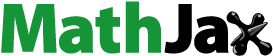
Abstract
Previous studies on probiotics in chickens have shown varying efficacy, and the use of phage as a supplement for improving chicken performance and health has gained interest. However, the combination of these supplements has not been thoroughly investigated. This study aimed to assess the effects of an Escherichia coli phage cocktail, both alone and in combination with probiotics, on specific gut populations, lipid profiles, heterophil-to-lymphocyte ratio, serum immunoglobulins, villus height to crypt depth ratio, and relative organ weights in chickens at day 21 and 35. The experiment followed a completely randomised design with factorial arrangements, consisting of three levels of phage cocktail dosage (0 g/kg, 1 g/kg, and 2 g/kg) and two levels of probiotics dosage (0 g/kg and 1 g/kg), with a total of 288 one-day-old male Cobb 500 broilers. Our study demonstrated that supplementing chicken diets with 1 g/kg phage cocktail and 1 g/kg probiotics resulted in significant (p < 0.05) improvements in growth performance and reduced Clostridium perfringens populations. It also reduced triglyceride levels and did not cause physiological stress, as indicated by the heterophil-to-lymphocyte ratio. Overall, this study provides important insights into the potential benefits of combining phages and probiotics in broiler chicken diets, which have not been extensively studied.
HIGHLIGHTS
Incorporating 1 g/kg of phage cocktail and 1 g/kg of probiotics significantly enhances chicken growth performance and decreases populations of harmful bacteria.
This dietary supplementation reduces blood lipid levels in chickens without causing physiological stress.
The study highlights the promising advantages of combining phages and probiotics in poultry diets, suggesting a novel approach to improve chicken health and productivity.
Keywords:
Introduction
The gut microbiota plays an important role in the maintenance of health in chickens. Several studies have documented that healthy gut boosts the host’s growth performance and immune health (Stanley et al. Citation2012; Wei et al. Citation2013; Sugiharto Citation2016; Lee et al. Citation2023). Indirectly, this has been achieved with the use of antibiotic growth promoters (AGPs) in poultry farming. However, AGPs have been progressively banned in certain parts of the world due to the rise of antibiotic-resistant bacteria (Dahiya et al. Citation2006; Wen et al. Citation2022). An alternative to AGPs is direly needed, and probiotics are one option that has been extensively investigated over the past few decades (Pan and Yu Citation2014; Park et al. Citation2016; Tran et al. Citation2023).
Probiotics, living microorganisms which may confer beneficial effects to the host when administered in a sufficient amount (De Vuyst et al. Citation2004; Kabir Citation2009). Bifidobacterium and Lactobacillus are among the most common bacterial genera used as probiotics. The positive effects of probiotics on the host have been reported based on various modes of action, such as reducing pathogen colonisation (Mazanko et al. Citation2022), improving host metabolism (Palamidi et al. Citation2016; Zhang et al. Citation2016; Yousaf et al. Citation2022), reducing harmful bacterial enzyme activity and ammonia production (Jin et al. Citation2000; Ghadban Citation2002; Yousaf et al. Citation2022), and improving the immune system (Mountzouris et al. Citation2010; Smith Citation2014; Yousaf et al. Citation2022). Because of this, various beneficial effects in poultry have been reported such as an increase in intestinal villi height (Samanya and Yamauchi Citation2002; Chichlowski et al. Citation2007; Mazanko et al. Citation2022), and a reduction of blood cholesterol (Panda et al. Citation2006; Toghyani et al. Citation2015; Pourakbari et al. Citation2016; Yousaf et al. Citation2022). However, various studies have also reported the variation in probiotics efficacy which has limited its application in poultry (Khosravi et al. Citation2010; Ashayerizadeh et al. Citation2011; Olnood et al. Citation2015; Oladokun et al. Citation2021). This could be due to the survivability of probiotics alongside colonising gut microbes (Mead Citation2005; O'Toole and Cooney Citation2008; Bermudez-Brito et al. Citation2012; Buahom et al. Citation2023). While phages have traditionally been employed for therapeutic purposes, they may potentially play a role in enhancing probiotic colonisation within the gut (Porter et al. Citation2022; Shaufi et al. Citation2023). By providing additional adhesion sites for probiotics to attach, phages could contribute to improved chicken growth performance and overall health.
Phages are bacteria-infecting viruses which multiply in a specific bacterial host and eventually lyse them (Loc-Carrillo and Abedon Citation2011). They are ubiquitous and can be isolated easily in the presence of host bacteria. Phage can be classified into two categories: virulent and temperate (Gill and Hyman Citation2010). The former type only divides through the lytic cycle, while the latter can multiply through both lysogenic and lytic cycles. In lytic cycle, phage infected host bacteria are immediately killed at the end of the cycle (Du Toit Citation2022; Petrovic Fabijan et al. Citation2023; Roughgarden Citation2023). The virulent phage which only follows lytic cycle is the most important type of phage that is used for phage therapy and modulation purposes. The use of phages has been proposed as a viable and sustainable solution to combat pathogens responsible for diseases and antibiotic resistance. Phages are known for their specificity, safety, and efficacy, and their application has the potential to enhance both poultry health and productivity (Upadhaya et al. Citation2021; Shaufi et al. Citation2023).
Félix d‘Herelle pioneered the first application of phage therapy in chickens in 1917 (d‘Herelle Citation1961). He successfully isolated and characterised a bacteriophage to combat fowl typhoid, a disease caused by Salmonella gallinarum in chickens. This groundbreaking work established the foundation for the use of phage therapy in controlling diseases caused by pathogens, particularly in poultry. Previous studies on phages were mainly reported for therapeutic purposes, where they were investigated for treating diseases of chickens challenged with pathogens of Escherichia coli, Salmonella spp., Campylobacter spp. and Clostridium perfringens (Huff et al. Citation2002; Toro et al. Citation2005; Wagenaar et al. Citation2005; Atterbury et al. Citation2007; Huff et al. Citation2010; Wernicki et al. Citation2017). For example, a recent study reported that the application of a bacteriophage commercial product Biotector S1 that significantly reduced the mortality rate in poultry challenged with Salmonella pullorum and Salmonella gallinarum. The treatment led to a 73% lower mortality rate compared to the control group (Abd-El Wahab et al. Citation2023). Recently, phage has been studied for the modulation of gut microbiota, which has improved the performance and health of chickens (Wang et al. Citation2013; Kim et al. Citation2014). In a recent study, the supplementation of a phage cocktail at a concentration of 0.05%, which included mixtures of phages targeting Salmonella gallinarum, S. typhimurium, S. enteritidis, and Escherichia coli at concentrations of 1.0x108 PFU/g, along with Clostridium perfringens at 1.0x106 PFU/g, resulted in a consistent increase in broiler weight gain during both the 1-7 day and 22-35 day periods. Furthermore, this supplementation positively influenced the gut microbiota, with a notable rise in the relative abundance of Lactobacillus salivarus compared to the control group (Upadhaya et al. Citation2021). However, the combination of phages and probiotics has never been studied in chickens.
Escherichia coli, a bacterium commonly found in the guts of humans and animals, can be either commensal or pathogenic and significantly influences chickens’ microbial dynamics and health. The reduction of E. coli populations in the chicken gut may decrease competition with probiotics and provide more adhesion sites for probiotics to attach to intestinal epithelial cells, which may be necessary for the probiotics to exert their beneficial effects on the host (Mead Citation2005; Bermudez-Brito et al. Citation2012).
