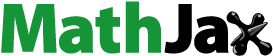
Abstract
In the European Union, the withdrawal of pharmaceutical zinc oxide (ZnO) poses challenges for post-weaning pigs management. The present work aims to elucidate, using a meta-analytical and a systematic review approach, the effects of different doses and forms of zinc (Zn) on health, performance, and gut health of weaning pigs. A total of 309 articles were identified from a literature research from 2001 to 2021; 85 were selected. Articles were divided in 3 datasets to evaluate: 1) the effects of ZnO doses (ZnO database); 2) the efficacy of ZnO on infection models (challenge database); 3) the effect of different Zn sources (special Zn database). The experimental groups were classified according to their Zn dose: low Zn (LZn: 0–200 ppm); medium (M1Zn: 201–630 ppm; M2Zn: 631–1600 ppm); high (HZn:>1601 ppm). Dataset 2 and 3 were analysed by a systematic review approach. Results showed that M1Zn and M2Zn guarantee comparable average daily gain, villus height and crypt depth to HZn. The HZn group had higher Escherichia coli (p = 0.04) in the small intestine. The ZnO database confirmed that Zn doses between 600 and 1600 ppm had comparable results for intestinal function and growth performance to pharmacological doses of Zn. The challenge dataset confirmed the positive effect of HZn in counteracting infections resulting in higher performance, lower diarrhoea and better intestinal morphology. The special zinc dataset suggested that coated, capsulated and nano Zn represent the most promising alternative sources to reduce the detrimental effects of weaning on pigs.
HIGHLIGHTS
EU ban on pharmacological levels of ZnO raises concerns in pig production.
Data indicates Zn’s effect is dose-dependent, but also Zn form influence the effectiveness.
Gut functionality and growth can be guaranteed with 600–1600 ppm of ZnO.
Coated, capsulated and nano Zn are promising sources to reduce the Zn doses.
Keywords:
Introduction
Weaning is one of the most stressful events in the life of pigs and is characterised by changes in the gut morphology and gut microbial ecosystem that can lead to post-weaning diarrhoea (PWD) (Campbell et al. Citation2013). Until June 2022, zinc oxide (ZnO) was used in the European Union (EU) at a pharmacological dose (2.5 g/kg) to prevent PWD occurrence in weaned pigs (Looft et al. Citation2012). The use of high dose of ZnO in pig diets began in the 1980s at doses above 2500 ppm (Bonetti et al. Citation2021). ZnO supplementation in diets can generally result in a lower incidence of diarrhoea, lower mortality, improved growth performance, digestion and feed intake in postweaning pigs (Bonetti et al. Citation2021). However, the strong correlation between the use of pharmacological ZnO in pig diets and accumulation of zinc (Zn) in the environment (Meyer et al. Citation2002; Buff et al. Citation2005;) led the European Commission to ban the use of high doses of ZnO in feed. In addition, the evidence of the synergy between antibiotic and pharmacological ZnO in the co-selection of bacteria resistant to antimicrobials, posing a potential threat to human, animal and global health, supports the EU decision (Schwarz and Chaslus-Dancla Citation2001; Broom et al. Citation2006;). Finding new alternatives to antibiotics and pharmacological ZnO levels is therefore essential to maintain the efficacy of current antimicrobials and to reduce Zn accumulation in the environment.
However, in order to define and develop feeding strategies capable of effectively replacing pharmacological levels of ZnO, an understanding of its mode of action is still required. Indeed, Zn is an important micronutrient which is involved in a large number of physiological processes in animals and it is involved in the synthesis of more than 300 enzymes which are related to gut growth and immune function (Bonetti et al. Citation2021). In fact, Zn is important for the maintenance of intestinal barrier function, as it aids in the regeneration of damaged epithelial tissue (Alam et al. Citation1994; McDonald Citation2002; Siddiqi et al. Citation2018) and the rise of brush border enzymes (Sloup et al. Citation2017). A deficiency in Zn leads to the villi atrophy in the jejunum, a reduction of the number of villi per unit area and, thus, a reduction in the absorptive area and secretion of digestive enzymes (Castillo et al. Citation2007; Heo et al. Citation2013).
Nowadays, in the EU, up to 150 ppm of Zn is allowed in the pigs’ diet, which is higher than the defined requirements for weaned pigs as reported by Revy et al. (Citation2006), whom analysed performance parameters as well as liver, bone and plasma Zn concentrations, and NRC (Citation2012). However, according to a recent study by Hansen et al. (Citation2022), the requirement for the first two weeks is 1400 ppm. Considering the European diets, an average amount of 30–40 ppm of Zn is provided with the feed ingredients (Blaabjerg and Poulsen Citation2017). Therefore, regardless the EU restriction, there is room to cover this gap with multiple Zn sources.
For this reason, inorganic and organic Zn sources, included in the diet at lower doses, were evaluated. Regarding the inorganic source of Zn, Zinc sulphate (ZnSO4) has been used as a primary nutritional source in the feed for a long time (Villagómez-Estrada et al. Citation2020), thanks to its good solubility in the gut and further systemic effect. Regarding the organic sources of Zn, Zn methionine (Zn-Met) and Zn glycine (Zn-Gly) have been evaluated (Hudson et al. Citation2005; Huang et al. Citation2007;) and have been found to positively impact the animal growth performance (Hudson et al. Citation2005; Huang et al. Citation2007; Diao et al. Citation2021) and immune status (Ao et al. Citation2009; Feng et al. Citation2010) when compared with inorganic Zn sources. More recently, additional sources of Zn including coated, capsulated, microencapsulated, porous or high surface Zn forms were investigated showing some promising results (Vahjen et al. Citation2016; Long et al. Citation2017; Dong et al. Citation2019; Liu et al. Citation2020; Cui et al. Citation2021). However, a systematic approach revising the effects of these Zn sources as compared to pharmacological doses of ZnO is still not available.
A systematic literature review followed by a meta-analytical approach represent a valid method to overcome the uncertainty of the results observed in different studies and to help understanding the mode of action and the effects of pharmacological ZnO levels and different Zn sources. To the authors’ knowledge, only one meta-analysis regarding the effect of ZnO in post-weaning pigs has been carried out but it was mainly focusing on the performance parameters (Sales Citation2013). Regarding the novel Zn forms, a recent review of Lei et al. (Citation2022) is available, however, the objective of this review was to analyse the effect of Zn as a growth promoter in monogastric animals without focusing the attention on the gut health in post-weaning pigs (Lei et al. Citation2022). Therefore, the aim of the present study was to improve the knowledge regarding the effect of the use of Zn at different doses and forms on the health, productive performance, and gut health of post-weaning pigs. The first approach adopted was to use a systematic review methodology; when enough studies were found, a meta-analytic approach was applied.
Materials and methods
Data collection and datasets creation
A systematic literature review was carried out using peer-reviewed articles published between 2001 and 2021. The online databases Web of Science and Google Scholar were used to search for scientific peer-reviewed articles by combining the keywords: ‘zinc’, ‘piglets’ and/or ‘pigs’. A total of 309 articles were found. Once collected, only the articles fulfilling the following criteria were retained: a) include in-vivo swine studies with a control group with at most 200 ppm of Zn (LZn group), b) published in English and c) reporting at least one parameter regarding growth performance, gut integrity, gut microbial population, blood parameters related to health, and genes expression. The meta-analysis, reviews and duplicate articles were excluded. The experimental groups included in the studies were classified into four Zn level classes as follows: 1) Low Zn (LZn) when total Zn in the feed formula was included between 0 ppm to 200 ppm; 2) Medium dose 1 (M1Zn) when Zn was included between 201 ppm to 630 ppm; 3) Medium dose 2 (M2Zn), when Zn was included between 631 ppm to 1600 ppm; 4) High Zn (HZn) with Zn levels from 1601 ppm to 3000 ppm. For some of the parameters analysed, a low number of studies were including the M1Zn and M2Zn groups; therefore, the M1Zn and M2Zn groups were merged into the MZn group (201 ppm to 1600 ppm) when < 3 studies were available.
All the response data regarding pigs were expressed as relative to the LZn group within each study as follow: (Luise et al. Citation2021).
ValueLZn: specific value of the LZn group
ValueTRT: specific value of the M1Zn, M2Zn or HZn groups.
The retained articles were then used to build three different databases:
ZnO level dataset: this dataset had the aim to gain a better understanding of how different doses of ZnO can affect the growth performance, health status, intestinal morphology, microbiology, and gene expression (tight junctions and cytokine) of weaned pigs. For this dataset, studies including a group in which the control group had other external elements such as probiotics or amino acids were removed. The ZnO level dataset included 41 studies; in these studies, the average initial age of pigs was 25.2 ± 4.8 days, the initial body weight (BW) was 7.42 ± 1.58 kg, and the average experimental duration of the in vivo trial was of 20.3 ± 10 days. This dataset was used to carry out a meta-analysis.
Challenge dataset: this dataset included only the studies in which ZnO was studied under a challenge infection model in post-weaning pigs. The Challenge dataset included 8 studies and the most common challenge model was with the enterotoxigenic Escherichia coli (ETEC) F4 followed by Salmonella and Transmissible Gastroenteritis Virus (TGEV). In these studies, the average initial age of pigs was 25.9 ± 2.9 days, the initial BW was 7.05 ± 0.67 kg, and the average experimental duration of the in vivo trial was of 21.6 ± 8.5 days. The results of this dataset were presented as a systematic review.
