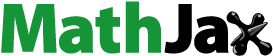
Abstract
Nowadays, waste discharge and contaminants in drinking water have emerged as a significant global problem. Therefore, it is necessary to remove these pollutants from the water and photocatalysis is the best technique for this purpose. The co-precipitation technique was employed to produce NiMn2O4 and ZnMn2O4 and a photocatalyst containing NiMn2O4/ZnMn2O4 heterostructure nanocomposite. Various analytical techniques have been ascribed to examine the physical, morphological and optical features of the fabricated catalysts. XRD diffraction peak patterns were utilized to confirm the presence of cubic NiMn2O4, tetragonal ZnMn2O4 and NiMn2O4/ZnMn2O4 phases in a nanocomposite. In photodegradation tests, the nanocomposite catalyst outperformed the individual catalysts. After 60 minutes of visible light exposure, this nanocomposite catalyst eliminated the methylene blue (MB) dye, attaining substantially higher degradation rates than pure NiMn2O4 (66%) and ZnMn2O4 (77%). The nanocomposite catalyst was highly effective against methylene blue (MB) dye with a degradation efficiency of 92%.
1. Introduction
In addition to dyes and pharmaceutical chemicals, industrial pollutants contribute significantly to the pollution problem because their hazardous components are complex and challenging to degrade [Citation1–5]. Developing cutting-edge wastewater treatment technology is essential to safeguarding the environment, public health, and sustainability [Citation2, Citation6–8]. Photocatalysis modifies the redox potentials of light-generated charge pairs and is a standard technique for degrading substances [Citation9, Citation10]. Another benefit of the approach is its negligible environmental influence [Citation11–15]. Developing and manufacturing photocatalysts capable of treating dye and pharmaceutical effluents at high efficiency is essential for ecological remediation and public health [Citation16, Citation17]. Recently, spinel has gained popularity for its use in H2 evolution from splitting of water, supercapacitors, carbon dioxide (CO2) reduction, and organic pollutant degradation [Citation18–20].
Modifying the structure and composition of spinel semiconductor materials can influence their physical and chemical properties, such as economical, higher chemical stability, different chemical states, durability, and they can easily attribute to dyes surfaces [Citation21–24]. NiMn2O4 [Citation25], CoMn2O4 [Citation26], CuAl2O4 [Citation27], NiAl2O4 [Citation28], and ZnMn2O4 [Citation29] are examples of spinel-type metal manganese oxides that have recently attained remarkable attention due to their potential uses [Citation17, Citation23, Citation30–32]. Due to their adaptable structure, morphology, and chemistry, metal manganese oxides have been widely utilized in various actions [Citation33–36]. However, nickel magnetite (NiMnO4) spinel oxide has only been examined and reported on by a few scientists. NiMn2O4 (NMO) rapidly recombines the photogenerated electron–hole pair (e-/h+), producing modest photocatalytic performance in visible light. Several ways have been discovered to intensify the photocatalytic performance of NMO, such as noble metal deposition, bandgap modification, and morphological alteration [Citation19, Citation37–39]. Recently, Luo et al. created and designed a LaFeO3/SnS2 nanomaterial utilizing a basic hydrothermal process using the direct Z-scheme. Due to presence of Z-scheme heterojunction among LaFeO3 & SnS2, this catalyst exhibits exceptional photocatalytic properties [Citation40]. As a result, the material was able to absorb more visible light, meanwhile, recombination occurring among electrons as well as holes was reduced [Citation41–43]. Building heterostructures using the Z-scheme is an excellent method for modifying charge separation [Citation44]. A Z-scheme heterojunction consists of dual semiconductors with appropriately positioned energy bands to maximize the uncoupled efficacy of photo-induced carriers while maintaining their robust redox capabilities. It is crucial to locate appropriate semiconductors for establishing a Z-scheme heterojunction [Citation17, Citation45]. In addition to keeping the correct charge redox potential, this would allow for strong redox behaviour at the interface of light-generated isolated carriers [Citation46, Citation47].
Heterojunction coupling has increased photocatalytic efficiency because interfacial charge transfer in NiMn2O4 facilitates the separation of photogenerated charge carriers [Citation48]. Two-component heterojunctions exhibited more photocatalytic activity than their single-component counterparts. Consequently, the photocatalytic effectiveness of Z-scheme composites containing nanoparticles was dramatically enhanced. This technology enhanced the carrier separation efficiency of Z-scheme heterojunctions [Citation49]. The two-component oxide dramatically increased visible light absorption, which significantly enhanced the system's photocatalytic activity [Citation50]. Consequently, when exposed to visible light, the binary nanocomposite exhibited exceptional MB degrading properties [Citation51].
The NiMn2O4/ZnMn2O4 nanocomposite offers a novel and exceptionally successful advancement in field of photocatalytic degradation of dye. The synergistic combination of two unique spinel oxide materials, NiMn2O4 and ZnMn2O4, results in improved photocatalytic activity due to their complimentary optical and electrical characteristics. The heterostructured nanocomposite not only broadens the absorption spectrum, allowing for the utilization of a wider variety of light wavelengths, but it also allows for separation of charge and its transfer, lowering recombination rates and increasing the formation of reactive species. This complex interaction at the nanoscale between NiMn2O4 and ZnMn2O4 not only improves the degradation efficiency of various organic dyes under visible light irradiation, but also shows the potential for designing advanced multifunctional nanomaterials with exceptional photocatalytic performance for environmental remediation applications.
