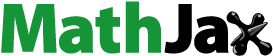
ABSTRACT
The adoption of sustainable 4D printing in automotive component manufacturing industry helps to produce environmentally conscious critical components. This article introduces the study in which 18 sustainable 4D printing barriers were identified through expert consultation and literature review. The identified barriers were analysed against six criteria, namely, Build Time (C1), Accuracy (C2), Customization (C3), Carbon Footprint (C4), Material Utilization (C5) and Complexity (C6) using Grey Technique for Order of Preference by Similarity to Ideal Solution (G-TOPSIS) approach. Experts’ inputs were gathered to derive the relative importance of each barrier against each criterion in the form of Grey value. Using G-TOPSIS approach, the priority order of barriers is achieved. Based on the findings, significant barriers are found to be ‘Improper disposal strategy (B18)’, ‘Lack of interaction between smart materials and 3D printing technology (B8)’ and ‘Lack of Smart materials compatible with AM technologies (B10)’. The study facilitated industry practitioners to meticulously examine sustainable 4D printing barriers for improving production efficiency.
Introduction
Additive manufacturing integration with smart manufacturing is recognised for numerous reasons, such as real-time data analysis, creating complex shapes with good quality, decentralised production and augmented reality (Wankhede and Vinodh Citation2021). Moreover, it shows enormous capability in dealing with environmental impacts of new components (Laverne et al. Citation2019). 4D printing is the latest study topic that emerged with the introduction of 3D printing process. ‘4D printing is the development in the property, shape, and usefulness of a 3D printed structure over time when it is subjected to light, heat, pH, water, and so on’ (Choi et al. Citation2015; Kumar et al. Citation2021). As a new subject of study, 4D printing has various limits and obstacles that must be solved quickly (Kumar et al. Citation2021). The three primary hurdles of 4D printing are material, technological and design problems. Technological obstacles include the lack of 3D printing technology (Ahmed et al. Citation2021). Once these constraints are overcome, 4D printing will revolutionise product manufacture and design in the future. It will shorten marketing time, capital need, reduce the size of products, make items readily transportable, thereby creating an efficient and new business model (Ahmed et al. Citation2021; Azhar and Pei Citation2022).
3D printing technology was first discovered in 1987, and it has since attracted governments, researchers and enterprises to use it to produce distinct products from 3D models utilising computer-aided design (CAD) software (Nugroho et al. Citation2021). This initiative is prompted by many benefits based on 3D printing when compared to conventional production processes like injection moulding and extrusion. Such a method can construct a 3D structure with complicated geometry, which is extremely difficult to create with traditional injection moulding (Jian et al. Citation2022; Nugroho et al. Citation2021). Furthermore, it is centred on additive manufacturing technology, which constructs an item by applying raw materials layer by layer to reduce waste (Ameta et al. Citation2022; Maraveas, Bayer, and Bartzanas Citation2022). Subtractive manufacturing, for instance computer numerical control (CNC) machining, on the other hand, produces a component by reducing the quantity of material used. However, 3D printing cannot completely replace traditional production due to challenges with surface smoothness, printing speed and mechanical qualities of printed items. Because the fabrication process of this technique is slower than that of injection moulding and extrusion, it may not be suited for large-scale manufacturing (Mallakpour, Tabesh, and Hussain Citation2021). The generated items from 3D printing often have a rough surface due to the staircase effect (Nugroho et al. Citation2021; Trenfield et al. Citation2019). 3D printing with programming, stimuli, time and smart materials may be converted into 4D printing. The term time in this context does not refer to the time it takes to manufacture items. Skylar Tibbits demonstrated how alterations in a static produced item occur over time at the MIT TED conference in 2012. The term ‘electronic commerce’ implies to the auction of goods and services over the internet. This resulted in a new printing age encompassing a new dimension in 3D printing, namely, time, and this was given its own name, 4D printing technology. The fourth dimension is time, which can be inferred as 4D printing is time as an additional dimension of 3D printing (Demoly et al. Citation2021; Kumar et al. Citation2021; Mallakpour, Tabesh, and Hussain Citation2021).
4D printing possesses sustainable benefits such as efficient product design, efficient production operations, efficient supply chain, process optimisation and environmental-friendly manufacturing (Saritha and Boyina Citation2021). Efficient product design depicts product design to utilise less waste. Integration of physical and digital logistics through additive manufacturing drives sustainability in 4D printing. However, several barriers to sustainable additive manufacturing need to be recognised and analysed. In a nutshell, this study focussed on following research questions:
What are the barriers hindering sustainable 4D printing adoption in automotive industry?
How to recognise and evaluate the barriers?
In order to address the above research questions, following objectives have been framed:
To recognise potential sustainable 4D printing barriers that can help in realising adoption in automotive industry.
To derive priority order to identified barriers using MCDM approach in order to understand the step-by-step process of sustainable 4D printing adoption.
