysis, may cause the increase in the synthetized nanoparticle's magnetization since the hematite phase has lower magnetization than the maghemite one. It is well known that the particle crystallinity and size control among each other, the magnetic behaviour of ferrite nanoparticles. This is attributed to the surface spin-canting effect [Citation56], below 30 nm of size the obtained nanoparticles show a superparamagnetism behaviour [Citation57]. Recently, Miri et al. [Citation39] have also observed the superparamagnetic behaviour in a-Fe2O3 nanoparticles prepared using Salvadora persica aqueous extract with an Ms = 1.5 emu/g. Narayanan et al. [Citation53] have noted the ferromagnetic behaviour of α-Fe2O3 nanoparticles synthetized via green chemistry by using root extract of Arisaema amurense, they measured a saturation magnetization (Ms) at 1.25 emu/g and remnant magnetization (Mr) at 0.50 emu/g and coercivity (Hc) at 330 G. The observed superparamagnetic behaviour in the green synthetized nanopowder suggests their potential biomedical application in drug delivery [Citation58] MRI contrast agent [Citation59] and in hyperthermia for cancer treatment [Citation60]. In Table , the values of the parameters, saturated and remnant magnetization and the coercivity fields reported by different authors are regrouped for comparison to our results. The obtained saturated magnetism (Ms) of the synthesized iron oxide nanoparticles is higher than the commercial α-Fe2O3 nanoparticles (Ms = 0.6 emu/g) [Citation61]. This can be attributed to the superparamagnetic properties of the synthesized nanoparticles due to their small grain size leading to the single magnetic domain in the nanoparticles [Citation62]. However, the obtained magnetic parameters are comparable to the reported ones in iron oxide nanoparticles prepared by green chemistry using different plant extracts.
Table 3. A comparative table of magnetic properties values: saturation (Ms), remnant (Mr) magnetization and coercivity field (Hc) of iron oxide reported by different authors.
4. Conclusion
In the present work, hematite (α-Fe2O3) nanoparticles were synthesized by using a simple and facile green synthesis by using Artemisia plant extract. The structural analysis reveals the synthesis success of hematite nanoparticles with 20 nm of average size. Small amounts of maghemite γ-Fe2O3 and hydroxide FeOOH are present in the nanoparticle indicating their incomplete transformation to hematite α-Fe2O3 after calcination at 500°C. The magnetic properties study reveals the superparamagnetic behaviour of the synthetized nanoparticles. The obtained nanoparticles have a saturated magnetization (Ms) of 0.96 emu/g, and low remnant magnetization Mr of 0.06 emu/g suggesting, thereafter, their potential biomedical applications.
Acknowledgements
The authors gratefully acknowledge technical and financial support from the Ministry of Education and King Abdulaziz University, Jeddah Saudi Arabia.
Disclosure statement
No potential conflict of interest was reported by the author(s).
Additional information
Funding
References
- Mirzaei A, Hashemi B, Janghorban K. α-Fe2O3 based nanomaterials as gas sensors. J Mater Sci Mater Electron. 2016;27:3109–3144.
- Shan H, Liu C, Liua L, et al. Highly sensitive acetone sensors based on La-doped α-Fe2O3 nanotubes. Sensors Actuats B Chem. 2013;184:243–247.
- Sun Y, Guo G, Yang B, et al. One-step solution synthesis of Fe2O3 nanoparticles at low temperature. Phys B. 2011;406:1013–1016.
- Torabian F, Rezayat AA, Nour MG, et al. Administration of silver nanoparticles in diabetes mellitus: a systematic review and meta-analysis on animal studies. Biol Trace Elem Res. 2022;200:1699–1709.
- Sabouri Z, Sabouri S, Tabrizi Hafez Moghaddas SS, et al. Facile green synthesis of Ag-doped ZnO/CaO nanocomposites with Caccinia macranthera seed extract and assessment of their cytotoxicity, antibacterial, and photocatalytic activity. Bioprocess Biosyst Eng. 2022;45:1799–1809.
