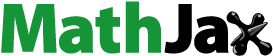
ABSTRACT
Malachite green is a pestilential micropollutant with a wide range of uses. In this work, cost-efficient and sustainable treatment of malachite green from aqueous medium was first studied using the biosorbent material obtained from Pyracantha coccinea M. J. Roemer plant. The biosorption kinetics followed the pseudo-second-order model (R2: 0.996). The standard Gibbs free energy change parameter ranging from −8.472 kJ mol−1 to −7.357 kJ mol−1 indicated a spontaneous and feasible treatment process. The biosorption isotherm was well described by Freundlich model with an R2 of 0.991. SEM and FTIR studies showed an irregular and uneven biosorbent surface morphology rich in active site. The biosorbent exhibited a good biosorption performance compared to its competitors, with a capacity of 117.745 mg g−1. As a result, this study indicated that P. coccinea M. J. Roemer-based biosorbent could be a promising candidate for the economical, effective, eco-friendly and sustainable treatment of malachite green micropollutant.
1. Introduction
The economic development of a country is significantly dependent on its rapid rise in the industrial field. Unfortunately, excessive amounts of wastewater are mostly produced as a result of this rapid rise. Today, the concern of commercial gain, which does not take into account the environment, has reached a level that threatens human health and ecological security. Despite the fact that there are many water resources in the world, the fact that there is very little water of usable quality further aggravates the picture. Water pollution is mainly caused by a variety of micropollutants, including biological, chemical, physical and radioactive substances [Citation1]. Malachite green is a chemical organic micropollutant with a wide range of uses. It is extensively applied in a wide variety of sectors, such as aquaculture, animal husbandry, leather, paper, textile, pharmaceutical and food for many purposes such as use as a colouration, antimicrobial, anthelminthic, antifungal, antiprotozoal and antiseptic agent [Citation2–4]. These anthropogenic activities are the main sources of malachite green discharge into the natural aquatic environment. Unfortunately, malachite green is a pestilential xenobiotic compound that is resistant to degradation through biological, chemical, physical and photolytic processes. Today, it is widely known that this micropollutant has carcinogenic, mutagenic and toxic effects and causes many health problems not only on humans but also on fauna and flora [Citation5–8]. Consequently, it is of vital importance to remove such micropollutants from the contaminated water environment, considering both the scarcity of usable water and their destructive effects on the ecological system.
Numerous biological, chemical and physicalapproaches are available to treat micropollutants from wastewater environment, such as ozonation, biological degradation, membrane separation, electrocoagulation, photocatalytic degradation and advanced oxidation. However, these treatment approaches have various limitations such as expensive chemical demand, high maintenance cost, inefficiency of treatment, high energy demand, fouling, high operating cost, undesirable toxic by-product formation and high chemical usage [Citation5, Citation9–11]. Conversely, natural biological material-based biosorption process is a simple, eco-friendly, economically viable, effective and flexible biotechnological method, and due to these remarkable properties, it has started to attract great attention in recent years [Citation3, Citation12–18].
In this regard, Pyracantha coccinea M. J. Roemer-based a novel biosorbent material was first investigated for environmentally friendly, economical, effective and sustainable treatment of malachite green micropollutant from aqueous medium in the present study. P. coccinea M. J. Roemer is an easy-care, robust shrub-shaped plant species. It is widely available in many countries around the world. In addition to the widespread use of the plant in many fields such as food, areal decoration, medicine, contaminant biomonitoring and removal, pest biocontrol and erosion prevention [Citation19–26], if it is successful, its use in such a new field will both provide added value to it and offer an eco-sustainable solution to the micropollutant trouble. Important operating parameters affecting malachite green treatment process were optimized using the single-factor experimental approach. The biosorption kinetics, thermodynamics, isotherm, characterization and comparison studies were conducted to evaluate malachite green treatment manner of the biosorbent material.