Literature review
The literature review with respect to 4D printing barriers and the related advancements is carried out as follows:
Choi et al. (Citation2015) performed review on 4D printing technology. The authors discussed recent advances in fabrication process and 3D printed materials development enabling fabrication of smart materials. Moreover, the study compared 3D and 4D printing by identifying integration factors. Further, the authors discussed applications of 4D printing technology. The study revealed that these novel technologies helped in reducing production time and human efforts to assemble the machines or products. Further, 4D printed structures also aid in fulfilling the customer requirement of customised designs or products. The study also found that 4D printing enable fabrication of multilateral parts with desired functionalities and adaptability.
Azhar and Pei (Citation2019) studied the relationship between 4D printing and manufacturing engineers and product designers through empirical studies. The study performed interviews and focussed group observation with six doctoral students of Brunel University. The research would help additive manufacturing and product engineers connect with each other in deploying 4D printing on parts.
González-Henríquez, Sarabia-Vallejos, and Rodriguez-Hernandez (Citation2019) reviewed 4D printing technology discussing its basic principles, advantages, disadvantages, printing mechanism, and most suitable polymer materials. In addition to this, the study also presented the limitations, features and properties of existing polymer-based additive manufacturing systems. The authors stated that 4D printed parts derived from 3D printing that is capable to vary with time. The study’s findings delivered an overview of most widely deployed methodologies to manufacture polymer-based additive manufactured parts. Further, shape memory alloys, smart polymeric materials, active polymer-based composites and hydrogels were considered at the centre of 4D printing. In the future, novel advancements in terms of materials and machines were suggested concerning 4D printing. Also, such translation from 3D to 4D was considered as one of the challenges in manufacturing system.
Carlson and Li (Citation2020) reported the kinetic assessment and development of low-cost-temperature-sensitive shape memory alloy for 4D printing. The study tested the filaments in fused deposition modelling process to develop 4D printed parts. The findings of the study revealed relationship amongst sample workpieces recovery rate, material concentration ratio and number of working cycles. Moreover, dynamic attributes such as angular velocity, angular acceleration, acceleration and recovery velocity were also examined to understand the shape memory polymer behaviour. The experimental analysis showed consistent fabricated filament with best shape memory attributes with 80% weight of PLA and 20% of TPU. The developed material also shown good shape recovery ratio and shape fixity. In future, similar research was suggested for other materials for 4D printing.
Liu and Chou (Citation2020) carried out an extensive review on past work with respect to material fabrication and characterisation using deposition process along with discussion on influence of process parameters for textile applications. Further, several approaches in 4D printing for attaining shape morphing overtime were reviewed. The study also observed several strategies for creating shape memory polymer based preforms using 4D printing. Some of the significant challenges and opportunities in developing multidirectional composites for textile parts were provided. In future, development of continuous fibre reinforced revealing high shape memory performance was suggested for 4D printing of multidirectional preforms.
Saritha and Boyina (Citation2021) executed a succinct literature review focusing on inception of 4D printing, applications, external stimuli response, conclusions and future opportunities. The authors mentioned 4D printing applications in tissue engineering, aerospace, organ engineering, medical technology and consumer products. The authors discussed two significant methods namely fused deposition modelling and stereolithography with regard to 4D printing. Further, 4D printing applications were shown in various fields such as clothing, automotive, healthcare, construction, defence and military.
Ahmed et al. (Citation2021) discussed the basics and governing laws of 4D printing and materials used in it. The study also presented several applications like soft robotics and impediments that need to overcome for its smooth adoption in manufacturing field. Factors such as mathematical modelling, interaction mechanism, smart materials, additive manufacturing processes and stimuli were considered as responsible for 4D printing. The authors emphasised on smart materials applications as it responds to external stimulus having behaviours such as self-healing, self-capability, shape memory and self-assembly. In future, discovery of more materials was suggested to fully utilise 4D printing process.
Mallakpour, Tabesh, and Hussain (Citation2021) discussed basic concept of 4D and 3D printing and their applications. The authors also mentioned about disadvantages of these technologies along with benefits. One disadvantage like low printing speed was mentioned that need to focus upon. The authors introduced 4D and 3D printing as novel technologies. The study shown significant applications of 4D printing in artificial organ production and other medical field. In future, discussion on challenges of 4D printing was recommended to explore.
Kumar et al. (Citation2021) performed a review on 4D printing of biomedical applications. The authors stated the significance of 4D printing in changing dimensions over time which helps in fabricating customised products for ear tissue, bone and muscle development. The study suggested to recognise the drawbacks of these technologies for smooth adoption in future.
Demoly et al. (Citation2021) aimed to emphasise potential barriers, status and challenges of 4D printing that need attention from a perspective of product-systems design. The authors made discussion on basics of smart materials, stimulus and additive manufacturing processes by highlighting the present status of 4D printing processes. The study further underlines challenges and opportunities of 4D printing from multiple viewpoints and developed a research framework for cross-disciplinary and engineering design. The findings of the study provided a research direction to the researchers for next decade towards smart product development.
