ABSTRACT
The characteristics of nocturnal-to-morning rainfall (NMR) during the warm season in South China are examined using hourly surface observations from 2015–2019. Results show that strong NMR is mainly located in coastal regions and mountainous areas. NMR mainly occurs during 0200–0800 LST. The distribution of NMR can be divided into two types. The first pattern is a coastal type where NMR is mainly located at windward sites, such as southern coastal mountain areas in Guangdong (GD) and in Guangxi (GX). The second type is an inland type where NMR is mainly located at windward sites, such as the northern mountainous areas in GX and the Pearl River Delta regions in GD. The strong convergence between the northerly wind over the mountainous regions and southerly wind, as well as the strong downhill winds strengthened by the narrow pipe effect over the valleys in mountainous areas, together contribute to the high frequency of inland NMR. The strong southeasterly onshore wind and the cyclonic circulations strengthened by the northeasterly wind over the northern mountainous areas contribute to the high frequency of coastal NMR. Though the GRAPES (Global/Regional Assimilation and Prediction System) model can capture the intensity and distribution of large-scale NMR, it exhibits low predictability of small-scale NMR, especially that in the warm sector.
GRAPHICAL ABSTRACT
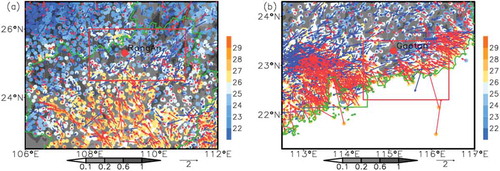
摘要
本文研究了华南暖季夜间到早晨降水 (NMR) 主要特征和可预报性。指出NMR主要发生在凌晨02–08时, 可分为两类:第一类是海岸型, 主要发生于山脉迎风坡, 如广东南部沿海山区和广西沿海山区。第二类是内陆型, 如广西北部山区和珠江三角洲中北部地区。山区北风与南侧强南风的对流辐合使得内陆型NMR频繁发生, 而东南向海风和北侧山区东北风形成的气旋性环流是造成海岸型NMR频发。尽管GRAPES模式可以预报出大尺度NMR, 但对暖区小尺度NMR预报能力较低。
1. Introduction
South China frequently encounters extreme precipitation over its coastal regions and inland mountainous areas. In recent decades, it has been shown that the rainfall in South China exhibits an evident diurnal cycle with two peaks in the early morning and in the late afternoon (Yu et al. Citation2007; Chen et al. Citation2018; Jiang et al. Citation2017; Zhong Citation2020). The rainfall in the early morning accounts for a large proportion of the whole day’s rainfall, especially in the pre-monsoon-onset period (Jiang et al. Citation2017). In particular, regulated by strong nocturnal low-level southwesterly winds in the warm sector, extreme rainfall can form over specific mountainous areas (Chen, Zhang, and Zhao, Citation2016; Zeng et al. Citation2019; Zhong et al. Citation2019a) and coastal urban areas (Du and Chen Citation2018, Citation2019; Wu et al. Citation2020) during the nocturnal-to-morning hours.
It has been pointed out that the maximum frequency of extreme precipitation events occurs in the late night and early morning in Southwest China (e.g. in the Guizhou Plateau and the Sichuan Basin (Li, Yu, and Zhou Citation2008; Zhang and Zhai Citation2011; Yu et al. Citation2014)), as well as over the Tibetan Plateau and Northwest China. Moreover, most coastal areas are found to experience a nocturnal maximum in rainfall activity (Kousky Citation1980; Du and Chen Citation2018). The land and sea breeze might induce nocturnal offshore convection produced by the convergence line between the land breeze and prevailing low-level monsoonal wind (Houze et al. Citation1981; Chen et al. Citation2015; Li, Li, and Yu Citation2019).
Investigations on nocturnal-to-morning rain (NMR) are important to gain insights into its characteristics and predictability. High-resolution simulations based on numerical weather prediction (NWP) models have been widely used to study the predictability of rainfall (Skamarock and Klemp Citation2008; Zhang, Pu, and Zhang Citation2013; Zhong et al. Citation2018, Citation2019b). However, owing to the combined effects of local topography and multi-scale weather systems between the midlatitude and tropical regions, NWP models exhibit poor performance in reflecting the rainfall diurnal variation over contiguous China (Yu et al. Citation2014; Zhong et al. Citation2019b; Zhang Citation2020). Improving the simulation performance requires reliable physical parameterizations related to rainfall processes, such as cumulus parameterization (Cai et al. Citation2018) and sub-grid orographic parameterization (Zhong et al. Citation2018), as well as improvements in the effectiveness of existing data assimilation algorithms and the development of new techniques for assimilating all available observations at high spatiotemporal resolutions (Zhong et al. Citation2019b; Zhang Citation2020).
