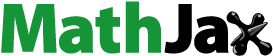
Abstract
The present study aimed to evaluate analytical performances of radial immunodiffusion (RID) technique for the quantification of milk lactoferrin (LF), and to investigate the main sources of variation of LF concentration in individual milk samples of Holstein Friesian (HF, n = 1516) and Simmental (SI, n = 230) cows. Repeatability (RSDr) and reproducibility (RSDR) of RID method were assessed as relative standard deviation of 15 measurements within the same day and 45 measurements across 3 d, respectively. Sources of variation of milk LF were investigated through a mixed linear model which included the fixed effects of classes of stage of lactation and parity (and their interaction), cow breed, udder health status (UHS, based on somatic cell count and differential somatic cell count), and the random effects of the herd-test day nested within breed and the residual. Method performances were satisfactory in terms of repeatability (RSDr < 9%) and reproducibility (RSDR < 8%), suggesting that the RID can be considered as a consistent cow-side test for the quantification of milk LF. Milk LF was positively associated with somatic cell score (r = 0.40) and negatively with lactose content (r = −0.33). Lower concentration of LF was observed in milk of ‘healthy’ and ‘susceptible’ cows (13.03 and 12.87 mg/dL, respectively) compared to ‘mastitic’ and ‘chronic’ cows (17.91 and 17.33 mg/dL, respectively). In this light, milk LF has the potential to be a useful biomarker to detect early mastitis. Further research is advisable to deepen the association between milk LF content and observed clinical mastitis.
Radial immunodiffusion method for milk lactoferrin quantification is repeatable and reproducible.
Somatic cell count is positively associated with milk lactoferrin content.
Milk lactoferrin is less concentrated in milk of healthy and susceptible than potentially mastitic and chronic cows.
Highlights
Introduction
Lactoferrin (LF) is an iron-binding glycoprotein (molecular weight 3125.8 g/mol). It belongs to the transferrin protein family and consists of a single protein chain composed of 703 amino acids folded into two globular lobes (González-Chávez et al. Citation2009). Lactoferrin is produced by mammalian mucosal epithelial cells and is present in different biological secretions including tears, mucus and saliva, and in colostrum and milk at particularly high concentrations (Franco et al. Citation2018). Lactoferrin is a multifunctional protein, with in vivo antibacterial, anti-inflammatory, osteogenic and antioxidant activities, and in vitro anti-tumor properties (Öztafl and Özgünefl Citation2005; Iglesias-Figueroa et al. Citation2019). Particularly, the antibacterial activity of LF is associated with two different mechanisms. Firstly, LF has the ability to bind iron, an essential element in the replication of microorganisms, thereby indirectly acting as a bacteriostatic agent (Giansanti et al. Citation2016; Niaz et al. Citation2019). Secondly, LF is able to promote the generation of peroxides, which in turn affect bacterial membrane permeability, acting directly towards bacterial cell lysis (Braun and Braun Citation2002). Thanks to its iron scavenger properties, LF reduces oxidative stress and excessive inflammatory response (Siqueiros-Cendón et al. Citation2014). Given the wide array of biological functions and potential health benefits, the addition of LF in functional foods (i.e. infant formula) and dietary supplements has become quite common (Wisgrill et al. Citation2018).
Milk LF concentration is affected by several factors, including stage of lactation (the concentration increases along lactation; Cheng et al. Citation2008), milk yield (the concentration decreases in milk from high-producing cows; Cheng et al. Citation2008) and milk somatic cell count (SCC; the concentration increases in milk with greater SCC; Cheng et al. Citation2008). The increase of LF concentration in milk as a physiological reaction to udder infection is mainly determined by two factors. On one hand, milk from an infected udder is richer in immune neutrophils, which indeed are able to synthesise LF (Lindmark-Månsson et al. Citation2000). On the other hand, in pathological conditions, the mammary gland tissue increases LF output (Harmon and Newbould Citation1980). Based on this evidence, milk LF has been suggested as a complementary indicator to SCC in the diagnosis of mastitis in dairy cattle (Cheng et al. Citation2008).
To date, analytical methods for milk LF quantification include electrophoresis (Mao et al. Citation2017), ion-exchange extraction coupled with high pressure liquid chromatography (Pochet et al. Citation2018), enzyme-linked immunosorbent assay (Ostertag et al. Citation2022) and radial immunodiffusion (RID; Croguennec et al. Citation2012). The RID is a fast, easy and specific technique, based on the radial diffusion of an antigenic target compound into a homogeneous gel containing the relative specific antibody. The antigen-conjugate-antibody complex precipitates in a growing circle until equilibrium is reached, in a way that circle area is directly proportional to milk LF concentration.
