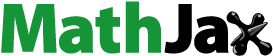
ABSTRACT
Antibody-based immune checkpoint blockade (ICB)-based therapeutics have become effective clinical applications for cancers. Applications of monoclonal antibodies (mAbs) to de-activate the PD-1-PD-L1 pathway could effectively reverse the phenotype of depleted activated thymocytes (T cells) to recover their anti-tumoral activities. High-resolution structures of the complexes of the therapeutic monoclonal antibodies with PD-1 or PD-L1 have revealed the key inter-molecular interactions and provided valuable insights into the fundamental mechanisms by which these antibodies inhibit PD-L1-PD-1 binding. Each anti-PD-1 mAb exhibits a unique blockade mechanism, such as interference with large PD-1-PD-L1 contacting interfaces, steric hindrance by overlapping a small area of this site, or binding to an N-glycosylated site. In contrast, all therapeutic anti-PD-L1 mAbs bind to a similar area of PD-L1. Here, we summarized advances in the structural characterization of the complexes of commercial mAbs that target PD-1 or PD-L1. In particular, we focus on the unique characteristics of those mAb structures, epitopes, and blockade mechanisms. It is well known that the use of antibodies as anti-tumor drugs has increased recently and both PD-1 and PD-L1 have attracted substantial attention as target for antibodies derived from new technologies. By focusing on structural characterization, this review aims to aid the development of novel antibodies targeting PD-1 or PD-L1 in the future.
Introduction
The introduction of immune checkpoint blockade (ICB)-based cancer therapeutic approaches has resulted in substantial changes in the clinical treatment paradigm.Citation1–3 One of the key molecular mechanisms of ICB therapy involves targeting of the immune suppression PD-1-PD-L1 pathway. PD-1 is localized on the cell surface of activated immune T-cells,Citation4 whereas its natural ligand PD-L1 is highly expressed on the surface of tumor cells.Citation5–7 Recognition between PD-1 and PD-L1 activates the PD-1/PD-L1 pathway to reduce the activity of the T-cell. Many types of cancer exploit these interactions to escape immune surveillance.Citation7–10
MAbs that bind PD-1 or PD-L1 have been successfully used to suppress the cancer cells’ ability to evade immune surveillance.Citation11,Citation12 The mAbs bind to PD-1 or PD-L1, block their recognition, and thus re-activate the tumor-specific T cells to kill cancer cells.Citation13,Citation14 As of mid-2022, 16 mAbs that target the PD-1-PD-L1 pathway had been approved by the Food and Drug Administration (FDA; USA) or the National Medical Products Administration (NMPA; China) as therapeutics for cancer. Of these, 11 are anti-PD-1 mAbs and 5 are anti PD-L1 antibodies.Citation15–29 Aside from envafolimab (KN035), which is a camelid-derived single-domain antibody (i.e., nanobody), all of the other above-mentioned therapeutic antibodies are full-length mAbs. Further details of these antibodies, including their manufacturers and the indications for which they are approved, are listed in .
Table 1. List of the programmed cell death protein 1 (PD-1), programmed cell death ligand 1 (PD-L1) and related structures deposited in the protein data bank.
Structural characterization of the epitopes on PD-1 or PD-L1 that underpin PD-1-mAb and PD-L1-mAb complexes provides valuable insights into the mechanisms by which these antibodies block the inhibitory signaling pathway of PD-1 and PD-L1.Citation48,Citation49 High-resolution structures of the complex of the mAbs with PD-1 or PD-L1 have been obtained to unravel the mechanisms of these antibodies.Citation33–Citation50–61 The mechanisms include a mAb’s binding plane blocking a large area of the PD-L1-binding site of PD-1,Citation33 or the mAb generating steric hindrance by overlapping a small area of this siteCitation36 or binding to an N-glycosylated site.Citation40 In contrast, most approved anti-PD-L1 mAbs bind PD-L1 at very similar sites (on the pre-β-sheet), and they all directly compete with PD-1.Citation44–62–Citation64 The aforementioned structural findings will aid the future rational design of novel and more effective antibodies.