It is well-established that the gut microbiota plays a crucial role in the growth performance and health of chickens. While probiotics have been utilised, they have certain limitations. This study aims to investigate whether a combination of phages and probiotics can positively impact growth performance and health parameters. Currently, there is a knowledge gap regarding the synergistic effects of probiotics and phages on chicken growth performance and health, as compared to their individual effects. Therefore, the primary objective of this study is to assess the effects of dietary supplementation with 1 g/kg and 2 g/kg of a phage cocktail, 1 g/kg of PrimaLac® probiotics, and their various combinations on specific gut populations (Escherichia coli, Lactobacillus spp., Bifidobacterium spp., and Clostridium perfringens), serum lipid profiles, the heterophil-to-lymphocyte ratio (H:L), serum immunoglobulins (IgY, IgA, and IgM), as well as measurements of ileal villus height, crypt depth, and relative organ weights in chickens at both 21 days (starter period) and 35 days (finisher period) of age. By gaining insights into their interactions, this study aims to provide a better understanding and knowledge of effective methods to modulate gut microbiota, offering a potential alternative to antibiotic growth promoters in poultry production.
Materials and methods
Phage and probiotics preparation
Four Escherichia coli phages were prepared in large-scale production at a titre of 1010 PFU/g each, as described by Wong et al. (Citation2014). E. coli bacteria and phages were isolated from the ileal and caecal contents of chickens at the ages of 7, 14, 21, and 42 days. These chickens were fed a basal diet without any added antibiotics. The isolation process was carried out as detailed in the study by Shaufi and Sieo (Citation2023). Briefly, skim milk (Oxoid, UK) (high-temperature protectant), maltodextrin (cryoprotectant) and calcium carbonate (CaCO3) (Merck, Germany) (antacid) and phage were added at a ratio of 2:2:1:5, respectively. The mixture was freeze-dried using a Labconco freeze dryer (Labconco, USA). The phage titre was quantified by double layer agar assay and ensured at a concentration of ∼1010 Plaque Forming Units per gram (PFU/g). Each phage was then mixed at a ratio of 1:1:1:1 as final phage cocktail supplement. Commercial PrimaLac® probiotics (Starlabs, USA) used in this study consisted of Lactobacillus acidophilus, Lactobacillus casei, Bifidobacterium termophilum, Enterococcus faecium and Aspergillus oryzae at a concentration of 109 Colony Forming Units per gram (CFU/g) each.
Experimental design, animals and diets
The experiment was performed based on completely randomised design with factorial arrangements of a 3 (phage cocktail dosage; 0 g/kg, 1 g/kg and 2 g/kg) x 2 (probiotics dosage; 0 g/kg and 1 g/kg) which resulted in 6 treatments. A total of 288 one-day-old male Cobb 500 broilers were utilised in this study. They were randomly allocated to six dietary treatments. Each treatment was replicated six times, with each replicate consisting of a battery cage housing eight chicks. Phage cocktail and probiotics supplements were added by replacing an equivalent amount of corn to achieve a final equivalent amount for each treatment group. The basal diets formulated for starter (1 to 21 days) and finisher (22 to 35 days) (Table ) periods were antibiotic-free, in mash form, and ensured to meet or exceed the energy and nutrient requirements as recommended by NRC (Citation1994) for each growing phase. Both feeds and water were provided ad libitum.
Table 1. Feed composition (as fed basis) of basal diets of starter and finisher.
Feed chemical analyses
The formulated feed was also chemically analysed on final crude protein, crude fat, crude fibre, calcium, phosphorus and sodium by an in-house test done by the Malaysian Agricultural Research and Development Institute (MARDI) using proximate feed analyses (Table ).
Sampling
For the assessment of growth performance, each experimental unit consisted of a cage housing eight Cobb500 broilers. The experiment was conducted with six replicate cages for each treatment (n = 6). Therefore, each value reported in this study for growth performance represents the mean of these six replicate cages. The body weight (BW) of the chickens and their body weight gain (BWG) were measured separately every week (at 1, 7, 14, 21, 28, and 35 days). The amount of feed intake (FI) was recorded on a daily basis. Subsequently, the feed conversion ratio (FCR) was calculated. The chicken body weight and the mucosal layer of ilea and caeca samples were used for a gut microbial population study based on real-time quantitative PCR (qPCR) at day 21 and 35. Twelve chickens per treatment (2 chickens per cage) were randomly chosen and euthanised at day 21 and 35. Blood samples were immediately collected from the jugular veins into 10 ml non-coagulated clot activator tubes (BD, USA) and coagulated K2EDTA tubes (BD, USA) for biochemical and serum immunoglobulins analyses, and haematological analysis, respectively. The ilea tissues were also collected to measure the villus height and crypt depth.
DNA extraction
Genomic DNA was extracted using QIAamp Fast DNA Stool Mini Kit (QIAGEN, Germany) following manufacturer instructions. The extracted genomic DNA was stored at −20 °C until further analysis.
Quantitative real-time PCR
For the assessment of gut microbiota, each experimental unit consisted of a cage housing one Cobb 500 broiler. The experiment was conducted with three replicate cages for each treatment (n = 3). Therefore, each value reported in this study for gut microbiota represents the mean of these three replicate cages. The genomic DNA extracted from ileal and caecal contents of 21 and 35 d chickens (3 chickens) were used as the templates for real-time qPCR. DNA amplification was conducted using SensiFAST SYBR No-ROX Kit (Bioline, UK) in CFX96 Real-Time PCR Detection System (Bio-Rad, USA). The 20 µL reaction mixtures contained 10 µL SensiFAST SYBR No-ROX mix, normalised genomic DNA template at 80 ng/µl, 1 µL of forward and reverse primers (10 pmol/µl) and sterile Mili-Q water. Both standard curve samples and no template control (NTC) were prepared in every run. The themoprofile and primer sequences are given in Table S1.
Serum lipid
For the assessment of serum lipid, each experimental unit consisted of a cage housing one Cobb 500 broiler. The experiment was conducted with six replicate cages for each treatment (n = 6). Therefore, each value reported in this study for serum lipid represents the mean of these six replicate cages. The blood samples from non-coagulated clot activator tubes (BD, USA) were processed immediately by centrifugation using JA-20.1 rotor of Beckman Avanti J-25I Centrifuge (Beckman, USA) at 3000 x g for 10 min. The serum samples were collected and transferred into sterile 2 mL microcentrifuge tubes and used for biochemistry analyses of triglycerides, cholesterol, high-density lipoprotein (HDL) and low-density lipoprotein (LDL) using COBAS reagents (Roche Diagnostics, USA) and analysed using Hitachi 902 Automatic Biochemistry Analyser (Hitachi, Japan). The same serum samples were stored at −20 °C for serum immunoglobulins analysis.
Haematological analysis
For the assessment of hematological parameters, each experimental unit consisted of a cage housing one Cobb 500 broiler. The experiment was conducted with six replicate cages for each treatment (n = 6). Therefore, each value reported in this study for hematological parameters represents the mean of these six replicate cages. Blood samples were collected from coagulated K2EDTA tubes (BD, USA) and subjected to analysis for hematological parameters. To determine the white blood cell (WBC) count, blood smears were prepared and stained with Wright’s stain (Sigma Chemical, USA). Manual examination of the stained smears enabled the categorisation of WBCs into different cell types, including heterophils and lymphocytes. Additionally, the heterophil-to-lymphocyte ratio (H:L) was calculated as part of the analysis.
Serum immunoglobulins
For the assessment of serum immunoglobulins, each experimental unit consisted of a cage housing one Cobb 500 broiler. The experiment was conducted with three replicate cages for each treatment (n = 3). Therefore, each value reported in this study for serum immunoglobulins represents the mean of these three replicate cages. Serum samples of three chickens from separate cages were selected for IgY, IgA and IgM analyses of every treatment for day 21 and 35 samplings. Serums for each sample were diluted at appropriate dilutions and quantified. Serum immunoglobulins were quantified using a double-antibody sandwich enzyme-linked immunosorbent assay (ELISA) kit of chicken IgY, IgA and IgM (Bethyl Laboratories Inc., USA) by following manufacturer instructions. Absorbance was measured using BioTek EL800 Absorbance Reader (BioTek, USA) at a wavelength of 450 nm. The standard curve was plotted from the graph of average absorbance measured versus known concentration of chicken IgY, IgA and IgM to determine and calculate the concentration of unknown samples. The data was then multiplied by the dilution factor used earlier to determine the amount of antibodies in the original undiluted sample.
