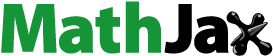
Abstract
Bioconversion of plant material with white-rot fungi has demonstrated improvements in in vitro digestibility, however, effects on rumen fermentation parameters and methane (CH4) production, when included as a dietary ingredient, are not clear. The aim of this study was to assess changes in rumen fermentation parameters and in vitro CH4 production by dietary inclusion of ryegrass-fescue hay biodegraded with P. ostreatus (H-PO) during 14 days of solid fermentation. The experiment used 2 diets with contrasting acid detergent fibre (aADFom) content which were added with 0, 3 and 6% H-PO. Substrates were incubated 3 times with sampling at 24 h. Results were analysed by ANOVA, and compared with Tukey’s test and polynomial contrasts. Inclusion of H-PO at concentrations of 3 and 6% resulted in increased in vitro dry matter (DM) disappearance (IVDMD) by 10 and 12% respectively, whereas high concentrations of aADFom reduced IVDMD by 10% as compared to low aADFom. Methane production (mg/g DM incubated) and yield (mg/g DM degraded) were reduced by increasing aADFom and P. ostreatus biodegraded hay concentrations in the diets (p < 0.001) whereas concentrations of total short-chain fatty acids were not affected by the inclusion of biodegraded hay (p = 0.080) or aADFom concentration (p = 0.076). Increasing concentrations of biodegraded hay resulted in increased acetate (p < 0.001), and reduced propionate proportions (p = 0.021) but did not affect butyrate (p = 0.233). In conclusion the use of biodegraded hay as a pre-treated ingredient improved the utilisation of low and high fibre diets by increasing the in vitro disappearance, and decreasing CH4 emissions, without increasing the propionate proportion.
The use of hay biodegraded with P. ostreatus (H-PO) improves the digestibility of ruminant diets.
The use of H-PO decreases methane, and increases acetate production in in vitro conditions.
The use of H-PO as an ingredient in ruminant diets could improve the sustainability of livestock systems.
HIGHLIGHTS
Introduction
The relationship between livestock production and greenhouse gas emissions is the subject of multiple global assessments and much public and policy commentary (Scoones Citation2022). Livestock systems face the challenge of increasing their productivity whilst reducing their greenhouse gases (GHG) emissions. As such, there is growing interest in developing local, low-cost strategies to improve feed utilisation efficiency for ruminants and reducing their contribution to GHG and climate change (Zuo et al. Citation2018). Among these strategies, biological pre-treatment of lignocellulose feeds involves improving their nutritional quality, thus reducing the accumulation or burning of residues such as stubble or straw and, as such, reducing CH4 emissions to the environment. Biological pre-treatment or bioconversion with white-rot fungi, such as Pleurotus ostreatus, has improved the nutritional value and ruminal digestibility of high lignocellulose residues such as wheat straw (Fazaeli et al. Citation2002; Shrivastava et al. Citation2012), coffee husk (Badarina et al. Citation2013), corn stover (Zuo et al. Citation2018), and can be applied in a wide range of ruminant feeds and agro-industrial by-products. An early report (Beg et al. Citation1986) concluded that the use of P. ostreatus increases digestibility of rice husk and suggests its use in combination with other ingredients to optimise nutrition, but to date only studies by Fazaeli et al. (Citation2002) and Badarina et al. (Citation2013) have evaluated complete diets including pre-treated wheat straw and coffee husk. White-rot fungi such as P. ostreatus degrade lignin by means of extracellular enzymes, such as laccase, manganese peroxidase and lignin peroxidase which break down the polysaccharide-lignin complex. This results in improved microbial access to digestible biomass and increased degradation of plant material (Shrivastava et al. Citation2012). Astudillo-Neira et al. (Citation2022) determined that unlike other white-rot fungi, the biodegradation of hay for 14 days with P. ostreatus is feasible for use in ruminant feeding. This pre-treatment generates extracellular enzymes which remain in the fungal mycelia. Thus, we hypothesised that when included in complete diets, pre-treated hay should also improve the disappearance of other feeds and reduce CH4 yield (CH4 mg/gDM digested). The objective of this study was to assess changes in rumen in vitro fermentation parameters in response to graded addition of P. ostreatus pre-treated rye grass-fescue hay in complete diets.
Materials and methods
This experiment was conducted at the Livestock Systems and Nutrition Laboratory of the Universidad de Concepción, Chillán, Chile. The care and management of the cows were certified by the animal ethics and welfare committee of the Universidad de Concepción.
Experimental design and treatments
A completely randomised experimental design was considered using a 2 × 3 factorial arrangement with two levels of aADFom in diets, and three levels of inclusion of P. ostreatus biodegraded hay (H-PO). The levels of aADFom in diets were: high (35.30%) and low (23.45%), whereas the H-PO inclusion were: 0, 3, and 6% DM. Thus, the trial included 6 treatments as follows: T1 (diet low in aADFom without H-PO), T2 (diet low in aADFom with 3% H-PO), T3 (diet low in aADFom with 6% H-PO), T4 (diet high in aADFom without H-PO), T5 (diet high in aADFom with 3% H-PO), and T6 (diet high in aADFom with 6% H-PO).