Herein, we review advances in structural studies of complexes formed between FDA- or NMPA-approved antibodies and PD-1 or PD-L1. We focus on the nature of PD-1-PD-L1 interactions, key contacts that stabilize the interactions between each mAb and PD-1 or PD-L1, and the mechanism by which each mAb blocks PD-1–PD-L1 interactions. An earlier work published in 2019 has reviewed the structure of PD-1-PD-L1 complex and the structures of mAbs complex with PD-1 or PD-L1.Citation58 As more antibodies targeting PD-1 or PD-L1 were approved during 2019 to mid-2021, we collected data for all these new approved antibodies and provided a more comprehensive summarization on the complex structures of mAbs with PD-1 or PD-L1.
Anti-PD-1/PD-L1 antibodies
Preparation of samples for the structural characterization of PD-1–mAb or PD-L1–mAb complexes
Most of the FDA- and/or the NMPA-approved therapeutic antibodies that specifically bind PD-1 or PD-L1 for cancer therapy are full-length immunoglobulin G (IgG) mAbs ().Citation65,Citation66 The isotypes (IgG1 or IgG4) of each mAb included here are summarized in . The crystal structures of the complex of PD-1 or PD-L1 with a range of approved antibodies have been reported.Citation58–67–Citation71 Because the full-length antibodies are typically too flexible to be crystallized, Fab or scFvs of mAbs have commonly been used to bind with PD-1 or PD-L1 to afford a crystallizable complex. A Fab domain is composed of four folded domains: a variable domain from light chain and heavy chain (VL and VH), and a constant domain from light chain and heavy chain (CL and CH1). The scFv is a fusion protein of a VH and a VL , which is a complete and smallest functional unit.Citation72 ()
Figure 1. Topology of monoclonal antibody and the scFv fragment.

Table 2. Details of antibodies.
The Fab regions or scFvs of mAbs are usually prepared by one of the three methods. Fab regions are prepared by enzymatic digestion of full-length antibodies. For example, papain degrades IgGs into two Fab regions and one Fc region, and the Fab regions can be further purified for structural studies.Citation121 Liu and colleagues used this approach to crystallize the complex of Fab region of toripalimab with PD-1, which in turn determines the complex structure.Citation41
Recombinant co-expression of a mAb’s VH and VL is an alternative approach in Fab preparation. DNA sequences encoding the VH and the VL are constructed in different plasmids, which are then co-transfected into expression hosts that subsequently co-express the VH and VL domains. This generates a random combination of VH and VL domains that form a library of VH–VL complexes,Citation122 which are screened to determine the optimal constructs for structural characterization. This approach has been used to determine the structures of the complexes of PD-1 with pembrolizumab-Fab,Citation33 nivolumab-Fab,Citation36 tislelizumab-FabCitation71 and atezolizumab-Fab.Citation43 Using the third method, scFv recombinant expression plasmids are constructed to contain the genes of an antibody’s VL and VH domains that are linked by an oligopeptide to form an scFv ().Citation123 This method has been used to express and crystallize camrelizumab,Citation40 avelumabCitation46 and durvalumab.Citation45 The second and third methods have obvious advantages in obtaining a Fab region or an scFv from a prokaryotic expression system, i.e., E. coli expression systems, and have been the most commonly used methods to obtain Fab regions or scFvs for structural studies.
Structures of PD-1–PD-L1 and PD-1–mAbs complexes
PD-1 and PD-L1 interact and form stable complex. The interface of an mAb and PD-1 or PD-L1 partially or fully overlaps with those of PD-1-PD-L1 complex, resulting in interfacial conflicts and partial steric hindrance that perturb the recognition of PD-L1 with PD-1.Citation124,Citation125 Examples of the reported structures are shown in .
Figure 2. Presentation of structures of PD-1-PD-L1 complex and PD-1-antibody complexes.