- Sabouri Z, Sabouri S, Tabrizi Hafez Moghaddas SS, et al. Plant-based synthesis of Ag-doped ZnO/MgO nanocomposites using Caccinia macranthera extract and evaluation of their photocatalytic activity, cytotoxicity, and potential application as a novel sensor for detection of Pb2+ ions. Biomass Convers Bioref. 2022. doi:10.1007/s13399-022-02907-1
- Sabouri Z, Sabouri S, Tabrizi Hafez Moghaddas SS, et al. Plant-based synthesis of cerium oxide nanoparticles using Rheum turkestanicum extract and evaluation of their cytotoxicity and photocatalytic properties. Mater Technol. 2022;37:555–568. doi:10.1080/10667857.2020.1863573
- Sabouri Z, Sabouri M, Tabrizi Hafez Moghaddas SS, et al. Design and preparation of amino-functionalized core-shell magnetic nanoparticles for photocatalytic application and investigation of cytotoxicity effects. J Environ Health Sci Eng. 2022. doi:10.1007/s40201-022-00842-x
- Mirzaei A, Janghorban K, Hashemi B, et al. Synthesis, characterization and gas sensing propertiesof Ag@α-Fe2O3 core-shell nanocomposites. Nanomaterilas. 2015;5:737–749.
- Fang XL, Chen C, Jin MS, et al. Single-crystal like hematite colloidal nanocrystal clusters: synthesis and applications in gas sensors, photocatalysis and water treatment. J Mater Chem 2009;19:6154–6160.
- Gupta AK, Gupta M. Synthesis and surface engineering of iron oxide nanoparticles for biomedical applications. Biomaterials. 2005;26:3995–4021.
- Mitra S, Das S, Mandal K, et al. Synthesis of a-Fe2O3 nanocrystal in its different morphological attributes: growth mechanism, optical and magnetic properties. Nanotechnology. 2007;18:2.
- Wagloehner S, Reichert D, Sorzano DL, et al. Kinetic modeling of the oxidation of CO on Fe2O3 catalyst in excess of O2. J Catal. 2008;260:305–314.
- Lemine OM, Madkhali N, Hjiri M, et al. Comparative heating efficiency of hematite (α-Fe2O3) and nickel ferrite nanoparticles for magnetic hyperthermia application. Ceram Int. 2020;46:28821–28827.
- Mohamed Racik K, Guruprasad K, Mahendiran M, et al. Enhanced electrochemical performance of MnO2/NiO nanocomposite for supercapacitor electrode with excellent cycling stability. J Mater Sci: Mater Electron. 2019;30:5222–5232.
- Taghavizadeh Yazdi ME, Darroudi M, Amiri MS, et al. Anticancer, antimicrobial, and dye degradation activity of biosynthesised silver nanoparticle using Artemisia kopetdaghensis. Micro Nano Lett. 2020;15:1046–1050.
- Qin W, Yang C, Yi R, et al. Hydrothermal synthesis and characterization of single crystalline (-Fe2O3 nanocubes. J Nanomater. 2011;5:159259.
- Khan I, Morishita S, Higashinaka R, et al. Synthesis, characterization and magnetic properties of ϵ-Fe2O3 nanoparticles prepared by sol-gel method. J Magnet Magnet Mater. 2021;538:168264.
- Wang WW, Zhu YJ, Ruan ML. Microwave-assisted synthesis and magnetic property of magnetite and hematite nanoparticles. J Nanopart Res. 2007;9(3):419–426.
- Lassoued A. Synthesis and characterization of Zn-doped α-Fe2O3 nanoparticles with enhanced photocatalytic activities. J Mol Struct. 2021;1239:130489.