Special Zn dataset: this dataset had the aim to highlight the main output of the use of different Zn sources, compared to HZnO doses, on the intestinal health of weaned pigs. The special Zn dataset included 36 studies. In these studies, the average initial age of pigs was 25.8 ± 6.5 days, the initial BW was 7.7 ± 1.79 kg, and the average experimental duration of the in vivo trial was of 22.4 ± 8.6 days. The results of this dataset were presented as a systematic review. The reason behind this approach is the lack of articles for each specific source of Zn.
Statistical analysis
Statistical analyses were carried out using the Minitab8 software (MINITAB®). The analyses were performed using a General Lineal Model and ANOVA model, considering the Zn level-doses (LZn, M1Zn, M2Zn, HZn) as fixed factor, the research study as random factor and the basal Zn (Zn included in the diet derived from the raw materials) as covariate. Pairwise comparisons for the one-way ANOVA model were then carried out between the groups. Growth performance, including average daily gain (ADG) and gain to feed (G:F), intestinal morphological parameters including villus height, villus width and crypt depth, Zn concertation in blood and liver, intestinal and faecal concertation of Escherichia coli (E. coli) and Lactobacillus, and intestinal expression of genes related to intestinal barrier and integrity and inflammation including Zonulin-1 (ZO-1), Occludin (OCLN), Interleukin-1 beta (IL-1β), Tumour necrosis factor (TNF-α), Interferon gamma (IFN-γ) and Transforming growth factor-β (TGF-β) were analysed as response parameters. For the intestinal morphology analysis, the intestinal tract segment (duodenum, jejunum, and ileum) was tested as factor, but it was never found significant.
Results and discussion
ZnO levels dataset
Effect of different doses of zinc oxide on growth performance and Zn concentrations in organs
Table shows the results of the different ZnO levels on the growth performance and Zn content in the blood and liver of post-weaning pigs. The Zn level classes significantly influenced the ADG (p < 0.001), G:F (p = 0.02), and the concentration of Zn in the blood (p = 0.003) and liver (p = 0.01). In particular, significantly higher ADG values were found in M2Zn and HZn groups compared with the LZn group (p < 0.05), while no difference was observed comparing the M1Zn with the other groups. G:F was significantly higher in the HZn group compared with LZn group (p < 0.05).
Table 1. Effect of different doses of ZnO on performance and Zn content in blood and liver of post weaning pig.
Several studies have reported positive effects of pharmacological level of ZnO (3000 mg kg−1) on the growth performance of weaned pigs (Carlson et al. Citation1999; Lei and Kim Citation2018; Hansen et al. Citation2022), but studies focused on defining an optimal dosage are scarce. A previous study by Hill et al. (Citation2001), conducted in nine university research facilities with 1,978 weaned pigs, reported that the ADG linearly increase with the inclusion of Zn from 200 ppm until reaching a plateau point around 1500–2000 ppm concentration. Comparable results were observed by Zhang and Guo (Citation2007), in which study reported that the ADG and ADFI of pigs did not change when dietary Zn increase from 2250 to 3000 mg/kg diet. In accordance with these results, the present meta-analysis showed that a dosage between 201 and 1600 ppm can give comparable ADG results to dosages comprised between 1601 and 3000 ppm. On the other hand, a study conducted by Mavromichalis et al. (Citation2000) found that a linear increase in ADG can be found in up to 3,000 mg Zn/kg diet. Differences observed in these studies can both be linked to different environmental and management conditions as weaning age, since Zn level can be more effective in pigs weaned earlier (Hill et al. Citation2001).
The blood Zn concentration was higher in the HZn group compared to the LZn group (p < 0.05), while the M1Zn group had intermediate values compared to LZn and HZn. This result indicated that Zn values in the blood are proportional to the dose of Zn in the diet. Similarly, the concentration of Zn in the liver increased with the increasing concentration of Zn level in the diet (p < 0.05). Due to the lack of the medium group, it was not possible to estimate whether the effect is linearly increased or not. This result is in accordance with the literature, confirming that the Zn concentration in the liver is directly proportional to the addition of Zn in the diet (Jensen-Waern et al. Citation1998).
Effect of different doses of zinc oxide on the intestinal morphological parameters and gene expression
The correct and timely development of the small intestine is fundamental for the proper digestive and immune function (Adewole et al. Citation2016). After weaning, pigs are subjected to changes in the gut morphology as crypt hyperplasia and villous atrophy, which lead to a temporary diminishment of absorption and digestion in the small intestine (McDonald Citation2002). The results of the present meta-analysis showed that the Zn level classes significantly affected villus height (p = 0.004) and villus width (p = 0.003), while no effect was observed on crypt depth (Table ). The HZn group had higher villus height and villus width values compared with the LZn group (p < 0.05), while no differences were observed between HZn and MZn for the villus height. The beneficial role of ZnO in maintaining the intestinal integrity and increasing the absorptive capacity of the intestinal mucosa is well known and it is one of the main mechanisms behind the beneficial effect of Zn on growth performance (Roselli et al. Citation2003; Pearce et al. Citation2015). No clear or univocal explanation for the results obtained can be found in the literature; it could be speculated that the beneficial effect of HZnO on the height of the intestinal villi could be related to the antibacterial activity of ZnO (Söderberg et al. Citation1990; Roselli et al. Citation2003), to the promoting effect that ZnO has on increasing insulin-like growth factor-1 levels in blood and intestinal ghrelin secretion in post-weaning pigs, which may result in stimulation of the mucosal growth (Carlson et al. Citation2004; Li et al. Citation2006; Yin et al. Citation2009). An additional mode of action by which HZnO can improve the intestinal villi is via reduction of the intestinal inflammation and particularly modulating the mitogen-activated protein kinase (MAPK) signalling pathway (especially the p38 and JNK signalling pathways) which, in turn, regulate the cytokine levels (Song et al. Citation2015). Furthermore, it has been suggested that HZnO can also regulate and restore intestinal permeability via modulation of the tight junction (TJ) proteins (Pié et al. Citation2004; Al-Sadi et al. Citation2009). The results obtained in the present meta-analysis for the expression of the genes related to intestinal inflammation and TJ proteins highlight the role of ZnO in these aspects. Indeed, as reported in Table , the level of ZnO significantly influenced the intestinal expression of IFN-γ (p = 0.01), which was higher in LZn group compared with HZn and tended to influence the expression of TNF-α (p = 0.09). Furthermore, the level of ZnO significantly influenced the intestinal expression of ZO-1 (p < 0.01), which was higher in the HZn group compared with the LZn group (p = 0.01) and tended to influence the Occludin expression (p = 0.06). The Interferon Gamma is a pro-inflammatory cytokine mainly generated by lymphocytes and antigen-presenting cells (Schroder et al. Citation2004), and it can work in tandem with the cytokine TNF-α and cause structural alterations of the TJ (Roselli et al. Citation2003). Pro-inflammatory cytokines including IFN-γ and IL-1β are usually upregulated in the intestinal mucosa of pigs immediately after weaning (Pié et al. Citation2004), and an excess in their upregulation can negatively impact the intestinal integrity, causing a pathological weakening of the TJ barrier and increase gut barrier vulnerability (Al-Sadi et al. Citation2009). The results of the present meta-analysis confirm the capacity of high levels of ZnO to reduce pro-inflammatory cytokines, including INF-y, while no clear effect was observed on anti-inflammatory cytokines. With regard to TJ proteins, the results suggested a primary role for high levels of ZnO on ZO-1 expression. The ZO-1 is one of the major intracellular located adaptor proteins that, together with Claudins, play a key role in regulating the flux of small molecules (pore pathway). It also has a synergistic relationship with Occludin, which regulates the flux of large molecules (leak pathway) and the intestinal permeability (Tajik et al. Citation2020). Furthermore, the present meta-analysis highlights a lack of information regarding the effect of Zn on the TJ, notable by the low number of studies available for these parameters, therefore additional research on these aspects would be desirable.
Table 2. Effect of different doses of ZnO on morphological parameters of small intestine.
Effect of different doses of zinc oxide on the intestinal bacterial abundance of post weaning pigs
It is widely reported that ZnO can modulate the bacteria population and exert antibacterial activity, therefore, in the present meta-analysis, the effect of the different doses of ZnO was investigated on E. coli and Lactobacillus population as main markers for detrimental and beneficial bacteria, respectively (Bonetti et al. Citation2021). The results showed no effect of ZnO level of E. coli and Lactobacillus abundance in the faeces and large intestine, while it significantly affected the abundance of E. coli and Lactobacillus in the small intestine (Table ). The abundance of E. coli was higher in the HZn group compared with the LZn (p = 0.04), and the abundance of Lactobacillus was highest in the LZn group compared with the HZn group (p = 0.05). The enhanced E. coli presence in HZn group could be explained by one of the hypothesised modes of action of Zn on the intestinal eubyosis. Indeed, it has been suggested that Zn can induce a decrease of the secretory capacity of the small intestinal epithelium, limiting the adherence of bacteria (Zhang et al. Citation2019), which may have altered the commensal bacteria favouring the colonisation of the gut by the E. coli. Moreover, the effect of ZnO on the gut microbiota could also be indirect and due to a modulation of the interaction between potentially pathogenic bacteria and the host (Carlson et al. Citation2004). However, considering the overall results of the present meta-analysis, the high abundance of E.coli and the reduced abundance of Lactobacilli due to ZnO supplementation would not necessarily imply an increase in the inflammatory processes but could be explained by an improvement in the pigs’ tolerance to E. coli colonisation (Fairbrother et al. Citation2005). In fact, according to our results on gene expression and morphological parameters, an increase in abundance in E. coli does not seems to be related to inflammatory processes or to disruption of intestinal functionality. Studies with results in agreement with ours (Roselli et al. Citation2003; Fairbrother et al. Citation2005; Hu et al. Citation2014) and with opposite results (Shi et al. Citation2019) can be found.