In Zhong (Citation2020), the duration and characteristics of heavy rainfall in the warm sector were discussed using surface observations over South China. However, the occurrence time and the distribution of the NMR were not clarified, as well as the amounts of NMR compared to daytime rainfall (DR) and the corresponding predictability in NWP models. The present work complements previous studies in the following aspects: The characteristics (e.g. distribution, amount, occurrence time) of NMR are discussed, and the predictability of NMR is further examined using the Global/Regional Assimilation and Prediction System for a Tropical Mesoscale Model (GRAPES_TMM). The data and methods are presented in section 2. The characteristics of NMR in terms of surface observations and its corresponding predictability are discussed in section 3. The paper concludes with some discussion in section 4.
2. Data and model configuration
In the present work, hourly surface observations from more than 13,000 stations over South China during the warm season from March to September in 2015–19 are used to discuss the characteristics of NMR. These archived data have been widely used for operational forecasting at the South China Regional Meteorological Center (Lin, Feng, and Huang Citation2006), and in studies on diurnal variations over South China (Zhong et al. Citation2019a; Zhong Citation2020). In terms of the definition of NMR, it is rainfall that takes place during the time period from 2000 LST to the next morning at 0800 LST.
The model used in this study is an operational NWP model over South China based on GRAPES_TMM (Zhong et al. Citation2018). The simulation domain comprises 913 × 513 grid points, with a horizontal resolution of 3 km and 65 layers in the vertical direction using the terrain-following vertical coordinate scheme. The initial conditions and lateral boundary fields update every 6 h from the 0.1° × 0.1° forecast fields of the European Centre for Medium-Range Weather Forecasts. The physical schemes adopted are the same configuration as in Zhong et al. (Citation2019b). Two cases of nocturnal-to-morning torrential rain (NMTR), each representing the most common types of rainfall caused by large-scale circulation and mesoscale systems, are selected to study the predictability of the GRAPES_TMM.
3. Results
To reveal the distributional characteristics of NMR, presents the distribution of cumulative NMR and DR during the warm season over South China. It can be seen that strong NMR is mainly located in coastal regions of South and Southeast Guangdong (GD) and South Guangxi (GX), as well as mountainous areas of Northwest and North GX and the Pearl River Delta (PRD) region. For strong DR, this is mainly located over GD and Hainan Province (HN). The magnitude of both the strong NMR and DR can reach more than 5000 mm over these areas. It can also be seen that both NMR and DR exhibit around four rainfall centers, which can be divided into two types. The first type is the coastal rainfall center at windward sites over the mountainous areas, such as the Yunkai Mountains (YK), Lianhua Mountains (LH) in GD, and Shiwandashan Mountains (SWDS) in GX, which are characterized by high amounts of NMR and DR. The second type is the inland rainfall center at windward sites over mountainous areas such as the Jiuwandashan Mountains (JWDS) in GX and the PRD region in GD, which are characterized by high amounts of NMR.
Figure 1. Distribution of (a) cumulative NMR, (b) cumulative DR (dots; units: mm), and (c) the terrain height over South China (units: km)

shows the distribution of the average occurrence time of NMR and NMTR during the warm season. It can be seen that NMR mainly occurs after midnight. It is interesting to note that the average time over coastal areas in GD and HN is about 1 h later than over inland areas in the north of GD and Jiangxi Province (JX) and Fujian Province (FJ). The occurrence time in coastal areas is mainly between 0300 and 0400 LST. However, the NMR over inland areas mainly occurs 1 h earlier than that in the coastal areas. The NMTR during 0400–0600 LST mainly takes place in the four centers of NMR (as shown in )), which means the torrential NMR is characterized by sudden, short-term, and severe precipitation over these regions (e.g. NMTR occurred over coastal areas of South China in the early morning of 11 May 2014 (see Du and Chen Citation2018) and an extreme local rainstorm occurred over the PRD region on 7 May 2017 (see Xiao et al. (Citation2019)).