2. Materials and methods
2.1. Preparation of P. coccinea M. J. Roemer-based biosorbent material
The fruit biomass of P. coccinea M. J. Roemer was collected from a local park in Sinop, Turkey. The raw plant biomass was thoroughly cleaned from dust and dirt by washing with tap water and distilled water. The biomass material was oven-dried at a temperature of 80 °C for about 24 h. It was crushed into powder with the help of a grinder and sieved with a 0.5 mm mesh sieve. The powdered biomass was then mixed with dilute sodium hydroxide solution (0.3 mol L−1) using a magnetic shaker at room temperature for about 24 h. After the chemical treatment, the biomass was thoroughly washed with distilled water to remove remaining chemical and achieve neutral pH. Finally, the plant biomass was dried again and stored in an airtight bottle for use as biosorbent material in the study of malachite green biosorption.
2.2. Solution of malachite green micropollutant
Malachite green (CAS No: 569-64-2) was provided from a local chemical supplier in Samsun, Turkey. A stock solution of the micropollutant (1000 mg L−1) was made by dissolving the required amount of malachite green in distilled water. It was then diluted to the required malachite green concentrations (5–15 mg L−1) using distilled water to get the desired micropollutant-laden solution grades. Dilute hydrochloric acid (0.1 mol L−1) and sodium hydroxide (0.1 mol L−1) solutions were used to regulate the pH levels of the prepared malachite green-laden solution samples. The chemicals of analytical purity were used in all of the experiments in malachite green biosorption study.
2.3. Malachite green biosorption study
Malachite green biosorption practice was conducted using batch experiment technique at room temperature on an orbital shaker. A certain amount of the biosorbent material and a certain concentration of malachite green solution were added to a conical flask with a working volume of 100 mL and mixed at 150 rpm for a predetermined time. After the experiment period was completed, approximately 1.5 mL of solution sample was taken from the mixture and then the biosorbent material was separated from the solution phase. The concentration of malachite green in the sample was quantified by measuring the absorbance at 617 nm with the aid of a UV-Visible spectrophotometer device (Thermo Genesys 10 S). The following equations were used to determine the efficiency of the biosorbent material to remove malachite green from the solution phase at a specified time (t) and equilibrium (e), respectively.
(1)
(1)
(2)
(2) where qt (mg g−1), qe (mg g−1), C0 (mg L−1), Ct (mg L−1), Ce (mg L−1), V (L) and m (g) are the efficiency of the biosorbent at a specified time, the efficiency of the biosorbent at equilibrium, malachite green concentration at start time, malachite green concentration at a specified time, malachite green concentration at equilibrium, the solution volume and the biosorbent mass, respectively.
2.4. Optimization of operating parameters affecting malachite green treatment process
Important operating parameters affecting the biosorption operation of malachite green from wastewater environment, such as contact time (t: 0–360 mins, C0: 15 mg L−1, pH: 6, m: 10 mg), malachite green charge (C0: 5–15 mg L−1, t: 120 mins, pH: 6, m: 10 mg), pH (pH: 4–8, t: 120 mins, C0: 7.5 mg L−1, m: 10 mg) and amount of biosorbent (m: 10–30 mg, t: 120 mins, C0: 12.5 mg L−1, pH: 6), were optimized using the single-factor experiment approach.
2.5. Biosorption kinetics
The kinetics study for malachite green treatment process was conducted using the pseudo-first-order, pseudo-second-order and intra-particle diffusion models [Citation27–30].
2.6. Biosorption thermodynamics
The standard Gibbs free energy change thermodynamics parameter was used to determine the feasibility and spontaneity of malachite green biosorption process [Citation2].
2.7. Biosorption isotherm
The isotherm analysis for the biosorption operation of malachite green was performed using Freundlich, Langmuir and Dubinin-Radushkevich models [Citation31–34].
2.8. Calculations
The mathematical calculations for malachite green biosorption study were done using the experimental data averages with the aid of the SigmaPlot 12.0 software. The agreement of the experimental data with the mathematical equations was determined by the nonlinear regression approach. The R2, adjusted R2 (adjR2) and RMSE were used to assess the fit quality of the mathematical equations.
2.9. SEM and FTIR experiments
The surface features and active site profile of P. coccinea M. J. Roemer-based biosorbent were studied by SEM and FTIR experiments using SEM (Zeiss Evo Ls 10) and FTIR (PerkinElmer Spectrum 400) instruments.