So far, little information is available in respect to RID method validation parameters such as repeatability and reproducibility. Furthermore, it is not clear if the RID method is sensible enough to detect LF variation in respect to milk composition traits and different physiological status of lactating animals. The outcome of the present is to provide information of bovine milk LF determination, to quantify its phenotypic variation and association to udder health status using a large dataset of Italian Holstein and Simmental milk samples. Therefore, the specific objectives are to i) assess repeatability and reproducibility of the RID technique for the quantification of LF in bovine milk and ii) investigate sources of variation of individual milk LF concentration measured through RID assay.
Materials and methods
Validation of RID assay
The validation of the RID assay was performed to assess method repeatability and reproducibility on a single raw milk (RM) sample. Repeatability and reproducibility were evaluated on the same RM sample with Bronopol (50:0.2 v/v) preservative (RMP) added and on the same RM sample subjected to 24 h freezing (RMF). In particular, repeatability of LF concentration measurements was calculated as the relative standard deviation (RSDr) of 15 consecutive measurements in 15 aliquots of RM, 15 aliquots of RMF and 15 aliquots of RMP, within the same day. Similarly, reproducibility of LF concentration measurements was calculated as the relative standard deviation (RSDR) of 45 measurements in 45 aliquots of RM, 45 aliquots of RMF and 45 aliquots of RMP, obtained across three days of analyses (Biswas et al. Citation2011; Niero et al. Citation2018).
To evaluate the similarity between the LF concentration measured in RM, and that measured in RMF (or RMP), the z-scores (z) were calculated as pairwise comparison between distributions of 45 observations each, according to the following formula:
where m is the average LF concentration in RM, and VALREF and SD are the median and the standard deviation of LF concentration in RMF (or RMP), respectively. The LF concentration in RMF (or RMP) is considered equal to the LF concentration in RM when |z| ≤ 2, similar when 2 < |z| ≤ 3 and different when |z| > 3 (Thompson et al. Citation2006).
Experimental design
Ethical approval was not required for the present study as cows belonged to commercial herds and milk was collected during official monthly test-day milk recording schemes by authorised personnel of the Breeders Association of Veneto Region (ARAV, Vicenza, Italy). A total of 2313 individual milk samples of Holstein Friesian (HF; 1966 cows, 29 herds) and Simmental cows (SI; 347 cows, 11 herds) were collected between October and December 2020. All herds involved in the current research were single breed and all animals were sampled once. For each cow, two milk aliquots of 50 mL were collected and 200 µL of preservative (Bronopol; 2-bromo-2-nitropropan-1,3-diol) was added. The two aliquots of the same samples were intended for subsequent analyses of milk chemical composition and LF concentration.
Milk chemical analyses
Gross chemical composition
One of the milk aliquots was transported to the ARAV laboratory at 4 °C for chemical analyses. Here, milk samples were warmed, gently mixed by inversion to promote solid homogenisation and analysed for fat (%), protein (%), casein (%), lactose (%) and urea (mg/dL) within 24 h using MilkoScan FT6000 (Foss, Hillerød, Denmark). The Fossomatic 7 DC (Foss, Hillerød, Denmark) was used to measure SCC (cells/µL) and differential somatic cell count (DSCC, %).
Analysis of milk lactoferrin through RID assay
The other milk aliquot collected during the milk recording scheme was transferred to the laboratory of the Department of Agronomy, Food, Natural resources, Animals and Environment of the University of Padova (Legnaro, Italy) and kept refrigerated at 4 °C until LF quantification. Lactoferrin concentration was assessed within 24 h, using 24 wells RID kit plates filled with a jelly medium containing a specific LF antibody (the name of the supplier is not mentioned to avoid any possible conflict of interest). Three out of the 24 wells were filled by pipetting 5 µl of LF standard solutions (i.e. 3, 16 and 32 mg/dL) included in the RID kit. The remaining 21 wells were filled by pipetting 5 µl of milk, previously warmed at room temperature and repeatedly inverted to promote solid homogenisation. Thereafter, each filled plate was incubated at room temperature for 24 h. During incubation LF creates a complex with the LF antibody and precipitates in a circle around the well, in a way that the area of the circle is directly proportional to the LF concentration. At the end of the reaction, RID plates were scanned and high-resolution images were made available for imaging analyses. ImageJ software was used to measure the area of each circle (ImageJ Citation2022). For each plate, calibration curves were calculated as the regression between the concentration of LF in the standard solutions and the corresponding circle area (R2 ≥ 0.95). For each milk sample, LF concentration was determined by comparing with standard calibration curves.