Structure of PD-1–PD-L1
PD-1 has an extracellular domain comprised by nine β-strands (A, A’, B, C, C’, D, E, F, G), folding into a β-sandwich Ig topology.Citation4,Citation36,Citation126 It also has eight loops connecting the -strands, and a flexible loop at the N-terminus ().Citation30,Citation34 PD-1 contains multiple N-glycosylated sites,Citation36 which affect the binding of certain antibodies.Citation40 Similar to PD-1, PD-L1 also has nine β-strands (A, B, C, C’, C’’, D, E, F, G) and the loops connecting them to fold into an extracellular Ig V domain.Citation44,Citation46 The structures of PD-1–PD-L1 complexes have been determined using PD-1 and PD-L1 from different species ().Citation30,Citation32 These structures have revealed that PD-1 and PD-L1 are oriented nearly orthogonal to each other (). PD-1 and PD-L1 associate mainly via hydrophobic interactions between residues on the front faces of both proteins ().Citation30 The inter-molecular H-bonds and π–π stacking are other key driving forces that stabilize PD-1–PD-L1 complexes.Citation30 The mAbs mainly compete within this interface to block the PD-1-PD-L1 interactions.
Figure 3. The structure of PD-1-PD-L1.

Structure of PD-1–camrelizumab-scFv
Anti-PD-1 camrelizumab was developed by Jiangsu Hengrui Medicine Co., Ltd. It is approved by the NMPA for the treatment of classic Hodgkin’s lymphoma, hepatocellular carcinoma, esophageal squamous cell carcinoma, nasopharyngeal carcinoma and non-squamous non-small-cell lung cancer (NSCLC).Citation21–86–Citation88–127 Gao and colleagues determined the structure of the PD-1-camrelizumab-scFv complex (PDB code: 7CU5) using a VL-(glycine)4–serine-VH construct of camrelizumab.Citation40 All three heavy chain complementarity-determining regions (HCDRs) and two of three light chain (LC) CDRs of camrelizumab form contacts with PD-1 (). Three loops of PD-1 constitute several inter-molecular H-bonds that are crucial for the complex stability, such as the H-bonds between the LCDR3 of camrelizumab and the FG loop of PD-1, and between the HCDR2 of camrelizumab and the BC loop of PD-1. The interface between PD-1 and camrelizumab-scFv partially overlaps with that of PD-1 and PD-L1, indicating that camrelizumab prevents the PD-L1-PD-1 interaction.
Figure 4. Illustration of the importance of the glycosylation of PD-1 in binding with camrelizumab and cemiplimabCitation37.

PD-1 contains several N-linked glycosylation sites, which can be modified with a glycan containing two NAGs, two MANs and one FUC. N-glycosylation modification on PD-1 has a pronounced effect on camrelizumab binding. The glycosylation of N58 was found to strengthen the camrelizumab-PD-1 binding. The absence of N58-glycoslyation, which can be achieved via a N58A single point mutation of PD-1 that eliminates the N-glycosylation site, largely reduced its binding affinity with camrelizumab ().Citation40 The structure of the PD-1–camrelizumab-scFv demonstrated the van der Waals contacts between the glycan chains on N58 and several residues of camrelizumab, such as the S30 of HCDR1 and the G54 and A56 of HCDR2. Moreover, FUC and MAN in the glycan chain form H-bonds with the S31 of HCDR1 and the G53 of HCDR2 (). These contacts between the glycan and the PD-1 make significant contributions to the stability of the PD-1–camrelizumab-scFv complex. Camrelizumab showed some low-affinity binding to other human receptors, leading to side effects in clinical trials, e.g., reactive capillary endothelial proliferation. The low-affinity binding between camrelizumab and other receptors may be correlated with its glycosylation-dependent high-affinity binding to PD-1.
In addition to camrelizumab, a recent publication has shown that glycosylation of PD-1 also affects its binding with cemiplimab, a human IgG4 mAb targeting PD-1 developed by Sanofi and Regeneron.Citation37 The interaction surface of PD-1-cemiplimab-scFv is highly similar to that of PD-1-camrelizumab-scFv. The glycosylation of N58 in PD-1 also promotes the PD-1-cemiplimab-scFv binding.Citation37 The HCDR2 of cemiplimab interacts with N58 glycosylation of PD-1.