A two-step approach was employed to study the photocatalytic activity of ZnMn2O4/NiMn2O4 under photodegradation of (MB) organic dye. According to our knowledge, it has not been reported as a composite for photocatalytic degradation of organic dye. Furthermore, being a superior visible-light photocatalyst, NiMn2O4 (NMO) is a p-type semiconductor having a small bandgap. Despite its limited specific surface area, this catalyst has a high electron–hole recombination efficiency. The present material shows the linear value of slope “k”, denotes the kinetic rate constant for the deprivation of organic pollutants, as well as the regression coefficient (R2) values for all the nano-catalysts NiMn2O4/ZnMn2O4 (0.993) nanocomposite, because the values are so near to one, the absence of organic dye is considered a first-order response. This resultant nanocomposite has high degradation efficiency of 92% under visible light compared to the others.
2. Experimental procedure
2.1. Chemicals and reagents
All the chemicals including nickel nitrate hexahydrate (Ni(NO3)2. 6H2O, Merck, 98.5%, CAS# 13478-00-7), manganese nitrate hexahydrate (Mn(NO3)2. 6H2O, Sigma Aldrich, 98%, CAS# 15710-66-4), zinc chloride (ZnCl2, Sigma Aldrich, 98%, CAS#7646-85-7), potassium hydroxide (KOH, Duksan, 85%, CAS#1310-58-3), ethanol (C2H5OH, Merck, 99%, CAS#64-17-5) were utilized as they received without any prior purification.
2.2. Synthesis of NiMn2O4 and ZnMn2O4 nanoparticles
For the fabrication of NiMn2O4, nickel nitrate (0.1 M) and manganese nitrate (0.1 M) were mixed in 100 ml of ultrapure deionized water and 20 ml of 4 M KOH solution was also poured into it under constant stirring to achieve a pH of 11 to obtained required precipitates. These precipitates were cleaned with deionized water as well as with ethanol through filtration. These obtained precipitates were allowed to dehydrate in an electric oven at 80°C for 12 h and then sintered at 700°C in a muffle electric furnace for three hours. The annealed product was kept to conduct characterization. The same procedure has been adopted for synthesizing the ZnMn2O4 using zinc chloride and manganese nitrate hexahydrate as a precursor.
2.3. Synthesis of NiMn2O4/ZnMn2O4 nanocomposite
As a part of this experiment, 100 ml of ethanol was poured into a beaker along with 1 mg of NiMn2O4 and ZnMn2O4 nanoparticles prepared in advance. Following the ultrasonic agitation for 45 min with heat treatment of 60°C, the nanoparticles were evenly distributed throughout the beaker, and for 7 h, the reaction mixture was stirred ferociously. After washing, the finally obtained product was dehydrated in the oven at 80°C for 12 h. The nanocomposite synthesis has been revealed in Scheme 1.
2.4. Physical characterization
Powder X-ray diffraction (XRD) was used to validate the crystalline and structural characteristics of the synthesized NiMn2O4, ZnMn2O4 and NiMn2O4/ZnMn2O4 nanocomposite. An Advanced Bruker D-8 X-ray system was used to record the transition peak pattern using a Cu-Kα radiations diffractometer. Fourier transform infrared spectroscopy (FTIR) performed on (Nicolet 170SXFTIR) provides the functional group information. To evaluate the nanoparticle size and dispersion, topographical images of the nanocomposite material were obtained using scanning electron microscopy (SEM) joined with energy dispersive spectroscopy (EDS). A diffuse reflectance spectrum (DRS) was performed by Shimadzu UV-2550 to investigate the photocatalytic dye degradation identified by UV-visible spectrophotometry. For the Electrochemical impedance spectroscopy (EIS), performance was analysed on the Autolab PGSTAT-204 workstation.
2.5. Photocatalytic setup
All photocatalytic properties were performed in a commercial photo-reactor system having a 200 W tungsten lamp. Furthermore, the photocatalytic performance of the created catalysts (NiMn2O4, ZnMn2O4 and NiMn2O4/ZnMn2O4) was investigated at room temperature. For this challenge, (MB) was nominated as the representative organic dye. To accelerate the degradation process, 100 ml of 20 mg/L of solution of dye was used with 10 mg of each nanocatalyst. Firstly, this solution and catalyst were mixed for 15 min to maintain the equilibrium process. After that, to track the degradation process, the changes in dye concentration were checked using a UV-visible spectrophotometer after every 15 min for two hours under visible light. The percentage degradation of resultant solution was estimated via Equation (1).
(1)
(1) In this C0 specifies the initial concentration, Ct shows its concentration at a given time of the results of polluted water.
3. Results and discussion
3.1. Structural analysis
The PXRD peak patterns of NiMn2O4, ZnMn2O4, and NiMn2O4/ZnMn2O4 nanocomposite were exhibited in Figure (a). There are 2θ values of 18.01°, 30.15°, 35.08°, 42.94°, 47.04°, 52.96°, 65.01°, 70.17°, and 74.11°, which are related to the lattice planes (111), (220), (311), (400), (331), (422), (531), (620), and (622), respectively and matched with the JCPDS No. 01-071-0852, the diffraction peaks related with the phase of cubic NiMn2O4 with the Fd-3 m space group [Citation52]. The ZnMn2O4 diffraction peaks of 2θ at 18.06°, 29.16°, 32.94°, 36.05°, 44.09°, 50.17°, 56.07°, 63.95°, 68.03°, and 76.07°, may also be associated to the lattice planes of (101), (112), (103), (211), (220), (204), (303), (116), (411), and (413), given a tetragonal structure with a I41/ space group (JCPDS: 01-077-0470) [Citation53]. There were no additional crystalline phases in the nanomaterials produced confirming the single phase of each material. The presence of peaks of these materials in the XRD pattern of nanocomposite confirm its successful formation. The mean crystallite size of NiMn2O4, ZnMn2O4 and NiMn2O4/ZnMn2O4 was estimated using Scherer's Equation (2) [Citation54–56].