Editing and statistical analyses
Days in milk (DIM) were restricted to be between 6 and 600 days, and parity between 1 and 10. Herds with less than 10 test day records were removed from the dataset. Values of LF below the minimum concentration of the standard solution (i.e. 3 mg/dL) and above the maximum concentration of the standard solution (i.e. 32 mg/dL) were removed. Lactoferrin concentrations calculated from plates with calibration curves having a coefficient of determination (R2) smaller than 0.95 were discarded. Outliers of LF concentration were defined as values deviating more than 3 standard deviations (SD) from the respective mean and were removed from the original dataset. Moreover, samples exceeding 3 standard deviations from the respective mean of milk yield, fat, protein, casein, and lactose percentages, urea concentration, SCS and DSCC were considered as outliers and set as missing values. The final dataset included 1746 test day records from 1516 HF (24 herds) and 230 SI cows (10 herds).
Pearson correlations (r) of milk LF concentration with milk yield and quality traits were assessed through the CORR procedure of the SAS software (SAS Institute Inc., Cary, NC, USA). Sources of variation of LF, milk yield and quality traits were investigated using the GLIMMIX procedure of SAS according to the following linear model:
where yijklm is the dependent variable (LF content, milk yield or milk quality traits); µ is the overall intercept of the model; stagei is the fixed effect of the ith class of lactation stage of the cow (i = 1 to 8, the first being a class from 6 to 45 DIM, followed by 6 classes of 45 DIM each, and the last being a class of DIM > 315); parityj is the fixed effect of the jth parity class of the cow (j = 1 to 5, with the last class including parities 5 to 10); breedk is the fixed effect of the kth cow breed (k = HF, SI); (stage*parity)ij is the fixed interaction effect between class of lactation stage and parity class; UHSl is the fixed effect of the lth udder health status (l = healthy when SCC ≤ 200 cells/µL and DSCC ≤ 66.3%, susceptible when SCC ≤ 200 cells/µL and DSCC > 66.3%, mastitic when SCC > 200 cells/µL and DSCC > 66.3%, chronic when SCC > 200 cells/µL and DSCC ≤ 66.3%) according to the thresholds defined by Zecconi et al. (Citation2019); HTDm(breedk) is the random effect of the mth herd-test date nested within the kth breed (n = 1 to 34) ∼N(0, σ2HTD(Breed)), where σ2HTD(Breed) is the HTD nested within breed variance; and eijklm is the random residual ∼ N(0, σ2e), where σ2e is the residual variance. Differences between least squares means of the fixed effects were tested using Bonferroni’s post hoc multiple comparison test (p < .05).
Results and discussion
Repeatability and reproducibility of the RID assay
Table reports repeatability RSDr calculated within each day of analysis on 15 measurements performed on 15 aliquots of RM, RMF and RMP samples. The best repeatability performances were registered for RM, which indeed showed the lowest within-day RSDr ranging from 1.04% (Day 3) to 7.08% (Day 2). Lower repeatability was obtained for RMF and RMP which showed RSDr ranging from 6.64% to 8.85% and 5.39% to 8.35%, respectively. Reproducibility RSDR, calculated across three days of analyses on 45 measurements in 45 milk aliquots, varied from 6.06% to 7.73% for RM and RMF, respectively (Table ). Compared to RM, the across-days reproducibility worsened in the case of RMF and RMP, confirming the trend of the within-day repeatability. In the case of RMF, the greater RSDr and RSDR may be the result of protein degradation occurring during sample freezing and melting (Vigolo et al. Citation2022), which may ultimately compromise the formation of antigen-conjugate-antibody complexes. This is also reflected in the average concentration of LF in RMF (from 12.26 to 11.78 mg/dL in Day 1 and Day 2, respectively) which is numerically lower compared to the average concentration of LF in RM (from 13.22 to 12.92 mg/dL in Day 1 and Day 2, respectively). Similar considerations can be done for milk samples to which Bronopol has been added (i.e. RMP), which indeed exhibited greater RSDr and RSDR together with numerically lower LF concentrations compared with RM. Findings of the present study agree with those reported by Vigolo et al. (Citation2022) who observed detrimental effect of Bronopol on the concentration of total whey protein and on β-lactoglobulin and α-lactalbumin fractions. Indeed, Bronopol activity against bacteria is due to its ability to release formaldehyde, which reacts with the amino groups of milk proteins, creating formal-protein complexes (Upadhyay et al. Citation2014).
