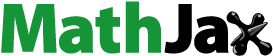
Abstract
With the aid of a solution casting technique, we created a blended film made of polyvinyl alcohol (PVA) and polyvinyl pyrrolidone (PVP). Gamma doses (5-40 kGy) were applied to samples of the synthetic PVA/PVP film. UV spectroscopy and the CIE colour alterations procedure were used to investigate the impact of the γ dose on the optical features of the synthesized film. The direct optical bandgap (Eg), affected by cross-linking, destroyed the crystalline structure and formed the amorphous regions, dropped from 2.53 to -2.08 eV with the increase in γ dose to 40 kGy. Thus, the PVA/PVP film's compact structure increases. The films displayed a transition which was directly allowed as the ϵ'' vs. hν plots revealed Eg approximately equal to the values derived from Tauc's equation. The colour variances, between the unaltered and irradiated films, were also identified. Significant colour differences could be seen in the pure PVA/PVP film.
1. Introduction
One of the appealing fields that caught the interest of scientists is polymer blend. It introduces a common method to give materials new properties that are much better than those of each polymer [Citation1,Citation2]. The ease of synthesis and the ability to alter the composition to control the physical properties of polymer blends are beneficial [Citation3]. The miscibility of the components of the blended polymers enhances the polymer's properties [Citation4]. Repulsion between segments within the blend, charge transfers for homopolymers and the forces between dipoles are examples of specific interactions that can lead to miscibility [Citation5].
Water-soluble polyvinyl alcohol (PVA) has good strength, a high capacity for storing charge and excellent optical behaviour [Citation6]. Its backbone chain has carbon and an OH function group that serve as a starting point for hydrogen bonds that help create polymer compounds [Citation6]. Polyvinyl alcohol has a broad optically bandgap plus excellent chemical resistances, thus its blend can be used in numerous requests in optical membranes [Citation7,Citation8].
When comparing polyvinyl pyrrolidone (PVP) with other polymers, PVP has a higher proportion of amorphous phases that enable rapid ionic mobility [Citation9]. PVP has good properties of film-forming, is water soluble and biodegradable [Citation10] and works well with PVA. Additionally, PVP is used in cathartic water and suspension physical stabilization [Citation11,Citation12]. Due to the addition of small qualities of PVP, it can combine with PVA to form a compact blend [Citation13]. PVA and PVP composition was studied by Mondal and Mandal [Citation14] to improve the final membrane's thermal stability for high-temperature applications. Lilleby Helberg et al. [Citation15] stated that the combination of PVA and PVP gives advantages for improved mechanical properties of PVA and elevated hydrophilicity of PVP. This enhances the ability of the polymeric matrices to retain water and hold carriers in their membrane.PVA can produce good films when combined with other polymers, according to [Citation16–18]. According to [Citation15], the homogeneity of the PVA/PVP blend and the miscibility of the polymers can be confirmed by the appearance of the sole glass transitional temperature. Singh et al. stated that the PVA/PEG/PVP blend's single glass transition indicates the creation of miscible blends [Citation19].
The γ irradiation, on the other hand, is crucial to help treat the deficits induced in the structure during polymerization. The radiation breaks the chains in the polymer and thus creates chemically reactive free radicals that then cause the chains to crosslink and form new bonds. The macromolecular constitutions of the polymer are affected by this [Citation20].
Optoelectronic device applications heavily rely on polymer optical behaviour [Citation21]. Additionally, the γ-irradiated polymers’ colour change is a crucial characteristic that aids in understanding how the properties of the polymer have changed. It can be applied to commercially viable needs, such as industry and dosimeter fields [Citation22]. The present work aimed to investigate the improvements in optical behaviour that can occur in PVA by blending with PVP polymer. Then it uses gamma radiation to enhance the optical character of the blend polymer, which combines the characteristics of PVA and PVP, to be used in optoelectronic devices.
2. Experimental
2.1. Samples
The PVA (Mw: 27,000 g/mol) and PVP (Mw: 55,000 g/mol) were provided by Sigma-Aldrich GmbH, Egypt and Sigma Aldrich, China, respectively.