Ameta et al. (Citation2022) carried out systematic review on advancements of 4D printing and 3D printing technologies and made discussion on significance of these technologies in bringing sustainability and revolution with respect to industrial developments. The authors reviewed 4D printing applications from several fields including drug delivery. The study mentioned 4D printing advantages as environmental-friendly, customised products fabrication, cost-effective, reliable, speedy process and accuracy.
Azhar and Pei (Citation2022) proposed theoretical framework for 4D printing by recognising the barriers of communication amongst engineers and designers in 4D printing parts design. In total, 60 designers and 50 engineers were involved to participate in the study. Findings of the study showed that 85% of participants were aware about 4D printing. Moreover, the study concludes that symbol analysis played significant role in ensuring easy understanding of 4D printing across all fields.
Based on the literature review, it can be seen that most of the studies critically reviewed 4D printing concept in order to derive challenges, current status, barriers and applications. The study conducted by Demoly et al. (Citation2021) aimed to emphasise potential barriers, status and challenges of 4D printing. One more study conducted by Ahmed et al. (Citation2021) discussed the basics and governing laws of 4D printing and materials used in it. Most of the studies focussed on applications of 4D printing in medical field. Moreover, Kumar et al. (Citation2021) and Mallakpour, Tabesh, and Hussain (Citation2021) suggested to explore the challenges of 4D printing for its smooth adoption. Ameta et al. (Citation2022) discussed about 4D printing and sustainability but in the form of review in medical field. Therefore, very few studies had been reported the barriers of sustainable 4D printing in automotive industry. In this viewpoint, the present study dealt with identification and evaluation of sustainable 4D printing barriers in the context of automotive component manufacturing organisation using MCDM tool.
Methodology
The methodological procedure of study is shown in .
Sustainable 4D printing adoption in automotive component manufacturing industry aids in creating environmentally conscious critical components. In order to address the issue of adoption of sustainable 4D printing, the present study identified 18 barriers and analysed against six criteria namely Build Time (C1), Accuracy (C2), Customization (C3), Carbon Footprint (C4), Material Utilization (C5) and Complexity (C6). The selection of barriers and criteria was made through extensive literature review and conversations with eminent experts from Industry and academia. Moreover, expert’s consultation was taken to shortlist the identified barriers of sustainable 4D printing. After finalising the barriers, the input sheet was prepared to gather expert opinion in the form of linguistic variables. These linguistic variables are further transformed into Grey numbers for analysis. The solution methodology used is G-TOPSIS. Using this approach, grey separation distance is computed which helped in deriving closeness coefficient. Grey number-based closeness coefficient is then converted into crisp number and the ranking of barriers was derived.
A flow chart depicting the flow of methodology which initiates with literature review on barriers of sustainable 4D printing. Further, finalisation of barriers are done using experts opinion followed by analysis of identified barriers using Grey-TOPSIS approach. The stability of derived ranking is ensured through sensitivity analysis. Finally, results, discussions, implications and conclusions are derived.
Grey TOPSIS approach
Very few studies focused on analysing sustainable 4D printing barriers using MCDM approaches. Most popular MCDM approaches includes analytical hierarchical process, fuzzy-based decision-making techniques, data envelopment analysis, TOPSIS etc. These approaches have been integrated with fuzzy theory to reduce the inconsistencies in decision-making process. However, in the literature, Prakash et al. (Citation2022), the authors utilised Grey-TOPSIS approach to capture the sustainable supply chain collaboration. Grey-based technique helped in overcoming ambiguity and vagueness in selection procedure and specifically during early stage of data gathering. Similar to other MCDM approaches, Grey TOPSIS approach also possesses few benefits and limitations. Benefits includes relative mathematical transparency and easy to use. It can be very operative in handling variety of factors. Moreover, Grey TOPSIS approach can provide effective solution with limited decision maker involvement. Acknowledging these benefits, the present study utilises Grey TOPSIS approach for analysis.
Theoretical background of Grey numbers
The Grey theory concept is based on Grey numbers (Deng Citation1989; Ju-Long Citation1982). Grey numbers can be utilised for several systems possessing incomplete, inadequate and discrete information with uncertainties (Bai and Sarkis Citation2018; Feng et al. Citation2019). Fundamentally, white system is with complete known information and black system with unknown information; however, if partial information is available with the system is termed as grey system (Badi, Bouraima, and Jibril Citation2022; Dey and Chakraborty Citation2016). Fundamental concept and definitions of Grey number could be referred from Dey and Chakraborty (Citation2016).
Suppose and
be two grey numbers with
, and
.