Table 1. Repeatability relative standard deviation (RSDr, %; n = 15), reproducibility relative standard deviation (RSDR, %; n = 45) and z-scores (z) for lactoferrin concentration (mg/dL) in raw milk (RM), raw frozen milk (RFM) and raw milk with preservative added (RPM).
Overall, RSDr and RSDR of the present study were below 9% and 8%, respectively. Such indicators of repeatability and reproducibility are less satisfactory compared to the performances of other laboratory methods based on time-demanding analytical procedures or which rely on costly laboratory equipment such as high-pressure liquid chromatography (Niero et al. Citation2018) or inductively coupled plasma mass spectrometry (Niero et al. Citation2019). Still, given the possibility to apply RID technique for the quantification of milk LF in field conditions and at relatively low cost (about 5€/sample), such performances may be considered reasonable.
Z-scores were calculated as pairwise comparison between distributions of 45 observations each to assess whether LF concentration measured in RMF and RMP agreed with LF concentration measured in RM. Results from 2 experimental conditions can be considered comparable for |z| ≤ 2, similar when 2 < |z| ≤ 3, and different when |z| > 3 (Thompson et al. Citation2006). The comparison between RM and RMF resulted in a z-score of 1.14. An even lower z-score (1.08) was obtained for the comparison between RM and RMP (Table ). In the light of these results, and from a practical view point, we can assume that freezing processes and the addition of preservatives affect the quantification of LF through RID assay by a negligible amount.
Descriptive statistics and phenotypic correlations
Descriptive statistics for i) LF quantified in individual milk samples through RID assay, ii) individual milk yield and iii) individual milk quality traits predicted through mid-infrared spectroscopy in HF and SI cows are summarised in Table . Milk LF averaged 13.06 mg/dL and 13.64 mg/dL in HF and SI cows, respectively. The great coefficient of variation of milk LF for both HF cows (55.01%) and SI cows (51.74%) suggests that exploitable phenotypic variation exists for this trait. Mean values of LF concentration in the present study were slightly lower than the value (16.91 mg/dL) reported by Hagiwara et al. (Citation2003), who measured LF through RID technique in quarter milk obtained from healthy cows and from cows with subclinical mastitis. In particular, average LF concentration varied from 0.7 mg/dL to 115 mg/dL on quarter milk samples obtained from healthy cows and from 0.7 mg/dL to 360 mg/dL on quarter milk samples obtained from animals with subclinical mastitis (Hagiwara et al. Citation2003). Conversely, average LF concentration observed in the present study was slightly greater compared to the value (11.45 mg/dL) reported by Cheng et al. (Citation2008), who quantified milk LF on a smaller sample size (198 HF cows) through sandwich ELISA assay. In the present study, SCC averaged 179.98 cells/µL and 175.21 cells/µL for HF and SI cows, respectively, and DSCC averaged 59.29% and 59.01%. Such values are below the thresholds of 200 cells/µL for SCC (Dohoo and Leslie Citation1991) and 66.30% for DSCC (Zecconi et al. Citation2019), which are commonly used to classify cow’s udder health status.
Table 2. Descriptive statistics of milk lactoferrin concentration, production-related traits and milk quality traits in Holstein Friesian and Simmental cows.
Pearson correlation coefficients between milk LF, milk yield and quality traits are reported in Table . All correlations significantly differed from zero (p < .001), except for the almost null association between LF and urea (r = −0.02, p > .05). The estimated correlation between LF and milk yield was weakly negative (r = −0.22), supporting the existence of a dilution effect as already reported for other milk protein fractions (Ng-Kwai-Hang et al. Citation1982; Niero et al. Citation2021) and other milk components (Visentin et al. Citation2018). The correlation between LF and protein content was positive (r = 0.28), which was expected because of LF being classified as a protein. A similar weak positive association was observed between LF and casein content (r = 0.27), probably because an increase in the casein fraction is translated into an increase of total protein content, which in turn reflects increased LF content. In the present study, the strongest phenotypic correlation was observed between LF and SCS (r = 0.40), in such a way that milk with greater SCS had greater LF concentration. A similar positive association (r = 0.375) was reported by Cheng et al. (Citation2008) who investigated factors influencing LF concentration in bovine milk. Such results confirmed the role of LF in the innate defense system of the host against pathogens related to udder infection and potential mastitis events (Valenti and Antonini Citation2005). The weakly positive correlation between LF and DSCC (r = 0.13) and the moderately negative association between LF and lactose (r = −0.33) confirm this hypothesis, since DSCC increases and lactose content decreases in presence of relatively high SCS and mastitis (Miglior et al. Citation2007; Damm et al. Citation2017).