- Vijayakumar R, Koltypin Y, Felner I, et al. Sonochemical synthesis and characterization of pure nanometer-sized Fe3O4 particles. Mater Sci Eng A. 2000;286:101.
- Vidal-Vidal J, Rivas J, López-Quintela MA. Synthesis of monodisperse maghemite nanoparticles by the microemulsion method”. Colloids Surf A. 2006;288:44.
- Desalegn B, Megharaj M, Zuliang Chen Z, Naidu R. Green synthesis of zero valent iron nanoparticle using mango peel extract and surface characterization using XPS and GC-MS. Heliyon. 2019;5:1–9.
- Rostamizadeh E, Iranbakhsh A, Majd A, et al. Green synthesis of Fe2O3 nanoparticles using fruit extract of Cornus mas L. and its growth-promoting roles in barley. J. Nanostruct. Chem. 2020;2:422–427.
- Markova Z, Novak P, Kaslik J, et al. Iron(II,III)–polyphenol complex nanoparticles derived from green tea with remarkable ecotoxicological impact. ACS Sustain Chem Engin. 2014;2:1674–1680.
- Ahmmad B, Leonard K, Shariful Islam M, et al. Green synthesis of mesoporous hematite (α-Fe2O3) nanoparticles and their photocatalytic activity. Adv Powder Technol. 2013;24:160–167.
- Prasad AS. Iron oxide nanoparticles synthesized by controlled bio-precipitation using leaf extract of Garlic Vine (Mansoa alliacea). Mater Sci Semiconductors Proc. 2016;53:79–83.
- Phumying S, Labuayai S, Thomas C, et al. Aloe vera plant-extracted solution hydrothermal synthesis and magnetic properties of magnetite (Fe3O4) nanoparticles. Appl Phys A. 2012;111:1187–1193.
- Venkateswarlu S, Rao YS, Balaji T, et al. Biogenic synthesis of Fe3O4 magnetic nanoparticles using plantain peel extract. Mater Lett. 2013;100:241–244.
- Jamzad M, Bidkorpeh MK. Green synthesis of iron oxide nanoparticles by the aqueous extract of Laurus nobilis L. leaves and evaluation of the antimicrobial activity. J Nanostructure Chem. 2020;10:193–201.
- Vasireddi R, Vakili M, Monteiro D, et al. Size controlled synthesis of γ-Fe2O3 nanoparticles by simple chemical method and study of optical properties. Intern J Sci Eng Manage (IJSEM). 2016;1:5.
- Asouf HM, Al-Antary TM, Awwad AM. Green route for synthesis hematite (α-Fe2O3 nanoparticles: toxicity effect on the green peach aphid, Myzus persicae (Sulzer). Environ Nanotechnol Monit Manage. 2018;9:107–111.
- Xu C, Cheng D, Gao B, et al. Preparation and characterization of -FeOOH-coated sand and its adsorption of Cr(VI) from aqueous solutions. Front Environ Sci Eng. 2012;6:455–462. doi:10.1007/s11783-010-0275-1
- Choudhary S, Annapoorni S, Malik R. Facile strategy to synthesize donut-shaped α-Fe2O3 nanoparticles for enhanced LPG detection. Sens Actuat B. 2021;334:129668.
- Wang L, Lu X, Han C, et al. Electrospun hollow cage-like α-Fe2O3 microspheres: synthesis, formation mechanism, and morphology-preserved conversion to Fe nanostructures. Cryst Eng Commun. 2014;16:10618.
- Kushwaha P, Chauhan P. Synthesis of spherical and rod-like EDTA assisted α-Fe2O3 nanoparticles via Co-precipitation method. Mater Today Proc. 2021;44:3086–3090.
- Li N, Jiang Y, He Y-L, et al. Sustainable synthesis of multiple-metal-doped Fe2O3 nanoparticles with enhanced photocatalytic performance from Fe-bearing dust. J Mater Res Technol. 2021;15:810–820.