Ileal villus height and crypt depth
For the assessment of intestinal histomorphology, each experimental unit consisted of a cage housing two Cobb 500 broilers. The experiment was conducted with six replicate cages for each treatment (n = 6). Therefore, each value reported in this study for intestinal histomorphology represents the mean of these six replicate cages. The 1 cm midpoint ileum was excised for measurements of the villi height and crypt depths from 12 chickens per treatment (2 chickens per replicate cage) for day 21 and 35 samplings. The tissue samples were rinsed in phosphate-buffered saline (PBS) buffer (pH 7.4) and fixed in 10% buffered formalin overnight. They were dried in an automatic tissue processor for 16 h using Leica ASP 3000 fully-enclosed tissue processor (Leica, Japan) and embedded in paraffin wax by using Leica EG1160 paraffin embedding station (Leica, Japan). The tissue samples were cut into sections at 4 µm thickness by using Leica RM2155 microtome (Leica, Japan), placed on glass slides and then stained with haematoxylin and eosin. The sections were examined under Olympus light microscope QG2-32 (Olympus, Japan) with a digital camera attached. The villus height was measured using Fiji ImageJ (Schindelin et al. Citation2012) from the top end of the villus to the bottom of the villus-crypt junction, while crypt depth from the basement membrane to the villus-crypt junction. An average of 3 villus heights and crypt depth were measured for each sample.
Relative weight of organs
For the assessment of relative organ weights, each experimental unit consisted of a cage housing two Cobb 500 broilers. The experiment was conducted with six replicate cages for each treatment (n = 6). Therefore, each value reported in this study for relative organ weights represents the mean of these six replicate cages. Seven different chicken organs (thymus, proventriculus, gizzard, pancreas, liver, spleen, bursa of Fabricius and heart) were dissected from 6 chickens per treatment (2 chickens per replicate cage) of day 21 and 35 samplings. The organs were thoroughly excised and cleaned before weighing. The relative weight (RW) of organs was then calculated as follows:
Statistical analysis
The experimental data were analysed based on General Linear Model (GLM) using Statistical Package for Social Science (SPSS) Statistics version 26 (IBM, USA). The replicate cage was used as the experimental unit for all parameters unless stated otherwise. Results were stated as means. Data were analysed as 3 × 2 factorial design including the control (three levels of phage cocktail by two levels of probiotics). A p value of ≤ 0.05 was considered significant. Duncan’s mean separation test was used to make pairwise comparisons among treatments when interaction was significant.
Results
Growth performance
The results of our study revealed a significant interaction between the phage cocktail and probiotics in relation to the FCR during both the 22–35 day period (p = 0.010) and the 1–35 day period (p = 0.005) (Table ). The FCR for the group receiving 1 g/kg phage cocktail and probiotics group was significantly (p < 0.05) lower than that of control group during both 22–35 day (FCR = 1.86) and 1–35 day periods (FCR = 1.57).
Table 2. Effects of dietary treatments on body weight gain (BWG), feed intake (FI) and feed conversion ratio (FCR) of chickens at 1–21 d, 22–35 d and 1–35 d periods.
Real-time PCR quantification of selected gut microbiota
The interaction between the phage cocktail and probiotic supplementation significantly influenced the populations of Bifidobacterium spp. (p = 0.046) and C. perfringens (p = 0.007) in the ilea of 35-day-old chickens (Table ). A positive interaction effect was observed for C. perfringens, where chickens fed with 1 g/kg of both the phage cocktail and probiotics had significantly lower C. perfringens populations (2.51 log10 DNA copy number) compared to those fed with 1 g/kg of the phage cocktail alone (2.91 log10 DNA copy number). Unexpectedly, a negative interaction was observed in chickens fed with 1 g/kg of both the phage cocktail and probiotics. These chickens had significantly lower Bifidobacterium spp. populations (2.21 log10 DNA copy number) at 35 days compared to those fed with 1 g/kg of probiotics alone (2.54 log10 DNA copy number).
Table 3. Effects of dietary treatments on E. coli, Lactobacillus spp., Bifidobacterium spp. and C. perfringens populations based on real-time qPCR in ilea of 21 d and 35 d chickens.
The addition of E. coli phage cocktail as a supplement was expected to reduce the population of E. coli compared to the other groups. This reduction was observed in the ilea of 21-day-old chickens when 1 g/kg of the phage cocktail and 1 g/kg of probiotics were administered, with E. coli recording the lowest population at 3.25 log10 DNA copy number compared to the other groups. However, this reduction was not statistically significant.
Surprisingly, the population count of Lactobacillus spp. was significantly lower (p = 0.014) in the ilea of 21-day-old chickens given probiotics alone (6.75 log10 DNA copy number) compared to those not given probiotics (7.56 log10 DNA copy number) (Table ). A similar trend was also observed for the population of Bifidobacterium spp. in the ilea of 21-day-old chickens, with the population count significantly lower (p = 0.045) in chickens given probiotics (1.88 log10 DNA copy number) compared to those not given probiotics (2.72 log10 DNA copy number).
Surprisingly, the population of Bifidobacterium spp. was significantly lower (p = 0.000) in the caeca of 21-day-old chickens fed with probiotics (4.54 log10 DNA copy number) compared to those in the non-probiotics group (5.28 log10 DNA copy number) (Table ). Conversely, the group receiving probiotics alone showed a significant reduction (p = 0.001) in the C. perfringens population (2.40 log10 DNA copy number) when compared to the non-probiotics group (2.87 log10 DNA copy number).
Table 4. Effects of dietary treatments on E. coli, Lactobacillus spp., Bifidobacterium spp. and C. perfringens populations based on real-time qPCR in caeca of 21 d and 35 d chickens.
The effect of 1 g/kg phage cocktail alone was observed to reduce C. perfringens in the caeca of 21-day-old chickens, although this reduction was not statistically significant when compared to the doses of 0 g/kg and 2 g/kg of the phage cocktail alone.
Lipid profiles
A positive significant interaction was observed, where the supplementation of a 1 g/kg phage cocktail and a 1 g/kg probiotics resulted in a significant decrease in triglycerides (TG) levels (43.99 mg/dL, p = 0.034) in 35-day-old chickens, compared to the control group (0 g/kg) and the 1 g/kg phage cocktail alone group (Table ). For low-density lipoprotein (LDL)7 levels, chickens supplemented with 1 g/kg phage cocktail and 1 g/kg probiotics recorded among the lowest LDL (17.72 mg/dL), although there were no significant differences between groups in 21-day-old chickens.
Table 5. Effects of dietary treatments on lipid profiles of chickens at 21 and 35 d.
Notably, the supplementation of 1 g/kg phage cocktail alone resulted in a significant reduction in cholesterol (CHL) levels (102.12 mg/dL) in 35-day-old chickens, compared to the control group (0 g/kg) (116.46 mg/dL) and the higher dosage of phage cocktail (2 g/kg) (121.23 mg/dL). Conversely, a higher inclusion level of the phage cocktail (2 g/kg) resulted in a significantly higher HDL mean (75.34 mg/dL) compared to lower (1 g/kg) inclusion levels (61.20 mg/dL).
Haematological analysis
Chickens supplemented with 1 g/kg phage cocktail and 1 g/kg probiotics demonstrated a significant negative interaction concerning the number of lymphocytes in 21-day-old chickens (p = 0.011) (Table ). The combination group exhibited a significantly lower lymphocyte count compared to the control (0 g/kg) and the 1 g/kg phage cocktail group. However, this interaction did not have a significant impact on the heterophils to lymphocytes (H:L) ratio at both 21 and 35 days.
Table 6. Effects of dietary treatments on heterophils to lymphocytes (H:L) ratio of chickens at 21 and 35 d.