3. Results and discussion
3.1. Effect of contact time
The effect of contact time on malachite green biosorption process is presented in Figure . At first, the biosorption process was rapid and most of malachite green biosorption occurred in this stage. The biosorbent material had a large number of the available active binding sites and the empty surface at this stage, resulting in rapid and intense malachite green biosorption. Later on, the biosorption performance of the biosorbent increased to a certain extent, but its biosorption rate gradually decreased. At this stage, most of the binding sites of the biosorbent were occupied by malachite green molecules as the biosorption process progressed, resulting in a reduction in the speed of the biosorption. Eventually, the biosorption activity hardly increased and the biosorption process reached the equilibrium state as the available binding sites of the biosorbent were almost completely filled by malachite green molecules [Citation15, Citation35]. Thus, the optimum contact time was determined to be 360 mins for malachite green treatment from water medium.
3.2. Effect of malachite green charge
The effect of malachite green charge on the biosorption process is shown in Figure . The biosorption capacity of the biosorbent increased from 30.524 to 79.363 mg g−1 as malachite green charge increased from 5 mg L−1 to 15 mg L−1. The high concentration of malachite green in the solution reduced the mass transfer resistance and increased the chance of effective collision between the biosorbent particles and malachite green molecules, resulting in increased the biosorption performance of the biosorbent. However, when the biosorption capacity of the biosorbent was calculated as a percentage, it was noticed that its biosorption yield decreased with the increase of malachite green charge (data not presented). At the low malachite green charge, the ratio of the active sites on the biosorbent material to the total malachite green molecules was high, which increased the biosorption efficiency by increasing the probability of interaction between the biosorbent particles and malachite green molecules [Citation36]. Thence, the optimum malachite green charge was selected to be 15 mg L−1.
3.3. Effect of pH
The effect of pH on malachite green biosorption operation is given in Figure . The biosorption capacity of the biosorbent increased from 24.884 to 34.107 mg g−1 with the increase of pH level from 4 to 8. At the low pH level, the surface of the biosorbent became positively charged by the protonation of the active sites on the biosorbent, which reduced the biosorption efficiency of the biosorbent as it created an electrostatic repulsion effect against the positively charged malachite green molecules. At the high pH level, the positively charged active sites on the biosorbent were deprotonated and became negatively charged. These negatively charged active sites enhanced the biosorption capacity of the biosorbent material by retaining the positively charged malachite green molecules well due to the force of electrostatic attraction [Citation37]. Therefore, the optimum level of pH was determined to be 8.
3.4. Effect of biosorbent dosage
The effect of the biosorbent amount on malachite green biosorption capacity of the biosorbent material is shown in Figure . The biosorption performance of the biosorbent material decreased from 66.490 to 31.630 mg g−1 as the amount of the biosorbent increased from 10 to 30 mg. At the high biosorbent amount, the overlapping of the biosorbent particles and the fact that a large number of the active binding sites remained empty caused a decrease in malachite green biosorption capacity of the biosorbent. However, when malachite green biosorption performance of the biosorbent was examined as a percentage, it was observed that the biosorption capacity of the biosorbent increased as the biosorbent amount increased (data not shown). This increase in the biosorption efficiency of the biosorbent was due to the availability of larger surface area and higher numbers of the active binding sites with rising the biosorbent amount [Citation38]. Thus, the optimum amount of the biosorbent for malachite green biosorption was selected to be 10 mg.
3.5. Kinetics study for malachite green biosorption
The biosorption kinetics study was carried out to evaluate malachite green treatment manner of the biosorbent material using the following models.
(3)
(3)
(4)
(4)
(5)
(5) where k1 (min−1), k2 (g mg−1 min−1), C (mg g−1) and kp (mg g−1 min−1/2) are the pseudo-first-order rate constant, the pseudo-second-order rate constant, a parameter about the boundary layer thickness and the intra-particle diffusion rate constant, respectively. The results of kinetics study for malachite green biosorption are shown in Table . The biosorption kinetics for the treatment of malachite green from wastewater environment followed the pseudo-second-order model (R2: 0.996). This result indicated that the chemisorption mechanism, which included the electron exchanging or sharing between the active sites on the biosorbent and malachite green molecules, dominated the biosorption operation [Citation39, Citation40].