- Das R, Witanachchi C, Nemati Z, et al. Magnetic vortex and hyperthermia suppression in multigrain iron oxide nanorings. Appl Sci. 2020;10:787. doi:10.3390/app10030787
- Miri A, Khatami M, Sarani M. Biosynthesis, magnetic and cytotoxic studies of hematite nanoparticles. J Inorg Organomet Polym Mater. 2020;30:767–774.
- Vasireddi R, Vakili M, Monteiro D, et al. Size controlled synthesis of γ-Fe2O3 nanoparticles by simple chemical method and study of optical properties. Int J Sci Eng Manage (IJSEM). 2016;1:10.
- Vernekar D, Jagadeesan D. Tunable acid–based bifunctional catalytic activity of FeOOH in an orthogonal tandem reaction”. Catal Sci Technol 2015;5:402.
- Mei L, Liao L, Wang Z, et al. Interactions between phosphoric/tannic acid and different forms of FeOOH. Adv Mater Sci Eng; 2015:250836. doi:10.1155/2015/250836
- Wu W, Wu Z, Yu T, et al. Recent progress on magnetic iron oxide nanoparticles: synthesis, surface functional strategies and biomedical applications. Sci Technol Adv Mater. 2015;16:023501–023544.
- Varadwaj KSK, Panigrahi MK, Ghose J. Effect of capping and particle size on Raman laser-induced degradation of γ-Fe2O3 nanoparticles. J Solid State Chem. 2004;177:4286–4292.
- Soler MAG, Alcantara GB, Soares FQ. Study of molecular surface coating on the stability of maghemite nanoparticles. Surf Sci 2007;601:3921–3925.
- Shim SH, Duffy TS. Raman spectroscopy of Fe2O3 to 62 GPa. Am Mineral. 2002;87:318–326.
- Li SZ, Zhang H, Wu JB, et al. Shape-Control fabrication and characterization of the airplane-like FeO(OH) and Fe2O3 nanostructures. Cryst Growth Des 2006;6:351–353.
- Chen L, Yang X, Liu J, et al. Continuous shape- and spectroscopy-tuning of hematite nanocrystals. Inorg Chem. 2010;49:8411–8420.
- White WB. The structure of particles and the structure of crystals: information from vibrational spectroscopy. J Ceram Process Res. 2005;6:1.
- Ahmmad B, Leonard K, Islam MS, et al. Green synthesis of mesoporous hematite (-Fe2O3) nanoparticles and their photocatalytic activity. Adv Powder Technol. 2013;24:160–167.
- Nagajyothi PC, Pandurangan M, Kim DH, et al. Green synthesis of iron oxide nanoparticles and their catalytic and In vitro anticancer activities. J Clust Sci. 2017;28:245–257. doi:10.1007/s10876-016-1082-z
- Herlekar M, Barve S, Kumar R. Plant-mediated green synthesis of iron nanoparticles. J Nanopart; 2014:140614. doi:10.1155/2014/140614
- Narayanan KB, Han SS. One-pot green synthesis of Hematite (α-Fe2O3) nanoparticles by ultrasonic irradiation and their in vitro cytotoxicity on human keratinocytes CRL-2310. J Clust Sci. 2016;27:1763–1775.
- Kant R, Kumar D, Dutta V. High coercivity α-Fe2O3 nanoparticles prepared by continuous spray pyrolysis. RSC Adv. 2015;5:52945.
- Teja AS, Koh PY. Synthesis, properties, and applications of magnetic iron oxide nanoparticles. Prog Cryst Growth Charact Mater. 2009;55:22–45. doi:10.1016/j.pcrysgrow.2008.08.003
- Kodama RH. Magnetic nanoparticles. J Magn Mater. 1999;200:359.