Structure of PD-1–toripalimab-Fab
Developed by Shanghai Junshi Biosciences Co., Ltd, toripalimab was approved by the NMPA in 2018. Toripalimab is currently indicated as a treatment for melanoma, nasopharyngeal cancer, urothelial cancer, and NSCLC.Citation91–128–Citation130 The PD-1–toripalimab-Fab structure showed that an unusually long HCDR3 (18 amino acids) at the VH domain of toripalimabCitation41 (), which is longer than the HCDR3 of any other anti-PD-1 mAb that has been characterized. This long HCDR3 and the LCDR1 and LCDR3 of toripalimab form multiple H-bonds with the residues on the FG loop of PD-1, as key binding factors (). In addition, van der Waals interactions between the HCDR1, HCDR2 and LCDR1 of toripalimab and the FG of PD-1 are critical in complex stability.Citation57 Like camrelizumab, the interaction sites of the PD-1–toripalimab-Fab partially overlap with that of the PD-1–PD-L1 complex, thus the antibody sterically hinders PD-1-PD-L1 binding.Citation41 In contrast to camrelizumab, toripalimab’s binding to PD-1 is not related to glycosylation. It is worth noting that this glycosylation-independent toripalimab-PD-1 binding makes toripalimab less likely to be affected by dysregulated glycosylation modifications of PD-1.
Figure 5. Structure of PD-1-toripalimab-fab (PDB:6JBT).

Structure of PD-1–nivolumab-Fab
Nivolumab was developed by Bristol Myers Squibb. First approved by the FDA in 2014, it is now marketed for the treatment of NSCLC, melanoma, advanced kidney cancer, head and neck squamous cell carcinoma, advanced classical Hodgkin’s lymphoma, and bladder cancer.Citation60–80,Citation131–138 Two structures of PD-1–nivolumab-Fab have been deposited in the Protein Data Bank (PDB: 5GGR, PDB: 5WT9).Citation35,Citation36 The binding interface of the complex comprises the LCDR2, LCDR1 and all three of the HCDRs of nivolumab and the N-terminal, FG and BC loops of PD-1 ().
Figure 6. Importance of the N-terminus of PD-1 in stabilization of PD-1-nivolumab-fab complex.

A crucial factor for PD-1–nivolumab-Fab binding was found to be nivolumab’s recognition of the N-terminal residues of PD-1. Truncation of N-terminus of PD-1 abolished its binding to nivolumab ().Citation36 The H-bonds connecting the N-terminus of PD-1 and the HCDR1–2 of nivolumab are the primary driving force to form the complex (). The remainder of the key factors of the interactions are between the FG and BC loops of PD-1 and nivolumab. In particular, the FG forms five H-bonds with the HCDR3 and LCDR2, whereas the BC loop has only one inter-molecular H-bond with the HCDR1 of nivolumab.
The nivolumab interferes with PD-1-PD-L1 contact via steric hindrance between its VL domain and PD-L1, which antagonize PD-1-PD-L1 binding. However, the overlap area of the nivolumab-PD-1 binding region with that of PD-L1-PD-1 is much smaller than the overlap area of camrelizumab or toripalimab on PD-1 with that of PD-L1-PD-1. A common characteristic of these three mAbs is that they all interact with the FG loop of PD-1, which shows the importance of the FG loop in mAb recognition, as suggested by recent research.Citation37 Moreover, the unique ability of nivolumab to bind the N-terminus of PD-1 indicates that the flexible segments of PD-1 are also promising targets for drug design.
Structure of PD-1–pembrolizumab-Fv
Pembrolizumab was approved by the FDA in 2014 and was the first anti-PD-1 mAb to achieve this milestone.Citation34 It was initially used to treat advanced melanoma and thereafter approved for the indications of NSCLC, metastatic head and neck squamous-cell carcinoma, refractory classical Hodgkin’s lymphoma and hepatocellular carcinoma.Citation74–Citation143 Crystal structures of both full-length pembrolizumab (PDB:5DK3)Citation144 and PD-1–pembrolizumab-Fv complexes (PDB:5JXE,Citation34 PDB:5B8CCitation33 have been determined.