(2)
(2) In this, D, λ, β, θ, and k stand for size of the crystal, X-ray beam wavelength, the width of the diffraction line at its maximum half-intensity, Bragg angle along with geometric form factor, usually 0.90. The diameters of the crystallites of NiMn2O4, ZnMn2O4 and NiMn2O4/ZnMn2O4 were 62, 48, and 39 nm, accordingly.
Figure 1. (a) XRD peak pattern, (b) FTIR pattern of the prepared NiMn2O4, ZnMn2O4 and NiMn2O4/ZnMn2O4.

Figure (b) depicts the FT-IR spectra of NiMn2O4, ZnMn2O4 and NiMn2O4/ZnMn2O4 between 4000 and 500 cm−1. The absorption of surface-bound hydroxyl ions by water molecules may result in the two peaks at 3420 cm−1 & 1827 cm−1, which are thought to be ?>produced by the O-H stretching mode and H-O-H bending mode [Citation57], correspondingly. There are some additional vibration peaks ranging 1400-1500 cm−1 indicating C = O due to the carbon dioxide available in the atmosphere. The metal–oxide (M-O) vibration of the Nickel-Oxide & Manganese-Oxide ions at tetrahedral sites is responsible for the 586 and 975 cm−1 bands in NiMn2O4 [Citation58]. All metal oxide (M-O) stretching vibration of the Zinc-Oxide and Manganese-Oxide are attributed at 655 cm−1 & 1133 cm−1 [Citation59]. The composite (NiMn2O4/ZnMn2O4) include all the peaks present in the pure NiMn2O4 and ZnMn2O4.
3.2. Morphological analysis
The shape and size of generated NiMn2O4, ZnMn2O4, NiMn2O4/ZnMn2O4 nanocrystals were assessed via FESEM analysis. The NiMn2O4 has the nanocrystal like morphology (Figure a) while ZnMn2O4 has the nanoflake like structure (Figure b) and then the NiMn2O4/ZnMn2O4 nanocomposite has the nanoflake embedded nanocrystal like structure (Figure c). This type of the morphology was also confirmed via TEM analysis as mentioned in Figure (d), indicating the internal sight view of the composite formation due to the presence of both phases. Which is helpful for degradation of MB dye because of presence of porous sites at the catalyst surface. The purity of this nanocomposite was assessed via energy-dispersive X-rays spectroscopy. The peaks of Mn, Zn, Ni, Mn, and O components of the synthesized nano-catalysts present in the EDX spectrum of NiMn2O4/ZnMn2O4 nanocomposite (Figure e) confirms the successful synthesis as also confirmed via XRD analysis. The ratio of the individual element has been confirmed by using following relation:
The ratio of elements calculated from the above relation is given in Table and their experimental ratios are in better agreement with that of the theoretical one.
Figure 2. SEM images of (a) NiMn2O4, (b) ZnMn2O4, (c) NiMn2O4/ZnMn2O4, (d) TEM image of the composite material and (e) EDX of the synthesized composite.

Table 1. EDX elemental compositional analysis for all the fabricated materials.
The specific surface area of NiMn2O4, ZnMn2O4 and NiMn2O4/ZnMn2O4 composites was determined using BET study. The nitrogen sorption data collected from the samples showed type IV isotherms, as shown in Figure (a). NiMn2O4, ZnMn2O4 and NiMn2O4/ZnMn2O4 specific BET surface areas were determined around 33.6, 25 m2/g &15 m2/g, accordingly. The specific surface area of the NiMn2O4/ZnMn2O4 nanocomposite reduced following decorating with ZnMn2O4 nanoparticles due to the limited exposure of the NiMn2O4 surface. The calculated pore volume of the synthesized samples, Such as NiMn2O4, ZnMn2O4, and NiMn2O4/ZnMn2O4 were 0.005, 0.013, 0.038 cm3/g, respectively, as mentioned in Figure (b). The results indicate higher pore volume of the nanocomposite causes many voids on its surface, increasing the amount of active sites, which are helpful for the photochemical reaction.
3.3. Optical study
The optical band gap (Eg) of all designed materials was examined using UV-visible spectra, as shown in Figure . The resultant spectra indicated an absorbance peak at 412, 425, and 441 nm for NiMn2O4, ZnMn2O4 and NiMn2O4/ZnMn2O4, respectively, confirming that the peaks were present in the visible region, and nanocomposite shows the higher capability for absorption. In general, the formula of Tauc is given in Equation (3) to calculate the band gap value [Citation60].
(3)
(3) In this A is an optical transition-dependent constant, hv denotes the input photon energy, and α is absorption coefficient. According to Figure , Tauc's plot indicates the deviation between the (αhv)2 and photon energy (hv) plots govern the Eg values for generated material. The fabricated materials like NiMn2O4, ZnMn2O4 and NiMn2O4/ZnMn2O4 were found to have Eg values of 2.6, 2.5 eV, &2.01 eV, accordingly. NiMn2O4/ZnMn2O4 displayed a smaller energy bandgap than NiMn2O4 and ZnMn2O4, according to the findings. This indicates that the smaller band gap will be responsible for the maximum visible light absorption in NiMn2O4/ZnMn2O4, which confirms the catalyst applicability for photocatalytic activity under sunlight, as 45% part of our solar spectrum is visible light.