Chickens supplemented with probiotics alone resulted in a significantly higher number of heterophils in 35-day-old chickens (16.55 x 103/µl with probiotics vs 12.12 x 103/µl without; p = 0.001). Additionally, it led to a significantly lower number of lymphocytes in 21-day-old chickens (5.27 x 103/µl with probiotics vs 9.69 x 103/µl without; p = 0.011) and significantly higher H:L ratio in 21-day-old chickens (1.98 with probiotics vs 1.27 without; p = 0.003), as compared to the control group.
Serum immunoglobulins
In 21-day-old chickens, a 1 g/kg phage cocktail supplement significantly (p = 0.037) reduced serum IgY levels (1.232 g/L) compared to the control (0 g/kg, 2.537 g/L) (Table ). However, no significant changes were recorded when chickens were supplemented with a combination of phage cocktail and probiotics, or probiotics alone, on chickens’ levels of IgY, IgA, or IgM at all time points.
Table 7. Effects of dietary treatments on serum immunoglobulins of chickens at 21 and 35 d.
Ileal villus height and crypt depth
Chickens supplemented with probiotics alone had a significant (p < 0.05) impact on villus height, crypt depth, and villus-to-crypt ratio (Table ) (). Specifically, when 1 g/kg of probiotics was added to the diet, there was a significant (p < 0.05) increase in villus height at day 21 and day 35, measuring 694.27 µm and 138.10 µm, respectively, compared to the control group (588.21 µm and 116.16 µm, respectively). Additionally, the crypt depth at day 21 and day 35 was significantly lower (measuring 730.62 µm and 167.27 µm, respectively) in the group receiving probiotics compared to the control group (650.20 µm and 195.38 µm, respectively). The villus-to-crypt ratio, a key indicator of gut health and absorptive capacity, was also significantly (p < 0.05) higher (measuring 4.55) when probiotics were administered compared to the control group (measuring 3.57) in 35-day-old chickens. However, no significant difference was observed in 21-day-old chickens. In contrast, the phage cocktail alone and its interaction with probiotics did not yield any significant effects (p > 0.05) in all cases. Although supplementation of the phage cocktail (both at 1 g/kg and 2 g/kg) and probiotics did enhance the villus-to-crypt ratio compared to the other groups, this difference was not statistically significant.
Table 8. Effects of dietary treatments on ileal villi height and crypt depth of chickens at 21 and 35 d.
Table 9. Effects of dietary treatments on relative organs weights of chickens at 21 and 35 d.
Relative weights of organs
The results demonstrated a significant (p < 0.05) positive interaction between the phage cocktail and the probiotics supplement regarding the relative thymus weight in 21-day-old chickens (Table ). Chickens fed with 1 g/kg phage cocktail and probiotics recorded significantly higher relative weight of thymus compared to chickens fed with 2 g/kg phage cocktail alone. However, this was not significantly different from other groups.
Furthermore, another significant positive interaction between the phage cocktail and probiotics supplementation was observed in the relative heart weight of 21-day-old chickens. Chickens fed with 1 g/kg phage cocktail and probiotics recorded significantly higher relative weight of heart compared to the control (0 g/kg) and chickens fed with 1 g/kg phage cocktail alone. However, this was not significantly different compared to the probiotics group alone, 2 g/kg phage cocktail alone, and 2 g/kg phage cocktail combined with probiotics group.
Discussion
This study investigated the multifactorial factors affecting growth performance, specific gut populations, serum lipid profiles, heterophil-to-lymphocyte ratio (H:L), serum immunoglobulins (IgY, IgA, and IgM), ileal villus height and crypt depth measurements, and relative weights of organs of chickens at 21 (starter period) and 35-day-old (d) (finisher period) fed a diet supplemented with locally isolated phage cocktail (Escherichia coli phage cocktail at different dosages (four different phages that lyse four different Escherichia coli strains at 1010 PFU/g each)) and commercial probiotics (Lactobacillus acidophilus, Lactobacillus casei, Bifidobacterium termophilum, Enterococcus faecium, and Aspergillus oryzae at 109 CFU/g each). To our knowledge, no prior study has been conducted on the multiple factorial analysis of phage cocktail and probiotics supplementation in chickens. Overall, our study found that there was a significant interaction between 1 g/kg phage cocktail and 1 g/kg probiotics that improves the overall growth performance and health of chickens.
Our study provides significant insights into the synergistic interaction between the 1 g/kg phage cocktail and 1 g/kg probiotics on the Feed Conversion Ratio (FCR) during the 22–35 and 1–35 day periods. The combination of these two treatments resulted in the lowest FCR compared to other groups during these time periods, indicating a potential enhancement in feed efficiency. This is further supported by the observed significant difference in FCR between the group fed with 1 g/kg phage cocktail and 1 g/kg probiotics and the control group (0 g/kg phage cocktail and 0 g/kg probiotics).
These findings suggest a potential synergistic effect of the phage cocktail and probiotics on feed efficiency, which could have substantial implications for poultry farming. It could lead to more cost-effective feeding strategies and contribute to sustainable farming practices. However, it is important to interpret these results with caution. To establish more conclusive evidence, future studies may need to increase the sample size of chickens used.
Moreover, the combination of the phage cocktail and probiotics in chickens remains an underexplored area of research. This makes direct comparisons with existing studies challenging and underscores the novelty and importance of our findings. Further research in this area could help elucidate the mechanisms underlying the observed effects and potentially uncover new strategies for improving feed efficiency in poultry farming.
A significant positive interaction was observed between the administration of a 1 g/kg phage cocktail and 1 g/kg probiotics in reducing C. perfringens populations in the caeca of 35-day-old chickens. C. perfringens is commonly used as an indicator of gut pathogens, and a low log10 DNA copy number suggests a healthy gut equilibrium. The mechanisms behind the reduction of C. perfringens were not clear in this study, as the populations of probiotics, such as Lactobacillus and Bifidobacterium, were not significantly high. Surprisingly, Bifidobacterium was found to be significantly lower, which may raise questions about the efficacy of the probiotic strains used in this particular study. However, in the same study in the paper that has been published by Shaufi et al. (Citation2023), it was found that the bacteria producing short-chain fatty acids (SCFAs) were significantly higher (p < 0.05) as revealed by 16S rRNA amplicon next-generation sequencing. Additionally, the genes associated with carbohydrate and amino acid metabolism were significantly elevated (p < 0.05) in the group treated with a combination of phage cocktail and probiotics. This could be due to the phage cocktail’s ability to clear some adhesion sites in the intestines, thereby allowing the proliferation of beneficial SCFA-producing bacteria. This, in turn, could have led to a reduction in the load of C. perfringens, thereby exerting beneficial effects on the host.
Surprisingly, probiotic supplementation alone did not increase probiotic populations at 21 and 35 days. In fact, these populations were lower than in the non-probiotics group. Generally, previous studies have consistently reported that probiotics supplementation in chickens improves the population of probiotics (Biswas et al. Citation2019; Chang et al. Citation2019). However, the observations in this study did not support the initial hypothesis. It is possible that the use of probiotics alone may not effectively colonise the chicken intestines. In this case, the introduction of a phage cocktail could potentially play a crucial role in reducing the population of other microbes, thus creating more adhesion sites for probiotics to attach to the intestines. This lack of colonisation by probiotics in the chicken gut is not uncommon, as previous studies have also reported failures in increasing the probiotics population. In a study conducted by Al-Khalaifa et al. (Citation2019), it was observed that the supplementation of probiotics did not lead to an increase in the population of lactic acid bacteria, namely Lactobacillus, Bacillus coagulans, and Saccharomyces cerevisiae. However, it is important to note a significant caveat in this study, which is the lack of quantification for other probiotics microbes such as Enterococcus faecium and Aspergillus oryzae. It is possible that these unmeasured probiotics strains were elevated in the probiotics-supplemented groups. Additionally, it should be mentioned that the number of samples used for quantifying specific gut microbes was small, with a sample size of only n = 3. It is important to note that variations in the species of probiotics used may also influence their effectiveness in chickens (Otutumi et al. Citation2012; Abd El-Hack et al. Citation2020).