Table 3. Pearson correlations of milk lactoferrin with milk yield and quality traits.
Sources of variation
F-values and significance of fixed effects included in the analysis of variance for LF content, milk yield and quality traits of HF and SI cows are reported in Table . Class of lactation stage (F-value = 41.03; p < .001), parity class (F-value = 10.91; p < .001) and udder health status class (F-value = 51.30; p < .001) were significant in explaining the variation of milk LF concentration. On the other hand, the breed (F-value = 0.15; p > .05) and the first order interaction between class of lactation stage and parity class (F-value = 1.41; p > .05) did not affect milk LF concentration. The random effect of HTD nested within cow breed explained 15.59% of the total variance of milk LF, being similar to the variance explained for lactose percentage (13.77%), lower than that explained for fat percentage, milk yield and urea concentration (24.42%, 38.20% and 56.77%, respectively), and greater than that accounted for protein and casein percentages (7.64% and 9.19%, respectively). The negligible effect of bovine breed in explaining LF variation was observed also in Soyeurt et al. (Citation2007), who reported significant differences only between Holstein and Jersey lactoferrin concentration predicted by mid-infrared spectrometry. This has been also confirmed in Fleming et al. (Citation2019) who investigated the phenotypic variation of LF and other fine milk constituents in 4 different Canadian breeds (Holstein, Jersey, Brown Swiss, and Ayrshire). Findings of the present study suggest that milk LF concentration is affected by environmental factors (i.e. farm management and feeding regimes) and indirectly corroborate the results of Soyeurt et al. (Citation2007) and Arnould et al. (Citation2009) who quantified the presence of additive genetic variation for milk LF, with an heritability estimate of 0.19 and 0.22, respectively.
Table 4. F-values and significance of fixed effects, and variance accounted by herd test-day nested within breed effect (σ2HTD(Breed), %) for lactoferrin concentration, milk yield and quality traits.
Effects of lactation stage, parity and udder health status
Least squares means of milk LF concentration across classes of lactation stages are depicted in Figure . Milk LF steadily increased across lactation (p < .001), varying from 10.92 mg/dL (between 6 and 45 DIM) to 19.30 mg/dL (for DIM > 315). Besides being significant from a statistical point of view, such a variation has physiological and biological meanings. The first relies on the well-known ‘dilution effect’, which translates into an increased LF concentration due to decreased productivity of the animals towards later lactation stages (Hagiwara et al. Citation2003; Cheng et al. Citation2008). In other terms, milk LF concentration increases due to a diminished milk production rather than to an augmented synthesis of this protein. A similar pattern of LF concentration within lactation was reported also in Leclercq et al. (Citation2013), with a nadir around the lactation peak. This also corroborates the negative association between milk LF and milk yield (r = −0.22; p < .001; Table ). The second reason reflects the increase of SCS which may occur in later lactation stages (Jones and Bailey, Citation2009). This translates into augmented LF synthesis boosted by the cells of the immune system of the host as a reaction against udder infection (Harmon and Newbould Citation1980; Lindmark-Månsson et al. Citation2000). This is in agreement with the positive correlation between milk LF and SCS (r = 0.40; p < .001; Table ) and DSCC (r = 0.13; p < .001; Table ).
Figure 1. Least squares means (with standard error) of milk lactoferrin concentration across classes of lactation stage (A) and parity classes (B). Means with different letters differ significantly (p <.05).

Milk LF concentration increased with parity order, exhibiting the lowest value in primiparous cows (13.61 mg/dL) and the greatest value in cows of parity ≥ 5 (16.28 mg/dL). This trend is somewhat opposite compared to that of caseins fractions and α-lactalbumin (Franzoi et al. Citation2019), and once again may reflect the protective effect of LF towards mastitis events which are more frequent in older cows (Costa et al. Citation2020). The increase of LF concentration across parity order corroborates with Fleming et al. (Citation2019), who reported that LF in milk produced by cows at 5th (and more) lactation was more than 20% greater compared to milk from first-lactating animals. A similar effect, and pattern, across lactations was reported also in Nudda et al. (Citation2003) investigating the variation of LF in ovine milk. However, investigations in other species suggested, for example, that in swine LF is greater in gilts compared to sows (Jahan et al., Citation2020).