2.2. Obtaining PVA/PVP film
The PVA/PVP (9:1) blend was created by thoroughly stirring 90 ml of distilled water with 0.9 g of PVA at 80°C till a clear solution was created. The solution was then allowed to cool to room temperature (RT). Following that, the PVP solution was created by separately combining 0.1 g with 10 ml of distilled water and stirring at RT to produce pure solutions. After 45 min of vigorous stirring, the PVA and PVP solutions were instantly combined at RT. The outcome was then poured into Petri dishes to dry. The remaining solvents were then removed by keeping the formed films in an electric oven at 30oC for two days. The produced film had an average thickness of 0.35 mm. A prior study [Citation23] employed the same method of preparation.
2.3. Irradiation tool
Designed by Atomic Energy of Canada, Ltd., the 60Co source was employed in this study at 1 kGy/h dose rate for irradiating the films at the Egyptian Atomic Energy Authority. The samples were radioactively irradiated.
2.4. Experimental device
The UV/Vis absorbance spectra were captured through an 1800 spectrophotometer manufactured by Tomos Life Science Group, China. The CIE colour alternations method was used to determine how the pristine film differed in colour from the irradiated ones. All detailed information concerning calculations was given in a prior article [Citation24].
3. The obtained results and their discussion
3.1. Study of absorbance
The values of absorbance of the non-irradiated and the irradiated samples were recorded (Figure ). With increasing wavelength, it can be seen that the absorbance is decreasing. This can be attributable to the excited n−π* transitions between the energy states and the π− π* phenyl rings for the decrease in absorbance with wavelength [Citation25]. This was controlled by the amount of conjugated phenyl rings in the PVA chain [Citation26]. The increase in γ dose led to the increase of absorbance. This could be due to the effect of γ radiation that alters the energy states [Citation27]. As a result of energy transfer created by γ radiation, crosslinks were achieved, resulting in the development of a new chemical configuration [Citation28]. Additionally, the absorption of UV light allows the split of molecules into their singlet or tripled states, which also initiates photochemical interactions in the polymeric matrix and increases absorbance [Citation29]. Since the intermediate atoms of the blend have likely absorbed the energy of the incident photons, the increase in absorbance with dose probably indicates this.
3.2. Study of the bandgap relations
The values of bandgap (Eg) were estimated using Tauc's formula [Citation30].
(1)
(1)
where hν is the energy of the photon,
is the absorption coefficient and B is a constant. To calculate the absorption coefficients, the following formula was used:
(2)
(2)
where D is the thickness of the samples and n is an index point to the kind of microelectronic transitions. It is 1/2 or 3/2 when the transition is direct and 2 or 3 when the transition is indirect. This depends on whether the transition is allowed or forbidden [Citation31]. Representing (αhν)1/n against hυ with prolonging the linear portion to the hν axis will allow us to calculate the Eg. The Tauc's relation, which was used to determine Eg for n = 1/2, is shown in Figure . When the γ dose was raised to 40 kGy, the values of Eg decreased (Table ). The rise of amorphous phases in the samples is what we believe is responsible for the decrease in Eg. As Eg falls, localized states expand and the Eg structure develops flaws that start microelectronic transitions. Gamma radiation causes crosslinking that leads to a decrease in Eg values [Citation32].
Table 1. Values of Eg obtained from Tauc’s relations and ε″ vs. hυ of the PVA/PVP blend films as a function of γ dose.
The Eg values attained from Tauc's model were compared to those obtained using the optical dielectric loss function (Figures and Figure ) as ε″ depends on the band construction of its material. Additionally, studying ε″ by using UV spectra helps in understanding the band construction of the material and the transitions among occupied and unoccupied levels [Citation33]. Aziz and others [Citation33] confirmed the existence of directly allowed transition, as indicated by the Eg values determined using (ε″) against hν relation. These values were close to the Eg values determined through Tauc's relation when considering n = 1/2. Consequently, Eg is direct. In another article [Citation31] they discovered that the Eg values computed using the relation ε″ against hν were closely equal to those of Tauc's principle when considering n = 3/2, but Eg of their pure sample, obtained using Tauc's principle when considering n = 1/2, were near to the one derived from the ε″ against hν drawing. They cconcluded that for the untreated film, direct transitions were permitted, while for the treated films, direct transitions were prohibited. Brza and colleagues found comparable outcomes. [Citation34]. The Eg values estimated from the relation ε″ against hν were close to those estimated from Tauc's plot when considering n = 3/2. The transition was expressly forbidden. Their conclusion was that the optical ε″ is used in accurately determining Eg and investing the band structure.