The results of this study demonstrated that supplementation with both 1 g/kg of a phage cocktail and 1 g/kg of probiotics led to a significant decrease in TG levels. This decrease was more pronounced than in the control group and the group that received only the 1 g/kg phage cocktail. Interestingly, the combination group did not significantly differ from the probiotics alone group in terms of TG levels, indicating that the lower dosage of phage cocktail maintains the efficacy of probiotics in reducing TG levels in chickens. While several previous studies have reported on the potential of probiotics to lower serum TG levels in chickens (Santoso et al. Citation1995; Kalavathy et al. Citation2003; Panda et al. Citation2006; Toghyani et al. Citation2015; Pourakbari et al. Citation2016), there is currently a dearth of scientific literature exploring the effects of phages or their combinations with probiotics on serum triglycerides. Therefore, our study fills an important knowledge gap in this field and provides novel insights of combining phages with probiotics on TG levels.
The present study discovered that the supplementation of chickens with a phage cocktail alone at a dosage of 1 g/kg led to a significant reduction in CHL levels. Moreover, when the chickens were supplemented with a higher dosage of 2 g/kg of the phage cocktail, there was a noteworthy increase in HDL levels. However, these effects were not observed in the group receiving probiotics alone. Previous studies have reported mixed results on the effects of probiotics on total cholesterol, HDL, and LDL in chickens. Shirisha et al. (Citation2017) reported no significant reduction on cholesterol when probiotics was supplemented in chickens. Other studies have shown the ability of probiotics to reduce serum cholesterol in chickens (Mohan et al. Citation1996; Jin et al. Citation1998; Kalavathy et al. Citation2003; Pourakbari et al. Citation2016). The precise mechanism through which phages are able to reduce CHL levels and increase HDL levels in chickens remains unclear. Moreover, there is a lack of research investigating the effects of phages on lipid levels in chickens, highlighting the need for further investigation in this area.
The H:L ratio is a critical indicator used to assess the physiological stress influencing a chicken’s immune system. Thiam et al. (Citation2022) reported that chickens with a lower H:L ratio demonstrated enhanced resistance to Salmonella infections in comparison to their counterparts exhibiting higher H:L ratios. The findings of our recent research demonstrated no significant elevation in the H:L ratio when chickens were administered a diet consisting solely of a phage cocktail, or in combination with probiotics. This finding suggests that the introduction of a phage cocktail does not impart physiological stress to chickens.
Our study also discovered that when chickens were given a diet supplemented with 1 g/kg of probiotics alone, the H:L ratio significantly increased compared to that of the control group at 21 days. This suggests that the dietary supplements may have induced physiological stress during the early growth stage. However, by the 35th day, both the lymphocyte count and the H:L ratio normalised to levels comparable with the control group, suggesting an adaptation of the chicken’s immune system to the dietary interventions. This finding contrasts with previous studies which reported negligible alterations in the H:L ratio in chickens supplemented with probiotics (Cengiz et al. Citation2015; Toghyani et al. Citation2015). The discrepancies between these findings and our own highlight the need for further investigations into the impact of probiotics supplementation on the physiological stress and immune response of chickens.
In the present investigation, a significant decrease in IgY levels was detected at 21 days in in chickens supplemented with 1 g/kg phage cocktail alone compared to control. These findings suggest that ad libitum administration of phages did not induce an increase in IgY production, even at high titres of the phage cocktail (1010 PFU/g). This was inconsistent with findings by Huff et al. (Citation2010) who discovered that chickens supplemented with phages had significantly higher IgY directly after phage supplementation due to activation of immune responses. This was also in contrast with a study in pigs where feeding with a phage cocktail did not significantly affect immunoglobulin levels (Kim et al. Citation2014). However, it is important to note that our study measured serum immunoglobulins only at 21 days and 35 days, rather than immediately after phage supplementation, as done in previous studies. Furthermore, it is crucial to consider factors such as the variation in the type of phages used and host genetics when interpreting these results.
In the present investigation, the addition of probiotics alone to the chicken diet resulted in a significant improvement in the villus height, crypt depth and villus to crypt ratio, indicating enhanced efficiency in nutrient absorption. This finding is consistent with previous research that has reported an elevation in the villus to crypt ratio in chickens supplemented with probiotics (Jin et al. Citation1998; Chichlowski et al. Citation2007; Liu et al. Citation2007; Awad et al. Citation2009; Giannenas et al. Citation2012; Kim et al. Citation2012; Dong et al. Citation2016). However, the addition of a phage cocktail alone or in combination with probiotics, did not provide any additional benefits. It is worth noting, though, that when the phage cocktail (at both 1 g/kg and 2 g/kg) and probiotics were combined, they showed a tendency towards enhancing the villus to crypt ratio compared to probiotics alone, although this difference did not reach statistical significance.
In the present investigation, we observed that the thymus relative weight (RW) of chickens supplemented with 1 g/kg of phage cocktail and probiotics at 21 days of age was significantly higher. This suggests that the immune system of the birds might have been stimulated, particularly with the administration of a higher dosage of phage cocktail and probiotics combination. However, we did not find any significant differences (p > 0.05) in the relative weight of the bursa of Fabricius among the different dietary treatments. This finding is consistent with previous studies that have also reported no significant differences in the bursa of Fabricius relative weight in probiotics-supplemented chickens (Zhang et al. Citation2012, Citation2013).
The chicken heart, similar to the human heart, consists of four chambers and is responsible for pumping deoxygenated blood to the lungs and oxygenated blood to the rest of the body. An increase in heart relative weight (RW) has been associated with a low heart rate and increased activity in birds (Machida and Aohagi Citation2001). In this study, we observed significant increases in heart RW in chickens supplemented with combination of phage cocktail and probiotics. However, these findings differ from those of Ghahri et al. (Citation2013), who found no significant difference in heart RW in birds fed with probiotics.
It is important to note that this study has certain limitations. Specifically, it did not measure the populations of other probiotics microbes, such as Enterococcus faecium and Aspergillus oryzae, which may have been increased in the groups receiving probiotics supplements. To our knowledge, no studies have been conducted on the impact of a phage cocktail and probiotics combination on factors such as specific chicken gut populations, blood serum biochemistry, antibody levels, intestinal physiology, and organ weight, making it challenging to draw comparisons. Future studies should include a positive control, such as antibiotics, in order to assess the effectiveness of this combination. Such investigations could contribute to the development of more efficient and sustainable alternatives for promoting growth in chickens.
Conclusions
To summarise, our study has demonstrated significant positive interactions with the combination of phage cocktail and probiotics in chicken diets. Specifically, the addition of 1 g/kg of phage cocktail and 1 g/kg of probiotics significantly (p < 0.05) improved growth performance, reduced the populations of Clostridium perfringens, and lowered triglyceride levels without causing physiological stress (as indicated by the absence of a significant change in the heterophil-to-lymphocyte [H:L] ratio). Additionally, there was a slight enhancement in the villus to crypt ratio, although it was not statistically significant. The significant (p < 0.05) elevation in the relative weight of the heart also indicated a possible improvement in heart function. Our study provides compelling evidence that the addition of 1 g/kg phage cocktail, in synergy with 1 g/kg probiotics, can significantly enhance growth performance and improve the overall health of chickens. These findings hold potential implications for poultry farming and animal health research. Further investigations into the underlying mechanisms and consideration of the study's limitations will contribute to a more comprehensive understanding of the observed effects.
Ethical approval
Procedures on chicken management, experimental design, methods and analyses have been approved by the Institutional Animal Care and Use Committee (IACUC) of Universiti Putra Malaysia (Ref: UPM/IACUC/AUP-R101/2015).