Table 1. Results of kinetics study for malachite green biosorption.
The effect of the intra-particle diffusion on malachite green biosorption is presented in Figure . The movement (mass transfer) of malachite green molecules from aqueous medium onto the biosorbent particles consisted of three stages. The first stage was the external (film) diffusion, in which malachite green molecules were transported from the solution bulk phase to the outer surface of the biosorbent. The second stage was the intra-particle diffusion, in which malachite green molecules were transported from the external surface of the biosorbent into its pores. The final stage referred to the biosorption (surface reaction), in which malachite green molecules were retained by the biosorbent. Furthermore, the curve of malachite green biosorption capacity (qt) against √t was non-linear and did not pass through the point of origin. These findings revealed that there were multiple stages (mechanisms) controlling malachite green treatment process [Citation36, Citation41].
3.6. Thermodynamics study for malachite green biosorption
The biosorption thermodynamics analysis was conducted to assess the feasibility and spontaneity of malachite green removal process using the standard Gibbs free energy change thermodynamics parameter by the following equations.
(6)
(6)
(7)
(7) where ΔG° (kJ mol−1), R (J mol−1 K−1), T (K), KD and Ceb (mg L−1) are the standard Gibbs free energy change, the gas constant, the temperature, the distribution coefficient and malachite green concentration on the biosorbent at equilibrium, respectively. The standard Gibbs free energy change was obtained in the range of −8.472 kJ mol−1 and −7.357 kJ mol−1 for malachite green biosorption process. The negative standard Gibbs free energy change indicates that the biosorption process is of a spontaneous and feasible nature [Citation42]. Again, the negative standard Gibbs free energy change parameter between −400 kJ mol−1 and −80 kJ mol−1 shows a chemisorption process, and between −20 kJ mol−1 and 0 kJ mol−1 indicates a physisorption process [Citation43]. Thus, for malachite green biosorption, the standard Gibbs free energy change ranging from −8.472 kJ mol−1 to −7.357 kJ mol−1 showed a physical, spontaneous and feasible treatment process.
3.7. Isotherm study for malachite green biosorption
The biosorption isotherm study was performed to evaluate malachite green treatment behaviour of the biosorbent using the following equations.
(8)
(8)
(9)
(9)
(10)
(10)
(11)
(11)
(12)
(12)
(13)
(13) where KF (mg g−1 (L mg−1)1/nF), nF, qm (mg g−1), KL (L mg−1), RL, B (mol2 kJ−2),
and E (kJ mol−1) are a parameter about malachite green biosorption capacity of the biosorbent material, a parameter about the biosorption intensity, the maximum malachite green biosorption efficiency, a parameter about the biosorption energy, the equilibrium (separation) factor, a parameter about the energy of biosorption, the Polanyi potential and the mean free energy, respectively. The results of isotherm study for malachite green biosorption are listed in Table . For the treatment of malachite green from aquatic medium, the biosorption isotherm was well described by Freundlich model with an R2 of 0.991. This result indicated that a multi-layer biosorption mechanism operated between malachite green molecules and the active binding sites on the heterogeneous surface of biosorbent material [Citation44]. Further, nF, RL and E parameters for malachite green biosorption were calculated as 1.414, 0.335–0.602 and 0.990 kJ mol−1, respectively. nF parameter (Freundlich isotherm model) between 0 and 10 shows a favourable biosorption process [Citation37]. Again, RL parameter (Langmuir isotherm model) between 0 and 1 also indicates a favourable biosorption operation [Citation9]. On the other hand, E parameter (Dubinin-Radushkevich isotherm model) between 0 and 8 kJ mol−1 refers a physisorption process, and between 8 and 16 kJ mol−1 refers a chemisorption process [Citation35]. These findings indicated a physical and favourable biosorption process for malachite green treatment.
Table 2. Results of isotherm study for malachite green biosorption.