The EIS spectra of NiMn2O4, ZnMn2O4 and NiMn2O4/ZnMn2O4 are shown in Figure . The charge’s speeds were determined using the size of the arcs in the EIS spectra. It is widely assumed that the smaller the charge transfer resistance, the smaller the arc radius. Because of its smaller arc radius, nanocomposite has less resistance to charge migration than pure NiMn2O4 and ZnMn2O4. The resultant charge transfer resistance of all the synthesized products like NiMn2O4, ZnMn2O4 and NiMn2O4/ZnMn2O4 nanocomposite were 9, 5.6, and 0.5 Ω, accordingly. The lower the Rct (charge transfer resistance) greater will be the photocatalytic activity of this fabricated materials. The electron lifetime before recombination was calculated using the following Equation [Citation61]:
(4)
(4) In this te is the average time perior to the recombination of e-/h+ and fmax denotes maximum frequency at the mid-frequency range's peak. The fmax and te values for the synthesized nanofibers are displayed in Table . The NiMn2O4/ZnMn2O4 has the longest lifetime of the group, mostly due to lowered rate of e-/h+ recombination. ZnMn2O4 may serve as traps, speeding up the charge transition process.
Table 2. Charge transfer resistance and lifetime of electron calculated from EIS of all the synthesized materials.
3.4. Evaluation of photocatalytic activity of all the synthesized products
The photocatalytic mechanism comprisis the three phases occurring on the surfaces of semiconductor materials during photocatalytic processes: (1) effective charge carrier separation, (2) photocatalytic reaction, and (3) significant light absorption [Citation62–64]. The photocatalytic experiments on NiMn2O4, ZnMn2O4, and NiMn2O4/ZnMn2O4 nanocomposite were performed. The degradation of organic methylene blue dye in occurrence of visible light was studied. The addition of NiMn2O4, ZnMn2O4, and NiMn2O4/ZnMn2O4 gradually decreases the UV-Vis maximum absorption of MB (670 nm) dye over the duration of 80 min under the visible light illumination as depicted in Figure (a–c). The absorbance of MB declines as exposure time increases, and no new absorbance bands resulted in the UV-Vis absorption. This demonstrates that when exposed to visible light, MB organic dye practically entirely degrade, implying that the NiMn2O4/ZnMn2O4 heterostructures formed are effective in photodegrading organic dye as mentioned in Figure (d). The C/Co of the MB dye was plotted versus time, and the photocatalyst samples spent absorbing light as shown in Figure (e). The percentage degradation efficiency of (MB) dye has calculated using this formula R = [(Co - Ct) / Co] × 100%, where Co and Ct indicate the starting concentrations of MB and the dye concentration at time (t), accordingly.
Figure 6. Absorption spectra variation of MB dye in existence of (a) NiMn2O4/ZnMn2O4, (b) ZnMn2O4, (c), NiMn2O4, (d) degradation efficiency (%), (e) plot of Co/Ct vs. irradiation time, (f) rate constant plots.

Table shows the degradation efficacy of NiMn2O4, ZnMn2O4, and NiMn2O4/ZnMn2O4 nano catalysts for MB dye. The NiMn2O4/ZnMn2O4 heterostructure nanocomposite displays remarkable photodegradation efficiency as compared to already reported materials as shown in Table . The Langmuir Hinshelwood first-order kinetic model was seen when MB was photo-catalytically degraded in the presence of NiMn2O4/ZnMn2O4 nano-catalysts, and ln (Ct/Co) = kt gives the reaction rate. Figures (f) depict the evolution of ln (Ct/Co). Table shows the regression coefficient (R2) values of the NiMn2O4 (0.989), ZnMn2O4 (0.992), and NiMn2O4/ZnMn2O4 (0.984) nano-catalysts, as well as linear slope value “k”, denotes the kinetic rate constant for deprivation of organic contaminants. These values suggest the deprivation of organic dye is the first order reaction as values are nearly equal to unity. Based on the experiment data and explanation presented, Figure depicts a proposed photocatalytic reaction pathway that may assist to explain why the current NiMn2O4/ZnMn2O4 have superior photocatalytic activity. NiMn2O4/ZnMn2O4 had higher photocatalytic activity than pure NiMn2O4 and ZnMn2O4. This might occur if h+ from ZnMn2O4 valence band (VB) goes to NiMn2O4 and e- from NiMn2O4 conduction band (CB) moves to ZnMn2O4 surface. Each of these phenomena is due to exposure of visible light. As a result, the production of NiMn2O4/ZnMn2O4 heterostructure substantially reduces the electron/hole recombination while increasing their photocatalytic activity. Furthermore, holes inside VB of NiMn2O4 generate active species that aids in oxidation of surface-attached water molecules, and as a result •OH are created. The photo-excited electrons of the ZnMn2O4 in the CB will combine with oxygen to form superoxide anions, that are required for dye reduction. UV experiment demonstrate that NiMn2O4/ZnMn2O4 nano-catalysts have remarkable photocatalytic properties, and this is because of the band gap of NiMn2O4/ZnMn2O4 as they delay the electron recombination, and the concentration of NiMn2O4/ZnMn2O4 nano-catalyst grows, the reconnection rate of e-/h+ pairs decrease.
Table 3. Comparison of the percent degradation and kinetic study.
Table 4. Percentage degradation comparison of prepared nanocomposite with already reported data.
A trapping test was conducted to better comprehend the operation of the nanocomposite which decreases the degradation efficiency from 92% to 38.0%, 72%, and 30.4%, engaging the p-benzoquinone as an •O-2 scavenger, EDTA-2Na acting as a h+ scavenger, and tertiary-butanol as scavenger of •OH [Citation74] as depicted in Figure (a). In this case, the •OH is the principal reactant species in the decomposition of MB, while •O2 and h+ are subsidiary reactant species. This was supported by prior investigations that shown the use of a •OH scavenger decreased photocatalysis efficiency by 30.4% as displayed in Figure (a).