The next equation was applied to compute ε ′′.
(3)
(3)
where n is the refractive index, k is a constant called the extinction coefficient (
, λ is the wavelength). k provides details about the incident photons’ dispersals which can be produced from the absorption and scattering paths per unit length of the target material. Using the following equation, the refractive index could be determined.
(4)
(4)
The values of the reflectance (R) could be esteemed by applying the transmission records (T), calculated using the absorbance (A) spectrum, applying the formula:
. Figure shows the spectra of ε″ that were estimated for the blend and irradiated films. The Eg values computed using the ε″ against hν drawings were found to be near to the values computed using Tauc’s equation considering n = 3/2, as shown in Table , specifying that the blended film showed forbidden transition [Citation31].
The measurements of the dielectric parameters yield knowledge about the materials’ optical characteristics [Citation35,Citation36]. The change of dielectric loss ε″ indicates the change of refractive index with dose. This was expected since n is related to the production of bonds. So, it is expected that n increases with raising the gamma doses due to cross-linking.
3.3. Colour change investigations
The red, green and blue lights were substituted by a set of mathematical lights, X, Y and Z, which are named the colour's tristimulus values [Citation37]. Additionally, a normalization procedure was defined to determine an additional set of chromaticity coordinates (x, y and z) that specify saturation. The transmission data measured in the wavelength range from 370 to 780 nm are shown in Figure and the colour parameters were computed by applying the CIE technique. The obtained values are represented in Table .
Table 2. The tristimulus values and chromaticity coordinates of PVA/PVP blend films as a function of γ dose.
The CIE L* a* b* colour space from 1976 is heavily applied in many industries. Using the CIE colour relations, the L*, a* and b* intercepts were calculated. The green–red, blue–yellow and dark-white axes are denoted by the a*, b* and L* values. For both a* and b*, respectively, the calculation had an uncertainty of 0.05 and 0.01 for L*. Figure displays the alternations in colour intercepts with γ dose. The intercept a* showed negative numerical values that grew when the dose was increased to 40 kGy, indicating the propensity of the green colour component to transform into red (Figure (a)). b* showed a non-monotonic behaviour. This was connected to the samples’ increasing darkness (L*) as shown in Figure (b).
The benefit of creating a consistent colour space is that it would make it possible to create a useful ruler for measuring the colour difference between two samples. The difference in colour among the unirradiated and irradiated films (colour intensity, ΔE) was determined by applying the CIE colour change equation [Citation24].
The plot of ΔE vs. γ dose is shown in Figure (b). Higher values of ΔE indicate major colour alternations [Citation38].
The free radicals produced from bond rupture activated the conjugation of the double bond, which leads to alternation in colour. The production of excited electrons in the visible range results from discolouration and a drop in transmission. Additionally, the created free radicals have unpaired spin electrons. Optical colouration can be produced by such species [Citation24].
4. Conclusions
The changes in the optical character of the PVA/PVP blended films could be attributed to chain crosslinks that occurred because of the ionizing effect of γ rays. This led to an increase in the amorphous phase and thus a drop in the values of the optical band gap. In addition, the investigation of bandgap proved direct transition in the blended films.
The γ radiation could alter the blended films’ colour. This was due to the conversion of the blue and green colours to yellow and red. Finally, the colour of the blend films became darker. The changes in colour were expected to be permanent because the values of colour intensity were greater than 5.
Disclosure statement
No potential conflict of interest was reported by the author(s).