Table 3. Effect of different doses of ZnO on pig’s microbial abundance.
Overall, the present meta-analysis has first highlighted that there is a lack of studies which have evaluated the effects of intermediate doses of Zn (200–600 ppm) on the main parameters related to intestinal health. However, it allowed to confirm that averagely high doses of Zn (600–1600 ppm) were able to lead to equally positive results for intestinal function (villus height) and growth performance compared to pharmacological doses of Zn. Furthermore, the present meta-analysis highlighted the potentially negative effect of pharmacological doses of Zn on the abundance of considered positive bacteria such as Lactobacilli, suggesting that there are other modes of action that could explain the positive effect of ZnO, especially administrated at higher doses, on intestinal health. It should also be emphasised that it is not possible to draw robust conclusions from observations based only on the abundance of Lactobacilli and E. coli, as the microbial population inhabiting the gut is very large and diverse, and further studies based on NGS technology will be helpful in the future.
Challenge database
The challenge models (infecting the pigs with specific pathogens, rearing them under stressful conditions), are considered a valid strategy to stress the tested dietary solutions, resulting in observations which would be more close to field situations (Luise et al. Citation2019). Therefore, different challenge models have been used; and one of the most used is based on ETEC F4 and F18 infection in weaning pigs (Luise et al. Citation2019). Indeed, among the studies identified with the systemic review, six out of eight studies were using the infection with ETEC F4 as main pathogen, one study infected the pigs with the Salmonella enterica Serovar Typhimurium DT104 and one study infected the pigs with TGEV.
The results of the systematic review regarding the effect of the ZnO levels on the performance, health and intestinal health under challenge situations are reported in Table . The results of LZn and MZn were reported in comparison with the HZn levels (2500–3000 ppm).
Table 4. Effect of different doses of zinc oxide on the performance, health, and gut health parameters in weaned pigs under challenge conditions.
Overall, it can be observed that the LZn dose did not achieve similar results compared to HZn, indeed, except in two studies performed by Mazzoni et al. (Citation2010) and Han et al. (Citation2018) in which the BW, ADG and ADFI were similar to the ones of the HZn groups (2500 ppm of ZnO in both studies), the other studies suggested that the LZn doses, under challenge condition, reduced the performance parameters of pigs. Less studies investigated the effect of MZn compared with HZn. According to the study of Stensland et al. (Citation2015) lower BW, ADG and ADFI were observed in pigs receiving 220 ppm of Zn compared with pigs receiving HZn dose (3000 ppm ZnO), while the study of Lei and Kim (Citation2020) suggested that results similar to the pigs fed with HZn (2500 ppm ZnO) can be obtained using Zn doses between 500 and 1000 ppm.
Considering the health parameters, and especially the diarrhoea and faecal score data, the systematic review suggested that both LZn and MZn were not able to archive similar results of the HZn dose. Those results confirmed the positive effect of the addition of pharmacological level of ZnO in reducing the incidence of diarrhoea, even in challenged animals.
However, it is interesting to note that in the study of Janczyk et al. (Citation2013), in which pigs were challenged with Salmonella enterica Serovar Typhimurium DT104, the pigs receiving the LZn (80 and 150 ppm) dose had a lower Salmonella shedding and higher cytotoxic T-cells in blood and a higher number of different T cells in the mesenteric lymph nodes six weeks after the feeding the two different diets. The authors suggested that the HZn dose (2500 ppm ZnO) was beneficial immediately after the infection while after two weeks of feeding all positive beneficial effects of HZn disappeared and rather negative drawback, including a suppressive effect on the pig’s immunity, occurred (Janczyk et al. Citation2013).
The results regarding the morphometric parameters agree with the results observed for the gut heath parameters. In fact, the LZn doses provided in the studies were not able to allow pigs to have similar morphological parameters and faecal pathogen expression as the HZn doses. In particular, the study of Kwon et al. (Citation2014) showed that pigs receiving the LZn dose had higher faecal shedding, higher pH in the stomach, jejunum, ileum, caecum and colon, lower goblet cells in the jejunum and colon and lower VH, CD and VH:CD ratio in the jejunum and ileum. Reduced duodenum VH were observed also by Mazzoni et al. (Citation2010) and Lei and Kim (Citation2020), reduced VH in jejunum and reduced VH:CD in duodenum and ileum were observed by Chai et al. (Citation2014) and Lei and Kim (Citation2020), respectively. In addition, higher jejunal expression of Interferon alfa (IFN- α) and 2′-5′-Oligoadenylate Synthetase 1 (OAS) and a higher jejunal permeability was observed in LZn pigs challenged with Transmissible gastroenteritis virus by Chai et al. (Citation2014). These results confirm that in challenged pigs the LZn doses were not able to maintain the same gut heath than HZn. According to the results of Lei and Kim (Citation2020), doses between 500 and 750 ppm were able to maintain a VH in the duodenum, jejunum and ileum and similar VH:CD ratio in the duodenum and jejunum as compared with HZn dose, suggesting that MZn doses could alleviate the gut impairment, while according to the study of Stensland et al. (Citation2015), the MZn were not able to maintain similar gut condition of HZn dose. In this latter study, the pigs with the MZn had higher ETEC F4, ETEC F4/Lactobacillus ratio and faecal valeric acid than pig receiving the HZn. Although the two studies analysed some parameters related to the gut health, they cannot be compared, therefore no clear conclusion can be drawn.
Intestinal morphology showed decreased VH and enhanced CD in low Zn group by Kwon et al. (Citation2014), similarly to what happen naturally in weaned pigs. On the contrary, it was shown an increased VH and lowered CD in animals with the addition of 100 ppm of coated Zn as well as them who received 2500 ppm of ZnO. This could suggest a beneficial effect of the gut structure induced by ZnO. Improved intestinal health results linked to the supplementation of coated Zn were also found in Han et al. (Citation2018), where higher VH:CD values in jejunum resulted in pigs receiving M1Zn dose compared to whom was supplemented with pharmacological level of ZnO. Moreover, the increased presence of the tight junction protein ZO-1 in pigs receiving 2500 ppm of ZnO could indicate and increased level of barrier against E. coli.
Overall, the administration of HZnO doses confirmed its positive effect in enhancing both productive and gut health parameters, even in animals challenged with ETEC F4, Salmonella and TGEV.
Special zinc database
One of the most promising strategies to reduce the quantity of ZnO in post-weaning diets is to identify different Zn forms which allow improving Zn bioavailability. According to the study performed by Schell and Kornegay (Citation1996), it was already known that the bioavailability of Zn from standard ZnO is usually lower than that from other Zn sources, including ZnSO4, Zn-methionine and Zn-lysine. More recently the technology allowed the production of ZnO nanoparticles (Siddiqi et al. Citation2018) or porous source of Zn (Peng et al. Citation2019; Wang et al. Citation2019) which allowed to increase in Zn availability and therefore reduced the doses needed in the diet. In this dataset, the effects of the different Zn sources including Zn chelate, coated, capsulated and microencapsulated Zn, nano Zn, and porous Zn were compared to the one of HZnO.
Zn chelates
Historically, a highly investigated strategy to improve the zinc absorption in the intestine is represented by the use of chelated Zn (Richards et al. Citation2010). The chelation refers to a bond formed between the metal/mineral and a ligand. The ligand can bond the mineral in several points resulting in a structure in which the metal atom becomes part of a ring. For their structure and chemical proprieties (amino and hydroxyl functional groups), amino acids (AAs) are the most suitable molecules to chelate minerals. Studies carried out in the 90s on rats and chickens showed how the use of zinc chelated with different AAs was able to have greater bioavailability than the form of zinc sulphate (Wapnir and Stiel Citation1986; Hortin et al. Citation1991; Wedekind et al. Citation1992). Thereafter, some studies were carried out in pigs; according to the study of Swinkels et al. (Citation1996) the Zn-chelate with AAs was able to enhance Zn concentration in serum and tissue compared to Zn sulphate in Zn depleted pigs. The authors hypothesised that the beneficial effects of the chelated Zn sources were related to the AAs since they were able to prevent the Zn binding to non-absorbable dietary compounds leading to increasing Zn absorption and (or) enhancing Zn retention. A positive effect on performance parameters was observed in the study by Pieper et al. (Citation2020), where they observed similar feed intake (FI) and ADG in both 2500 ppm ZnO and 110 Zn-Lysinate groups, suggesting the possibility of using a nutritional dose of Zn-Lysinate to improve growth parameters at weaning. Similarly, in the study by Case and Carlson (Citation2002), the administration of 500 mg/kg as chelated ZnO with AAs complex was able to achieve similar G:F, ADG and average daily feed intake (ADFI) compared to HZnO (3000 ppm as ZnO). In addition to AAs, Zn can also be chelated by various types of polysaccharides. In the same study by Case and Carlson (Citation2002) showed that 500 ppm of SQM-Zn (Zn chelated with polysaccharides) was able to achieve the same ADG, G:F and ADFI as the administration of 3000 ppm of ZnO during the first three weeks post-weaning. On the contrary, in the study by Bosi et al. (Citation2003), the 200 ppm of Zn-glutamate resulted in similar duodenal villus height as in pigs fed 2500 ppm ZnO, and specific ETEC F4 IgA concertation in the serum 4 days post infection, but it was not able to improve ADG and to reduce the ETEC and E. coli abundance in the faeces. Similarly, the study conducted by Bouwhuis et al. (Citation2017) showed that 500 ppm of Zn chelate with methionine was not able to reach the same ADG and faecal score as the HZnO group (2300 ppm as ZnO, pigs were not challenged). On the contrary, Oh et al. (Citation2021) found that pigs supplemented with 200 ppm of Zn chelate with glycine were able to control the incidence of diarrhoea as well as HZnO (2500 ppm, pigs were not challenged) throughout the trial and to improve the apparent total tract digestibility of dry matter and gross energy compared in the first week after weaning compared to the groups without Zn, reaching the same levels as with 2500 ppm HZnO; these latter results may indicate that a low level of Zn glycinate may also increase the activation of digestive enzymes in the small intestine and pancreatic tissue as previously suggested for HZnO (Hu et al. Citation2012). However, according to the study performed by Pieper et al. (Citation2020), ileal nutrient digestibility was not improved in the group of pigs fed with 110 ppm of Zn-Lysinate. Therefore, there are no consistent results regarding the effect of Zn-chelate on nutrient digestibility. Furthermore, in both studies by Oh et al. (Citation2021) and Pieper et al. (Citation2020), an effect of the Zn-chelate on the gut microbiota was observed, showing the ability of a low dose of Zn to interact with the commensal bacteria. Chitosan, which is a natural product obtained from partial N-deacetylation of chitin, has also been widely used to chelate Zn. According to the results obtained by Han et al. (Citation2014), the use of chitosan-Zn at a dose of 100 mg Zn/kg diet was able to reduce intestinal permeability, improve the integrity of the intestinal mucosa and alleviate the stress caused by weaning similarly to 3000 ppm of ZnO, and was more effective than ZnSO4 in inhibiting the inflammatory response. Furthermore, Oh et al. (Citation2021) showed that Zn-glycinate was able to increase the number of FoxP3+ and T-bet + T cells compared to the HZnO and had similar IL-6 values of HZnO. These results may suggest that the low dose of Zn-glycinate can limit the inflammatory response and promote the activity against viral and bacterial pathogens. Furthermore, in the study by Hu et al. (Citation2018), a decrease in the expressions of proteins associated with cell death was observed in pigs fed 150 ppm of chitosan-Zn compared to the effect of ZnSO4.