Figure 8. (a) Scavenger test, (b) cyclic stability, (c) MB and TOC removal at optimized process and (d) post stability test XRD

Its stability and reusability of a photocatalytic catalyst are crucial. As a result, the composite material underwent five consecutive runs. Figure (b) demonstrates that the photocatalytic performance of composite materials does not vary significantly over time. Total organic carbon (TOC) analysis has performed to evaluate mineralization efficiency. Figure (c) shows the methylene blue dye and TOC removal under optimized circumstances. It was observed that dye degraded under these particular circumstances, with a degradation efficiency of 92% and a TOC removal of 78%. The results indicate that MB could Figure (d) illustrates the XRD pattern of the nanocomposite material after five consecutive runs. This was done to determine the structural stability of nanocomposite material. According to the XRD pattern, all peaks associated with composite materials were still visible, but their intensities were significantly less. This demonstrates the stability and reproducibility of the photocatalyst.
4. Conclusion
Herein, by employing the efficient, low-cost coprecipitation approach, novel NiMn2O4/ZnMn2O4 heterojunction nano-catalyst was created. All the fabricated materials were analysed via various analytical tools to confirms the structure, purity, morphology, and optical properties. Using visible light, fabricated nano-catalysts were subsequently employed to destroy MB dye molecules as an organic pollutant. Among all the NiMn2O4/ZnMn2O4 exhibit a higher photocatalytic degradation efficiency of 92% than pure ZnMn2O4 (77%) and NiMn2O4 (66%) due to lower band gap of nanocomposite (2.01 eV). Also, this may be due to their ability to separate charge carriers, diminish high light absorption, and allow photo-excited e-/h+ couples to migrate away from the constrictive heterojunction interface. As a result, the NiMn2O4/ZnMn2O4 act as visible-light-sensitive photocatalysts and might be utilized to clean the environment by photo-catalytically decomposing organic contaminants.
Acknowledgements
K.F.F express appreciation to the Deanship of Scientific Research at King Khalid University Saudi Arabia for funding through research groups program under grant number R.G.P. 2/376/44. Princess Nourah bint Abdulrahman University Researchers Supporting Project number (PNURSP2024R55), Princess Nourah bint Abdulrahman University, Riyadh, Saudi Arabia.
Disclosure statement
No potential conflict of interest was reported by the author(s).
Additional information
Funding
References
- Liu L, Chen Z, Zhang J, et al. Treatment of industrial dye wastewater and pharmaceutical residue wastewater by advanced oxidation processes and its combination with nanocatalysts: a review. J Water process Eng. 2021;42:102122-19. doi:10.1016/j.jwpe.2021.102122
- Akhundi A, Habibi-Yangjeh A, Abitorabi M. Review on photocatalytic conversion of carbon dioxide to value-added compounds and renewable fuels by graphitic carbon nitride-based photocatalysts. Catal Rev. 2019;61(4):595–628. doi:10.1080/01614940.2019.1654224
- Munawar T, Sardar S, Mukhtar F, et al. Fabrication of fullerene-supported La2O3–C60 nanocomposites: dual-functional materials for photocatalysis and supercapacitor electrodes. Phys Chem Chem Phys. 2023;25(9):7010–7027. doi:10.1039/D2CP05357H
- Nisa MU, Abid AG, Gouadria S, et al. Boosted electron-transfer/separation of SnO2/CdSe/Bi2S3 heterostructure for excellent photocatalytic degradation of organic dye pollutants under visible light. Surf Interf. 2022;31:102012-13. doi:10.1016/j.surfin.2022.102012
- Taghavi Fardood S, Ramazani A, Asiabi P. A novel green synthesis of copper oxide nanoparticles using a henna extract powder. J Struct Chem. 2018;59:1737–1743. doi:10.1134/S0022476618070302
- Kurade MB, Ha Y-H, Xiong J-Q, et al. Phytoremediation as a green biotechnology tool for emerging environmental pollution: a step forward towards sustainable rehabilitation of the environment. Chem Eng J. 2021;415:129040-19. doi:10.1016/j.cej.2021.129040
- Sojobi AO, Zayed J. Impact of sewer overflow on public health: a comprehensive scientometric analysis and systematic review. Environ Res. 2022;203:111609-29. doi:10.1016/j.envres.2021.111609
- Munawar T, Fatima S, Nadeem MS, et al. Tunability of physical properties of NiO by the introduction of rare earth metal (Y, Ho) dual doping for natural sunlight-driven photocatalysis. J Mater Sci: Mater Electr. 2023;34(7):1–24. doi:10.1007/s10854-023-10095-5
- Guo R-t, Wang J, Bi Z-x, et al. Recent advances and perspectives of g–C3N4–based materials for photocatalytic dyes degradation. Chemosphere; 2022:133834-17.
- Yu H, Jiang L, Wang H, et al. Modulation of Bi2MoO6-based materials for photocatalytic water splitting and environmental application: a critical review. Small. 2019;15(23):1901008-30. doi:10.1002/smll.201901008
- Tolliver C, Keeley AR, Managi S. Drivers of green bond market growth: the importance of nationally determined contributions to the Paris agreement and implications for sustainability. J Cleaner Prod. 2020;244:118643-15. doi:10.1016/j.jclepro.2019.118643
- Wang L, Zhu Z, Wang F, et al. State-of-the-art and prospects of Zn-containing layered double hydroxides (Zn-LDH)-based materials for photocatalytic water remediation. Chemosphere. 2021;278:130367-20. doi:10.1016/j.chemosphere.2021.130367
- Moradnia F, Fardood ST, Ramazani A, et al. Green synthesis of recyclable MgFeCrO4 spinel nanoparticles for rapid photodegradation of direct black 122 dye. J Photochem Photobiol A. 2020;392:112433-6. doi:10.1016/j.jphotochem.2020.112433
- Moradnia F, Fardood ST, Ramazani A, et al. Magnetic Mg0. 5Zn0. 5FeMnO4 nanoparticles: green sol-gel synthesis, characterization, and photocatalytic applications. J Cleaner Prod. 2021;288:125632-13. doi:10.1016/j.jclepro.2020.125632
- Moradnia F, Taghavi Fardood S, Ramazani A, et al. Green sol–gel synthesis of CoMnCrO4 spinel nanoparticles and their photocatalytic application. Micro Nano Lett. 2020;15(10):674–677. doi:10.1049/mnl.2020.0189
- Al-Tohamy R, Ali SS, Li F, et al. A critical review on the treatment of dye-containing wastewater: Ecotoxicological and health concerns of textile dyes and possible remediation approaches for environmental safety. Ecotoxicol Environ Saf. 2022;231:113160-17. doi:10.1016/j.ecoenv.2021.113160
- Akhundi A, Zaker Moshfegh A, Habibi-Yangjeh A, et al. Simultaneous dual-functional photocatalysis by g-C3N4-based nanostructures. ACS ES&T Engineering. 2022;2(4):564–585. doi:10.1021/acsestengg.1c00346
- Ali SA, Ahmad TJ. Chemical strategies in molybdenum based chalcogenides nanostructures for photocatalysis. Int J Hydrogen Energy. 2022;47:29255–29283.