To further examine the detailed evolutions of the rainfall, presents the distributional frequency of NMR over South China. It can be seen that for the first half of the night (2000–0000 LST), NMR events are mainly located over central-eastern GD and FJ, and it can be inferred that the NMR during this period is caused by the eastward evolution of rain belts occurring in the late afternoon (see Yu et al. Citation2007; Jiang et al. Citation2017; Chen et al. Citation2018; Zhong Citation2020). Whereas, for the second half of the night to the next morning (0200–0800 LST), the NMR mainly occurs in the northern mountainous areas of GX, and southern coastal regions of GX and GD. Therefore, the difference between the two phases of NMR over the above regions leads to the distributional difference in the NMR occurrence time between the northern inland and coastal regions over GD and HN in ).
Figure 3. Distribution of the frequency of NMR from 2000 LST to 0800 LST at 2-h intervals. The rectangle in (e), (f), and (g) denotes the NMTR areas over the mountainous regions in the north of GX

Previous studies have shown that high-frequency occurrences of torrential rain in the afternoon are mainly attributable to the regulation of convergence between the enhanced southerly winds and northerly winds over mountainous regions (Chen et al. Citation2018; Jiang et al. Citation2017; Luo et al. Citation2017; Zhong Citation2020), as well as the effects of orographic lift (Lin, Feng, and Huang Citation2006; Zhong et al. Citation2019a) and surface heating (Wu et al. Citation2020; Zhong Citation2020). To examine the characteristics and environment of NMR, presents the average surface wind differences between −3 h (3 h before the onset hour of heavy rainfall) and the occurrence time of NMR over the north of GX and southeast coast of GD. It can be clearly seen that the strong NMTR regions over the north of GX (e.g. Rongan) exhibit strong convergence between the northerly winds over the mountainous regions and southerly winds. On the other hand, the strong NMTR regions over the southeast coast of GD show stronger southeasterly sea breezes (Chen et al. Citation2015; Li, Li, and Yu Citation2019) from the South China Sea and relative weaker northeasterly winds from northern inland areas, which lead to significant cyclonic wind circulations over mountainous regions (e.g. Gaotan). The stronger downhill winds might further be strengthened by the narrow pipe effect over the valleys in mountainous areas. Also, strong warm southerly winds can be clearly seen over the south of Rongan and Gaotan, together contributing to the high frequency of NMTR over these regions.
Figure 4. Distribution of average surface winds at −3 h (3 h before the occurrence time of heavy rainfall, where the red vectors denote southerly-wind differences and the blue vectors denote northerly-wind differences) and the average surface temperature at −3 h (dots; units: °C) over the (a) north of GX and (b) southeast coast of GD. The red rectangle denotes the key NMTR region over the north of GX and southeast coast of GD

To examine the predictability of NMR, compares observations and simulations of two NMTR cases, which represent two different types of strong NMTR caused by large-scale circulation on 9 July 2019 (case 1) and mesoscale systems on 7 May 2017 (case 2) (Wu et al. Citation2018). It can be seen that, for case 1, the GRAPES model can successfully capture the intensity and distribution of the NMTR belts. The model is able to simulate the convergence between the southerly and northerly winds over the north and northwest of GX (not shown). However, for case 2, though GRAPES captures the large-scale rain belt over GX, it misses the extreme precipitation over the PRD region in GD. Furthermore, the model underestimates the surface temperature in the warm sector, with some of the biases reaching more than 3°C (see Zhong et al. Citation2019b, Citation2019c; Xiao et al. Citation2019). In general, the model can provide reliable forecasts for large-scale NMTR over South China. However, at smaller scales, the model exhibits low predictability for the forecasting of NMTR.
4. Conclusions and discussion
This paper has examined the characteristics and predictability of NMR during the warm season over the complex regions of South China. Results show that strong NMR is mainly located in coastal regions and mountainous areas over the northwest and north of GX and the PRD region. NMR mainly occurs during 0200–0800 LST, which can be divided into two types. The first is a coastal type where the coastal NMR center is mainly located at windward sites over mountainous areas, such as YK and LH in GD, and SWDS in GX. The second type is an inland type where the inland NMR center is mainly located at windward sites over mountainous areas such as JWDS in GX and the PRD region in GD. The strong convergence between the northerly winds over mountainous regions and southerly winds, as well as the strong downhill winds strengthened by the narrow pipe effect over the valleys in mountainous areas, contributes to the high frequency of inland NMTR.
The GRAPES model exhibits some limitations in forecasting NMTR. For large-scale NMTR, it successfully captures the intensity and distribution of NMTR belts as well as the convergence between the southerly and northerly winds over the north and northwest of GX. However, the model fails to simulate small-scale NMTR, especially in the warm sector. It is speculated that the strongly underestimated surface temperature in the warm sector might lead to this low capability in NMTR simulation (see Zhong et al. Citation2019a, Citation2019b), as well as the poor representation of sub-grid processes, such as orographic (Zhong et al. Citation2018; Du and Chen Citation2019) and boundary layer (Chen, Zhang, and Zhao Citation2016) processes.