Biodegraded hay (H-PO; Table ) was obtained from solid fermentation of low quality ryegrass-fescue hay (Lolium perenme- Festuca arundinacea) during 14 days with Pleurotus ostreatus (strain 136UBB). The ryegrass-fescue hay was ground with a 2 mm sieve (Grain Mill, Breuer, Temuco, Chile), sterilised in an autoclave (Vertical type steam steriliser, model HL8-340) at a temperature of 121 °C. C, pressure of 120 W/KA, in a cycle of 15 min (Rahman et al. Citation2011) and finally dried at 50 °C for 48 h. Solid state fermentation was carried out in 48 Erlenmeyer flasks (250 mL) with 50 mL of Hagen’s medium, plant substrate (5%), and mycelium. The flasks were immediately incubated at 30 °C in the dark for 21 days, under stationary conditions (Akpinar and Urek Citation2012; Akinfemi and Ogunwole Citation2012) (Figure ). Once the colonisation of the plant substrate was completed, the total content of the flasks was ground in a mortar, samples were collected to evaluate of enzymatic activity, before including the remaining product as an ingredient of the experimental diets. The enzymatic activity of this biodegraded hay was 19.51 U/L for lignin peroxidase, 6.68 U/L for manganese peroxidase, and 34.17 U/L for laccase (Astudillo-Neira et al. Citation2022).
Table 1. Ingredients and chemical composition of experimental diets, and biodegraded hay used for in vitro incubations.
The treatments were designed to determine the effect of the inclusion of P. ostreatus biodegraded hay (H-PO) in ruminant diets with contrasting aADFom content. The incubation substrates were a mixture of forages and concentrate feeds, which were mixed in different proportions depending on whether the diet was high or low in aADFom (Table ). All ingredients were ground to 2 mm using a grain mill (Breuer, Temuco, Chile) before mixing with H-PO at concentrations of 0, 3, and 6% DM.
In vitro batch incubations
Rumen fluid was obtained from two non-lactating, cannulated, adult Aberdeen Angus cows fed twice daily a diet with a forage:concentrate ratio of 70:30 DM for two weeks prior to the experiment. Two hours after the morning feeding, rumen contents were collected and filtered through 4 layers of cheesecloth, and immediately transported to the laboratory in prewarmed (39 °C) thermal containers. Inoculum for fermentation was prepared by mixing ruminal fluid with an incubation buffer solution (Menke et al. Citation1979) in a ratio of 1:3 (v/v), respectively. Inoculum (25 mL) was transferred to amber serum bottles, which were gassed with CO2, sealed with rubber stoppers and incubated at 39 °C (Forma Series II 3110 Water-Jacketed CO2 Incubator, Thermo Fisher Scientific, Waltham, MA, USA) on an orbital shaker (Heidolph Unimax 2010, Schwabach, Germany) at 90 oscillations/minute. Serum bottles contained ANKOM(R) -F57 filter bags with 500 mg of each experimental treatment. Vials with blanks were included to estimate net gas production (Ávila et al. Citation2011). Three replicates were included for each treatment and sampling time (24 h) and the complete 24 h incubation was repeated three times in three consecutive weeks. The 24 h incubation period was used according to the gas production technique described by Menke and Steingass (Citation1988) and has been further validated by multiple authors (Getachew et al. Citation2002; Purcell et al. Citation2011; Foggi et al. Citation2022; Suescún-Ospina et al. Citation2022). The total number of bottles incubated per run was 20 (6 treatments x 3 replicates + 2 blanks).
Estimation of ruminal gas production, CH4, and dry matter disappearance
Gas production (GP) of each bottle was measured at 6, 12 and 24 h of incubation by water displacement (Fedorah and Hrudey Citation1983). Prior to each GP measurement, a 15 mL sample of gas was collected with a syringe and transferred to a 5.9 mL Exetainer (Labco Ltd., High Wycombe, Buckinghamshire, UK), for CH4 concentration determination by gas chromatography. The gas chromatograph (GC; Agilent 7890B, Agilent Technologies, Inc., Santa Clara, CA, USA) was equipped with a thermal conductivity detector (TCD) and a 30 m x 0.32 mm x 3 μm column (GS–CarbonPLOT, Agilent Technologies, Italy). Helium was used as carrier gas with a flow rate of 1.33 mL/min, and an isothermal oven temperature of 35 °C. The injector and detector temperatures were set to 185 °C and 150 °C, respectively (Vera et al. Citation2021). Methane gas of analytical quality (99.99%) was utilised to prepare the standards (Linde®, Santiago, Chile). Standards of CH4 (15, 10, 7.5, 5.0, 2.5 and 1.0%) were prepared by diluting stock CH4 gas with N gas at room temperature. After gas sampling, the bottles were opened to measure pH (Thermo Scientific, Orion Star A121 pH Portable Metre, USA) and remove of the ANKOM®-F57 bags. The bags were washed until water ran clear and thereafter dried at 60° C for 24 h to determine IVDMD (Vera et al. Citation2018). The Partition Factor (PF) was calculated for 24 h of incubation and represents the ratio of degraded DM (DMd; mg) to net gas volume (mL), and represents a measure of fermentation efficiency as proposed by Blümmel et al. (Citation1997).