Figure reports least squares means of milk LF concentration for different classes of udder health status (i.e. healthy, susceptible, mastitic and chronic), defined according to the SCC and DSCC thresholds proposed by Zecconi et al. (Citation2019). Milk LF concentration was significantly lower in the case of healthy (13.03 mg/dL) and susceptible animals (12.87 mg/dL) having SCC ≤ 200 cells/µL compared to mastitic and chronic animals (17.91 mg/dL and 17.33 mg/dL, respectively) having SCC ≥ 200 cells/µL. Similar results were reported by Kawai et al. (Citation1999), who studied the association between milk LF and clinical mastitis, and more recently by Raj et al. (Citation2021) who investigated variations in the levels of acute-phase proteins and LF in bovine milk during subclinical mastitis. In line with the results of the present study, Huang et al. (Citation2012) observed increased secretion of LF from the mammary epithelial cells during acute clinical mastitis. Galfi et al. (Citation2016) reported that milk LF concentration quickly increased in cows affected by subclinical and clinical mastitis. Findings of the present study suggest that milk LF concentration is mostly affected by the amount of SCC rather than by the percentage of DSCC. Indeed, the phenotypic correlation calculated between milk LF and SCC (r = 0.40; p < .001) was considerably greater than that calculated between milk LF and DSCC (r = 0.13; p < .001). Based on the results of the present study, the RID method represents a relatively low-cost method helpful in field conditions to discriminate milk with low and high SCC and possibly for the early detection of mastitis.
Figure 2. Distribution of the data and least squares means of milk lactoferrin concentration (mg/dL) according to udder health status classification proposed by Zecconi et al. (Citation2019): healthy (SCC ≤ 200 cells/µL and DSCC ≤ 66.3%), susceptible (SCC ≤ 200 cells/µL and DSCC ≥ 66.3%), mastitic (SCC ≥ 200 cells/µL and DSCC ≥ 66.3%) and chronic (SCC ≥ 200 cells/µL and DSCC ≤ 66.3%). Means with different letters differ significantly (p <.05).

Conclusions
Results of the present study indicate that RID method adopted for milk LF quantification has fairly satisfactory performances in terms of repeatability (RSDr < 9%) and reproducibility (RSDR < 8%). In the light of this, RID technique may be used to determine milk LF in field conditions and at relatively marginal cost. Among studied traits, SCC had the strongest Pearson correlation with milk LF (r = 0.40, p < .001). Milk LF increased significantly across lactation and parity order. Based on the thresholds of SCC and DSCC used to define UHS, we observed that LF was less concentrated in milk of healthy (13.03 mg/dL) and susceptible cows (12.87 mg/dL) than potentially mastitic (17.91 mg/dL) and chronic cows (17.33 mg/dL). Therefore, RID technique has potential to be used as a screening method to detect milk with low and high SCC and as an indicator of early detection of mastitis. Further research is needed to understand whether the RID method is enough sensible to detect possible differences in LF concentration across different bovine breeds.
Ethical approval
Procedures adopted in this study do not fall into the scope of an animal ethics evaluation, as they do not reach the thresholds established in the ‘Directive 2010/63/EU (Art. 1) of the European Parliament and of the council of 22nd September 2010 on the protection of animals used for scientific purposes’.
Acknowledgements
The authors thank the Breeders Association of Veneto Region (ARAV, Vicenza, Italy), the National Association of Holstein, Brown Swiss, and Jersey Breeds (ANAFIBJ, Cremona, Italy) and the National Association of Italian Simmental Breed (ANAPRI, Udine, Italy) for technical support.
Disclosure statement
No potential conflict of interest was reported by the author(s). The authors alone are responsible for the content and writing of this article.
Data availability statement
None of the data were deposited in an official repository. The data that support the findings presented in this study are available from the first author or corresponding author upon reasonable request.
Additional information
Funding
References
- Arnould VR, Soyeurt H, Gengler N, Colinet FG, Georges MV, Bertozzi C, Portetelle D, Renaville R. 2009. Genetic analysis of lactoferrin content in bovine milk. J Dairy Sci. 92(5):2151–2158.
- Biswas AK, Sahoo J, Chatli MK. 2011. A simple UV-Vis spectrophotometric method for determination of β-carotene content in raw carrot, sweet potato and supplemented chicken meat nuggets. LWT Food Sci Technol. 44:1809–1813.
- Braun V, Braun M. 2002. Active transport of iron and siderophore antibiotics. Curr Opin Microbiol. 5(2):194–201.
- Cheng JB, Wang JQ, Bu DP, Liu GL, Zhang CG, Wei HY, Zhou LY, Wang JZ. 2008. Factors affecting the LF concentration in bovine milk. J Dairy Sci. 91(3):970–976.
- Costa A, Bovenhuis H, Penasa M. 2020. Changes in milk lactose content as indicators for longevity and udder health in Holstein cows. J Dairy Sci. 103(12):11574–11584.