The greater degrees for interval grey numbers are specified as:
if , then the grey number
is greater than
(Bai and Sarkis Citation2018). These relationships aid in advancing TOPSIS approach.
The adjusted weight for each criterion C and expert E can be computed using below Equation 2 (Malaga and Vinodh Citation2021):
The average adjusted criteria significance value is computed as:
Equation 3 signifies the average adjusted criteria significance weight of ith criteria of all experts. Further, for realising the effect of barriers against each criterion, the aggregated unadjusted grey values have been computed. Based on the number of decision makers E, grey values are computed as shown in Equation 4:
Further, adjusted aggregate grey values of barriers for each criterion are calculated using Equation 5 as:
TOPSIS approach
TOPSIS is applied to rank the identified barriers regarding adoption of sustainable 4D printing in automotive components manufacturing scenario. Various Multi-Criteria Decision Making (MCDM) techniques are established to deal with the unstructured problems containing several and contradictory goals, but TOPSIS approach is most widely used method. This method assumes two artificial hypotheses as follows:
H1 Ideal alternative: hypothesis having the best level of all the barriers identified.
H2 Negative ideal alternative: hypothesis having worst level of all barriers identified.
TOPSIS chooses the closest alternative to the positive ideal solution and furthest alternative from negative ideal solution.
The methodological stages of GTOPSIS are (Bai and Sarkis Citation2018; Dey and Chakraborty Citation2016):
To select set of criteria explaining alternatives.
To create decision matrix
. In this matrix,
signify the grey assessment of ith alternative with regards to jth criterion.
Computing normalising decision matrix.
To compute criteria weights wj.
To create the grey weighted normalised decision matrix.
Recognize the positive and negative ideal solutions.
(6)
(6)
(7)
(7)
where represents index value of highest Performance and
represents index value of lowest performance.
(7) Computation of grey separation measures from positive and negative ideal solutions.
where represents positive ideal solution and
represents negative ideal solution.
(8) To compute relative closeness coefficient and derive ranking of the barriers.
where represents closeness coefficient.
Case study
Manufacturing organisations particularly in the automotive sector are expecting to develop sustainable products through additive manufacturing. The automotive organisations are moving towards embracing Industry 4.0 and planning for its smooth adoption in order to develop digital technological hub in organisation. In additive manufacturing, 4D printing is gaining much importance due to its ability of self-repair, self-assembly and multifunctionality. Moreover, organisations are struggling to improve production efficiency which can be achieved through adoption of 4D printing. However, adoption of sustainable 4D printing possess several barriers in organisation that need significant attention. In this viewpoint, the case organisation approached with a problem statement of recognising sustainable 4D printing barriers that can help industry in effortless implementation of it. Therefore, in line with organisation goals, an extensive literature was done to identify possible barriers of sustainable 4D printing and analysed it using MCDM approach. This work aims to examine identified sustainable 4D printing barriers using Grey TOPSIS approach and derive priority order to realise significant barrier.
Selection of sustainable 4D printing barriers and criteria
With the help of extensive literature review, possible barriers of sustainable 4D printing were identified related to automotive component organisation. From identified barriers, 18 barriers and 6 criteria were finalised in discussion with academia and industrial experts. Considering the expertise on 4D printing and sustainability, a group of three experts from automotive component manufacturing industry and academic institution is formed. The first expert was a strategic manager in the case automotive component organisation, whereas the second expert was selected from an associated automotive organisation working at a senior level position. Both the experts had rich expertise in additive manufacturing and aimed to focus on 4D printing process. The third expert was chosen from an academic institute serving at professor level position and possessing expertise in Industry 4.0 and additive manufacturing. The experts were serving at strategic managerial positions having more than 10 years of valuable experience 3D printing and manufacturing automation. The industry experts are responsible for carrying out tactical decision-making with respect to product innovation and fabrication. The academia expert performed several studies with respect to concept implementation and helped industries in smooth adoption of it. Further, a brainstorming session was done with experts to realise the present situation of the organisation. The experts utilised their rich experience in finalising the barriers of sustainable 4D printing. depicts a description of sustainable 4D printing barriers finalised through expert opinion.
Table 1. Barriers of sustainable 4D printing and their description.
The experts inputs are gathered for criteria and barriers analysis using linguistic variables and presented in .
Table 2. Experts inputs for criteria and barriers analysis.
Calculations using G-TOPSIS approach
The experts opinion of linguistic form has been transformed into grey numbers as shown in (Feng et al. Citation2019; Malaga and Vinodh Citation2021; Prakash et al. Citation2022). Moreover, the experience is also given weightage by considering its importance level as depicted in . In this study, based on the experience of experts, higher weightage is assigned to all experts, that is Very Important [0.9, 1.0] for the analysis.
Table 3. Criteria weights greyscale.
Table 4. Greyscale for barriers rating.
Table 5. Experts importance scale.