Determination of total short-chain fatty acids (tSCFA)
After incubation, 1.5 mL samples were collected from each bottle, transferred to 2 mL vials (Biologix Research Co., USA) and mixed with 300 µL metaphosphoric acid (0.25; w/v), centrifuged, filtered (ClarinertTM, 22 μm, Agela Technologies, China) and stored at −20 °C until analysis. The determination of tSCFA was performed by gas chromatography (Agilent 7890B; Agilent Technologies. Inc., Santa Clara, CA, USA) using a 30 m × 0.250 mm × 0.25 μm stainless steel column (DB-FFAP, Agilent Technologies, Milan, Italy) and a flame ionisation detector (Ávila-Stagno et al. Citation2014). The oven initial temperature was 150° C, increased by 5 °C/min to 195 °C, held for 5 min, and maintained for 8 min. Injector and detector temperatures were 225 °C and 250 °C, carrier gas was helium. Concentrations were determined by comparing the retention time and peak area with a SCFA Supelco standard by means of the ChemStation v. 3.2 software (Agilent Technologies, Santa Clara, CA, USA).
Chemical analyses
Samples of diet and biodegraded hay were analysed according to AOAC (Citation1997) procedures for dry matter (DM) (method #934.01), total ash (method #942.05), crude protein (method #954.01), acid detergent fibre (method #973.18; aADFom), acid detergent lignin (method #973.18) and neutral detergent fibre (aNDFom) was determined according to Mertens (Citation2002).
Statistical analysis
Data were analysed with STATA 14 (StataCorp LP, College Station, Texas, USA) using the model: Yjk = µ + δ + αj + βk + (αβ)jk + εijk, where Yjk: is the experimental data; µ: overall mean (of GP, pH, CH4, IVDMD and tSCFA), δ: run effect (random), αj: factor 1 effect (aADFom, fixed effect), βk: factor 2 effect (H-PO, fixed effect), (αβ)jk: interaction between factors and εijk: error. When significant differences were detected between treatments (p < 0.05), a comparison of means was performed with the Tukey test, considering the same level of significance. In addition, polynomial contrasts were used to assess linear and quadratic effects of increasing H-PO in high and low aADFom diets.
Results
In vitro dry matter disappearance, gas and CH4 production
The IVDMD was affected by aADFom concentration in the substrates (p < 0.001) and percentage of inclusion of H-PO (p < 0.001). Inclusion of H-PO at concentrations of 3 and 6% resulted in linear increases of IVDMD by 10 and 12% respectively, whereas high concentrations of aADFom reduced IVDMD by 10% as compared to low aADFom. Total GP was reduced by 19% in the high aADFom diet as compared to low aADFom (p < 0.001), but was unaffected by H-PO concentrations in the diet (p > 0.500). The highest GP was 124.0 mL/g DM incubated (DMi) in the low aADFom diet, whereas for the high aADFom diet it reached only 100.6 mL/g DMi (Table ). The PF was unaffected by H-PO but was increased by aADFom concentration (p = 0.023). Increasing concentrations of aADFom and H-PO resulted in important reductions of net CH4 (mg; p < 0.001). Methane production (mg/g DMi) and CH4 yield (mg/g DMd) were reduced by increasing aADFom and H-PO concentrations in the diets (p < 0.001). Significant aADFom x H-PO interactions were also recorded for these latter 2 parameters and are presented in Figure . The CH4 production and yield were linearly decreased (p < 0.001); the CH4 production varied between 10.5 and 8.2 mg/g DMi, and the yield varied between 18.4 and 12.6 mg/g DMd.
Figure 2. Interaction of acid detergent fibre (aADFom) in the diet × H-PO (P. ostreatus biodegraded hay) concentration sliced by high aADFom and low aADFom diets for (A) methane (CH4) production (mg/g DMi) and (B) CH4 yield (mg/g DMd) at 24 h. Different letters between bars, for CH4 production and CH4 yield indicate significant differences (p < 0.05), for the inclusion of biodegraded hay.

Table 2. Effects of P. ostreatus biodegraded hay and acid detergent fibre in the diet on in vitro dry matter disappearance, gas production, partition factor, and methane (CH4) output production and yield, at 24 h of incubation.
Concentration total short-chain fatty acids (tSCFA)
No interactions between H-PO and aADFom were detected for the concentration of tSCFA (p = 0.910). However, they tended to decrease by aADFom (p = 0.076) and H-PO (p = 0.080) concentrations in the diets. Increasing aADFom concentrations in the diet resulted in increased acetate proportions (p < 0.001) and A:P ratio (p = 0.001), and reduced propionate (p = 0.038) and butyrate proportions (p = 0.001). Increasing concentrations of biodegraded hay also increased acetate proportions (p < 0.001), A:P ratio (p = 0.001), and reduced propionate proportions (p = 0.021) but did not affect butyrate proportions (p = 0.233) (Table ).