The overall topology of the PD-1–pembrolizumab-Fv is unusual in involving more key amino acid residues in binding with PD-1 than other mAbs (). The pembrolizumab-PD-1 binding interface involves 21 amino acid residues of pembrolizumab, whereas camrelizumab, nivolumab, and toripalimab have 10–13 amino acid residues in contacting with PD-1.Citation33 All of the LCDRs and the HCDRs of pembrolizumab interact with the -sheet of PD-1, mainly by hydrophilic interactions, i.e., H-bonds and salt bridges. The PD-1–pembrolizumab-binding interface overlaps with most of the binding interface of PD-1–PD-L1 (), demonstrating that pembrolizumab sterically prevents the PD-L1-PD-1 contacts.Citation33 Because of the extensive hydrophilic inter-molecular interactions, the pembrolizumab binds to PD-1 with higher affinity than that PD-L1 binds to PD-1.
Figure 7. Comparison of PD-1 binding sites to pembrolizumab, PD-L1, camrelizumab, nivolumab and toripalimab.

Structure of PD-1–tislelizumab-Fab and PD-1–serplulimab-Fab
Developed by Beigene, Ltd., tislelizumab was approved by the NMPA in 2019. It was developed to exhibit less binding to the Fc-γ receptor 1,Citation145 the interactions of which have negative effect on anti-cancer activity by the anti-PD-1 mAbs.Citation146 Tislelizumab is used to treat hepatocellular carcinoma, Hodgkin’s lymphoma, urothelial carcinoma, and NSCLC.Citation81,Citation84,Citation108,Citation147,Citation148 Heo and colleagues solved the PD-1–tislelizumab-Fab structure (PDB:7BXA,Citation38 PDB:7CGWCitation39, which revealed that, unlike other mAbs that recognize the PD-1 loop region, tislelizumab mainly associates with the β-sheet of PD-1, closely mimicking PD-L1-PD-1 binding. In particular, the three LCDRs and the HCDR3 bind PD-1 via several H-bonds and many van der Waals contacts. The binding of tislelizumab to PD-1 sterically hinders the binding of PD-L1 to PD-1.Citation39
Serplulimab was developed by Shanghai Fuhong Hanlin Biotechnology Co., Ltd., and was approved by the NMPA in 2022 for the indications of unresectable, metastatic, and highly microsatellite-unstable solid tumors.Citation17,Citation96 Issafras and colleagues determined the structure of PD-1–serplulimab-Fab. The three flexible loops of PD-1 and all six CDRs of serplulimab comprise the interface of the complex. The residue Arg86 of PD-1 was found to be vital for complex formation, by forming multiple contacts with residues in the HCDR3 of serplulimab, including salt bridges with Asp104, hydrogen bonds with residue Ser98 and Tyr99, and a π–π interaction with Tyr323. Structural data of the complex demonstrated that many epitopes of PD-1 that are bound by serplulimab are also those contacting with PD-L1. Moreover, it was found that serplulimab binding triggers a structural alteration on the CC’ loop of PD-1.
Structures of PD-L1–mAb
As described above, the various anti-PD-1 antibodies exhibit significantly different topologies of binding with PD-1. In contrast, the interface of mAbs with PD-L1 are similar, typically on the β-sheet ().
Figure 8. The topology of structures of PD-L1-antibodies.

Structures of PD-L1–full-length mAbs
There are four full-length mAbs targeting PD-L1 on the market: atezolizumab, avelumab, durvalumab and sugemalimab. Atezolizumab was developed by Genentech and approved by the FDA in 2016, both avelumab, developed by Merck KGaA, and durvalumab, developed by AstraZeneca, were approved by FDA in 2017, while sugemalimab, developed by Cstone, was approved in China 2021. The indications of these mAbs are listed in .Citation101-103–Citation106–Citation114–153 Except sugemalimab, the complex structure of all these anti-PD-L1 mAbs with PD-L1 have been determined.
Zhang and colleagues determined the crystal structures of PD-L1–atezolizumab-Fab complexes (PDB:5XXYCitation43, PDB:5X8LCitation44, which showed that the VH domain dominates the binding. All three HCDRs are involved in binding with PD-1, while only LCDR3, but not the other two LCDRs, of the VL form contacts with PD-1. Atezolizumab is associated to the front β-sheet (C chain, C’ chain, F chain, and G chain), C’C loop and FG loop of PD-L1 via its LCDR3 and all three of its HCDRs.Citation43 The atezolizumab-Fab and PD-L1 binding interface consist of 13 hydrogen bonds and approximately 82 other interactions (polar interactions, hydrophobic interactions, and π–π stacking interactions).