Supplemental Material
Download MS Word (17.7 KB)Acknowledgments
The research was conducted as part of Mohd Asrore Mohd Shaufi’s Ph.D. thesis. We would like to express our gratitude to the members of the Laboratory of Immunotherapeutics and Vaccines (LIVES), Institute of Bioscience, Universiti Putra Malaysia, including Mr. Khairul, Madam Haw, as well as to the members of the Animal Research Centre (ARC), at Universiti Putra Malaysia, for their invaluable contributions to the success of this project. Additionally, I would like to thank my late supervisor, Associate Prof. Dr. Sieo Chin Chin, for her motivating and inspiring guidance. Her spirit will always live in us.
Disclosure statement
No potential conflict of interest was reported by the author(s).
Data availability statement
The data that support the findings of this study are available from the corresponding author, Mohd Shaufi MA, upon reasonable request.
Additional information
Funding
References
- Abd-El Wahab A, Basiouni S, El-Seedi HR, Ahmed MFE, Bielke LR, Hargis B, Tellez-Isaias G, EISenreich W, Lehnherr H, Kittler S, et al. 2023. An overview of the use of bacteriophages in the poultry industry: successes, challenges, and possibilities for overcoming breakdowns. Front Microbiol. 14:1136638. doi:10.3389/fmicb.2023.1136638.
- Abd El-Hack ME, El-Saadony MT, Shafi ME, Qattan SYA, Batiha GE, Khafaga AF, Abdel-Moneim AE, Alagawany M. 2020. Probiotics in poultry feed: a comprehensive review. J Anim Physiol Anim Nutr. 104(6):1835–1850. doi:10.1111/jpn.13454.
- Al-Khalaifa H, Al-Nasser A, Al-Surayee T, Al-Kandari S, Al-Enzi N, Al-Sharrah T, Ragheb G, Al-Qalaf S, Mohammed A. 2019. Effect of dietary probiotics and prebiotics on the performance of broiler chickens. Poult Sci. 98(10):4465–4479. doi:10.3382/ps/pez282.
- Ashayerizadeh A, Dabiri N, Mirzadeh K, Ghorbani M. 2011. Effects of dietary inclusion of several biological feed additives on growth response of broiler chickens. J Cell Anim Biol. 5(4):61–65.
- Atterbury RJ, Van Bergen MA, Ortiz F, Lovell MA, Harris JA, De Boer A, Wagenaar JA, Allen VM, Barrow PA. 2007. Bacteriophage therapy to reduce salmonella colonization of broiler chickens. Appl Environ Microbiol. 73(14):4543–4549. doi:10.1128/AEM.00049-07.
- Awad WA, Ghareeb K, Abdel-Raheem S, Böhm J. 2009. Effects of dietary inclusion of probiotic and synbiotic on growth performance, organ weights, and intestinal histomorphology of broiler chickens. Poult Sci. 88(1):49–56. doi:10.3382/ps.2008-00244.
- Bermudez-Brito M, Plaza-Díaz J, Muñoz-Quezada S, Gómez-Llorente C, Gil A. 2012. Probiotic mechanisms of action. Ann Nutr Metab. 61(2):160–174. doi:10.1159/000342079.
- Biswas A, Junaid N, Kumawat M, Qureshi S, Mandal AB. 2019. Influence of dietary supplementation of probiotics on intestinal histo-morphometry, blood chemistry and gut health status of broiler chickens. SA J an Sci. 48(5):968–976. doi:10.4314/sajas.v48i5.17.
- Buahom J, Siripornadulsil S, Sukon P, Sooksawat T, Siripornadulsil W. 2023. Survivability of freeze- and spray-dried probiotics and their effects on the growth and health performance of broilers. Vet World. 16(9):1849–1865. doi:10.14202/vetworld.2023.1849-1865.
- Cengiz Ö, Köksal BH, Tatlı O, Sevim Ö, Ahsan U, Üner AG, Ulutaş PA, Beyaz D, Büyükyörük S, Yakan A, et al. 2015. Effect of dietary probiotic and high stocking density on the performance, carcass yield, gut microflora, and stress indicators of broilers. Poult Sci. 94(10):2395–2403. doi:10.3382/ps/pev194.
- Chang CH, Teng PY, Lee TT, Yu B. 2019. Effects of multi-strain probiotics combined with gardeniae fructus on intestinal microbiota, metabolites, and morphology in broilers. J Poult Sci. 56(1):32–43. doi:10.2141/jpsa.0170179.
- Chichlowski M, Croom WJ, Edens FW, McBride BW, Qiu R, Chiang CC, Daniel LR, Havenstein GB, Koci MD. 2007. Microarchitecture and spatial relationship between bacteria and ileal, cecal, and colonic epithelium in chicks fed a direct-fed microbial, PrimaLac, and salinomycin. Poult Sci. 86(6):1121–1132. doi:10.1093/ps/86.6.1121.
- d‘Herelle M. 1961. Sur un microbe invisible antagoniste des bacilles dysentériques. Buenos Aires, Argentina: Acta Kravsi.
- Dahiya JP, Wilkie DC, Van Kessel AG, Drew MD. 2006. Potential strategies for controlling necrotic enteritis in broiler chickens in post-antibiotic era. Anim Feed Sci Technol. 129(1–2):60–88. doi:10.1016/j.anifeedsci.2005.12.003.
- De Vuyst L, Avonts L, Makras L. 2004. Probiotics, prebiotics and gut health. In: Remacle C, Reusens B, editors. Functional Foods, Ageing and Degenerative Disease. Cambridge (UK): Woodhead Publishing; p. 416–482.
- Dong ZL, Wang YW, Song D, Hou YJ, Wang WW, Qi WT, Yun TT, Li AK. 2016. The effects of dietary supplementation of pre-microencapsulated Enterococcus fecalis and the extract of Camellia oleifera seed on growth performance, intestinal morphology, and intestinal mucosal immune functions in broiler chickens. Anim Feed Sci Technol. 212:42–51. doi:10.1016/j.anifeedsci.2015.11.014.
- Du Toit A. 2022. Decisions, decisions. Nat Rev Microbiol. 20(3):125–125. doi:10.1038/s41579-021-00677-7.
- Ghadban G. 2002. Probiotics in broiler production-a review. Arch Für Geflugelkunde. 66(2):49–58.
- Ghahri H, Toloei T, Soleimani1 B. 2013. Efficacy of antibiotic, probiotic, prebiotic and synbiotic on growth performance, organ weights, intestinal histomorphology and immune response in broiler chickens. Glob J Anim Sci. 1(1):25–41.
- Giannenas I, Papadopoulos E, Tsalie E, Triantafillou E, Henikl S, Teichmann K, Tontis D. 2012. Assessment of dietary supplementation with probiotics on performance, intestinal morphology and microflora of chickens infected with Eimeria tenella. Vet Parasitol. 188(1-2):31–40. doi:10.1016/j.vetpar.2012.02.017.
- Gill JJ, Hyman P. 2010. Phage choice, isolation, and preparation for phage therapy. Curr Pharm Biotechnol. 11(1):2–14. doi:10.2174/138920110790725311.
- Huff WE, Huff GR, Rath NC, Balog JM, Donoghue AM. 2002. Prevention of Escherichia coli infection in broiler chickens with a bacteriophage aerosol spray. Poult Sci. 81(10):1486–1491. doi:10.1093/ps/81.10.1486.
- Huff WE, Huff GR, Rath NC, Donoghue AM. 2010. Immune interference of bacteriophage efficacy when treating colibacillosis in poultry. Poult Sci. 89(5):895–900. doi:10.3382/ps.2009-00528.
- Jin LZ, Ho YW, Abdullah N, Jalaludin S. 1998. Growth performance, intestinal microbial populations, and serum cholesterol of broilers fed diets containing Lactobacillus cultures. Poult Sci. 77(9):1259–1265. doi:10.1093/ps/77.9.1259.
- Jin LZ, Ho YW, Abdullah N, Jalaludin S. 2000. Digestive and bacterial enzyme activities in broilers fed diets supplemented with Lactobacillus cultures. Poult Sci. 79(6):886–891. doi:10.1093/ps/79.6.886.