Calculation of criteria weight
The adjusted importance weight of each criterion is computed using EquationEquation 2(2)
(2) . Further, final average criteria weight is calculated using EquationEquation 3
(3)
(3) as shown in .
Table 6. Adjusted importance weights of criteria.
Calculation of unadjusted aggregate grey values of barriers on each criterion
Here, unadjusted aggregate grey values of barriers for each criterion are computed using Equation 4. The calculation of unadjusted aggregate grey value for Barrier 1 ‘Lack of availability of materials that respond in real-time’ with respect to Criteria 1 ‘Build Time’ is shown below:
Similarly, unadjusted aggregate grey values for all barriers are computed and presented in .
Table 7. Unadjusted aggregate grey values of barriers with respect to criteria.
Calculation of adjusted aggregate barriers grey values
Findings of unadjusted grey values are further converted into adjusted aggregated grey values for all barriers using EquationEquation 5(5)
(5) . Sample computation of Barrier 1 is shown below:
For Barrier 1, ‘Lack of availability of materials that respond in real-time’ with respect to Criterion 1 ‘Build Time’ is:
The adjusted aggregated grey values of all barriers are shown in .
Table 8. Adjusted aggregated barriers grey values.
Results and discussion
The present study intended to prioritise sustainable 4D printing barriers pertaining to automotive component manufacturing organisation using G-TOPSIS approach. Based on the G-TOPSIS analysis, provides aggregated adjusted grey values of each barrier with respect to each criterion. In continuation of the same, Positive ideal and Negative ideal solutions for each criterion have been computed. The computation of positive and negative ideal solution has been done by referring to the EquationEquations 6(6)
(6) and Equation7
(7)
(7) . shows Positive and Negative Ideal solutions for each criterion.
Table 9. Positive and negative ideal solutions of criteria.
Calculation of Grey separation measure and closeness coefficient
Using positive and negative ideal solutions, grey separation distance from positive ideal and negative points for each barrier is computed. EquationEquations 8(8)
(8) and Equation9
(9)
(9) have been utilised to compute grey separation measure for each barrier.
Sample Calculation of grey separation measure for first barrier ‘Lack of availability of materials that respond in real-time (B1)’
Similarly, grey separation measures for all the barriers have been computed. shows the grey separation measure of barriers.
Table 10. Closeness coefficient and Grey separation measure of barriers.
Table 11. Final priority order of barriers.
EquationEquation 10(10)
(10) has been utilised to compute Grey closeness coefficient.
Sample Calculation
For barrier ‘Lack of availability of materials that respond in real-time (B1)’:
Grey Closeness Coefficient = [
),
)] = (0.084,0.463)
Similarly, Grey closeness coefficient has been computed to derive the priority order of all the barriers as shown in .
Ranking of barriers
Since the closeness coefficient value is in grey form, it becomes difficult to rank the barriers. The grey numbers have been converted into crisp values and ranking of barriers is derived as depicted in .
Sample Calculation
For Barrier ‘B1’: Closeness Coefficient (Average) = (0.084 + 0.463)/2 = 0.2735.
In a similar way, average closeness coefficient value for all barriers is computed and ranking is derived.
With reference to , ranking order of the sustainable 4D printing barriers is ‘Improper disposal strategy (B18)’ > ‘Lack of interaction between smart materials and 3D printing technology (B8)’ > ‘Lack of Smart materials compatibles with AM technologies (B10)’ > ‘Environmental-friendly technologies (B17)’ > ‘Lack of availability of materials that respond in real-time (B1)’ > ‘Synthetic versatility and adaptability of polymers (B4)’ > ‘Lack of dynamic response for structures and systems (B6)’ > ‘Consolidating technical functions with multi-material and multi-functional objects (B13)’ > ‘Difficulty in inducing anisotropy by the layer-by-layer deposition mode (B11)’ > ‘Lack of design innovation with AM approaches for multi-material, transformation, and multifunctional skills through 4D printing (B15)’ > ‘Requirement of Designing of the smart structures (B9)’ > ‘Lack of awareness about 4D printing benefits (B3)’ > ‘Requirement of additional developments to meet robust system (B5)’ > ‘Need of programming for materials responsive towards moisture due to slow reverse response (B16)’ > ‘Inconvenience in creating complicated shapes (B2)’ > ‘Degradation of smart materials with continuous deformation (B7)’ > ‘Integrating multi-material functionality and technical with user-interface, user-centric, systems functions and user-experience (B14)’ > ‘Requirement of post-processing step (B12)’. ‘Improper disposal strategy (B18)’ is found to be most influential barriers in adoption of sustainable 4D printing.
Sensitivity analysis
Sensitivity analysis aids in stabilising the identified ranking of alternatives. The reason being performing sensitivity analysis is variation in expert opinion on criteria. Therefore, in order to address this issue of variation, several scenarios, that is experiments need to be performed by interchanging weights of criteria (Bai and Sarkis Citation2018; Malaga and Vinodh Citation2021). shows the interchanged weights considered for sensitivity analysis. Using these weights, the ranking of barriers for each experiment obtained is depicted in .