- Croguennec T, Li N, Phelebon L, Garnier-Lambrouin F, Gésan-Guiziou G. 2012. Interaction between lactoferrin and casein micelles in skimmed milk. Int Dairy J. 27:34–39.
- Damm M, Holm C, Blaabjerg M, Bro MN, Schwarz D. 2017. Differential somatic cell count - A novel method for routine mastitis screening in the frame of Dairy Herd Improvement testing programs. J Dairy Sci. 100(6):4926–4940.
- Dohoo IR, Leslie KE. 1991. Evaluation of changes in somatic cell counts as indicators of new intra-mammary infections. Prev Vet Med. 10:225–237.
- Fleming A, Schenkel FS, Ali RA, Corredig M, Carta S, Gregu CM, Malchiodi F, Macciotta NPP, Miglior F. 2019. Phenotypic investigation of fine milk components in bovine milk and their prediction using mid-infrared spectroscopy. Can J Anim Sci. 99:218–227.
- Franco I, Pérez MD, Conesa C, Calvo M, Sánchez L. 2018. Effect of technological treatments on bovine lactoferrin: n overview. Food Res Int. 106:173–182.
- Franzoi M, Niero G, Visentin G, Penasa M, Cassandro M, De Marchi M. 2019. Variation of detailed protein composition of cow milk predicted from a large database of mid-infrared spectra. Animals. 9:176.
- Galfi AL, Radinović MŽ, Boboš SF, Pajić MJ, Savić SS, Milanov DS. 2016. Lactoferrin concentrations in bovine milk during involution of the mammary glands with different bacteriological findings. Veterinaryarhiv. 86:487–497.
- Giansanti F, Panella G, Leboffe L, Antonini G. 2016. LF from milk: Nutraceutical and pharmacological properties. Pharmaceuticals. 9:61.
- González-Chávez SA, Arévalo-Gallegos S, Rascón-Cruz Q. 2009. Lactoferrin: structure, function, and applications. Int J Antimicrob Agents. 33:3011–3018.
- Hagiwara SI, Kawai K, Anri A, Nagahata H. 2003. Lactoferrin concentrations in milk from normal and subclinical mastitic cows. J Vet Med Sci. 65(3):319–323.
- Harmon RJ, Newbould FHS. 1980. Neutrophil leukocyte as a source of lactoferrin in bovine milk. Am J Vet Res. 41:1603–1606.
- Huang YQ, Morimoto K, Hosoda K, Yoshimura Y, Isobe N. 2012. Differential immunolocalization between lingual antimicrobial peptide and lactoferrin in mammary gland of dairy cows. Vet Immunol Immunopathol. 145(1-2):499–504.
- Iglesias-Figueroa BF, Espinoza-Sánchez EA, Siqueiros-Cendón TS, Rascón-Cruz Q. 2019. Lactoferrin as a nutraceutical protein from milk, an overview. Int Dairy J. 89:37–41.
- ImageJ 2022. Image processing and analysis in Java. https://imagej.nih.gov/ij/index.html.
- Jahan M, Francis N, Wang B. 2020. Milk lactoferrin concentration of primiparous and multiparous sows during lactation. J Dairy Sci. 103(8):7521–7530.
- Jones GM, Bailey TL. 2009. Understanding the basics of mastitis. Virginia Cooperative Extension. Publication pp. 404.
- Kawai K, Hagiwara S, Anri A, Nagahata H. 1999. Lactoferrin concentration in milk of bovine clinical mastitis. Vet Res Commun. 23(7):391–398.
- Leclercq G, Gengler N, Soyeurt H, Bastin C. 2013. Genetic variability of the mid-infrared prediction of lactoferrin content in milk for Walloon Holstein first-parity cows. Livest Sci. 151:158–162.
- Lindmark-Månsson H, Svensson U, Paulsson M, Aldén G, Frank B, Johnsson G. 2000. Influence of milk components, somatic cells and supplemental zinc on milk processability. Int Dairy J. 10:423–433.
- Mao K, Du H, Bai L, Zhang Y, Zhu H, Wang Y. 2017. Poly (2-methyl-2-oxazoline) coating by thermally induced immobilization for determination of bovine lactoferrin in infant formula with capillary electrophoresis. Talanta. 168:230–239.
- Miglior F, Sewalem A, Jamrozik J, Bohmanova J, Lefebvre DM, Moore RK. 2007. Genetic analysis of milk urea nitrogen and lactose and their relationships with other production traits in Canadian Holstein cattle. J Dairy Sci. 90(5):2468–2479.