3.8. SEM and FTIR studies
The surface features and active site profile of biosorbent material were investigated by SEM and FTIR experiments to characterize the interaction mechanism between malachite green molecules and the biosorbent surface. Figure presents the image of SEM for the biosorbent (a) and malachite green-laden biosorbent (b). SEM study showed an irregular and uneven biosorbent surface morphology. Such a surface structure provided the biosorbent material with sufficient space for the biosorption of a large number of malachite green molecules [Citation16, Citation30, Citation45, Citation46]. The change in surface morphology of the biosorbent after malachite green biosorption resulted from the binding of malachite green molecules to the surface of the biosorbent. Figure displays the spectrum of FTIR for the biosorbent (blue) and malachite green-loaded biosorbent (red). The main FTIR spectrum bands observed on the biosorbent material were at the positions of 3333, 2922, 2854, 2378, 2349, 2309, 1730 cm−1, 1603, 1508, 1417, 1317, 1259, 1028, 894, 648 and 599 cm−1. These FTIR bands showed the presence of chemical groups such as O–H, C–C, N–H, C–H, C=O, C–N, C=C, C–O and C–O–C, which are in the structure of biological macromolecules in the biosorbent material [Citation3, Citation9, Citation13, Citation35, Citation36, Citation47]. FTIR characterization study indicated that the biosorbent surface was rich in active site. The change in FTIR spectrum of the biosorbent after malachite green biosorption showed that this rich active site composition played an important role in malachite green treatment operation.
3.9. Performance comparison study for malachite green biosorption
Table presents a literature review comparing the performance of various biosorbent materials for malachite green biosorption from aqueous medium. The biosorbent exhibited a good biosorption performance compared to its competitors, with a capacity of 117.745 mg g−1. The comparison study indicated that the biosorbent material could be a promising candidate for the effective, economical, eco-friendly and sustainable treatment of malachite green micropollutant from wastewater medium.
Table 3. Performance comparison study for malachite green biosorption.
4. Conclusions
The treatment of malachite green, a model micropollutant with a wide range of uses, was first investigated using a cost-efficient and environmentally friendly biosorbent material obtained from P. coccinea M. J. Roemer in this study. The maximum biosorption efficiency was obtained at contact time of 360 mins, malachite green charge of 15 mg L−1, pH of 8 and biosorbent amount of 10 mg. The biosorption kinetics followed the pseudo-second-order model (R2: 0.996), while the biosorption isotherm followed Freundlich model (R2: 0.991). Thermodynamics study showed a physical, spontaneous and feasible treatment process. The SEM and FTIR experiments study indicated that the biosorbent had an irregular and uneven surface morphology rich in active site. The biosorbent material exhibited a good biosorption performance compared to its competitors. Finally, the present study showed that P. coccinea M. J. Roemer-based natural material could be a promising biosorbent for the low-cost, effective and environmentally friendly treatment of malachite green-contaminated wastewater environment.
Disclosure statement
No potential conflict of interest was reported by the author(s).
Data availability statement
All data generated or analysed during the study are included in the article.