- Akhundi A, Badiei A, Ziarani GM, et al. Graphitic carbon nitride-based photocatalysts: toward efficient organic transformation for value-added chemicals production. Molecular Catalysis. 2020;488:110902-14. doi:10.1016/j.mcat.2020.110902
- Mukhtar F, Munawar T, Nadeem MS, et al. Multi metal oxide NiO-Fe2O3-CdO nanocomposite-synthesis, photocatalytic and antibacterial properties. Appl Phys A. 2020;126:1–14. doi:10.1007/s00339-019-3176-6
- Alahmari F, Almessiere M, Ünal B, et al. . Electrical and optical properties of Ni0· 5Co0. 5-xCdxNd0. 02Fe1·78O4 (x≤ 0.25) spinel ferrite nanofibers. Ceram Int. 2020;46(15):24605–24614. doi:10.1016/j.ceramint.2020.06.249
- Mukhtar F, Munawar T, Nadeem MS, et al. Enhancement in carrier separation of ZnO-Ho2O3-Sm2O3 hetrostuctured nanocomposite with rGO and PANI supported direct dual Z-scheme for antimicrobial inactivation and sunlight driven photocatalysis. Adv Powder Technol. 2021;32(10):3770–3787. doi:10.1016/j.apt.2021.08.022
- Mukhtar F, Munawar T, Nadeem MS, et al. Highly efficient tri-phase TiO2–Y2O3–V2O5 nanocomposite: Structural, optical, photocatalyst, and antibacterial studies. Journal of Nanostructure in Chemistry. 2022;12:547–564.
- Mukhtar F, Munawar T, Nadeem MS, et al. Enhanced sunlight-absorption of Fe2O3 covered by PANI for the photodegradation of organic pollutants and antimicrobial inactivation. Adv Powder Technol. 2022;33(8):103708-15. doi:10.1016/j.apt.2022.103708
- Rohokale MS, Dhabliya D, Sathish T, et al. A novel two-step co-precipitation approach of CuS/NiMn2O4 heterostructured nanocatalyst for enhanced visible light driven photocatalytic activity via efficient photo-induced charge separation properties. Phys B. 2021;610:412902-7. doi:10.1016/j.physb.2021.412902
- Wang L, Zhao X, Lu Y, et al. CoMn2O4 spinel nanoparticles grown on graphene as bifunctional catalyst for lithium-air batteries. J Electrochem Soc. 2011;158(12):A1379-8 . doi:10.1149/2.068112jes
- Hassanzadeh-Tabrizi S, Pournajaf R, Moradi-Faradonbeh A, et al. Nanostructured CuAl2O4: Co-precipitation synthesis, optical and photocatalytic properties. Ceram Int. 2016;42(12):14121–5. doi:10.1016/j.ceramint.2016.06.026
- Sahli N, Petit C, Roger A-C, et al. Ni catalysts from NiAl2O4 spinel for CO2 reforming of methane. Catal Today. 2006;113(3-4):187–193. doi:10.1016/j.cattod.2005.11.065
- Luo L, Qiao H, Chen K, et al. Fabrication of electrospun ZnMn2O4 nanofibers as anode material for lithium-ion batteries. Electrochim Acta. 2015;177:283–289. doi:10.1016/j.electacta.2015.01.100
- Tomas M. Corrosion of ferritic stainless steels used in solid oxide fuel cells-investigation of novel coatings in single-and dual-atmosphere conditions: chalmers Tekniska Hogskola (Sweden); 2022.
- Taghavi Fardood S, Ramazani A, Golfar Z, et al. Green synthesis using tragacanth gum and characterization of Ni–Cu–Zn ferrite nanoparticles as a magnetically separable catalyst for the synthesis of hexabenzylhexaazaisowurtzitane under ultrasonic irradiation. J Struct Chem. 2018;59:1730–1736. doi:10.1134/S0022476618070296
- Kiani MT, Ramazani A, Taghavi Fardood S. Green synthesis and characterization of Ni0. 25Zn0.75Fe2O4 magnetic nanoparticles and study of their photocatalytic activity in the degradation of aniline. Appl Organomet Chem. 2023;37(4):7053-11. doi:10.1002/aoc.7053
- Zhu S, Ho S-H, Jin C, et al. Nanostructured manganese oxides: natural/artificial formation and their induced catalysis for wastewater remediation. Environmental Science: Nano. 2020;7(2):368–396. doi:10.1039/C9EN01250H
- Sabri M, Habibi-Yangjeh A, Rahim Pouran S, et al. Titania-activated persulfate for environmental remediation: the-state-of-the-art. Catalysis Review. 2023;65:118–173.