Table 3. Total short-chain fatty acids (tSCFA), and percentage of the main tSCFA according to the concentration of P. ostreatus biodegraded hay and acid detergent fibre, at 24 h of incubation.
pH
Incubation pH varied between 6.52 and 6.92 at 24h, with a significant linear increase as the H-PO concentration increased.
Discussion
In vitro dry matter disappearance (IVDMD)
The inclusion of 3 or 6% H-PO in both low and high aADFom diet resulted in increased IVDMD, with the highest values for 6% H-PO. This increase in IVDMD may be attributed to the biodegradation of the hay by P. ostreatus, which alters the structure of the plant cell wall by breaking-down the hay lignocellulosic complex (Shrivastava et al. Citation2012; Zuo et al. Citation2018).
This contrasts with the results of Fazaeli et al. (Citation2002) who found no differences in the IVDMD of diets with forage:concentrate ratios of 1:1 when alfalfa hay was replaced by wheat straw biodegraded by P. ostreatus for 7 weeks, in proportions of 0, 10, 20 and 30% DM. These authors reported unaffected total tract digestibilities of 69% DM. The lack of improvement in dry matter disappearance in the study of Fazaeli et al. (Citation2002) may be due to the excessive time of solid fermentation of wheat straw, leading to fungal fructification with a greater depletion of nutrients in wheat straw. Astudillo-Neira et al. (Citation2022) determined that 14 days corresponds to the minimum time required by P. ostreatus to biodegrade the lignocellulosic complex, through the production of enzymes, without significantly altering the proportion of cellulose and hemicellulose.
Contrastingly, Badarina et al. (Citation2013) reported a decrease of IVDMD at 48 h of incubation of tropical diets including coffee husk fermented with P. ostreatus for 60 days at 0, 10, 20, 30 and 40% DM, concluding that the negative effects on IVDMD were attributable to the characteristics of the substrate, highlighting the need to select proper ingredients for biodegradation, avoiding anti-nutritional components for the rumen ecosystem.
Total gas production
The inclusion of H-PO did not affect GP, although there were differences according to aADFom concentration in the diet. Literature provides examples of fermentations of single ingredients fermented with P. ostreatus, but not complete diets. Among these, wheat straw fermented for 21 days decreased GP in 24 h in vitro incubations (Valizadeh et al. Citation2008), whereas gas volume at 24 h of incubation increased from 30 ml in control to 38 ml in Pleurotus ostreatus treated rice straw (Akinfemi and Ogunwole Citation2012). Corn stover and palm fronds fermented with P. ostreatus for 42 days produced less gas in 72h rumen in vitro incubations than a control substrate as opposed to rice straw which maintained similar GP as compared to control (Tuyen et al. Citation2013). These authors have attributed the differences in GP to variations in the chemical composition of the substrates prior to fungal fermentation, to differences in enzymatic activity between different P. ostreatus strains, and to differences in the fermentation process in solid state (culture medium, temperature, duration of the fungal fermentation).
Partitioning Factor (mg DM/ml gas), H-PO was unaffected, varying between 4.80 and 5.56. Since the gas produced is inversely related to microbial yield (Blümmel et al. Citation1997), the PF value reflects variations in microbial biomass yield (Sembiring and Baba Citation2022). Therefore, the increase in gas production due to the use of diets low in aADFom implies a decrease in FP, which could imply a lower partition of nutrients for microbial performance.
Methane production
The inclusion of H-PO in both diets resulted in decreased CH4 concentration in gas, CH4 production and CH4 yield. Rumen archaea, the main microbes responsible for CH4 production have lipid membranes with glycerol molecules linked to long-chain isoprenoid alcohols through ether bonds (Miller and Wolin Citation2001; Wolin and Miller Citation2006; Jain et al. Citation2014). These membranes require for their synthesis mevalonate, which is formed through the reduction of hydroxymethylglutaryl - Coenzyme A (HMG - CoA), mediated by the action of HMG - CoA reductase (Miller and Wolin Citation2001; Wolin and Miller Citation2006; Jahromi et al. Citation2013). The activity of this enzyme is inhibited by statins, such as lovastatin which is a secondary metabolite of fungi such as Aspergillus terreus, Monascus ruber, Penicillum brevicumpactum and Pleurotus ostreatus (Alarcón and Águila Citation2006). Therefore, the presence of lovastatin in the growth media of P. ostreatus may be inhibiting the formation of membranes of methanogenic archaea without affecting rumen eubacteria, because most of their lipids are glycerol esters of long-chain fatty acids (Wolin and Miller Citation2006). Alarcón et al. (Citation2003) studied the concentration of lovastatin in strain 136 UBB of P. ostreatus, detecting after 10 days a concentration of 70 mg/L, which is higher than the concentrations of other studied strains (124 UBB, 127 UBB and 143 UBB). Miller and Wolin (Citation2001), and Wolin and Miller (Citation2006), demonstrated inhibition of cultures of Methanobrevibacter with 5.6 nmol of mevastatin/mL of growth medium, but did not inhibit the growth of strains of Ruminococcus albus, R. flavefaciens, Butyrivibrio fibrisolvens, Fibrobacter succinogenes and Selenomonas ruminantium with 5.1 nmol of mevastatin/mL of growth medium.