Although the majority of Zn absorption is mediated by the ZIP4 transporter, which is the major uptake pathway for dietary Zn into the organism (Brugger et al. Citation2021); chelated Zn with AAs appears to be absorbed more rapidly via peptide or AAs transporters (Nitrayova et al. Citation2012). Nevertheless, the data reported so far have shown that in the majority of the studies comparable results were obtained with ZnO, and AAs chelated Zn, suggesting that the zinc-positive effect may not be directly dependent on its transport pathway.
Overall, the lack of similarity in pig response between the different forms of chelated Zn is probably related to the different molecules to which the Zn is bound, and further investigations are needed to draw a conclusion.
Coated ZnO
Coating is a common means of drug delivery where the Zn supplements have a protective coating or film on the outer surface, and it is used to improve the chemical stability and absorption of ZnO (Luo et al. Citation2020). According to the present systematic search, 7 studies testing coated ZnO were found. Coated ZnO, with the protection of the covering layer, allows a higher percentage of ZnO to reach the gut, where ZnO can perform its primary function. Some studies had shown that ZnO coated with smectite or with lipids at a dose of 380 mg Zn/kg, can achieve the same effect as HZnO (Hu et al. Citation2013; Shen et al. Citation2014), while when used at lower doses (100 or 200 mg Zn/kg), coated ZnO did not affect growth performance (Byun et al. Citation2018).
In the studies by Lei and Kim (Citation2018) and Dong et al. (Citation2019), the diarrhoeal index was reduced in the HZnO group (2500 and 2250 ppm of ZnO, respectively; pigs were not challenged), as well as in the lipid coated group, suggesting the importance of ZnO in the gut ecosystem and in reducing the incidence of diarrhoea and that the administration of lower levels of coated ZnO could be used as an alternative to pharmacological level of ZnO. On the other hand, Byun et al. (Citation2018) found an increase in the faecal score in pigs fed with lipid-coated Zn. In addition, Jang et al. (Citation2014) and Upadhaya et al. (Citation2018) observed a significantly lower serum Zn concentration in pigs fed with coated Zn compared to 2500 ppm of ZnO. Furthermore, in the studies by Lei and Kim (Citation2018), Song et al. (Citation2018) and Dong et al. (Citation2019) the results of villus height in the duodenum of pigs fed with lipid coated Zn were not different from the results of 2250 ppm ZnO, showing that lower doses of coated ZnO could be a substitute for HZnO in improving gut morphology. Furthermore, Dong et al. (Citation2019) also observed an increase in ZO-1 and Occludin expression in HZnO and 380 ppm lipid coated zinc groups. This result suggests that dietary administration of coated ZnO, especially the lipid coating, could also prevent or even improve intestine integrity in weaned pigs.
Capsulated and microencapsulated ZnO
As mentioned above, the capsulation of ZnO could be an additional strategy to reduce the doses required in the post-weaning diet. Capsulated zinc refers to Zn supplements that are encapsulated in a shell or capsule. Increased local concentration of the substrates is one of the properties induced by molecular encapsulation (Kleij and Reek Citation2006). In general, different sources of lipids are used to encapsulate ZnO. The term microencapsulation refers to micron-sized particles an inert (which is related to the reactivity of the shell with the core material) shell (Swapan Citation2006). The comparison between the effects of capsulated and microencapsulated Zn to HZnO doses on performance, health, and gut health of post-weaning pigs is reported in Table .
Table 5. Effect of capsulated Zn on productive and health parameters in weaned pigs.
As shown by the studies by Wang et al. (Citation2012), Jang et al. (Citation2014) and Park et al. (Citation2015), the administration of 1500 ppm, 139 ppm and 100 ppm of lipid capsulated ZnO allowed to obtain final BW and G:F values analogous to HZnO (3000 ppm, 2500 ppm and 2500 ppm as ZnO, respectively). Considering diarrhoea as a response parameter, the study by Wang et al. (Citation2012) and Jang et al. (Citation2014) showed comparable diarrhoea ratios between lipid capsulated and HZnO. However, the lower dose tested by Park et al. (Citation2015) did not allow to achieve similar diarrheal score of HZnO (none of the studies included challenged animals). Nevertheless, the study by Park et al. (Citation2015) showed a Zn concentration in the liver and blood similar to the control diet (100 ppm as ZnO) and lower than HZnO groups (2500 ppm as ZnO), suggesting that the Zn concentrations are directly correlated with dietary Zn concentrations, despite the Zn source. An increase in jejunal villus height and VH:CD ratio in the capsulated Zn group was also observed by Wang et al. (Citation2012). Furthermore, ZO-1 and Occludin expression were found at the same level between HZnO and capsulated groups, suggesting a positive connection with the decreased diarrhoea ratio in animals receiving both sources of ZnO.
On the contrary, in the study by Grilli et al. (Citation2015), when lipid microencapsulated ZnO was included in the diet at a dose of 300 or 800 mg/kg, the pigs showed an increase in growth performance and an increase in ileum villus height at the same level of HZnO (3000 ppm as ZnO). Regarding the level of inflammatory cytokines, similar levels of IFN-γ and TNF-α expression were found by Grilli et al. (Citation2015) in pigs fed with 300 and 800 ppm of microencapsulated ZnO or with 3000 ppm ZnO. The explanation for these results may be the mechanism behind the action of ZnO in decreasing intestinal permeability and improving the overall health status of the pig gut.
Zn oxide nanoparticles
The term nanoparticles refers to ZnO particles smaller than 100 nanometres. A summary of the comparison between the effects of Zn nanoparticles and HZnO doses on performance, health and gut health of post-weaning pigs is reported in Table . The use of ZnO nanoparticles resulted in a similar ADG to the HZnO groups (2000 ppm; 3000 ppm; 3000 ppm; 3000 ppm; 2000 ppm; 2000 ppm; 1650 ppm; 3000 ppm as ZnO, respectively) in the studies performed by Xia et al. (Citation2017; 600 mg Zn/kg), Milani et al. (Citation2017; 15 to 60 mg Zn/kg), Wang et al. (Citation2018; 800 mg/kg), Pei et al. (Citation2019; 150–450 mg Zn/kg), Sun et al. (Citation2019; 400, 500 and 600 mg Zn/Kg), Kociova et al. (Citation2020; 500 and 1000 mg/kg phosphate nano Zn: prepared by dissolving Zn(NO3)2·6H2O in water and heating to 60 °C and followed by a second step of dissolving (NH4)2HPO4 in water, Cui et al. (Citation2021; 200 to 500 mg Zn/kg), and Szuba-Trznadel et al. (Citation2021; 150 mg Zn/kg). The clear consistency showed by these studies confirms that that doses ranging from 15 to 800 mg of nanoparticles Zn could play a role similar to that of HZnO. The authors hypothesise that these positive results of low doses of nanoparticles ZnO can be achieved thanks to the higher solubility that nano Zn can have compared to normal ZnO.
Table 6. Effect of Zn oxide nanoparticles on productive and health parameters in weaned pigs.
The occurrence of diarrhoea was not different from the high dose of HZnO with the use of nanoparticles, according to Pei et al. (Citation2019), in whose study the addition of doses from 150 to 450 ppm of nano Zn gave the same beneficial results as 3000 ppm of ZnO, and Sun et al. (Citation2019), whom found comparable results in both groups receiving either 600 ppm of nano Zn and 2000 ppm of ZnO. However, opposite results were obtained by Milani et al. (Citation2017; 15 to 60 mg Zn/kg) and Xia et al. (Citation2017; 600 mg Zn/kg), where the HZnO significantly reduced the incidence of diarrhoea compared with the use of nano Zn (none of these studies included challenged animals). However, diarrhoea can be due to different reasons, resulting to different outcomes among the studies.