- Tadic M, Panjan M, Damnjanovic V, et al. Magnetic properties of hematite (α-Fe2O3) nanoparticles prepared by hydrothermal synthesis method. Appl Surf Sci. 2014;320:183–187. doi:10.1016/j.apsusc.2014.08.193
- Chourpa I, Douziech-Eyrolles L, Ngaboni-Okassa L, et al. Molecular composition of iron oxide nanoparticles, precursors for magnetic drug targeting, as characterized by confocal Raman microspectroscopy. Analyst. 2005;130:1395.
- Burtea C, Laurent S, Roch A, et al. C-MALISA (cellular magnetic-linked immunosorbent assay), a new application of cellular ELISA for MRI. Inorg Biochem. 2005;99:1135.
- Arias LS, Pessan JP, Vieira APM, et al. Iron oxide nanoparticles for biomedical applications: a perspective on synthesis, drugs. Antimicrobial activity, and toxicity. Antibiotics. 2018;7:1–32.
- Tang ZX, Nafs S, Sorensen MC. Magnetic properties of aerosol synthesized iron oxide particles. J Magn Magn Mater. 1989;80:285–289.
- Li Q, Kartikowati CW, Horie S, et al. Correlation between particle size/domain structure and magnetic properties of highly crystalline Fe3O4 nanoparticles. Sci Rep. 2017;7:9894.
- Hjiri M, Alshammari S, Besbes H, et al. The effect of Ni/Fe ratio on the physical properties of NiFe2O4 nanocomposites. Mater Res Express. 2019;6:086107.
- Chernyshovay IV, Hochella MF, Madden AS. Size-dependent structural transformations of hematite nanoparticles. 1. Phase transition. Phys Chem Chem Phys. 2007;9:1736–1750.
- Rao A, Bankar A, Kumar AR, et al. Removal of hexavalent chromium ions by Yarrowia lipolytica cells modified with phyto-inspired Fe0/Fe3O4 nanoparticles. J Contam Hydrol. 2013;146:63–73.
- Thakur S, Karak N. One-step approach to prepare magnetic iron oxide/reduced graphene oxide nanohybrid for efficient organic and inorganic pollutants removal. Mater Chem Phys. 2014;144:425–432.
- Wang T, Jin X, Chen Z, et al. Green synthesis of Fe nanoparticles using eucalyptus leaf extracts for treatment of eutrophic wastewater. Sci Total Environ. 2014;466-46l7:210–213.
- Huang L, Weng X, Chen Z, et al. Synthesis of iron-based nanoparticles using oolong tea extract for the degradation of malachite green. Spectrochim Acta, Part A. 2014;117:801–804.
- Kayani ZN, Afzal A, Butt MZ, et al. Structural, optical and magnetic properties of iron oxide nano-particles. Mater Today Proc. 2015;10:5660–5663.
- Majeed MI, Guo J, Yan W, et al. Preparation of magnetic iron oxide nanoparticles (MIONs) with improved saturation magnetization using multifunctional polymer ligand. Polymers (Basel). 2016;8:392.
- Kumar P, No-Lee H, Kumar R. Synthesis of phase pure iron oxide polymorphs thin films and their enhanced magnetic properties. J Mater Sci Mater Electron. 2014;25:4553–4561.
- Erdem S, Erdem B, Mustafa Öksüzoğlu R. Magnetic mesoporous silica nanocomposites prepared with different sized Fe3O4: structural and magnetic properties, catalytic effects. Anadolu Univ J Sci Technol A Appl Sci Eng. 2018;19:5.
- Lassoued A, Lassoued MS, Dkhil B, et al. Synthesis, structural, morphological, optical and magnetic characterization of iron oxide (α-Fe2O3) nanoparticles by precipitation method: effect of varying the nature of precursor. Phys E. 2018;97:328–334.
- Narayanan KB, Han SS. One-pot green synthesis of hematite (-Fe2O3) nanoparticles by ultrasonic irradiation and their in vitro cytotoxicity on human keratinocytes CRL-2310. J Clust Sci. 2016;27:1763–1775.