Table 12. Experiment-wise weights for each criterion.
Table 13. Sensitivity analysis.
A clustered column chart of average closeness coefficient of each barrier with respect to each experiment performed for sensitivity analysis. Clustered columns show different experiments ensuring stability of ranking of sustainable 4D printing barriers.
Sensitivity analysis has been carried out to understand the ranking stability of obtained results. Sensitivity analysis ranking stability has been shown in . In total, six experiments (Exp.) were carried out. In each experiment, criteria weights were interchanged, and was obtained. It can be clearly seen from that ranking stability of barriers obtained from average closeness coefficient is achieved. Thus, the findings of the study have been proved to be stable.
Implications
The present study analysed sustainable 4D printing adoption barriers in Indian automotive component organisation using G-TOPSIS technique. Eighteen potential barriers hindering 4D printing adoption in organisation are recognised in discussion with eminent experts and extensive literature review. Six criteria were used for the analysis and derived priority order of barriers. The outcomes from the study recommended to concentrate on disposal strategy with regard to 3D printing ensuring sustainability. 4D printing produces less waste compared to 3D printing as minor adjustments can be immediately made in software to avoid the printing issues. However, organisations need to focus on appropriate disposal strategy for adoption of 4D printing. The study also provides enough understanding on 4D printing and sustainability that would help industry practitioners to create awareness about it. The study further recommends developing sufficient finance for adoption of 4D printing in order to achieve its long-term benefits. The barriers ‘Lack of interaction between smart materials and 3D printing technology (B8)’, ‘Lack of Smart materials compatibles with AM technologies (B10)’, Environmental-friendly technologies (B17)’ and ‘Lack of availability of materials that respond in real-time (B1) also need focus for taking advantage of 4D printing. Moreover, automotive manufacturing can develop short-term strategy to transform towards 4D printing using given priority order of barriers. Industry practitioners recognised the significance of the present approach of deriving ranking of barriers and taken necessary steps towards implementation of it.
Conclusions
Sustainability is gaining much attention over the last decade. It delivers understanding on energy and resource consumption which in turn reduces material quantity requirement in supply chain and ensures sustainable practices in manufacturing processes. The present study aimed to examine barriers of sustainable 4D printing and analysed using Grey TOPSIS approach. In total,18 barriers were identified through literature review and expert consultation and analysed against six criteria. Examination of each barrier was done through several rounds of discussion with the experts and gathered their opinion as per Grey TOPSIS methodology. Post data collection, Grey TOPSIS computations was done and derived priority order. The study outcomes showed that barrier ‘Improper disposal strategy (B18)’ as the most influential one that need to overcome for smooth adoption of sustainable 4D printing followed by ‘Lack of interaction between smart materials and 3D printing technology (B8)’, ‘Lack of Smart materials compatibles with AM technologies (B10)’, ‘Environmental-friendly technologies (B17)’ and ‘Lack of availability of materials that respond in real-time (B1)’. The study delivered sufficient understanding on 4D printing and sustainability that would help industry practitioners to build awareness about it.
Findings from the study steered the industry practitioners to take suitable measures for adoption of 4D printing. This study holds certain limitations that may act as opportunities for conducting future research. The study identified 18 sustainable 4D printing barriers and six criteria with respect to Indian automotive manufacturing organisation; in future, additional barriers could be included in line with technological advancements of additive manufacturing. Also, the life cycle assessment studies of as built product of 4D printing could be done. The present study deployed Grey TOPSIS approach for evaluation of barriers; in future integrated MCDM approaches could be used with additional barriers and criteria for deeper realisation of 4D printing adoption strategy. Moreover, future researchers may focus on performing comparative analysis of this study with other MCDM approaches. Since, the present study focusses on sustainable 4D printing barriers, future researchers may attempt to develop sustainable guidelines for 4D printing component and derive priority order of such guidelines. The structural model could also be developed for sustainable 4D printing barriers depicting hierarchical adoption strategy for industry. Furthermore, 4D printing barriers could be mapped with circular economy strategies and sustainable framework could be developed.
Disclosure statement
No potential conflict of interest was reported by the author(s).