It is reported that nano Zn can have a high antibacterial effect (Sun et al. Citation2019;Kociova et al. Citation2020). Indeed, Sun et al. (Citation2019) showed a similar E. coli abundance in caecum between HZnO and 400–600 ppm of nano Zn showing that nano Zn can be more effective in preventing the growth of E. coli in pigs’ gut ecosystem. Likewise, Kociova et al. (Citation2020) showed reducing coliforms abundance in the nano Zn groups when compared to the same doses of the normal ZnO. According to a recent review by Gudkov et al. (Citation2021), the mechanisms of action of ZnO nanoparticles on bacteria can be 1) disruption of the cell membrane; 2) binding to proteins and DNA, generation of reactive oxygen species (ROS); 3) disruption of the processes of bacterial DNA replication, alteration (more often, down-regulation) of expression in a wide range of genes. These modes of action can vary according to the size (increasing with smaller nano-zinc size) and production methods, which can affect the physical and morphological characteristics of nano-zinc. For more information on the antibacterial effect of nano-Zn produced by different methods, we recommend the review by Gudkov et al. (Citation2021).
Xia et al. (Citation2017) and Cui et al. (Citation2021) showed also interesting beneficial effects of nano Zn on the intestinal morphology and integrity parameters. The use of HZnO and nano ZnO resulted in higher jejunal villus height and VH:CD ratio of pigs, and the decreased in the expression of pro-inflammatory cytokines such as IFN-γ and TNF-α in both nano Zn and HZnO fed animals compared to the control group. Cui et al. (Citation2021) showed that the 200–500 ppm of nano zinc was as functional as 1600 ppm of ZnO in the increasing villus height and crypt depth in jejunum, ileum, and duodenum, leading to a potential beneficial effect in order to maintain the membrane functions, and the addition of 200 ppm of nano Zn enhanced ileal and jejunal IL-6 content, suggesting a protective immune effect given by this Zn source. These two studies indicate that low doses of nano zinc can alleviate the inflammation and promote the functionality of the gut health in the digestion and absorption. However, the expression of Occludin in jejunum in the nano Zn group was not comparable with the one obtained in the HZnO, suggesting that the addition of nano Zn could not be as effective as ZnO in maintaining gut integrity (Xia et al. Citation2017).
Furthermore, according to the study by Kociova et al. (Citation2020), nano Zn can showed a higher absorption compared to normal ZnO, as shown by the higher Zn concentration observed in the blood when comparing the same feeding doses of nano Zn and normal Zn; in fact, this higher absorption could partially explain the beneficial effect of nano Zn in gut health and growth parameters.
Indeed, as supported by the majority of these results, it can be hypothesised that the administration of ZnO in the form of nano Zn, can maintain or even increase the positive effects of this form of Zn. Indeed, the smaller molecules have the potential to increase the absorption area in the gut, especially in the small intestine, therefore exploiting the same beneficial effects of pharmacological doses of ZnO.
Porous and high surface forms of zinc oxide
Among the alternatives to HZnO, porous and high surface area of ZnO have also been investigated. In this category, one of the most investigated and available forms is a porous source of Zn source (HiZox®, Animine, France), which is a porous high surface area of ZnO with a patented manufacturing technology. The results of the effects of this form of Zn on performance, health and intestinal health parameters compared to the effects of HZnO are shown in Table . None of the studies involved challenged animals. According to Cho et al. (Citation2015; 110 ppm and 220 ppm,), Peng et al. (Citation2019; 200 ppm), and Wang et al. (Citation2019; 200 ppm and 500 ppm) the use of porous ZnO allowed to obtain ADG, ADFI and G:F ratios with similar values to those archived with HZnO (3000 ppm; 3000 ppm; 2400 ppm as ZnO, respectively). Furthermore, in the study by Morales et al. (Citation2012), higher ADG results were observed in groups receiving 110 ppm of porous ZnO when administrated to pigs in the starter phase (42 to 63 d of age). Comparable results regarding the diarrhoea parameters (diarrhoea incidence and faecal score) were observed between the porous form of Zn and HZnO doses in the studies performed by Morales et al. (Citation2012), Cho et al. (Citation2015), Vahjen et al. (Citation2016), Long et al. (Citation2017), and Wang et al. (Citation2019).
Table 7. Effect of porous form of Zn on productive and health parameters in weaned pigs.
Regarding the effect of porous ZnO on the intestinal health parameters, it can be noted that according to Long et al. (Citation2017), similar results can be obtained in jejunum and ileum crypt depth values and VH with 400 ppm. Furthermore, in the study by Peng et al. (Citation2019), lower levels of ileum and caecum E. coli ileum and caecum were found in the porous ZnO group, compared to pharmacological levels of ZnO.
More recently, a new technology combining the advantages of nano and porous Zn was tested by Su et al. (Citation2023). This new form of Zn, characterised by small particles with high porosity and a specific surface area (48.916 m2/g), was able to reduce the ETEC F4 activity at a dose of 200 ppm, and at a dose of 300 ppm had better diarrhoea-preventive effects, nutrient digestibility and integrity and functionality (increase in villus height) of the gastrointestinal tract, especially when combined with the use of probiotics.
Overall, the results collected for porous and porous Zn sources are promising and highlight these sources of Zn as a valid strategy to reduce the necessary dose to be included in the post-weaning diet.
Conclusion
The EU ban on the use of pharmacological level of ZnO is an issue of increasing interest and concern in the field of pig production. Based on the data presented, there is evidence that the effect of the Zn is dose dependent. However, the data highlighted that the form of Zn also plays a role in the efficacy of this micronutrient in promoting pig health. The solubility seems to be a key point, in fact, the higher efficacy of ZnO, characterised by a lower solubility than ZnSO4, can be explained by its role to cover the demand by a systemic effect, as well as to exert a local effect reducing the oxidative stress of the gut mucosa and strengthening the recovery of the gut integrity. The present data confirm that with the dose of Zn allowed in the EU (150 ppm) is almost impossible to observe the same effect obtained with a high ZnO dose, regardless the source used. Therefore, a possible strategy to replace the high ZnO dose should be the use of a form of Zn that is able to cover the requirements, to sustain the physiology of the pigs as well as to have a local effect on the gut mucosa.
Acknowledgements
The authors thank Professor Paolo Bosi for his support in the making of this article. The authors also thank Animine (France) for the support of the PhD scholarship, and Stephane Durosoy, Agathe Romeo and Alessandra Monteiro for their critical review of the manuscript.
Disclosure statement
The authors declare that the study was conducted in the absence of any commercial or financial relationships which could be construed as a potential conflict of interest.
Data availability statement
The data analysed in this study are available from the corresponding author under reasonable request.
Additional information
Funding
References
- Adewole DI, Kim IH, Nyachoti CM. 2016. Gut health of pigs: challenge models and response criteria with a critical analysis of the effectiveness of selected feed additives—a review. Asian-Australas J Anim Sci. 29(7):909–924. doi:10.5713/ajas.15.0795.
- Alam AN, Sarker SA, Wahed MA, Khatun M, Rahaman MM. 1994. Enteric protein loss and intestinal permeability changes in children during acute shigellosis and after recovery: effect of zinc supplementation. Gut. 35(12):1707–1711. doi:10.1136/gut.35.12.1707.
- Al-Sadi R, Boivin M, Ma T. 2009. Mechanism of cytokine modulation of epithelial tight junction barrier. Front Biosci (Landmark Ed). 14(7):2765–2778. doi:10.2741/3413.
- Ao T, Pierce JL, Power R, Pescatore AJ, Cantor AH, Dawson KA, Ford MJ. 2009. Effects of feeding different forms of zinc and copper on the performance and tissue mineral content of chicks. Poult Sci. 88(10):2171–2175. doi:10.3382/ps.2009-00117.
- Blaabjerg K, Poulsen HD. 2017. The use of zinc and copper in pig production. DCA – Nationalt Center for Jordbrug Og Fødevarer. 23:1–17. https://pure.au.dk/ws/files/116535883/2017_05_01_Pigs_zinc_and_copper_review.pdf
- Bonetti A, Tugnoli B, Piva A, Grilli E. 2021. Towards zero zinc oxide: feeding strategies to manage post-weaning diarrhea in pigs. Animals. 11(3):642. doi:10.3390/ani11030642.
- Bosi P, Merialdi G, Sarli G, Casini L, Gremokolini C, Preziosi R, Brunetti B, Trevisi P. 2003. Effects of doses of ZnO or Zn-Glutamate on growth performance, gut characteristics, health and immunity of early-weaned pigs orally challenged with E. coli K88. Ital J Anim Sci. 2:361–363. doi:10.4081/ijas.2003.11676011.
- Bouwhuis MA, Sweeney T, Mukhopadhya A, Thornton K, McAlpine PO, O'Doherty JV. 2017. Zinc methionine and laminarin have growth-enhancing properties in newly weaned pigs influencing both intestinal health and diarrhoea occurrence. J Anim Physiol Anim Nutr (Berl). 101(6):1273–1285., doi:10.1111/jpn.12647.
- Broom LJ, Miller HM, Kerr KG, Knapp JS. 2006. Effects of zinc oxide and Enterococcus faecium SF68 dietary supplementation on the performance, intestinal microbiota and immune status of weaned pigs. Res Vet Sci. 80(1):45–54. doi:10.1016/j.rvsc.2005.04.004.
- Brugger D, Hanauer M, Ortner J, Windisch WM. 2021. The response of zinc transporter gene expression of selected tissues in a pig model of subclinical zinc deficiency. J Nutr Biochem. 90:108576. doi:10.1016/j.jnutbio.2020.108576.