References
- Gautam RK, Jaiswal N, Singh AK, et al. Ultrasound-enhanced remediation of toxic dyes from wastewater by activated carbon-doped magnetic nanocomposites: analysis of real wastewater samples and surfactant effect. Environ Sci Poll Res. 2021;28:36680–36694. doi:10.1007/s11356-021-13256-3
- Bharath Balji G, Surya A, Govindaraj P, et al. Utilization of fly ash for the effective removal of hazardous dyes from textile effluent. Inorg Chem Commun. 2022;143:109708. doi:10.1016/j.inoche.2022.109708
- Mahadevan H, Nimina PVM, Krishnan KA. An environmental green approach for the effective removal of malachite green from estuarine waters using Pistacia vera L. shell-based active carbon. Sustain Water Resour Manag. 2022;8:38. doi:10.1007/s40899-022-00612-5
- Sarreshtehdar Aslaheh H, Poursattar Marjani A, Gozali Balkanloo P. Pelargonium as a cost-effective additive in bio-composite adsorbent in removing dyes from wastewater: equilibrium, kinetic, and thermodynamic studies. J Polym Environ. 2023;31:3230–3247. doi:10.1007/s10924-023-02794-1
- Adenan NH, Lim YY, Ting ASY. Removal of triphenylmethane dyes by Streptomyces bacillaris: a study on decolorization, enzymatic reactions and toxicity of treated dye solutions. J Environ Manage. 2022;318:115520. doi:10.1016/j.jenvman.2022.115520
- Giri BS, Sonwani RK, Varjani S, et al. Highly efficient bio-adsorption of malachite green using Chinese fan-palm biochar (Livistona chinensis). Chemosphere. 2022;287:132282. doi:10.1016/j.chemosphere.2021.132282
- Pathy A, Krishnamoorthy N, Chang SX, et al. Malachite green removal using algal biochar and its composites with kombucha SCOBY: an integrated biosorption and phycoremediation approach. Surf Interfaces. 2022;30: 101880. doi:10.1016/j.surfin.2022.101880
- Singh AK, Gautam RK, Agrahari S, et al. Graphene oxide supported Fe3O4-MnO2 nanocomposites for adsorption and photocatalytic degradation of dyestuff: ultrasound effect, surfactants role and real sample analysis. Int J Environ Anal Chem. 2022:1–27. doi:10.1080/03067319.2022.2091930
- Asghari E, Saraji M. Evaluating cottonwood seeds as a low-cost biosorbent for crystal violet removal from aqueous matrics. Int J Phytorem. 2023;25:137–145. doi:10.1080/15226514.2022.2064816
- El-Kammah M, Elkhatib E, Gouveia S, et al. Cost-effective ecofriendly nanoparticles for rapid and efficient indigo carmine dye removal from wastewater: adsorption equilibrium, kinetics and mechanism. Environ. Technol Innov. 2022;28:102595.
- El-Kammah M, Elkhatib E, Gouveia S, et al. Enhanced removal of indigo carmine dye from textile effluent using green cost-efficient nanomaterial: adsorption, kinetics, thermodynamics and mechanisms. Sustain Chem Pharm. 2022;29:100753. doi:10.1016/j.scp.2022.100753
- Bukhari A, Ijaz I, Zain H, et al. Removal of eosin dye from simulated media onto lemon peel-based low cost biosorbent. Arab J Chem. 2022;15:103873.
- Wang Z, Chen H, Gao X, et al. A novel self-floating cyclodextrin-modified polymer for cationic dye removal: preparation, adsorption behavior and mechanism. Sep Purif Technol. 2022;290:120838. doi:10.1016/j.seppur.2022.120838
- Gul A, Muhammad S, Nawaz S, et al. Ficus religiosa bark an efficient adsorbent for alizarin red s dye: equilibrium and kinetic analysis. J Iran Chem Soc. 2022;19:1737–1746. doi:10.1007/s13738-021-02413-7
- Deng Q, Wang X, Shao M, et al. Synthesis of chitosan-modified magnetic metal-organic framework and its adsorption of Congo red and antibacterial activity. Microporous Mesoporous Mater. 2022;342:112042. doi:10.1016/j.micromeso.2022.112042
- Kumar MR, King P, Wolde Z, et al. Application of optimization response surface for the biosorption of crystal violet dye from textile wastewater onto Clerodendrum fragrans leaves. Biomass Convers Biorefin. 2022. doi:10.1007/s13399-13022-02419-y
- Shah AJ, Soni B, Karmee SK. Application of cotton stalk biochar as a biosorbent for the removal of malachite green and its microwave-assisted regeneration. Energy Ecol Environ. 2022;7:88–96. doi:10.1007/s40974-021-00217-2