- Elshypany R, Selim H, Zakaria K, et al. Elaboration of Fe3O4/ZnO nanocomposite with highly performance photocatalytic activity for degradation methylene blue under visible light irradiation. Environmental Technology & Innovation. 2021;23:101710-13. doi:10.1016/j.eti.2021.101710
- Elshypany R, Selim H, Zakaria K, et al. Magnetic ZnO crystal nanoparticle growth on reduced graphene oxide for enhanced photocatalytic performance under visible light irradiation. Molecules. 2021;26(8):2269-16. doi:10.3390/molecules26082269
- Li Z, Wang K, Zhang J, et al. Enhanced photocatalytic activity of hierarchical Bi2WO6 microballs by modification with noble metals. Catalysts. 2022;12(2):130-13. doi:10.3390/catal12020130
- Nada AA, Bekheet MF, Roualdes S, et al. Functionalization of MCM-41 with titanium oxynitride deposited via PECVD for enhanced removal of methylene blue. J Mol Liq. 2019;274:505–515. doi:10.1016/j.molliq.2018.10.154
- Nada AA, Nasr M, Viter R, et al. Mesoporous ZnFe2O4@ TiO2 nanofibers prepared by electrospinning coupled to PECVD as highly performing photocatalytic materials. J Phys Chem C. 2017;121(44):24669–24677. doi:10.1021/acs.jpcc.7b08567
- Garcia-Muñoz P, Fresno F, de la Peña O’Shea VA, et al. Ferrite materials for photoassisted environmental and solar fuels applications. In: Munoz-Batista MJ, Munoz AN, Luque R, editors. Heterogeneous photocatalysis recent advances. Cham: Springer.
- Dang X, Xie M, Dai F, et al. Ultrathin 2D/2D ZnIn2S4/g-C3N4 nanosheet heterojunction with atomic-level intimate interface for photocatalytic hydrogen evolution under visible light. Adv Mater Inter. 2021;8(10):2100151-9.
- Samira M, Hassana HH, El-Maghrabid HH, et al. Fabrication of titanium dioxide/reduced graphene oxide nanofibers for photodegradation of tartrazine dye in aqueous solution. Desal water Treat. 2022;255:110–119. doi:10.5004/dwt.2022.28327
- Nada AA, Tantawy HR, Elsayed MA, et al. Elaboration of nano titania-magnetic reduced graphene oxide for degradation of tartrazine dye in aqueous solution. Solid State Sci. 2018;78:116–125. doi:10.1016/j.solidstatesciences.2018.02.014
- Hou L, Li W, Wu Z, et al. Embedding ZnCdS@ ZnIn2S4 into thiazole-modified g-C3N4 by electrostatic self-assembly to build dual Z-scheme heterojunction with spatially separated active centers for photocatalytic H2 evolution and ofloxacin degradation. Sep Purif Technol. 2022;290:120858-14. doi:10.1016/j.seppur.2022.120858
- Hassani A, Krishnan S, Scaria J, et al. Z-scheme photocatalysts for visible-light-driven pollutants degradation: a review on recent advancements. Current Opinion in Solid State & Material Science. 2021;25(5):100941-25. doi:10.1016/j.cossms.2021.100941
- Li Y, Gu M, Zhang X, et al. 2D g-C3N4 for advancement of photo-generated carrier dynamics: status and challenges. Mater Today. 2020;41:270–303. doi:10.1016/j.mattod.2020.09.004
- Munawar T, Nadeem MS, Mukhtar F, et al. Transition metal-doped SnO2 and graphene oxide (GO) supported nanocomposites as efficient photocatalysts and antibacterial agents. Environ Sci Pollut Res. 2022;29(60):90995–91016. doi:10.1007/s11356-022-22144-3
- Mohammed R, Ali MEM, Gomaa E, et al. Promising MoS2-ZnO Hybrid nanocomposite photocatalyst for antibiotics, and dyes remediation in wastewater applications. Environ Nanotechn, Monit Manag. 2023;19:100772-14.
- Wang J, Wang M, Kang J, et al. The promoted tetracycline visible-light-driven photocatalytic degradation efficiency of g-C3N4/FeWO4 Z-scheme heterojunction with peroxymonosulfate assisting and mechanism. Sep Purif Technol. 2022;296:121440-12. doi:10.1016/j.seppur.2022.121440
- Zhang W, Ma Y, Zhu X, et al. Fabrication of Ag decorated g-C3N4/LaFeO3 Z-scheme heterojunction as highly efficient visible-light photocatalyst for degradation of methylene blue and tetracycline hydrochloride. J Alloys Compd. 2021;864:158914-12. doi:10.1016/j.jallcom.2021.158914
- Mohammad A, Khan ME, Cho MH, et al. Fabrication of binary SnO2/TiO2 nanocomposites under a sonication-assisted approach: tuning of band-gap and water depollution applications under visible light irradiation. Ceram Int. 2021;47(11):15073–15081. doi:10.1016/j.ceramint.2021.02.152
- Heo JN, Son N, Shin J, et al. Efficient hydrogen production by low-temperature steam reforming of propane using catalysts with very small amounts of Pt loaded on NiMn2O4 particles. Int J Chem Reactor Eng. 2020;45(41):20904–20921.