- Buff CE, Bollinger DW, Ellersieck MR, Brommelsiek WA, Veum TL. 2005. Comparison of growth performance and zinc absorption, retention, and excretion in weanling pigs fed diets supplemented with zinc-polysaccharide or zinc oxide. J Anim Sci. 83(10):2380–2386. doi:10.2527/2005.83102380x.
- Byun Y-J, Lee CY, Kim MH, Jung DY, Han JH, Jang I, Song YM, Park B-C. 2018. Effects of dietary supplementation of a lipid-coated zinc oxide product on the fecal consistency, growth, and morphology of the intestinal mucosa of weanling pigs. JAST. 59:29. doi:10.1186/s40781-017-0159-z.
- Campbell JM, Crenshaw JD, Polo J. 2013. The biological stress of early weaned pigs. J. Anim. Sci. Biotechnol. 4:19. doi:10.1186/2049-1891-4-19.
- Carlson MS, Hill GM, Link JE. 1999. Early- and traditionally weaned nursery pigs benefit from phase-feeding pharmacological concentrations of zinc oxide: effect on metallothionein and mineral concentrations. J Anim Sci. 77(5):1199–1207. doi:10.2527/1999.7751199x.
- Carlson D, Poulsen HD, Sehested J. 2004. Influence of weaning and effect of post weaning dietary zinc and copper on electrophysiological response to glucose, theophylline and 5-HT in pig small intestinal mucosa. Comp Biochem Physiol A Mol Integr Physiol. 137(4):757–765. doi:10.1016/j.cbpb.2004.02.011.
- Carlson D, Poulsen HD, Vestergaard M. 2004. Additional dietary zinc for weaning pigs is associated with elevated concentrations of serum IGF-I. J Anim Physiol Anim Nutr (Berl). 88(9-10):332–339. doi:10.1111/j.1439-0396.2004.00488.x.
- Case CL, Carlson MS. 2002. Effect of feeding organic and inorganic sources of additional zinc on growth performance and zinc balance in nursery pigs. J Anim Sci. 80(7):1917–1924. doi:10.2527/2002.8071917x.
- Castillo M, Martín-Orúe SM, Nofrarías M, Manzanilla EG, Gasa J. 2007. Changes in caecal microbiota and mucosal morphology of weaned pigs. Vet Microbiol. 124(3-4):239–247. doi:10.1016/j.vetmic.2007.04.026.
- Chai W, Zakrzewski SS, Günzel D, Pieper R, Wang Z, Twardziok S, Janczyk P, Osterrieder N, Burwinkel M. 2014. High-dose dietary zinc oxide mitigates infection with transmissible gastroenteritis virus in pigs. BMC Vet Res. 10(1):75. doi:10.1186/1746-6148-10-75.
- Cho JH, Upadhaya SD, Kim IH. 2015. Effects of dietary supplementation of modified zinc oxide on growth performance, nutrient digestibility, blood profiles, fecal microbial shedding and fecal score in weanling pigs. Anim Sci J. 86(6):617–623. doi:10.1111/asj.12329.
- Cui Y, Tian Z, Lu H, Deng D, Liu Z, Rong T, Yu M, Ma X. 2021. Zinc oxide nanoparticles improve gut health and reduce faecal zinc excretion in pigs. Livest. Sci. 251:104610. doi:10.1016/j.livsci.2021.104610.
- Diao H, Yan J, Li S, Kuang S, Wei X, Zhou M, Zhang J, Huang C, He P, Tang W. 2021. Effects of dietary zinc sources on growth performance and gut health of weaned pigs. Front Microbiol. 12:771617. doi:10.3389/fmicb.2021.771617.
- Dong X, Xu Q, Wang C, Zou X, Lu J. 2019. Supplemental-coated zinc oxide relieves diarrhoea by decreasing intestinal permeability in weanling pigs. J. Appl. Anim. Res. 47(1):362–368. doi:10.1080/09712119.2019.1645673.
- Fairbrother JM, Nadeau É, Gyles CL. 2005. Escherichia coli in postweaning diarrhea in pigs: an update on bacterial types, pathogenesis, and prevention strategies. Anim Health Res Rev. 6(1):17–39. doi:10.1079/ahr2005105.
- Feng J, Ma WQ, Niu HH, Wu XM, Wang Y, Feng J. 2010. Effects of zinc glycine chelate on growth, hematological, and immunological characteristics in broilers. Biol Trace Elem Res. 133(2):203–211. doi:10.1007/s12011-009-8431-9.
- Grilli E, Tugnoli B, Vitari F, Domeneghini C, Morlacchini M, Piva A, Prandini A. 2015. Low doses of microencapsulated zinc oxide improve performance and modulate the ileum architecture, inflammatory cytokines and tight junctions expression of weaned pigs. Animal. 9(11):1760–1768. doi:10.1017/S1751731115001329.
- Gudkov SV, Burmistrov DE, Serov DA, Rebezov MB, Semenova AA, Lisitsyn AB. 2021. A Mini Review of Antibacterial Properties of ZnO Nanoparticles. Frontiers in Physics. 9:641481. doi:10.3389/fphy.2021.641481.
- Han X-Y, Ma Y-F, Lv M-Y, Wu Z-P, Qian L-C. 2014. Chitosan-zinc chelate improves intestinal structure and mucosal function and decreases apoptosis in ileal mucosal epithelial cells in weaned pigs. Br J Nutr. 111(8):1405–1411. doi:10.1017/S0007114513004042.
- Han JH, Song MH, Kim HN, Jang I, Lee CY, Park B-C. 2018. Effects of the lipid-coated zinc oxide dietary supplement on intestinal mucosal morphology and gene expression associated with the gut health in weanling pigs challenged with enterotoxigenic Escherichia coli K88. Can J Anim Sci. 98(3):538–547. doi:10.1139/cjas-2017-0127.
- Hansen SV, Nørskov NP, Nørgaard JV, Woyengo TA, Poulsen HD, Nielsen TS. 2022. Determination of the optimal level of dietary zinc for newly weaned pigs: a dose-response study. Animals. 12(12):1552. doi:10.3390/ani12121552.
- Heo JM, Kim JC, Hansen CF, Mullan BP, Hampson DJ, Maribo H, Kjeldsen N, Pluske JR. 2010. Effects of dietary protein level and zinc oxide supplementation on the incidence of post-weaning diarrhoea in weaner pigs challenged with an enterotoxigenic strain of Escherichia coli. Livest Sci. 133(1-3):210–213. doi:10.1016/j.livsci.2010.06.066.
- Heo JM, Opapeju FO, Pluske JR, Kim JC, Hampson DJ, Nyachoti CM. 2013. Gastrointestinal health and function in weaned pigs: a review of feeding strategies to control post‐weaning diarrhoea without using in‐feed antimicrobial compounds. J Anim Physiol Anim Nutr. 97(2):207–237. doi:10.1111/j.1439-0396.2012.01284.x.
- Hill GM, Mahan DC, Carter SD, Cromwell GL, Ewan RC, Harrold RL, Lewis AJ, Miller PS, Shurson GC, Veum TL, NCR-42 Committee on Swine Nutrition. 2001. Effect of pharmacological concentrations of zinc oxide with or without the inclusion of an antibacterial agent on nursery pig performance. J Anim Sci. 79(4):934–941. doi:10.2527/2001.794934x.
- Hortin AE, Bechtel PJ, Baker DH. 1991. Efficacy of pork loin as a source of zinc and effect of added cysteine on zinc bioavailability. J. Food Sci. 56(6):1505–1507. doi:10.1111/j.1365-2621.1991.tb08626.x.
- Hu C, You Z, Zhu K, Luan Z. 2012. Effects of nano zinc oxide on growth performance and intestinal mucosal barrier in weaner pigs. Chin J Anim Nutr. 24:285–290.
- Hu C, Song J, Li Y, Luan Z, Zhu K. 2013. Diosmectite-zinc oxide composite improves intestinal barrier function, modulates expression of pro-inflammatory cytokines and tight junction protein in early weaned pigs. Br J Nutr. 110(4):681–688. doi:10.1017/S0007114512005508.
- Hu Y, Dun Y, Li S, Zhao S, Peng N, Liang Y. 2014. Effects of Bacillus subtilis KN-42 on growth performance, diarrhea and faecal bacterial flora of weaned pigs. Asian Australas J Anim Sci. 27(8):1131–1140. doi:10.5713/ajas.2013.13737.
- Hu L, Cheng S, Li Y, Geng S, Ma Y, Han X. 2018. Chitosan-Zn chelate downregulates TLR4-NF-κB signal pathway of inflammatory response and cell death-associated proteins compared to inorganic zinc. Biol Trace Elem Res. 184(1):92–98. doi:10.1007/s12011-017-1174-0.
- Huang YL, Lu L, Luo XG, Liu B. 2007. An optimal dietary zinc level of broiler chicks fed a corn-soybean meal diet. Poult Sci. 86(12):2582–2589. doi:10.3382/ps.2007-00088.
- Hudson BP, Dozier WA, Wilson JL. 2005. Broiler live performance response to dietary zinc source and the influence of zinc supplementation in broiler breeder diets. Anim. Feed Sci. Technol. 118(3-4):329–335. doi:10.1016/j.anifeedsci.2004.10.018.
- Janczyk P, Kreuzer S, Assmus J, Nöckler K, Brockmann GA. 2013. No protective effects of high-dosage dietary zinc oxide on weaned pigs infected with Salmonella enterica Serovar Typhimurium DT104. Appl Environ Microbiol. 79(9):2914–2921. doi:10.1128/AEM.03577-12.