- Gautam RK, Goswami M, Mishra RK, et al. Biochar for remediation of agrochemicals and synthetic organic dyes from environmental samples: a review. Chemosphere. 2021;272:129917. doi:10.1016/j.chemosphere.2021.129917
- Vélez-Gavilán J. Pyracantha coccinea (scarlet firethorn). Wallingford: CABI Publishing; 2020. Available from: cabi.org/isc/datasheet/45994
- Ulmer R, Couty A, Eslin P, et al. The firethorn (Pyracantha coccinea), a promising dead-end trap plant for the biological control of the spotted-wing Drosophila (Drosophila suzukii). Biol Control. 2020;150:104345. doi:10.1016/j.biocontrol.2020.104345
- Dong C, Li X, Xi Y, et al. Micropropagation of Pyracantha coccinea. HortScience. 2017;52:271–273. doi:10.21273/HORTSCI11301-16
- Deniz F. A novel green solution to metal pollution in aquatic environment: Pyracantha coccinea M. J. Roemer. J Taibah Univ Sci. 2023;17:2170678. doi:10.1080/16583655.2023.2170678
- Deniz F. An eco-sustainable and effective treatment practice by Pyracantha coccinea M. J. Roemer for food wastewater carrying synthetic dye. Biomass Convers Biorefin 2023. doi:10.1007/s13399-13023-04421-13394
- Deniz F, Tezel Ersanli E. An efficient biosorbent material for green remediation of contaminated water medium. Int J Phytorem 2023. doi:10.1080/15226514.15222023.12191742
- Akar T, Ozkara E, Celik S, et al. Chemical modification of a plant origin biomass using cationic surfactant ABDAC and the biosorptive decolorization of RR45 containing solutions. Colloids Surf B Biointerfaces. 2013;101:307–314. doi:10.1016/j.colsurfb.2012.06.016
- Gorgulu Ari A, Celik S. Biosorption potential of orange G dye by modified Pyracantha coccinea: batch and dynamic flow system applications. Chem Eng J. 2013;226:263–270. doi:10.1016/j.cej.2013.04.073
- Ho YS. Review of second-order models for adsorption systems. J Hazard Mater. 2006;136:681–689. doi:10.1016/j.jhazmat.2005.12.043
- Lagergren S. About the theory of so-called adsorptıon of soluble substances. K Sven Vetenskapsakad Handl. 1898;24:1–39.
- Weber WJ, Morris JC. Kinetics of adsorption on carbon from solution. J Sanit Eng Div Am Soc Civ Eng. 1963;89:31–60. doi:10.1061/JSEDAI.0000430
- Chikri R, Elhadiri N, Benchanaa M, et al. Applications of sugar scum-sawdust composite for dye removal from aqueous solutions: kinetic and isotherm studies. Biomass Convers Biorefin. 2021. doi:10.1007/s13399-13021-02110-13398
- Freundlich HMF. Over the adsorption in solution. Z Phys Chem. 1906;57:385–470.
- Dubinin MM, Radushkevich LV. Equation of the characteristic curve of activated charcoal. Proc Acad Sci Phys Chem Sec USSR. 1947;55:331–333.
- Langmuir I. The adsorption of gases on plane surfaces of glass, mica and platinum. J Am Chem Soc. 1918;40:1361–1403. doi:10.1021/ja02242a004
- Al-Zaban MI, Alharbi NK, Albarakaty FM, et al. Experimental modeling investigations on the biosorption of methyl violet 2b dye by the brown seaweed Cystoseira tamariscifolia. Sustainability. 2022;14:5285. doi:10.3390/su14095285
- Alouache A, Selatnia A, Sayah HE, et al. Biosorption of hexavalent chromium and Congo red dye onto Pleurotus mutilus biomass in aqueous solutions. Int J Environ Sci Technol. 2022;19:2477–2492. doi:10.1007/s13762-021-03313-2
- Salah Omer A, El Naeem GA, Abd-Elhamid AI, et al. Adsorption of crystal violet and methylene blue dyes using a cellulose-based adsorbent from sugercane bagasse: characterization, kinetic and isotherm studies. J Mater Res Technol. 2022;19:3241–3254. doi:10.1016/j.jmrt.2022.06.045
- Sukumar S, Arockiasamy S, Moothona MC. Removal of cationic dye from aqueous solution using recombinant gellan gum-coated MgO nanoparticles. Chem Pap. 2022;76:3155–3168. doi:10.1007/s11696-021-02035-6
- Miyah Y, Benjelloun M, Salim R, et al. Experimental and DFT theoretical study for understanding the adsorption mechanism of toxic dye onto innovative material Fb-HAp based on fishbone powder. J Mol Liq. 2022;362:119739. doi:10.1016/j.molliq.2022.119739
- Al-Ghamdi YO. Immobilization of cellulose extracted from Robinia pseudoacacia seed fibers onto chitosan: chemical characterization and study of methylene blue removal. Arab J Chem. 2022;15:104066.