- Kabir S. 2009. The role of probiotics in the poultry industry. IJMS. 10(8):3531–3546. doi:10.3390/ijms10083531.
- Kalavathy R, Abdullah N, Jalaludin S, Ho YW. 2003. Effects of Lactobacillus cultures on growth performance, abdominal fat deposition, serum lipids and weight of organs of broiler chickens. Br Poult Sci. 44(1):139–144. doi:10.1080/0007166031000085445.
- Khosravi A, Boldaji F, Dastar B, Hasani S. 2010. Immune response and performance of broiler chicks fed protexin and propionic acid. Int J Poult Sci. 9(2):188–191. doi:10.3923/ijps.2010.188.191.
- Kim GB, Seo YM, Kim CH, Paik IK. 2011. Effect of dietary prebiotic supplementation on the performance, intestinal microflora, and immune response of broilers. Poult Sci. 90(1):75–82. doi:10.3382/ps.2010-00732.
- Kim JH, Kim JW, Lee BB, Lee GI, Lee JH, Kim GB, Kil DY. 2014. Effect of dietary supplementation of bacteriophage on growth performance and cecal bacterial populations in broiler chickens raised in different housing systems. Livest Sci. 170:137–141. doi:10.1016/j.livsci.2014.09.005.
- Kim JS, Ingale SL, Kim YW, Kim KH, Sen S, Ryu MH, Lohakare JD, Kwon IK, Chae BJ. 2012. Effect of supplementation of multi-microbe probiotic product on growth performance, apparent digestibility, cecal microbiota and small intestinal morphology of broilers. J Anim Physiol Anim Nutr. 96(4):618–626. doi:10.1111/j.1439-0396.2011.01187.x.
- Kim KH, Ingale SL, Kim JS, Lee SH, Lee JH, Kwon IK, Chae BJ. 2014. Bacteriophage and probiotics both enhance the performance of growing pigs but bacteriophage are more effective. Anim Feed Sci Technol. 196:88–95. doi:10.1016/j.anifeedsci.2014.06.012.
- Kok RG, de Waal A, Schut F, Welling GW, Weenk G, Hellingwerf KJ. 1996. Specific detection and analysis of a probiotic Bifidobacterium strain in infant feces. Appl Environ Microbiol. 62(10):3668–3672. doi:10.1128/aem.62.10.3668-3672.1996.
- Lee MD, Pedroso AA, Lumpkins B, Cho Y, Maurer JJ. 2023. Pioneer colonizers: bacteria that alter the chicken intestinal morphology and development of the microbiota. Front Physiol. 14:1139321. doi:10.3389/fphys.2023.1139321.
- Liu JR, Lai SF, Yu B. 2007. Evaluation of an intestinal Lactobacillus reuteri strain expressing rumen fungal xylanase as a probiotic for broiler chickens fed on a wheat-based diet. Br Poult Sci. 48(4):507–514. doi:10.1080/00071660701485034.
- Loc-Carrillo C, Abedon ST. 2011. Pros and cons of phage therapy. Bacteriophage. 1(2):111–114. doi:10.4161/bact.1.2.14590.
- Machida N, Aohagi Y. 2001. Electrocardiography, heart rates, and heart weights of free-living birds. J Zoo Wildl Med. 32(1):47–54. doi:10.1638/1042-7260(2001)032[0047:EHRAHW]2.0.CO;2.
- Mazanko MS, Popov IV, Prazdnova EV, Refeld AG, Bren AB, Zelenkova GA, Chistyakov VA, ALGburi A, Weeks RM, Ermakov AM, et al. 2022. Beneficial effects of spore-forming bacillus probiotic bacteria isolated from poultry microbiota on broilers’ health, growth performance, and immune system. Front Vet Sci. 9:877360. doi:10.3389/fvets.2022.877360.
- Mead G. 2005. The use of probiotics to control foodborne pathogens in poultry. Food safety control in the poultry industry. Cambridge (UK): Woodhead Publishing Limited. 216–236.
- Mohan B, Kadirvel R, Natarajan A, Bhaskaran M. 1996. Effect of probiotic supplementation on growth, nitrogen utilisation and serum cholesterol in broilers. Br Poult Sci. 37(2):395–401. doi:10.1080/00071669608417870.
- Mountzouris KC, Tsitrsikos P, Palamidi I, Arvaniti A, Mohnl M, Schatzmayr G, Fegeros K. 2010. Effects of probiotic inclusion levels in broiler nutrition on growth performance, nutrient digestibility, plasma immunoglobulins, and cecal microflora composition. Poult Sci. 89(1):58–67. doi:10.3382/ps.2009-00308.
- NRC. 1994. Nutrient requirements of poultry. Ninth Revised Edition, 1994. Washington, DC (US): The National Academies Press.
- O'Toole PW, Cooney JC. 2008. Probiotic bacteria influence the composition and function of the intestinal microbiota. Interdiscip Perspect Infect Dis. 2008:175285–175289. doi:10.1155/2008/175285.
- Oladokun S, Koehler A, MacIsaac J, Ibeagha-Awemu EM, Adewole DI. 2021. Bacillus subtilis delivery route: effect on growth performance, intestinal morphology, cecal short-chain fatty acid concentration, and cecal microbiota in broiler chickens. Poult Sci. 100(3):100809. doi:10.1016/j.psj.2020.10.063.
- Olnood CG, Beski SSM, Choct M, Iji PA. 2015. Novel probiotics: their effects on growth performance, gut development, microbial community and activity of broiler chickens. Anim Nutr. 1(3):184–191. doi:10.1016/j.aninu.2015.07.003.
- Otutumi LK, Góis MB, Garcia EM, Loddi MM. 2012. Variations on the efficacy of probiotics in poultry. London (UK): Intech.
- Palamidi I, Fegeros K, Mohnl M, Abdelrahman WHA, Schatzmayr G, Theodoropoulos G, Mountzouris KC. 2016. Probiotic form effects on growth performance, digestive function, and immune related biomarkers in broilers. Poult Sci. 95(7):1598–1608. doi:10.3382/ps/pew052.
- Pan D, Yu Z. 2014. Intestinal microbiome of poultry and its interaction with host and diet. Gut Microbes. 5(1):108–119. doi:10.4161/gmic.26945.
- Panda AK, Rao SVR, Raju MVLN, Sharma SR. 2006. Dietary supplementation of Lactobacillus Sporogenes on performance and serum biochemico-lipid profile of broiler chickens. J Poult Sci. 43(3):235–240. doi:10.2141/jpsa.43.235.
- Park YH, Hamidon F, Rajangan C, Soh KP, Gan CY, Lim TS, Abdullah WN, Liong MT. 2016. Application of probiotics for the production of safe and high-quality poultry meat. Korean J Food Sci Anim Resour. 36(5):567–576. doi:10.5851/kosfa.2016.36.5.567.
- Petrovic Fabijan A, Iredell J, Danis-Wlodarczyk K, Kebriaei R, Abedon ST. 2023. Translating phage therapy into the clinic: recent accomplishments but continuing challenges. PLOS Biol. 21(5):e3002119. doi:10.1371/journal.pbio.3002119.
- Porter SB, Johnston BD, Kisiela D, Clabots C, Sokurenko EV, Johnson JR. 2022. Bacteriophage cocktail and microcin-producing probiotic Escherichia coli protect mice against gut colonization with multidrug-resistant Escherichia coli sequence Type 131. Front Microbiol. 13:887799. doi:10.3389/fmicb.2022.887799.
- Pourakbari M, Seidavi A, Asadpour L, Martínez A. 2016. Probiotic level effects on growth performance, carcass traits, blood parameters, cecal microbiota, and immune response of broilers. An Acad Bras Cienc. 88(2):1011–1021. doi:10.1590/0001-3765201620150071.