On the other hand, Jahromi et al. (Citation2013) evaluated the lignocellulose degradation potential and CH4 decrease of a methanolic extract obtained from rice straw fermented with Aspergillus terreus. The lignocellulose reduction was significant, but affected cellulose and hemicellulose rather than lignin. The use of a lovastatin-enriched extract from fermented rice straw decreased gas production, CH4 production and increased the concentration of SCFA, particularly acetate, whereas in vitro fermentation of fermented rice straw reduced CH4 production and GP rates without effects on tSCFA production. Authors indicated that the addition of pure lovastatin significantly suppresses methanogenesis, but is too expensive to be used in ruminant diets as a CH4 abatement strategy as compared to the use of agricultural wastes or forages fermented with lovastatin-producing fungi (Ábrego-García, Poggi-Varaldo, Mendoza-Vargas et al. 2021). The main disadvantage of the use of A. terreus would be given by the production of cellulolytic enzymes, which decrease available carbohydrates for microbial fermentation in the rumen, unlike P. ostreatus, which is characterised by its production of lignolytic enzymes (Astudillo-Neira et al. Citation2022). In in vitro incubations of Cenchrus clandestinus (kikuyu grass) with 0.1 mg lovastatin/mL of the buffer/inoculum mixture caused decreases in IVDMD (from 44 to 40%), GP (113 to 97.5 ml/g DMi) and CH4 (14.7 to 10.1 ml/g DMi) (Ramírez et al. Citation2015). These authors attributed the decrease in CH4 to the toxic effect of lovastatin on methanogenic populations. Akinfemi and Ogunwole (Citation2012) reported a 20% reduction in CH4 production from the effect of rice straw biodegradation with P. ostreatus for 21 days, going from 12 to 10 ml CH4 at 24 h. In contrast, Tuyen et al. (Citation2013) reported no effect on CH4 production by incubating corn stover biodegraded by P. ostreatus, but higher productions in rice straw. Although the values presented in the literature are variable, the effect of pre-treatment with P. ostreatus in high-fibre ruminant feeds fermented in vitro causes a decrease in CH4 production or maintains the levels similar to non-pre-treated ingredients.
It is of common consent that fibre (cell walls) increase CH4 production as a result of increased rumen fermentation of structural carbohydrates associated with a lower passage rate and increased acetate to propionate ratio (Boadi et al. Citation2004). However, depending on the composition of these cell walls fermentation can be limited by lignin which can have a barrier effect on cellulose and hemicellulose preventing direct contact of microbes with the substrate (Ellis et al. Citation2007; Kumari and Das Citation2015). In our study the high aADFom diet contained more lignin as compared to the low aADFom diet (3.82 vs 2.82% DM). This variation may have limited the fermentation of cellulose and hemicellulose and as such reduced gas production and net CH4 production (30% lower in the High aADFom diet; Table ). This was partially compensated by the increased IVDMD of the low aADFom diet (10% higher than the high aADFom diet) and resulted in reduced CH4 productions and yield in the high aADFom diets. Additionally, there is a significant interaction between dietary aADFom and H-PO inclusion in the diets on CH4 production and yield. This is explained in Figure which indicates that CH4 production and yield only differ between high and low aADFom diets when 6% H-PO was included.
Total short chain fatty acids (tSCFA)
Production of tSCFA was not affected by the inclusion of H-PO (Table ), but the proportion of acetic acid was increased. Badarina et al. (Citation2013) reported SCFA values at 24 and 48 h that ranged from 107.50 to 158.30 mmol/L, which are similar to those of our study, although SCFA production decreased significantly as a higher proportion of P. ostreatus-fermented coffee husk was included mainly due to a reduction in IVDMD. Soto-Sánchez et al. (Citation2015) reported increases in propionate production and decreases in acetate in 48 h in vitro incubations of barley straw, barley sprout, and combination of both, fermented with P. ostreatus. The inhibition of the HMG-CoA reductase enzyme by the statins present in the growth medium of P. ostreatus blocks the mevalonate synthesis pathway which would imply the accumulation of HMG-CoA, resulting in the accumulation of its precursors acetyl-CoA and acetoacetyl-CoA which could be responsible for the higher proportion of acetic acid production (Arias-Islas et al. Citation2020). This could occur simultaneously with increased fibre degradation by microorganisms, which will have better access to the cell wall matrix thanks to the action of lignolytic enzymes (Nsereko et al. Citation2000) present in the mycelium of P. ostreatus and the biodegradation process prior to ruminal fermentation, which results in a higher proportion of acetate. The type of fermentation product generated from pyruvate depends on the microorganisms and ruminal conditions, such as pH and dilution rate (Nagaraja Citation2016). The carbohydrate fermentation route with high forage diets usually results in increased acetate, decreased propionate, and increased CH4 production, while changing to a low forage diet results in decreased acetate, increased propionate, and CH4 production decreases (Figure ). The inclusion of biodegraded hay implied a decrease in the production and yield of CH4, accompanied by a higher proportion of acetate, suggesting a greater energy efficiency of the rumen microorganisms.