- Mehmandost N, Goudarzi N, Chamjangali MA, et al. Removal of methylene blue and crystal violet in binary aqueous solution by magnetic Terminalia catappa kernel shell biosorbent using Box-Behnken design. J Iran Chem Soc. 2022;19:3769–3781. doi:10.1007/s13738-022-02552-5
- Sulthana R, Taqui SN, Syed UT, et al. Biosorption of crystal violet by nutraceutical industrial fennel seed spent equilibrium, kinetics, and thermodynamic studies. Biocatal Agric Biotechnol. 2022;43:102402. doi:10.1016/j.bcab.2022.102402
- Khan TA, Siddiqui MF, Abbasi N, et al. Adsorptive decolouration of anionic dye from water by goat dropping activated carbon prepared via microwave-assisted H3PO4 activation: process optimization using response surface methodology, isotherm and kinetics modelling. Biomass Convers Biorefin. 2022;12:5409–5425. doi:10.1007/s13399-022-02766-w
- Khezami L, Aissa MAB, Modwi A, et al. Efficient removal of organic dyes by Cr-doped ZnO nanoparticles. Biomass Convers Biorefin. 2022. doi:10.1007/s13399-13022-02952-w
- Bonyadi Z, Nasoudari E, Ameri M, et al. Biosorption of malachite green dye over Spirulina platensis mass: process modeling, factors optimization, kinetic, and isotherm studies. Appl Water Sci. 2022;12:167. doi:10.1007/s13201-022-01690-8
- Bensalah J, Benhiba F, Habsaoui A, et al. The adsorption mechanism of the anionic and cationic dyes of the cationic resin A®IRC-50, kinetic study and theoretical investigation using DFT. J Indian Chem Soc. 2022;99:100512. doi:10.1016/j.jics.2022.100512
- Homagai PL, Poudel R, Poudel S, et al. Adsorption and removal of crystal violet dye from aqueous solution by modified rice husk. Heliyon. 2022;8:e09261. doi:10.1016/j.heliyon.2022.e09261
- Narayan M, Sadasivam R, Packirisamy G, et al. Electrospun polyacrylonitrile-Moringa olifera based nanofibrous bio-sorbent for remediation of Congo red dye. J Environ Manage. 2022;317:115294. doi:10.1016/j.jenvman.2022.115294
- Ebrahimi F, Nabavi SR, Omrani A. Fabrication ofhydrophilic hierarchical PAN/SiO2 nanofibers by electrospray assisted electrospinning for efficient removal of cationic dyes. Environ Technol Innov. 2022;25:102258. doi:10.1016/j.eti.2021.102258
- Khalatbary M, Sayadi MH, Hajiani M, et al. Adsorption studies on the removal of malachite green by γ-Fe2O3/MWCNTs/Cellulose as an eco-friendly nanoadsorbent. Biomass Convers Biorefin. 2022. doi:10.1007/s13399-13022-02475-13394
- Al-Musawi TJ, Arghavan SMA, Allahyari E, et al. Adsorption of malachite green dye onto almond peel waste: a study focusing on application of the ANN approach for optimization of the effect of environmental parameters. Biomass Convers Biorefin. 2022. doi:10.1007/s13399-13021-02174-13396
- Bayram O, Köksal E, Gode F, et al. Decolorization of water through removal of methylene blue and malachite green on biodegradable magnetic Bauhinia variagata fruits. Int J Phytorem. 2022;24:311–323. doi:10.1080/15226514.2021.1937931
- Deniz F, Bural H. Sustainable environmental remediation approach for biocide removal from water medium: a model biosorption study using activated biological waste. Int J Phytorem. 2021;23:111–118. doi:10.1080/15226514.2020.1798872