The structure of the PD-L1–avelumab-scFv (PDB:5GRJ) was determined by Liu and colleagues.Citation46 Similar to atezolizumab, it binds PD-L1 mainly using the VH domain. All three HCDR loops are involved in binding, whereas the VL domain only uses LCDR1 and LCDR3 to form partial contacts. The epitope of PD-L1 comprises parts of the β-sheets and its CC’ loop. The CC’ loop of PD-L1 makes multiple H-bonds with the HCDR3 and LCDR3 of avelumab, and the latter loops occupy the binding surfaces of the four chains of PD-L1().
Figure 9. Structure of PD-L1-avelumab-scFv.

In contrast to the VH-dominated interactions that are exhibited by atezolizumab and avelumab, Tan and colleagues determined the structure of a PD-L1–durvalumab-scFv (PDB:5XJ4)Citation45 and identified that the VL and VH of durvalumab contribute equally to its binding to PD-L1. In addition, the structure of the PD-L1-durvalumab-Fab has also been reported by Heo and Lee (PDB:5X8M).Citation44 The three HCDRs and the LCDR1 and LCDR3 of durvalumab participate in PD-L1 binding; the LCDR1 and HCDR2 contact with the A and F chains of PD-L1, respectively, whereas the HCDR3, HCDR2 and LCDR3 of durvalumab form multiple H-bonds with G chain of PD-L1. The three mAbs- and PD-1-binding surfaces on PD-L1 are highly overlapping, which leads to competing mechanisms between mAbs and PD-1.Citation30
Structure of PD-L1–KN035
Nanobodies are highly stable and easy to produce in high yields through simple bacterial expression systems, making them a promising tool for research and treatment.Citation154 Envafolimab (KN035) was developed by Alphamab Oncology and approved by NMPA in 2021 for advanced biliary tract cancer and soft tissue sarcoma. It was the first and currently the only nanobody approved as a cancer therapeutic. Zhou and colleagues of Alphamab OncologyCitation47 obtained KN035 by immunizing camels with human PD-L1 and screening a VH-only nanobody phage library. Subsequently, the structure of KN035-PD-L1 complex was determined. It revealed that KN035 has three CDR loops (CDR1, CDR2 and CDR3),Citation155,Citation156 and that CDR1 folds into a short α helix, whereas CDR3 contains a short α helix and a 310 helix. A disulfide bond connects the short α helix of CDR3 to CDR1, and another disulfide bond connects the B and F chains of KN035 ().
Figure 10. Structure of PD-L1-KN035-scFv.

The interface of KN035 and PD-L1 is composed of the CDR1 and CDR3 of KN035 and the CC’FG chains of PD-L1, mainly through hydrophilic interactions, i.e., H-bonds and salt bridges (). KN035 binds PD-L1 on the front β-sheet of PD-L1, in a similar manner as PD-1-PD-L1, which suggested that KN035 blocks the PD-1-PD-L1 binding via a sterically hindering mechanism.
Summary and perspectives
This review summarizes the structures of FDA- and/or NMPA-approved antibodies that recognize PD-1 or PD-L1. The structures of these complexes reveal key driving forces that stabilize interactions and provide insight into the mechanisms of the antibodies to affect the PD-1–PD-L1 recognition. Although the anti-PD-1 mAbs primarily bind PD-1 on its front β-sheet and loops, each mAb exhibits a unique binding pattern that interferes with PD-1-PD-L1 interactions. In contrast, all anti-PD-L1 mAbs recognize nearly identical sites of PD-L1. In addition to the clinically approved antibodies mentioned above, there are other approved anti-PD-1 mAbs (sintilimab, penpulimab, dostarlimab, and zimberelimab) and an anti-PD-L1 mAb (sugemalimab), whose structures and interfaces with PD-1 or PD-L1 have yet to be revealed.