Figure 3. Diagram of carbohydrate ruminal fermentation, indicating end products (tSCFA) and methane (CH4). Some of the potential targets for decreasing CH4 emissions from ruminants are indicated in italics. Source: Adapted from Patra (Citation2012), Ramírez et al. (Citation2015) and Arias-Islas et al. (Citation2020).

These results may seem contradictory as acetate is in normal conditions positively correlated with CH4 production. In this study increases in acetate proportions did not result in increased acetate production as tSCFA were numerically reduced by aADFom and H-PO (p = 0.076 and 0.080 respectively). As such, increased acetate production may have been compensated by reduced tSCFA and GP in the high aADFom diets. Additionally, the inclusion of the H-PO treated hay increased IVDMD with unaffected GP. In this case, results suggest that if fungal statins did inhibit methanogens, hydrogen flow may also have been partially redirected to reductive acetate formation (McAllister and Newbold Citation2008).
As seen in the balances above. Acetogens cannot thermodynamically compete for H2 with methanogens, but in a medium where methanogens are inhibited, hydrogen will flow to alternative pathways which include reductive acetogenesis (Figure ) through the Wood-Ljungdahl pathway (Choudhury et al. Citation2022) thus increasing acetate proportions. This apparent increased energy efficiency was not accompanied by improved feed efficiency due to the increased A:P ratio. A decrease in the A:P ratio in the rumen indicates increased feed efficiency, because acetate is not gluconeogenic in animal tissues, and much of it is oxidised or dissipated as heat during feed digestion in the rumen; in contrast, the amount of propionate is proportional to the non-fibrous carbohydrate contents of the diet (Zuo et al. Citation2018; Wu et al. Citation2016).
pH
Changes in pH values are within ranges considered normal for rumen fermentation as expected when using Menkés Buffer (Menke et al. Citation1979). Despite being significantly increased by aADFom and H-PO, magnitude of changes was rather low and did not affect fermentation results.
Applications and challenges
Although the use of enzymes to improve fibre digestibility in the rumen, is a well-known innovation, the high cost of purified enzymes remains a constraint. The inclusion of P. ostreatus biodegraded hay represents not only a way to include exogenous enzymes into the rumen environment, but also hay with a lignocellulosic matrix undergoing partial degradation, thanks to the 14-day treatment. The use of this biodegraded hay has shown in this experiment encouraging results to improve in vitro DM disappearance, and simultaneously decrease CH4 production. From these results, the two main challenges are to scale up biodegradation technology for hay and other high lignin materials used in animal feed to reach volumes that allow in vivo studies, and to clarify the modifications that occur in the products of ruminal fermentation and ruminant performance.
Conclusions
Results indicate that the inclusion of Pleurotus ostreatus pre-treated hay in both high and low aADFom ruminant diets improves in vitro DM disappearance, reduces CH4 production in a dose-dependent manner and has the potential to be an environmentally friendly feeding alternative for fibrous feedstuffs and by-products.
Ethical approval
CBE-09-2020.
Acknowledgements
The authors thank the staff of the Laboratory of Synthesis and Biotransformation of Natural Products. Department of Basic Sciences, Universidad del Bío-Bío, Chillán, Chile.
Disclosure statement
No potential conflict of interest was reported by the authors.
Data availability statement
The data that support the findings of this study are available from the corresponding author, J.A.S., upon reasonable request.
Additional information
Funding
References
- Ábrego-García A, Poggi-Varaldo HM, Mendoza-Vargas A, Mercado-Valle FG, Ríos-Leal E, Ponce-Noyola T, Calva-Calva G. 2021a. Effects of fermented oat straw as a lovastatin carrier on in vitro methane production and rumen microbiota. Front Energy Res. 9:630701.
- Akinfemi A, Ogunwole OA. 2012. Chemical Composition and in vitro digestibility of rice straw treated with Pleurotus ostreatus, Pleurotus pulmonarius and Pleurotus tuber-regium. Slovak J Anim Sci. 45(1):14–20.
- Akpinar M, Urek RO. 2012. Production of ligninolytic enzymes by solid-state fermentation using Pleurotus eryngii. Prep Biochem Biotechnol. 42(6):582–597.
- Alarcón J, Águila S, Arancibia-Ávila P, Fuentes O, Zamorano-Ponce E, Hernández M. 2003. Production and purification of statins from Pleurotus ostreatus (basidiomycetes) strains. Z Naturforsch. 58c:62–64.
- Alarcón J, Águila S. 2006. Lovastatin Production by Pleurotus ostreatus: effects of the C: n Ratio. Z Naturforsch. 61c:95–98.
- AOAC 1997. Association of official analytical chemists international official methods of analysis. 16th ed. Arlington, Commonwealth of Virginia: AOAC International.
- Arias-Islas E, Morales-Barrera J, Prado-Rebolledo O, García-Castillas A. 2020. Metabolismo en rumiantes y su asociación con analitos bioquímicos sanguíneos. Abanico Vet. 10:1–24.