Additional information
Notes on contributors
Vishal Ashok Wankhede
Vishal Ashok Wankhede is an Assistant Professor in the Department of Operations Management and Quantitative Techniques, Indian Institute of Management, Bodhgaya since July 2023. Prior to joining IIM Bodhgaya, he worked as an Assistant Professor in Department of Mechanical Engineering, School of Technology, PDEU Gandhinagar from March 2022 to June 2023. He also holds three years of teaching experience as an Assistant Professor in Department of Industrial Engineering, Pandit Deendayal Energy University, Gandhinagar from July 2016 – June 2019. He received his Ph.D.in the area of Industry 4.0 from Department of Production Engineering, National Institute of Technology, Tiruchirappalli in July 2022. He received his M.Tech in the area of Cloud Manufacturing from Department of Production Engineering, National Institute of Technology, Tiruchirappalli, Tamilnadu in 2016. He received his B.Tech in Mechanical Engineering from Dr. Babasaheb Ambedkar Technological University, Lonere, Raigad, Maharashtra (Govt. Autonomous), Maharashtra in 2014. He has also received “Budding Researcher Award” on Institute Day 2022 of NIT Tiruchirappalli for the outstanding research performance during Ph.D. (July 2019 – March 2022) at Department of Production Engineering, NIT Tiruchirappalli. He is actively associated with various professional bodies such as Institution of Engineers (India), Indian Society for Technical Education, American Society of Mechanical Engineers and Indian Institution of Industrial Engineering. His research area includes Industry 4.0, Smart Manufacturing, Additive manufacturing, Circular economy, Sustainable Manufacturing etc.
S. Vinodh
S. Vinodh is a Professor in the Department of Production Engineering, National Institute of Technology, Tiruchirappalli, Tamil Nadu, India. He completed his PhD degree under AICTE National Doctoral Fellowship Scheme from PSG College of Technology, Coimbatore, India. He completed his Master’s in Production Engineering from PSG College of Technology, Coimbatore, India and Bachelor’s in Mechanical Engineering from Government College of Technology, Coimbatore, India. He has published over 175 papers in international journals. He received Highly Commended Paper Award from Emerald Publishers for the year 2016. He is the recipient of Innovative Student Project Award 2010 based on his PhD thesis from Indian National Academy of Engineering (INAE), New Delhi, India. His research interests include sustainable manufacturing, lean manufacturing, agile manufacturing, rapid manufacturing, product development and Industry 4.0.
References
- Ahmed, A., S. Arya, V. Gupta, H. Furukawa, and A. Khosla. 2021. “4D Printing: Fundamentals, Materials, Applications and Challenges.” Polymer, Elsevier Ltd 228 (May): 123926. https://doi.org/10.1016/j.polymer.2021.123926.
- Ameta, K. L., V. S. Solanki, S. Haque, V. Singh, A. P. Devi, and R. S. Chundawat. 2022. “Critical Appraisal and Systematic Review of 3D & 4D Printing in Sustainable and Environment-Friendly Smart Manufacturing Technologies.” Sustainable Materials and Technologies e00481. https://doi.org/10.1016/j.susmat.2022.e00481.
- Azhar, F. E., and E. Pei 2019, “Investigating the Communication of 4D Printing Among Product Designers and Manufacturing Engineers.” https://bura.brunel.ac.uk/bitstream/2438/18701/1/FullText.pdf.
- Azhar, F. E., and E. Pei. 2022. “A Conceptual Framework to Improve the Symbol Implementation of 4D Printing Communication Between Designers and Engineers.” Designs 6 (1): 3. https://doi.org/10.3390/designs6010003.
- Badi, I., M. B. Bouraima, and M. L. Jibril. 2022. “Risk Assessment in Construction Projects Using the Grey Theory.” Journal of Engineering Management and Systems Engineering 1 (2): 58–66. https://doi.org/10.56578/jemse010203.
- Bai, C., and J. Sarkis. 2018. “Integrating Sustainability into Supplier Selection: A Grey-Based Topsis Analysis.” Technological and Economic Development of Economy 24 (6): 2202–2224. https://doi.org/10.3846/tede.2018.5582.
- Carlson, M., and Y. Li. 2020. “Development and Kinetic Evaluation of a Low-Cost Temperature-Sensitive Shape Memory Polymer for 4-Dimensional Printing.” The International Journal of Advanced Manufacturing Technology 106 (9–10): 4263–4279. https://doi.org/10.1007/s00170-020-04927-5.
- Choi, J., O. C. Kwon, W. Jo, H. J. Lee, and M. W. Moon. 2015. “4D Printing Technology: A Review.” 3D Printing and Additive Manufacturing 2 (4): 159–167. https://doi.org/10.1089/3dp.2015.0039.
- Demoly, F., M. L. Dunn, K. L. Wood, H. J. Qi, and J. C. André. 2021. “The Status, Barriers, Challenges, and Future in Design for 4D Printing.” Materials & Design 212:110193. https://doi.org/10.1016/j.matdes.2021.110193.
- Deng, J.-L. 1989. “Introduction to Grey System.” Journal of Grey System 1 (1): 1–24.
- Dey, S., and S. Chakraborty. 2016. “A Study on the Machinability of Some Metal Alloys Using Grey Topsis Method.” Decision Science Letters 5 (1): 31–41. https://doi.org/10.5267/j.dsl.2015.9.002.