- Jang I, Kwon CH, Ha DM, Jung DY, Kang SY, Park MJ, Han JH, Park B-C, Lee CY. 2014. Effects of a lipid-encapsulated zinc oxide supplement on growth performance and intestinal morphology and digestive enzyme activities in weanling pigs. J Anim Sci Technol. 56(1):29. doi:10.1186/2055-0391-56-29.
- Jensen-Waern M, Melin L, Lindberg R, Johannisson A, Petersson L, Wallgren P. 1998. Dietary zinc oxide in weaned pigs—effects on performance, tissue concentrations, morphology, neutrophil functions and faecal microflora. Res Vet Sci. 64(3):225–231. doi:10.1016/S0034-5288(98)90130-8.
- Kleij AW, Reek JNH. 2006. Ligand-Template Directed Assembly: an efficient approach for the the supramolecular encapsulation of transition-metal catalysts. Chemistry. 12(16):4218–4227. doi:10.1002/chem.200500875.
- Kociova S, Dolezelikova K, Horky P, Skalickova S, Baholet D, Bozdechova L, Vaclavkova E, Belkova J, Nevrkla P, Skladanka J, et al. 2020. Zinc phosphate-based nanoparticles as alternatives to zinc oxide in diet of weaned pigs. J Animal Sci Biotechnol. 11(1):59. doi:10.1186/s40104-020-00458-x.
- Kwon C-H, Lee CY, Han S-J, Kim S-J, Park B-C, Jang I, Han J-H. 2014. Effects of dietary supplementation of lipid-encapsulated zinc oxide on colibacillosis, growth and intestinal morphology in weaned pigs challenged with enterotoxigenic Escherichia coli. Anim Sci J. 85(8):805–813. doi:10.1111/asj.12215.
- Lei XJ, Kim IH. 2018. Low dose of coated zinc oxide is as effective as pharmacological zinc oxide in promoting growth performance, reducing fecal scores, and improving nutrient digestibility and intestinal morphology in weaned pigs. Anim Feed Sci Technol. 245:117–125. doi:10.1016/j.anifeedsci.2018.06.011.
- Lei XJ, Kim IH. 2020. Evaluation of coated zinc oxide in young pigs challenged with enterotoxigenic Escherichia coli K88. Anim Feed Sci Technol. 262:114399. doi:10.1016/j.anifeedsci.2020.114399.
- Lei XJ, Liu ZZ, Park JH, Kim IH. 2022. Novel zinc sources as antimicrobial growth promoters for monogastric animals: a review. J Anim Sci Technol. 64(2):187–196. doi:10.5187/jast.2022.e1.
- Li X, Yin J, Li D, Chen X, Zang J, Zhou X. 2006. Dietary supplementation with zinc oxide increases IGF-I and IGF-I receptor gene expression in the small intestine of weanling pigs. J Nutr. 136(7):1786–1791. doi:10.1093/jn/136.7.1786.
- Liu X, Ma Y, Chen L, Yu X, Feng J. 2020. Effects of different zinc sources on growth performance, antioxidant capacity and zinc storage of weaned pigs. Livest. Sci. 241:104181. doi:10.1016/j.livsci.2020.104181.
- Long L, Chen J, Zhang Y, Liang X, Ni H, Zhang B, Yin Y. 2017. Comparison of porous and nano zinc oxide for replacing high-dose dietary regular zinc oxide in weaning pigs. PLoS One. 12(8):e0182550. doi:10.1371/journal.pone.0182550.
- Looft T, Johnson TA, Allen HK, Bayles DO, Alt DP, Stedtfeld RD, Sul WJ, Stedtfeld TM, Chai B, Cole JR, et al. 2012. In-feed antibiotic effects on the swine intestinal microbiome. Proc Natl Acad Sci U S A. 109(5):1691–1696. doi:10.1073/pnas.1120238109.
- Luise D, Lauridsen C, Bosi P, Trevisi P. 2019. Methodology and application of Escherichia coli F4 and F18 encoding infection models in post-weaning pigs. J Animal Sci Biotechnol. 10(1):53. doi:10.1186/s40104-019-0352-7.
- Luise D, Chalvon-Demersay T, Lambert W, Bosi P, Trevisi P. 2021. Meta-analysis to evaluate the impact of the reduction of dietary crude protein on the gut health of post-weaning pigs. Ital. J. Anim. Sci. 20(1):1386–1397. doi:10.1080/1828051X.2021.1952911.
- Luo F, Wang M, Huang L, Wu Z, Wang W, Zafar A, Tian Y, Hasan M, Shu X. 2020. Synthesis of zinc oxide eudragit FS30D nanohybrids: structure, characterization, and their application as an intestinal drug delivery system. ACS Omega. 5(20):11799–11808. doi:10.1021/acsomega.0c01216.
- Mavromichalis I, Peter CM, Parr TM, Ganessunker D, Baker DH. 2000. Growth-promoting efficacy in young pigs of two sources of zinc oxide having either a high or a low bioavailability of zinc. J Anim Sci. 78(11):2896–2902. doi:10.2527/2000.78112896x.
- Mazzoni M, Merialdi G, Sarli G, Trevisi P, Bosi P. 2010. Effect of two doses of different zinc sources (Inorganic vs. Chelated form) on the epithelial proliferative activity and the apoptotic index of intestinal mucosa of early-weaned pigs orally challenged with E. coli K88. Asian Australas J Anim Sci. 23(6):777–785. doi:10.5713/ajas.2010.90352.
- McDonald P. 2002. Anim Nutr. 6th edn. Edinburgh, Great Britain: Pearson Educational Limited.
- Meyer TA, Lindemann MD, Cromwell GL, Monegue HJ, Inocencio N. 2002. Effects of pharmacological levels of zinc as zinc oxide on fecal zinc and mineral excretion in weanling pigs11this manuscript is based on research supported in part by the kentucky agricultural experiment station and is published by the kentucky agricultural experiment station as paper no. 01-07-177. Prof. Anim. Sci. 18(2):162–168. doi:10.15232/S1080-7446(15)31506-0.
- Milani NC, Sbardella M, Ikeda NY, Arno A, Mascarenhas BC, Miyada VS. 2017. Dietary zinc oxide nanoparticles as growth promoter for weanling pigs. Anim. Feed Sci. Technol. 227:13–23. doi:10.1016/j.anifeedsci.2017.03.001.
- Morales J, Cordero G, Piñeiro C, Durosoy S. 2012. Zinc oxide at low supplementation level improves productive performance and health status of pigs. J Anim Sci. 90 Suppl 4(suppl_4):436–438. doi:10.2527/jas.53833.
- Nitrayova S, Windisch W, von Heimendahl E, Müller A, Bartelt J. 2012. Bioavailability of zinc from different sources in pigs. J Anim Sci. 90 (suppl_4):185–187. doi:10.2527/jas.53895.
- [NRC] National Research Council. 2012. Nutrient Requirements of Swine. 11th ed. Washington, DC: National Academies Press.
- Oh SM, Kim MJ, Hosseindoust A, Kim KY, Choi YH, Ham HB, Hwang SJ, Lee JH, Cho HJ, Kang WS, et al. 2020. Hot melt extruded-based nano zinc as an alternative to the pharmacological dose of ZnO in weanling pigs. Asian Australas J Anim Sci. 33(6):992–1001. doi:10.5713/ajas.19.0140.
- Oh H-J, Park Y-J, Cho JH, Song M-H, Gu B-H, Yun W, Lee J-H, An J-S, Kim Y-J, Lee J-S, et al. 2021. Changes in diarrhea score, nutrient digestibility, zinc utilization, intestinal immune profiles, and fecal microbiome in weaned pigs by different forms of zinc. Animals. 11(5):1356. doi:10.3390/ani11051356.
- Park BC, Jung DY, Kang SY, Ko YH, Ha DM, Kwon CH, Park MJ, Han JH, Jang I, Lee CY. 2015. Effects of dietary supplementation of a zinc oxide product encapsulated with lipid on growth performance, intestinal morphology, and digestive enzyme activities in weanling pigs. Anim Feed Sci Technol. 200:112–117. doi:10.1016/j.anifeedsci.2014.11.016.
- Pearce SC, Sanz Fernandez M-V, Torrison J, Wilson ME, Baumgard LH, Gabler NK. 2015. Dietary organic zinc attenuates heat stress–induced changes in pig intestinal integrity and metabolism. Anim Sci J. 93(10):4702–4713. doi:10.2527/jas.2015-9018.
- Pei X, Xiao Z, Liu L, Wang G, Tao W, Wang M, Zou J, Leng D. 2019. Effects of dietary zinc oxide nanoparticles supplementation on growth performance, zinc status, intestinal morphology, microflora population, and immune response in weaned pigs. J Sci Food Agric. 99(3):1366–1374. doi:10.1002/jsfa.9312.
- Peng P, Chen J, Yao K, Yin Y, Long L, Fang R. 2019. The effects of dietary supplementation with porous zinc oxide on growth performance, intestinal microbiota, morphology, and permeability in weaned pigs. Anim Sci J. 90(9):1220–1228. doi:10.1111/asj.13228.
- Pié S, Lallès JP, Blazy F, Laffitte J, Sève B, Oswald IP. 2004. Weaning is associated with an upregulation of expression of inflammatory cytokines in the intestine of pigs. J Nutr. 134(3):641–647. doi:10.1093/jn/134.3.641.
- Pieper R, Dadi TH, Pieper L, Vahjen W, Franke A, Reinert K, Zentek J. 2020. Concentration and chemical form of dietary zinc shape the porcine colon microbiome, its functional capacity and antibiotic resistance gene repertoire. Isme J. 14(11):2783–2793. doi:10.1038/s41396-020-0730-3.