References
- Nouh SA, Benthami K, Abutalib MM. Modification of structural and optical properties of polyvinyl alcohol/polyethylene glycol thin film by laser irradiation. Radiat Eff Defects Solids. 2016;171:87–95. doi:10.1080/10420150.2015.1135153
- Nouh SA, Benthami K, Abou Elfadl A, et al. Modification induced by gamma irradiation in polystyrene/poly(methyl methacrylate) blends. Int Polym Proces. 2017;32:253–259. doi:10.3139/217.3342
- Peesan M, Supaphol P, Rujiravanit R. Effect of casting solvent on characteristics of hexanoyl chitosan/polylactide blend films. J Appl Polym Sci. 2007;105:1844–1852. doi:10.1002/app.26341
- Rajulu AV, Reddy RL, Raghavendra SM, et al. Miscibility of PVC/PMMA blend by the ultrasonic and refractive index method. Eur Polym J. 1999;35:1183–1186. doi:10.1016/S0014-3057(98)00078-0
- Mohammad SK, Raina AQ, Mian SW. Miscibility studies of PVC/PMMA and PS/PMMA blends by dilute solution Viscometry and FTIR. Afr J Pure Appl Chem. 2008;2:41–45. doi:10.1201/b13410-5
- Sheha E, Khoder H, Shanap TS, et al. Structure, dielectric and optical properties of p-type (PVA/CuI) nanocomposite polymer electrolyte for photovoltaic cells. Optik (Stuttg). 2012;123:1161–1166. doi:10.1016/j.ijleo.2011.06.066
- Virtanen S, Vartianen J, Setala H, et al. Modified nanofibrillated cellulose–polyvinyl alcohol films with improved mechanical performance. RSC Adv. 2014;4:11343–11350. doi:10.1039/c3ra46287k
- Abdelaziz M, Ghannam MM. Influence of titanium chloride addition on the optical and dielectric properties of PVA films. Phys B. 2010;405:958–964. doi:10.1016/j.physb.2009.10.030
- Abdelrazek EM, Abdelghany AM, Badr SI, et al. Structural, optical, morphological and thermal properties of PEO/PVP blend containing different concentrations of biosynthesized Au nanoparticles. J Mater Res Technol. 2018;7:419–419. doi:10.1016/j.jmrt.2017.06.009
- Mallakpour S, Behranvand V. in: V.K. Thakur, M.K. Thakur, R.K. Gupta (Eds.), Hybrid polymer composite materials Applications. Woodhead Publishing is an imprint of Elsevier Ltd; 2017, pp. 263–289.
- Hayama M, Yamamoto KI, Kohori F, et al. How polysulfone dialysis membranes containing polyvinylpyrrolidone achieve excellent biocompatibility? J. Membr Sci. 2004;234:41–49. doi:10.1016/j.memsci.2004.01.020
- Bolong N, Ismail AF, Salim MR. Sustainable membrane technology for energy, water, and environment. NJ: John Wiley & Sons Inc.; 2012.
- Kadajji VG, Betageri GV. Water soluble polymers for pharmaceutical applications. Polymers (Basel). 2011;3:1972–2009. doi:10.3390/polym3041972
- Mondal A, Mandal B. CO2 separation using thermally stable crosslinked poly(vinyl alcohol) membrane blended with polyvinylpyrrolidone/polyethyleneimine/tetraethylenepentamine. J Membr Sci. 2014;460:126–138. doi:10.1016/j.memsci.2014.02.040
- Helberg RML, Dai Z, Ansaloni L, et al. Pva/PVP blend polymer matrix for hosting carriers in facilitated transport membranes: synergistic enhancement of CO2 separation performance. Green Energy Environ. 2020;5:59–68. doi:10.1016/j.gee.2019.10.001
- Bin-Dahman OA, Jose J, Al-Harithi MA. Compatibility of poly (acrylic acid)/starch blends. Starch-Stärke. 2015;67:1061–1069.
- Bin-Dahman, O. A.; Jose, J.; Al-Harithi, M. A. Effect of natural weather aging on the properties of poly (vinyl alcohol)/starch/graphene nanocomposite. Starch-Stärke. 2017, 69, 1600005.