- Feng, Y., Z. Zhang, G. Tian, A. M. Fathollahi-Fard, N. Hao, Z. Li, W. Wang, and J. Tan. 2019. “A Novel Hybrid Fuzzy Grey TOPSIS Method: Supplier Evaluation of a Collaborative Manufacturing Enterprise.” Applied Sciences (Switzerland) 9 (18): 3770. https://doi.org/10.3390/app9183770.
- González-Henríquez, C. M., M. A. Sarabia-Vallejos, and J. Rodriguez-Hernandez. 2019. “Polymers for Additive Manufacturing and 4D-Printing: Materials, Methodologies, and Biomedical Applications.” Progress in Polymer Science 94:57–116. https://doi.org/10.1016/j.progpolymsci.2019.03.001.
- Jian, B., F. Demoly, Y. Zhang, H. J. Qi, J. C. André, and S. Gomes. 2022. “Origami-Based Design for 4D Printing of 3D Support-Free Hollow Structures.” Engineering, Chinese Academy of Engineering 12:70–82. https://doi.org/10.1016/j.eng.2021.06.028.
- Ju-Long, D. 1982. “Control Problems of Grey Systems.” Systems & Control Letters. Elsevier 1 (5): 288–294. https://doi.org/10.1016/S0167-6911(82)80025-X.
- Kumar, S. B., J. Jeevamalar, P. Ramu, G. Suresh, and K. Senthilnathan. 2021. “Evaluation in 4D Printing - a Review.” Materials Today: Proceedings. Elsevier Ltd 45:1433–1437. https://doi.org/10.1016/j.matpr.2020.07.335.
- Laverne, F., R. Marquardt, F. Segonds, I. Koutiri, and N. Perry. 2019. “Improving Resources Consumption of Additive Manufacturing Use During Early Design Stages: A Case Study.” International Journal of Sustainable Engineering, Taylor & Francis 12 (6): 365–375. https://doi.org/10.1080/19397038.2019.1620897.
- Liu, Y., and T. W. Chou. 2020. “Additive Manufacturing of Multidirectional Preforms and Composites: From Three-Dimensional to Four-Dimensional.” Materials Today Advances, Elsevier Ltd 5:100045. https://doi.org/10.1016/j.mtadv.2019.100045.
- Malaga, A., and S. Vinodh. 2021. “Benchmarking Smart Manufacturing Drivers Using Grey TOPSIS and COPRAS-G Approaches.” Benchmarking. Emerald Publishing Limited 28 (10): 2916–2951. https://doi.org/10.1108/BIJ-12-2020-0620.
- Mallakpour, S., F. Tabesh, and C. M. Hussain. 2021. “3D and 4D Printing: From Innovation to Evolution.” Advances in Colloid and Interface Science 294 (June): 102482. https://doi.org/10.1016/j.cis.2021.102482.
- Maraveas, C., I. S. Bayer, and T. Bartzanas. 2022. “4D Printing: Perspectives for the Production of Sustainable Plastics for Agriculture.” Biotechnology Advances. Elsevier Inc 54 (May 2021): 107785. https://doi.org/10.1016/j.biotechadv.2021.107785.
- Nugroho, W. T., Y. Dong, A. Pramanik, J. Leng, and S. Ramakrishna. 2021. “Smart Polyurethane Composites for 3D or 4D Printing: General-Purpose Use, Sustainability and Shape Memory Effect.” Composites Part B: Engineering, Elsevier Ltd 223 (March): 109104. https://doi.org/10.1016/j.compositesb.2021.109104.
- Prakash, S., S. Kumar, G. Soni, V. Jain, S. Dev, and C. Chandra. 2022. “Evaluating Approaches Using the Grey-TOPSIS for Sustainable Supply Chain Collaboration Under Risk and Uncertainty.” Benchmarking Emerald Publishing Limited. Vol. ahead-of-p. No. ahead-of-print available at: https://doi.org/10.1108/BIJ-05-2022-0319.
- Saritha, D., and D. Boyina. 2021. “A Concise Review on 4D Printing Technology.” Materials Today: Proceedings. Elsevier Ltd 46:692–695. https://doi.org/10.1016/j.matpr.2020.12.016.
- Trenfield, S. J., A. Awad, C. M. Madla, G. B. Hatton, J. Firth, A. Goyanes, S. Gaisford, et al. 2019. “Shaping the Future: Recent Advances of 3D Printing in Drug Delivery and Healthcare.” Expert Opinion on Drug Delivery Taylor & Francis 16 (10): 1081–1094. https://doi.org/10.1080/17425247.2019.1660318.
- Wankhede, V. A., and S. Vinodh. 2021. “Application of Total Interpretive Structural Modeling for Analyzing Factors of Additive Manufacturing and Industry 4.0 Integration.” Rapid Prototyping Journal 27 (8): 1591–1608. https://doi.org/10.1108/RPJ-02-2021-0028.