- Ng-Kwai-Hang KF, Hayes JF, Moxley JE, Monardes HG. 1982. Environmental influences on protein content and composition of bovine milk. J Dairy Sci. 65(10):1993–1998.
- Niaz B, Saeed F, Ahmed A, Imran M, Maan AA, Khan MKI, Tufail T, Anjum FM, Hussain S, Suleria HAR. 2019. Lactoferrin (LF): a natural antimicrobial protein. Int J Food Prop. 22:1626–1641.
- Niero G, Franzoi M, Manuelian CL, Visentin G, Penasa M, De Marchi M. 2021. Protein profile of cow milk from multibreed herds and its relationship with milk coagulation properties. Ital J Anim Sci. 20:2232–2242.
- Niero G, Franzoi M, Vigolo V, Penasa M, Cassandro M, Boselli C, Giangolini G, De Marchi M. 2019. Validation of a gold standard method for iodine quantification in raw and processed milk, and its variation in different dairy species. J Dairy Sci. 102(6):4808–4815.
- Niero G, Penasa M, Berard J, Kreuzer M, Cassandro M, De Marchi M. 2018. Development and validation of an HPLC method for the quantification of tocopherols in different types of commercial cow milk. J Dairy Sci. 101(8):6866–6871.
- Nudda A, Feligini M, Battacone G, Macciotta NPP, Pulina G. 2003. Effects of lactation stage, parity, β-lactoglobulin genotype and milk SCC on whey protein composition in Sarda dairy ewes. Ital J Anim Sci. 2:29–39.
- Ostertag F, Sommer D, Berensmeier S, Hinrichs J. 2022. Development and validation of an enzyme-linked immunosorbent assay for the determination of bovine lactoferrin in various milk products. Int Dairy J. 125:105246.
- Öztafl YE, Özgünefl N. 2005. Lactoferrin: a multifunctional protein. Adv Mol Med. 1:149–154.
- Pochet S, Arnould C, Debournoux P, Flament J, Rolet-Répécaud O, Beuvier E. 2018. A simple micro-batch ion-exchange resin extraction method coupled with reverse-phase HPLC (MBRE-HPLC) to quantify LF in raw and heat-treated bovine milk. Food Chem. 259:36–45.
- Raj A, Kulangara V, Vareed TP, Melepat DP, Chattothayil L, Chullipparambil S. 2021. Variations in the levels of acute-phase proteins and lactoferrin in serum and milk during bovine subclinical mastitis. J Dairy Res. 88(3):321–325.
- Siqueiros-Cendón T, Arévalo-Gallegos S, Iglesias-Figueroa BF, García-Montoya IA, Salazar-Martínez J, Rascòn-Cruz Q. 2014. Immunomodulatory effects of LF. Acta Pharmacol Sin. 35(5):557–566.
- Soyeurt H, Colinet FG, Arnould M-R, Dardenne P, Bertozzi C, Renaville R, Portetelle D, Gengler N. 2007. Genetic variability of lactoferrin content estimated by mid-infrared spectrometry in bovine milk. J Dairy Sci. 90(9):4443–4450.
- Thompson M, Ellison SL, Wood R. 2006. The international harmonized protocol for the proficiency testing of analytical chemistry laboratories (IUPAC Technical Report). Pure Appl. Chem. 78:145–196.
- Upadhyay N, Goyal A, Kumar A, Ghai DL, Singh R. 2014. Preservation of milk and milk products for analytical purposes. Food Rev. Int. 30:203–224.
- Valenti P, Antonini G. 2005. Lactoferrin: An important host defense against microbial and viral attack. Cell Mol Life Sci. 62(22):2576–2587.
- Vigolo V, Niero G, Penasa M, De Marchi M. 2022. Effects of preservative, storage time, and temperature of analysis on detailed milk protein composition determined by reversed-phase high-performance liquid chromatography. J Dairy Sci. 105(10):7917–7925.
- Visentin G, Penasa M, Niero G, Cassandro M, De Marchi M. 2018. Phenotypic characterization of major mineral composition predicted by mid-infrared spectroscopy in cow milk. Ital J Anim Sci. 17:549–556.
- Wisgrill L, Wessely I, Spittler A, Förster-Waldl E, Berger A, Sadeghi K. 2018. Human lactoferrin attenuates the proinflammatory response of neonatal monocyte-derived macrophages. Clin Exp Immunol. 192(3):315–324.
- Zecconi A, Vairani D, Cipolla M, Rizzi N, Zanini L. 2019. Assessment of subclinical mastitis diagnostic accuracy by differential cell count in individual cow milk. Ital J Anim Sci. 18:460–465.