Further studies should be conducted to investigate in detail the characteristics of NMR using observations at high spatiotemporal resolutions. The mechanisms controlling NMR events need to be understood to clarify the influences of multiple factors such as the specific orographic effects and the effects of micro–mesoscale convective systems. In addition, in view of the poor predictability of NMTR events, more detailed evaluations should be carried out to examine the effects of the model physical schemes and data assimilation methods. To this end, it is necessary to improve the corresponding physical processes and data assimilation techniques to gain better model initial conditions and effective physical representations by the parameterization schemes.
Disclosure statement
No potential conflict of interest was reported by the authors.
Additional information
Funding
References
- Cai, Y., X. Lu, G. Chen, and S. Yang. 2018. “Diurnal Cycles of Mei-yu Rainfall Simulated over Eastern China: Sensitivity to Cumulus Convective Parameterization.” Atmospheric Research 213: 236–251. doi:10.1016/j.atmosres.2018.06.003.
- Chen, G., R. Lan, W. Zeng, H. Pan, and W. Li. 2018. “Diurnal Variations of Rainfall in Surface and Satellite Observations at the Monsoon Coast (South China).” Journal of Climate 31: 1703–1724. doi:10.1175/JCLI-D-17-0373.1.
- Chen, X., F. Zhang, and K. Zhao. 2016. “Diurnal Variations of the Land–Sea Breeze and Its Related Precipitation over South China.” Journal of the Atmospheric Sciences 73: 4793–4815. doi:10.1175/JAS-D-16-0106.1.
- Chen, X., K. Zhao, M. Xue, B. Zhou, X. Huang, and W. Xu. 2015. “Radar‐observed Diurnal Cycle and Propagation of Convection over the Pearl River Delta during Mei‐Yu Season.” Journal of Geophysical Research-Atmospheres 120 (24): 12557–12575. doi:10.1002/2015JD023872.
- Du, Y., and G. Chen. 2018. “Heavy Rainfall Associated with Double Low-Level Jets over Southern China. Part I: Ensemble-Based Analysis.” Monthly Weather Review 146: 3827–3844. doi:10.1175/MWR-D-18-0101.1.
- Du, Y., and G. Chen. 2019. “Heavy Rainfall Associated with Double Low-Level Jets over Southern China. Part II: Convection Initiation.” Monthly Weather Review 147: 543–565. doi:10.1175/MWR-D-18-0102.1.
- Houze, R. A., S. G. Geotis, F. D. Marks, and A. K. West. 1981. “Winter Monsoon Convection in the Vicinity of North Borneo. Part I: Structure and Time Variation of the Clouds and Precipitation.” Monthly Weather Review 109: 1595–1614. doi:10.1175/1520-0493(1981)109<1595:WMCITV>2.0.CO;2.
- Jiang, Z., D. L. Zhang, R. Xia, and T. Qian. 2017. “Diurnal Variations of Presummer Rainfall over Southern China.” Journal of Climate 30 (2): 755–773. doi:10.1175/JCLI-D-15-0666.1.
- Kousky, V. E. 1980. “Diurnal Rainfall Variation in Northeast Brazil.” Monthly Weather Review 108: 488–498. doi:10.1175/1520-0493(1980)108<0488:DRVINB>2.0.CO;2.
- Li, J., N. Li, and R. Yu. 2019. “Regional Differences in Hourly Precipitation Characteristics along the Western Coast of South China.” Journal of Climate Meteorology 58: 2717–2732. doi:10.1175/JAMC-D-19-0150.1.
- Li, J., R. Yu, and T. Zhou. 2008. “Seasonal Variation of the Diurnal Cycle of Rainfall in Southern Contiguous China.” Journal of Climate 21: 6036–6043. doi:10.1175/2008JCLI2188.1.
- Lin, L. X., Y. R. Feng, and Z. Huang. 2006. Guangdong Weather Forecast Technical Manual (In Chinese). Beijing: Chinese Meteorological Press.
- Luo, Y., R. Zhang, Q. Wan, B. Wang, W. K. Wong, Z. Hu, and Y. Zhu. 2017. “The Southern China Monsoon Rainfall Experiment (SCMREX).” Bulletin of the American Meteorological Society 98 (5): 999–1013. doi:10.1175/BAMS-D-15-00235.1.