- Revy PS, Jondreville C, Dourmad JY, Nys Y. 2006. Assessment of dietary zinc requirement of weaned pigs fed diets with or without microbial phytase. J Anim Physiol Anim Nutr. 90(1-2):50–59. doi:10.1111/j.1439-0396.2005.00576.x.
- Richards J, Zhao J, Harrell R, Atwell C, Dibner J. 2010. Trace mineral nutrition in poultry and swine. Asian Australas J Anim Sci. 23(11):1527–1534. doi:10.5713/ajas.2010.r.07.
- Roselli M, Finamore A, Garaguso I, Britti MS, Mengheri E. 2003. Zinc oxide protects cultured enterocytes from the damage induced by Escherichia coli. J Nutr. 133(12):4077–4082. doi:10.1093/jn/133.12.4077.
- Sales J. 2013. Effects of pharmacological concentrations of dietary zinc oxide on growth of post-weaning pigs: a meta-analysis. Biol Trace Elem Res. 152(3):343–349. doi:10.1007/s12011-013-9638-3.
- Schell TC, Kornegay ET. 1996. Zinc concentration in tissues and performance of weanling pigs fed pharmacological levels of zinc from ZnO, Zn-methionine, Zn-lysine, or ZnSO4. J Anim Sci. 74(7):1584–1593. doi:10.2527/1996.7471584x.
- Schroder K, Hertzog PJ, Ravasi T, Hume DA. 2004. Interferon-gamma: an overview of signals, mechanisms and functions. J Leukoc Biol. 75(2):163–189. doi:10.1189/jlb.0603252.
- Schwarz S, Chaslus-Dancla E. 2001. Use of antimicrobials in veterinary medicine and mechanisms of resistance. Vet Res. 32(3/4):201–225. doi:10.1051/vetres:2001120.
- Shen J, Chen Y, Wang Z, Zhou A, He M, Mao L, Zou H, Peng Q, Xue B, Wang L, et al. 2014. Coated zinc oxide improves intestinal immunity function and regulates microbiota composition in weaned pigs. Br J Nutr. 111(12):2123–2134. doi:10.1017/S0007114514000300.
- Shi H, Upadhaya SD, Kim IH. 2019. Comparative effects of low zinc oxide dose with or without probiotics relative to high zinc oxide dose on the performance, nutrient digestibility, blood metabolites, and noxious gases emission in weaned pigs. Can J Anim Sci. 99(4):881–889. doi:10.1139/cjas-2019-0059.
- Siddiqi, Khwaja Salahuddin, ur Rahman, Aziz, Husen, Azamal, Tajuddin, 2018. Properties of zinc oxide nanoparticles and their activity against microbes.Nanoscale Res Lett, 13:141. doi:10.1186/s11671-018-2532-3.
- Sloup V, Jankovská I, Nechybová S, Peřinková P, Langrova I. 2017. Zinc in the animal organism: a review. Sci Agric Bohem. doi:10.1515/sab-2017-0003.
- Söderberg TA, Sunzel B, Holm S, Elmros T, Hallmans G, Sjöberg S. 1990. Antibacterial effect of zinc oxide in vitro. Scand J Plast Reconstr Surg Hand Surg. 24(3):193–197. doi:10.3109/02844319009041278.
- Song ZH, Xiao K, Ke YL, Jiao LF, Hu CH. 2015. Zinc oxide influences mitogen-activated protein kinase and TGF-β1 signaling pathways, and enhances intestinal barrier integrity in weaned pigs. Innate Immun. 21(4):341–348. doi:10.1177/1753425914536450.
- Song YM, Kim MH, Kim HN, Jang I, Han JH, Fontamillas GA, Lee CY, Park B-C. 2018. Effects of dietary supplementation of lipid-coated zinc oxide on intestinal mucosal morphology and expression of the genes associated with growth and immune function in weanling pigs. Asian-Australas J Anim Sci. 31(3):403–409. doi:10.5713/ajas.17.0718.
- Stensland I, Kim JC, Bowring B, Collins AM, Mansfield JP, Pluske JR. 2015. A Comparison of diets supplemented with a feed additive containing organic acids, cinnamaldehyde and a permeabilizing complex, or zinc oxide, on post-weaning diarrhoea, selected bacterial populations, blood measures and performance in weaned pigs experimentally infected with enterotoxigenic E. coli. Animals. 5:1147–1168. doi:10.3390/ani5040403.
- Su W, Li Z, Gong T, Wang F, Jin M, Wang Y, Lu Z. 2023. An alternative ZnO with large specific surface area: preparation, physicochemical characterization and effects on growth performance, diarrhea, zinc metabolism and gut barrier function of weaning pigs. Sci Total Environ. 882:163558. doi:10.1016/j.scitotenv.2023.163558.
- Sun YB, Xia T, Wu H, Zhang WJ, Zhu YH, Xue JX, He DT, Zhang LY. 2019. Effects of nano zinc oxide as an alternative to pharmacological dose of zinc oxide on growth performance, diarrhea, immune responses, and intestinal microflora profile in weaned pigs. Anim. Feed Sci. Technol. 258:114312. 114312. doi:10.1016/j.anifeedsci.2019.114312.
- Swapan KG. 2006. Functional coatings. 1st ed. John Wiley & Sons, Ltd. doi:10.1002/3527608478.
- Swinkels JW, Kornegay ET, Zhou W, Lindemann MD, Webb KE, Verstegen MW. 1996. Effectiveness of a zinc amino acid chelate and zinc sulfate in restoring serum and soft tissue zinc concentrations when fed to zinc-depleted pigs. J Anim Sci. 74(10):2420–2430. doi:10.2527/1996.74102420x.
- Szuba-Trznadel A, Rząsa A, Hikawczuk T, Fuchs B. 2021. Effect of zinc source and level on growth performance and zinc status of weaned pigs. Animals. 11(7):2030. doi:10.3390/ani11072030.
- Tajik N, Frech M, Schulz O, Schälter F, Lucas S, Azizov V, Dürholz K, Steffen F, Omata Y, Rings A, et al. 2020. Targeting zonulin and intestinal epithelial barrier function to prevent onset of arthritis. Nat Commun. 11(1):1995. doi:10.1038/s41467-020-15831-7.
- Upadhaya SD, Kim YM, Lee KY, Kim IH. 2018. Use of protected zinc oxide in lower doses in weaned pigs in substitution for the conventional high dose zinc oxide. Anim. Feed Sci. Technol. 240:1–10. doi:10.1016/j.anifeedsci.2018.03.012.
- Vahjen W, Roméo A, Zentek J. 2016. Impact of zinc oxide on the immediate postweaning colonization of enterobacteria in pigs1. Anim. Sci. J. 94(suppl_3):359–363. doi:10.2527/jas.2015-9795.
- Villagómez-Estrada S, Pérez JF, Darwich L, Vidal A, van Kuijk S, Melo-Durán D, Solà-Oriol D. 2020. Effects of copper and zinc sources and inclusion levels of copper on weanling pig performance and intestinal microbiota. Anim Sci J. 98(5):skaa117. doi:10.1093/jas/skaa117.
- Wang C, Xie P, Liu LL, Dong XY, Lu JJ, Zou XT. 2012. Use of lower level of capsulated zinc oxide as an alternative to pharmacological dose of zinc oxide for weaned pigs. Asian J Anim Veter Adv. 7(12):1290–1300. doi:10.3923/ajava.2012.1290.1300.
- Wang C, Zhang L, Ying Z, He J, Zhou L, Zhang L, Zhong X, Wang T. 2018. Effects of dietary zinc oxide nanoparticles on growth, diarrhea, mineral deposition, intestinal morphology, and barrier of weaned pigs. Biol Trace Elem Res. 185(2):364–374. doi:10.1007/s12011-018-1266-5.
- Wang W, Van Noten N, Degroote J, Romeo A, Vermeir P, Michiels J. 2019. Effect of zinc oxide sources and dosages on gut microbiota and integrity of weaned pigs. Animal Physiology Nutrition. 103(1):231–241. doi:10.1111/jpn.12999.
- Wapnir RA, Stiel L. 1986. Zinc intestinal absorption in rats: specificity of amino acids as ligands. J Nutr. 116(11):2171–2179. doi:10.1093/jn/116.11.2171.
- Wedekind KJ, Hortin AE, Baker DH. 1992. Methodology for assessing zinc bioavailability: efficacy estimates for zinc-methionine, zinc sulfate, and zinc oxide. Anim. Sci. J. 70(1):178–187. doi:10.2527/1992.701178x.
- Xia T, Lai W, Han M, Han M, Ma X, Zhang L. 2017. Dietary ZnO nanoparticles alters intestinal microbiota and inflammation response in weaned pigs. Oncotarget. 8(39):64878–64891. doi:10.18632/oncotarget.17612.
- Yin J, Li X, Li D, Yue T, Fang Q, Ni J, Zhou X, Wu G. 2009. Dietary supplementation with zinc oxide stimulates ghrelin secretion from the stomach of young pigs. J Nutr Biochem. 20(10):783–790. doi:10.1016/j.jnutbio.2008.07.007.
- Zhang B, Guo Y. 2007. Beneficial effects of tetrabasic zinc chloride for weanling pigs and the bioavailability of zinc in tetrabasic form relative to ZnO. Anim Feed Sci Technol. 135(1-2):75–85. doi:10.1016/j.anifeedsci.2006.06.006.
- Zhang G, Xia T, Zhao J, Liu L, He P, Zhang S, Zhang L. 2019. Moderate tetrabasic zinc chloride supplementation improves growth performance and reduces diarrhea incidence in weaned pigs. Asian Australas J Anim Sci. 33(2):264–276. doi:10.5713/ajas.18.0914.