- Astudillo-Neira R, Muñoz-Nuñez E, Quiroz-Carreno S, Ávila-Stagno J, Alarcon-Enos J. 2022. Bioconversion in Ryegrass-Fescue hay by Pleurotus ostreatus to increase their nutritional value for ruminant. Agriculture. 12:534.
- Ávila JS, Chaves AV, Hernández-Calva M, Beauchemin KA, McGinn SM, Wang Y, Harstad OM, Mc Allister TA. 2011. Effects of replacing barley grain in feedlot diets with increasing levels of glycerol on in vitro fermentation and methane production. Anim Feed Sci Techno. 166–167:265–268.
- Ávila-Stagno J, Chaves AV, Ribeiro GO, Jr, Ungerfeld EM, McAllister TA. 2014. Inclusion of glycerol in forage diets increases methane production in a rumen simulation technique system. Br J Nutr. 111(5):829–835.
- Badarina I, Evvyernie D, Toharmat T, Herliyana EN, Darusman LK. 2013. Nutritive value of coffee husk fermented with Pleurotus ostreatus as ruminant feed. Media Peternakan. 36(1):58–63.
- Bath DL, Marble VL. 1989. Testing alfalfa for its feeding value. Leaflet. 21437. WREP 109. Division of agricultural natural resources. University California, Oakland, CA.
- Beg S, Zafar SI, Shah FH. 1986. Rice husk biodegradation by Pleurotus ostreatus to produce a ruminant feed. Agricul Wastes. 17:15–21.
- Blümmel M, Steingass H, Becker K. 1997. The relationship between in vitro gas production, in vitro microbial biomass yield and 15N incorporation and its implications for the prediction of voluntary feed intake of roughages. Br J Nutr. 77(6):911–921.
- Boadi DA, Wittenberg KM, Scott SL, Burton D, Buckley K, Small JA, Ominski KH. 2004. Effect of low and high forage diet on enteric and manure pack greenhouse gas emissions from a feedlot. Can. J Anim Sci. 84:445–453.
- Choudhury PK, Jena R, Tomar SK, Puniya AK. 2022. Reducing Enteric Methanogenesis through Alternate Hydrogen Sinks in the Rumen. Methane. 1:320–341.
- Ellis JL, Kebreab E, Odongo NE, McBride BW, Okine EK, France J. 2007. Prediction of Methane Production from Dairy and Beef Cattle. J. Dairy Sci. 90(7):3456–3466.
- Fazaeli H, Jelan ZA, Mahmodzadeh H, Liang JB, Azizi A, Osman A. 2002. Effect of fungal treated wheat straw on the diet of lactating cows. Asian-Aust J Anim Sci. 15(11):1573–1578.
- Fedorah PM, Hrudey SE. 1983. A simple apparatus for measuring gas production by methanogenic culture in serum bottles. Environ Sci Technol Lett. 4(10):425–432.
- Foggi G, Terranova M, Conte G, Mantino A, Amelchanka SL, Kreuzer M, Mele M. 2022. In vitro screening of the ruminal methane and ammonia mitigating potential of mixtures of either chestnut or quebracho tannins with blends of essential oils as feed additives. It J Anim Sci. 21(1):1520–1532.
- Getachew G, Crovetto GM, Fodevila M, Krishnamoorthy U, Sigh B, Spanghero M, Steingass H, Robinson PH, Kailas MM. 2002. Laboratory variation of 24 h in vitro gas production and estimated metabolizable energy values of ruminant feeds Anim. Feed Sci Technol. 102:171–182.
- Jahromi MF, Liang JB, Mohamad R, Goh YM, Shokryazdan P, Ho YW. 2013. Lovastatin-enriched rice straw enhances biomass quality and suppresses ruminal methanogenesis. BioMed Res Int. 2013:397934.
- Jain S, Caforio A, Driessen AJM. 2014. Biosynthesis of archaeal membrane ether lipids. Front Microbiol. 5:1–16.
- Khalil JK, Sawaya WN, Hyder SZ. 1986. Nutrient composition of Atriplex leaves grown in Saudi Arabia. Journal of Range Management. 39:104–107.
- Kumari S, Das D. 2015. Improvement of gaseous energy recovery from sugarcane bagasse by dark fermentation followed by biomethanation process. Bioresour Technol. 194:354–363.
- McAllister TA, Newbold CJ. 2008. Redirecting rumen fermentation to reduce methanogenesis. Aust J Exp Agric. 48:7–13.
- Menke KH, Raab L, Salewski A, Steingass H, Fritz H, Schrieder W. 1979. The estimation of the digestibility and metabolizable energy content of ruminant feeding stuffs from the gas production when they are incubated with rumen liquor in vitro. J Agric Sci. 93:217–222.
- Menke KH, Steingass H. 1988. Estimation of the energetic feed value obtained from chemical analysis and gas production using rumen fluid. Anim Res Dev. 28:7–55.
- Mertens DR. 2002. Gravimetric determination of amylase-treated neutral detergent fiber in feeds with refluxing in beakers or crucibles: collaborative study. J AOAC Int. 85(6):1217–1240.