- Bin-Dahman O, Rahaman M, Khastgir D, et al. Electrical and dielectric properties of poly(vinyl alcohol)/starch/graphene nanocomposites. Can J Chem Eng. 2018;96:903–911. doi:10.1002/cjce.22999
- Singh P, Bharati DC, Gupta PN, et al. Vibrational, thermal and ion transport properties of PVA-PVP-PEG-MeSO4Na based polymer blend electrolyte films. J Non-Cryst Solids. 2018;494:21–21. doi:10.1016/j.jnoncrysol.2018.04.052
- Nouh SA, Barakat MM, Benthami K, et al. Synthesis and characterization of PANI-Co/PC nanocomposite: gamma induced changes in the structure and thermal properties. Chem Pap. 2021;75:2197–2205. doi:10.1007/s11696-020-01459-w-020-01459-w
- Naim A, Alnaim A, Ibrahim N, et al. Effect of gamma irradiation on the mechanical properties of PVC/ZnO polymer nanocomposite. J Radiat Res Appl Sci. 2017;10:165–171. doi:10.1016/j.jrras.2017.03.004
- Yamauchi T, Nakai H, Somaki Y, et al. Formation of CO2 gas and OH groups in CR-39 plastics due to gamma-Ray and ions irradiation. Radiat Meas. 2003;36:99–103. doi:10.1016/S1350-4487(03)00102-1
- Nouh SA, Alhazime AA, Benthami K, et al. Structural and optical studies of gamma irradiated polyvinyl alcohol-, polyethylene glycol-, and lignosulfonate thin film. Radiat Eff Defects Solids. 2023. doi:10.1080/10420150.2023.2186865
- Tommalieh MJ, Barakat MME, Bahareth RA, et al. Thermal, optical, and color modification in makrofol VLG 7-1 nuclear track detector due to gamma irradiation. J. Macromol. Sci. B. 2022;61:479–493. doi:10.1080/00222348.2022.2052456
- El-Mesady IA, Rammah YS, Abdalla AM, et al. Gamma irradiation effect towards photoluminescence and optical properties of makrofol DE 6-2. Radiat Phys Chem. 2020;168:108578. doi:10.1016/j.radphyschem.2019.108578
- Eisa WH, Zayed MF, Abdel-Moneam YK, et al. Water-Soluble gold/polyaniline core/shell nanocomposite: synthesis and characterization. Synth Met. 2014;195:23–28. doi:10.1016/j.synthmet.2014.05.012
- Rathore BS, Gaur MS, Singh KS. Investigation of optical and thermally stimulated properties of SiO2 nanoparticles-filled polycarbonate. J Appl Polym Sci. 2012;126:960–968. doi:10.1002/app.37004
- Alhazime AA, Benthami KA, Alsobhi BO, et al. Pani-Ag/PVA nanocomposite: gamma induced changes in the thermal and optical characteristics. J Vinyl Addit Technol. 2021;27:47–53. doi:10.1002/vnl.21782
- Karthikeyan B, Hariharan S, Mangalaraja RV, et al. Studies on NiO-PVA composite films for opto-electronics and optical limiters. IEEE Photon Technol Lett. 2018;30:1539–1542. doi:10.1109/LPT.2018.2859042
- Tauc J. Optical properties of solids, Abeles, F., Eds. North Holland: Elsevier; 1972; p. 77.
- Aziz SB, Abdullah OG, Hussein AM, et al. From insulating PMMA polymer to conjugated double bond behavior: green chemistry as a novel approach to fabricate small band Gap polymers. Polymers (Basel). 2017;9:626. doi:10.3390/polym9110626
- Palija T, Dobi J, Jai M. A photochemical method for improvement of color stability at polymer–wood biointerfaces. Colloids Surf B Biointerfaces. 2013;108:152–157. doi:10.1016/j.colsurfb.2013.02.045
- Aziz SB, Dannoun EMA, Tahir DA, et al. Synthesis of PVA/CeO2 based nanocomposites with tuned refractive index and reduced absorption edge: structural and optical studies. Materials (Basel). 2021;14:1570. doi:10.3390/ma14061570
- Brza MA, Aziz SB, Anuar H, et al. From green remediation to polymer hybrid fabrication with improved optical band gaps. IJMS. 2019;20:3910. doi:10.3390/ijms20163910
- Soylu M, Al-Ghamdi AA, Yakuphanoglu F. Transparent CdO/n-GaN(0001) heterojunction for optoelectronic applications. J Phys Chem Solids. 2015;85:26–33. doi:10.1016/j.jpcs.2015.04.015
- Bhavsar V, Tripathi D. Study of refractive index dispersion and optical conductivity of PPy doped PVC films. Indian J Pure Appl Phys. 2016;54:105–110.
- Nassau K. Color for science, Art and technology. New York: Elsevier; 1998.
- Benthami K, Barakat MM, Nouh SA. Modification of optical properties of PC-PBT/Cr2O3 and PC-PBT/CdS nanocomposites by gamma irradiation. Eur Phys J Appl Phys. 2020;92:20402. doi:10.1051/epjap/2020200201