- Skamarock, W. C., and J. B. Klemp. 2008. “A Time-split Nonhydrostatic Atmospheric Model for Weather Research and Forecasting Applications.” Journal of Computational Physics 227 (7): 3465–3485. doi:10.1016/j.jcp.2007.01.037.
- Wu, N., X. Ding, Z. Wen, G. Chen, Z. Meng, L. Lin, and J. Min. 2020. “Contrasting Frontal and Warm-sector Heavy Rainfalls over South China during the Early-summer Rainy Season.” Atmospheric Research 235: 104693. doi:10.1016/j.atmosres.2019.104693.
- Wu, Z. F., J. J. Cai, L. X. Lin, S. Hu, H. L. Zhang, and K. H. Wei. 2018. “Analysis of Mesoscale Systems and Predictability of the Torrential Rain Process in Guangzhou on 7 May 2017.” Meteorological Monthly (In Chinese) 44 (4): 485–499. doi:10.7519/j.issn.1000-0526.2018.04.002.
- Xiao, H., Q. L. Wan, X. T. Liu, T. F. Zheng, L. Feng, F. Xia, and J. H. Chen. 2019. “Numerical Prediction of an Extreme Rainstorm over the Pearl River Delta Region on 7 May 2017 Based on WRF-EnKF.” Journal of Tropical Meteorology 25 (3): 312–323.
- Yu, R., J. Li, H. Chen, and W. Yuan. 2014. “Progress in Studies of the Precipitation Diurnal Variation over Contiguous China.” Journal of Meteorological Research 28 (5): 877–902. doi:10.1007/s13351-014-3272-7.
- Yu, R., T. Zhou, A. Xiong, Y. Zhu, and J. Li. 2007. “Diurnal Variations of Summer Precipitation over Contiguous China.” Geophysics Research Letter 34: 1–4. doi:10.1029/2006GL028129.
- Zeng, W., G. Chen, Y. Du, and Z. Wen. 2019. “Diurnal Variations of Low-Level Winds and Precipitation Response to Large-Scale Circulations during a Heavy Rainfall Event.” Monthly Weather Review 147: 3981–4004. doi:10.1175/MWR-D-19-0131.1.
- Zhang, D. L. 2020. “Rapid Urbanization and More Extreme Rainfall Events.” Science Bulletin 65: 516–518. doi:10.1016/j.scib.2020.02.002.
- Zhang, H., Z. Pu, and X. Zhang. 2013. “Examination of Errors in Near-surface Temperature and Wind from WRF Numerical Simulations in Regions of Complex Terrain.” Weather and Forecasting 28 (3): 893–914. doi:10.1175/WAF-D-12-00109.1.
- Zhang, H., and P. Zhai. 2011. “Temporal and Spatial Characteristics of Extreme Hourly Precipitation over Eastern China in the Warm Season.” Advances in Atmospheric Sciences 28 (5): 1177–1183. doi:10.1007/s00376-011-0020-0.
- Zhong, S. X. 2020. “Diurnal Variation of the Duration and Environment for Heavy Rainfall during the Warm Season in South China.” Atmospheric Science Letters 1–6. doi:10.1002/asl.969.
- Zhong, S. X., Z. T. Chen, D. S. Xu, and Y. X. Zhang. 2018. “Evaluating and Improving Wind Forecasts over South China: The Role of Orographic Parameterization in the GRAPES Model.” Advances in Atmospheric Sciences 35: 713–722. doi:10.1007/s00376-017-7157-4.
- Zhong, S. X., X. Y. Li, S. Yang, and Z. T. Chen. 2019a. “Characteristics and Synoptic Environment of Torrential Rain in the Warm Sector over South China: A Composite Study.” Meteorology and Atmospheric Physics 131 (5): 1191–1203. doi:10.1007/s00703-018-0629-y.
- Zhong, S. X., S. Yang, and Z. T. Chen. 2019c. “Evaluation of the Parameterization Schemes and Nudging Techniques in GRAPES for Warm Sector Torrential Rains Using Surface Observations.” Journal of Tropical Meteorology 25 (3): 353–364. doi:10.16555/j.1006-8775.2019.03.007.
- Zhong, S. X., S. Yang, C. Y. Guo, and Z. T. Chen. 2019b. “Capabilities and Limitations of Grapes Simulations of Extreme Precipitation in the Warm Sector over a Complex Orography.” Journal of Tropical Meteorology 25: 180–191. doi:10.16555/j.1006-8775.2019.02.005.