- Miller TL, Wolin MJ. 2001. Inhibition of growth of methane-producing bacteria of the ruminant forestomach by Hydroxymethylglutaryl ∼ SCoA reductase inhibitors. J Dairy Sci. 84(6):1445–1448.
- Nagaraja TG. 2016. Chapter 2, Microbiology of the rumen. In: Millen D, De Beni-Arrigoni M, Lauritano-Pacheco R, editors. Rumenology. Cham: Springer. p. 39–61.
- Nsereko VL, Morgavi DP, Rode LM, Beauchemin KA, McAllister TA. 2000. Effects of fungal enzyme preparations on hydrolysis and subsequent degradation of alfalfa hay fiber by mixed rumen microorganisms in vitro. Anim Feed Sci Technol. 88:153–170.
- Patra AK. 2012. Enteric methane mitigation technologies for ruminant livestock: a synthesis of current research and future directions. Environ Monit Assess. 184(4):1929–1952.
- Purcell PJ, ÓBrien M, Boland TM, ÓDonovan M, ÓKiely P. 2011. Impacts of herbage mass and sward allowance of perennial ryegrass sampled throughout the growing season on in vitro rumen methane production. Anim. Feed Sci Technol. 166–167: 405–411.
- Rahman MM, Lourenço M, Hassim HA, Baars J, Sonnenberg A, Cone JW, De Boever J, Fievez V. 2011. Improving ruminal degradability of oil palm fronds using white rot fungi. Anim Feed Sci Technol. 169(3-4):157–166.
- Ramírez JF, Posada-Ochoa S, Rosero-Noguera R. 2015. Effect of lovastatin on in vitro methane production and dry matter digestibility of kikuyu grass (Cenchrus clandestinus). Ces Med Vet Zootec. 10(2):111–121.
- Scoones I. 2022. Livestock, methane, and climate change: the politics of global assessments. WIREs Clim Change. 14:e790.
- Sembiring M, Baba ASH. 2022. In Vitro Gas Production Test: towards Rapid Nutritional Evaluation of Roughages for Ruminant Feeding. Formosa J Sci Technol. 1(4):247–258.
- Shrivastava B, Nandal P, Sharma A, Jain KK, Khasa YP, Das TK, Mani V, Kewalramani NJ, Kundu SS, Kuhad RC. 2012. Solid state bioconversion of wheat straw into digestible and nutritive ruminant feed by Ganoderma sp. rckk02. Bioresour Technol. 107:347–351.
- Soto-Sánchez A, Ramírez-Bribiesca JE, Meneses-Mayo M, Loera-Corral O, Miranda-Romero LA, Bárcena-Gama R. 2015. Effects of Pleurotus sapidus (Schulzer) Sacc. treatment on nutrient composition and ruminal fermentability of barley straw, barley rootless and a mixture of the two. Chil J Agr Res. 75(3):313–319.
- Suescún-Ospina ST, Vera N, Astudillo R, Yunda C, Williams P, Allende R, Avila-Stagno J. 2022. Effects of País grape marc inclusion in high and low forage diets: ruminal fermentation, methane production and volatile fatty acids. Ital J Anim Sci. 21(1):924–933.
- Tuyen DV, Phoung HN, Cone JW, Baars JJP, Sonnenberg ASM, Hendriks WH. 2013. Effect of fungal treatments of fibrous agricultural by-products on chemical composition and in vitro rumen fermentation and methane production. Bioresour Technol. 129:256–263.
- Valizadeh R, Sobhanirad S, Mojtahedi M. 2008. Chemical composition, ruminal degradability and in vitro gas production of wheat straw inoculated by Pleurotus ostreatus Mushrooms. J Anim Vet Adv. 7(11):1506–1510.
- Vera N, Gutiérrez C, Allende R, Williams P, Fuentealba C, Ávila-Stagno J. 2018. Dose–response effect of a pine bark extract on in vitro ruminal ammonia and methane formation kinetics. Acta Agric Scand A Animal Sci. 68:181–189.
- Vera N, Gutiérrez C, Williams P, Fuentealba C, Allende R, Ávila-Stagno J. 2021. Low concentrations of a polyphenolic extract from pine bark in high–concentrate diets decrease in vitro rumen ammonia nitrogen but not methane production. J Appl Anim Res. 49(1):413–422.
- Wolin MJ, Miller TL. 2006. Control of rumen methanogenesis by inhibiting the growth and activity of methanogens with hydroxymethylglutaryl-SCoA inhibitors. Inte Congr Ser. 1293:131–137.
- Wu H, Meng Q, Yu Z. 2016. Evaluation of ferric oxide and ferric citrate for their effects on fermentation, production of sulfide and methane, and abundance of select microbial populations using in vitro rumen cultures. Bioresour Technol. 211:603–609.
- Zuo S, Niu D, Zheng M, Jiang D, Tian P, Li R, Xu C. 2018. Effect of Irpex lacteus, Pleurotus ostreatus and Pleurotus cystidiosus pretreatment of corn stover on its improvement of the in vitro rumen fermentation. J Sci Food Agric. 98(11):4287–4295.