- Roughgarden J. 2023. Lytic/lysogenic transition as a life-history switch. bioRxiv.1-12. doi:10.1101/2023.01.03.522622.
- Samanya M, Yamauchi KE. 2002. Histological alterations of intestinal villi in chickens fed dried Bacillus subtilis var. natto. Comp Biochem Physiol A Mol Integr Physiol. 133(1):95–104. doi:10.1016/s1095-6433(02)00121-6.
- Santoso U, Tanaka K, Ohtani S. 1995. Effect of dried Bacillus subtilis culture on growth, body composition and hepatic lipogenic enzyme activity in female broiler chicks. Br J Nutr. 74(4):523–529. doi:10.1079/bjn19950155.
- Schindelin J, Arganda-Carreras I, Frise E, Kaynig V, Longair M, PieTzsch T, Preibisch S, Rueden C, Saalfeld S, Schmid B, et al. 2012. Fiji: an open-source platform for biological-image analysis. Nat Methods. 9(7):676–682. doi:10.1038/nmeth.2019.
- Shaufi MAM, Sieo CC. 2023. Escherichia coli phages isolated from broiler chickens showed ideal characteristics in gut modulation. AsPac J. Mol. Biol. Biotechnol. 31(2):1–25. doi:10.35118/apjmbb.2023.031.2.01.
- Shaufi MAM, Sieo CC, Chong CW, Geok Hun T, Omar AR, Han Ming G, Wan Ho Y. 2023. Effects of phage cocktail, probiotics, and their combination on growth performance and gut microbiota of broiler chickens. Animals. 13(8):1328. doi:10.3390/ani13081328.
- Shirisha, R., Raju, M.V. L.N., Reddy, S. Sai, Reddy, V. Ravinder, Krishnadaida,. 2017. Effect of dietary supplementation of probiotic (problend) on immune Status, biochemical profile and E. coli counts in commercial broiler chicken. J. Anim. Rese. 7(4):717–721. doi:10.5958/2277-940X.2017.00110.3.
- Smith JM. 2014. A review of avian probiotics. J Avian Med Surg. 28(2):87–94. doi:10.1647/2012-031.
- Stanley D, Denman SE, Hughes RJ, Geier MS, Crowley TM, Chen H, Haring VR, Moore RJ. 2012. Intestinal microbiota associated with differential feed conversion efficiency in chickens. Appl Microbiol Biotechnol. 96(5):1361–1369. doi:10.1007/s00253-011-3847-5.
- Sugiharto S. 2016. Role of nutraceuticals in gut health and growth performance of poultry. J Saudi Soc Agric Sci. 15(2):99–111. doi:10.1016/j.jssas.2014.06.001.
- Thiam M, Wang Q, Barreto Sánchez AL, Zhang J, Ding J, Wang H, Zhang Q, Zhang N, Wang J, Li Q, et al. 2022. Heterophil/lymphocyte ratio level modulates salmonella resistance, cecal microbiota composition and functional capacity in infected chicken. Front Immunol. 13:816689. doi:10.3389/fimmu.2022.816689.
- Toghyani M, Mosavi SK, Modaresi M, Landy N. 2015. Evaluation of kefir as a potential probiotic on growth performance, serum biochemistry and immune responses in broiler chicks. Anim Nutr. 1(4):305–309. doi:10.1016/j.aninu.2015.11.010.
- Toro H, Price SB, McKee AS, Hoerr FJ, Krehling J, Perdue M, Bauermeister L. 2005. Use of bacteriophages in combination with competitive exclusion to reduce Salmonella from infected chickens. Avian Dis. 49(1):118–124. doi:10.1637/7286-100404R.
- Tran C, Horyanto D, Stanley D, Cock IE, Chen X, Feng Y. 2023. Antimicrobial properties of bacillus probiotics as animal growth promoters. Antibiotics. 12(2):407. doi:10.3390/antibiotics12020407.
- Upadhaya SD, Ahn JM, Cho JH, Kim JY, Kang DK, Kim SW, Kim HB, Kim IH. 2021. Bacteriophage cocktail supplementation improves growth performance, gut microbiome and production traits in broiler chickens. J Anim Sci Biotechnol. 12(1):49. doi:10.1186/s40104-021-00570-6.
- Wagenaar JA, Van Bergen MA, Mueller MA, Wassenaar TM, Carlton RM. 2005. Phage therapy reduces Campylobacter jejuni colonization in broilers. Vet Microbiol. 109(3–4):275–283. doi:10.1016/j.vetmic.2005.06.002.
- Walter J, Hertel C, Tannock GW, Lis CM, Munro K, Hammes WP. 2001. Detection of Lactobacillus, Pediococcus, Leuconostoc, and Weissella species in human feces by using group-specific PCR primers and denaturing gradient gel electrophoresis. Appl Environ Microbiol. 67(6):2578–2585. doi:10.1128/AEM.67.6.2578-2585.2001.
- Wang JP, Yan L, Lee JH, Kim IH. 2013. Evaluation of bacteriophage supplementation on growth performance, blood characteristics, relative organ weight, breast muscle characteristics and excreta microbial shedding in broilers. Asian-Australas J Anim Sci. 26(4):573–578. doi:10.5713/ajas.2012.12544.
- Wang RF, Cao WW, Franklin W, Campbell W, Cerniglia CE. 1994. A 16S rDNA-based PCR method for rapid and specific detection of Clostridium perfringens in food. Mol Cell Probes. 8(2):131–137. doi:10.1006/mcpr.1994.1018.
- Wei S, Morrison M, Yu Z. 2013. Bacterial census of poultry intestinal microbiome. Poult Sci. 92(3):671–683. doi:10.3382/ps.2012-02822.
- Wen R, Li C, Zhao M, Wang H, Tang Y. 2022. Withdrawal of antibiotic growth promoters in China and its impact on the foodborne pathogen Campylobacter coli of swine origin. Front Microbiol. 13:1004725. doi:10.3389/fmicb.2022.1004725.
- Wernicki A, Nowaczek A, Urban-Chmiel R. 2017. Bacteriophage therapy to combat bacterial infections in poultry. Virol J. 14(1):179. doi:10.1186/s12985-017-0849-7.
- Wong CL, Sieo CC, Tan WS, Abdullah N, Hair-Bejo M, Abu J, Ho YW. 2014. Evaluation of a lytic bacteriophage, Phi st1, for biocontrol of Salmonella enterica serovar Typhimurium in chickens. Int J Food Microbiol. 172:92–101. doi:10.1016/j.ijfoodmicro.2013.11.034.
- Yousaf S, Nouman HM, Ahmed I, Husain S, Waseem M, Nadeem S, Tariq M, Sizmaz O, Chudhry MFZ. 2022. A review of probiotic applications in poultry: improving immunity and having beneficial effects on production and health. Postepy Mikrobiologii. 61(3):115–123. doi:10.2478/am-2022-010.
- Zhang L, Zhang L, Zhan X, Zeng X, Zhou L, Cao G, Chen A, Yang C. 2016. Effects of dietary supplementation of probiotic, Clostridium butyricum, on growth performance, immune response, intestinal barrier function, and digestive enzyme activity in broiler chickens challenged with Escherichia coli K88. J Anim Sci Biotechnol. 7(1):3. doi:10.1186/s40104-016-0061-4.
- Zhang ZF, Cho JH, Kim IH. 2013. Effects of Bacillus subtilis UBT-MO2 on growth performance, relative immune organ weight, gas concentration in excreta, and intestinal microbial shedding in broiler chickens. Livest Sci. 155(2–3):343–347. doi:10.1016/j.livsci.2013.05.021.
- Zhang ZF, Zhou TX, Ao X, Kim IH. 2012. Effects of β-glucan and Bacillus subtilis on growth performance, blood profiles, relative organ weight and meat quality in broilers fed maize–soybean meal based diets. Livest Sci. 150(1-3):419–424. doi:10.1016/j.livsci.2012.10.003.