- Taghavi Fardood S, Moradnia F, Ramazani A. Green synthesis and characterisation of ZnMn2O4 nanoparticles for photocatalytic degradation of Congo red dye and kinetic study. Micro Nano Lett. 2019;14(9):986–991. doi:10.1049/mnl.2019.0071
- Alam U, Verma NJC, Physicochemical SA. Direct Z-scheme-based novel cobalt nickel tungstate/graphitic carbon nitride composite: enhanced photocatalytic degradation of organic pollutants and oxidation of benzyl alcohol. Colloids Surf, A. 2021;630:127606-12. doi:10.1016/j.colsurfa.2021.127606
- Mukhtar F, Munawar T, Nadeem MS, et al. Dual S-scheme heterojunction ZnO–V2O5–WO3 nanocomposite with enhanced photocatalytic and antimicrobial activity. Mat Chem Phys. 2021;263:124372-13. doi:10.1016/j.matchemphys.2021.124372
- Bashir A, Munawar T, Mukhtar F, et al. Dual-functional fullerene supported NiO-based nanocomposite: efficient electrocatalyst for OER and photocatalyst for MB dye degradation. Mat Chem Phys. 2023;293:126886-14. doi:10.1016/j.matchemphys.2022.126886
- Nisa MU, Gouadria S, Houda S, et al. Coral like gadolinium doped hematite nanostructure as stable and robust electrocatalyst for oxygen evolution water splitting. Fuel. 2023;338:127313-11. doi:10.1016/j.fuel.2022.127313
- Brunold TC, Tamura N, Kitajima N, et al. Spectroscopic study of [Fe2 (O2)(OBz) 2 {HB (pz ‘) 3} 2]: nature of the μ-1, 2 Peroxide− Fe (III) bond and its possible relevance to O2 activation by non-heme iron enzymes. J Am Chem Soc. 1998;120(23):5674–5690. doi:10.1021/ja980129x
- Nisa MU, Manzoor S, Abid AG, et al. CdSe supported SnO2 nanocomposite with strongly hydrophilic surface for enhanced overall water splitting. Fuel. 2022;321:124086-10. doi:10.1016/j.fuel.2022.124086
- Idris AO, Mafa PJ, Oseghe EO, et al. A facile approach for the preparation of NiONPs@ MnO2 NRs nanocomposite material and its photocatalytic activity. J Nanopart Res. 2021;23:1–14. doi:10.1007/s11051-020-05135-8
- Nada AA, Bekheet MF, Viter R, et al. BN/GdxTi (1-x) O (4-x)/2 nanofibers for enhanced photocatalytic hydrogen production under visible light. Appl Catal, B. 2019;251:76–86. doi:10.1016/j.apcatb.2019.03.043
- Zuo Z, Li Y. Emerging electrochemical energy applications of graphdiyne. Joule. 2019;3(4):899–903. doi:10.1016/j.joule.2019.01.016
- Xue Y, Huang B, Yi Y, et al. Anchoring zero valence single atoms of nickel and iron on graphdiyne for hydrogen evolution. Nat Commun. 2018;9(1):1460-10. doi:10.1038/s41467-018-03896-4
- Zhang L, Mohamed HH, Dillert R, et al. Kinetics and mechanisms of charge transfer processes in photocatalytic systems: a review. Journal of Photochemistry and Photobiology C. 2012;13(4):263–276. doi:10.1016/j.jphotochemrev.2012.07.002
- Hammache Z, Soukeur A, Omeiri S, et al. Physical and photo-electrochemical properties of MgFe2O4 prepared by sol gel route: application to the photodegradation of methylene blue. J Mater Sci: Mater Electron. 2019;30:5375–5382. doi:10.1007/s10854-019-00830-2
- Charradi K, Ahmed Z, BenMoussa MA, et al. A facile approach for the synthesis of spinel zinc ferrite/cellulose as an effective photocatalyst for the degradation of methylene blue in aqueous solution. Cellulose. 2022;29:2565–2576.
- Ajithkumar Mohana P, Sumathi S. Synthesis, characterization, optical and photocatalytic activity of yttrium and copper co-doped zinc ferrite under visible light. J Mater Sci: Mater Electron. 2020;31:1168–1182. doi:10.1007/s10854-019-02628-8
- Abroshan E, Farhadi S, Zabardasti A. Novel magnetically separable Ag3PO4/MnFe2O4 nanocomposite and its high photocatalytic degradation performance for organic dyes under solar-light irradiation. Sol Ener Mater Sol Cell. 2018;178:154–163. doi:10.1016/j.solmat.2018.01.026
- Padmapriya G, Manikandan A, Krishnasamy V, et al. Spinel NixZn1− xFe2O4 (0.0 ≤ x≤ 1.0) nano-photocatalysts: synthesis, characterization and photocatalytic degradation of methylene blue dye. J Mol Struct. 2016;1119:39–47. doi:10.1016/j.molstruc.2016.04.049
- Hosseini S, Azizi N. New insight into highly efficient CSA@g-C3N4 for photocatalytic oxidation of benzyl alcohol and thioanisole: NAEDS as a promoter of photoactivity under blue LED irradiation. PhotoChem. PhotoBio. 2023: 1–214966.
- Shao R, Sun L, Tang L, et al. Preparation and characterization of magnetic core–shell ZnFe2O4@ ZnO nanoparticles and their application for the photodegradation of methylene blue. Chem Eng J. 2013;217:185–191. doi:10.1016/j.cej.2012.11.109
- Sharma R, Singhal S. Structural, magnetic and electrical properties of zinc doped nickel ferrite and their application in photo catalytic degradation of methylene blue. Phys B. 2013;414:83–90. doi:10.1016/j.physb.2013.01.015
- Agboola PO, Shakir I, Haider S. Development of internal electric field induced NiFe2O4/CdO pn nano-heterojunctions for solar light activated photodegradation of methylene blue dye. Ceram Int. 2022;48(10):13572–9. doi:10.1016/j.ceramint.2022.01.236
- Nadeem MS, Munawar T, Mukhtar F, et al. Facile synthesis of sunlight driven photocatalysts Zn0. 9Ho0. 05M0. 05O (M = Pr, Sm, Er) for the removal of synthetic dyes from wastewater. Surfaces and Interfaces. 2022;34:102376-14. doi:10.1016/j.surfin.2022.102376