The comparison of the structures of mAbs targeting PD-1 or PD-L1 with the same epitopes revealed that the physicochemical properties of the amino acid residues within the epitopes determine the type of residues within the CDRs that interact with these epitopes. Specifically, the polar residues within the epitopes tend to recognize polar or charged residues within the CDRs. Interestingly, different mAbs can utilize different CDRs to interact with the same epitope, despite having the same type of amino acid residues to recognize the epitope. Therefore, it is valuable to gather additional structural data to elucidate the correlations between CDR sequences and complex structures. This knowledge can be applied to the design of novel mAbs or the prediction of mAb-antigen structures. Moreover, it can be integrated with mAb discovery methods to draw comprehensive conclusions.
The structural data of mAbs with PD-1 or PD-L1 also facilitated the optimization of antibodies through structure-based mutagenesis. This optimization aimed to improve the affinity of the antibodies with PD-1 or PD-L1, as well as their solubility and stability.Citation157–160 For instance, Bullock and colleagues analyzed the structure of nivolumab and identified specific amino acid residues that could influence the solubility of the mAb. This analysis led to the generation of a solubility-enhancing mutant of nivolumab.Citation161 Horita and colleaguesCitation33 used the structural data of the pembrolizumab-PD-1 complex to design cyclopeptide derivatives that bind to PD-1.
All the antibody structures described here do not contain the Fc region. The Fc region should have an impact on the overall structure of the antibody. Scapin and colleagues discovered the unique 120° rotation of the CH2 domain of pembrolizumab,Citation144 and mentioned this as one possibility among many conformations. The crystal structure obtained by analytical analysis is the result of an instantaneous state, while the hinge region has great flexibility. The specific effect of Fc regions on the overall structures of complexes between mAbs described above with PD-1 or PD-L1 are still unclear. The Fc region mainly mediates the functional effects of antibodies, such as antibody-dependent cellular cytotoxicity (ADCC) and complement-dependent cytotoxicity (CDC).Citation162 How the Fc region affects the overall structure of mAbs would reveal new structure–function relationships of anti-PD-1 and anti-PD-L1 mAbs. It is particularly important to IgG1 type anti-PD-L1 mAbs, which act on the surface of tumor cells and take advantage of Fc functions to induce the phagocytosis of target cells. Citation163
Another research perspective of clinical anti-PD-1 or anti-PD-L1 mAbs involves the structures in complicated physiological environments (e.g., the blood) or tumor micro-environments.Citation164 Recently, it has been shown that membrane environments have profound effects on PD-L1 activity.Citation165 However, all structures described here were obtained in crystals. Thus, structural characterization using integrative structural biology approaches, i.e., NMR spectroscopy and cryogenic electron microscopy, are anticipated to reveal the complex structures of mAbs with PD-1 or PD-L1 in a more native-like conditions, which may shed light on new aspects of the mechanisms of anti-PD-1 or anti-PD-L1 mAbs.
Abbreviations
ADCC | = | antibody-dependent cellular cytotoxicity |
CDC | = | complement-dependent cytotoxicity |
CH | = | constant domain from heavy chain; |
CL | = | constant domain from light chain; |
FDA | = | the Food and Drug Administration; |
HCDR | = | heavy chain complementarity-determining region |
ICB | = | immune checkpoint blockade (ICB); |
IgG | = | immunoglobulin G; |
KN035 | = | envafolimab; |
LCDR | = | light chain complementarity-determining region; |
mAb | = | monoclonal antibody; |
NMPA | = | the National Medical Products Administration; |
NSCLC | = | non-squamous non-small-cell lung cancer; |
PD-1 | = | programmed cell death 1; |
PD-L1 | = | Programmed cell death-ligand 1; |
PDB | = | the Protein Data Bank; |
T cells | = | thymocytes; |
VH | = | variable domain from heavy chain; |
VL | = | variable domain from light chain |
Acknowledgments
This work was supported by the National Natural Science Foundation of China (grant numbers 22274050) and the Fundamental Research Funds for the Central Universities. The authors thank Mr. Weidong Kong and the WZ Biosciences Inc. (Shandong, China) for providing access to research facilities during the COVID-19 lockdown. The authors thank Prof. Xianglei Liu from China State Institute of Pharmaceutical Industry for critical reading and suggestion on the manuscirpt.
Disclosure statement
No potential conflict of interest was reported by the author(s).
Additional information
Funding
References
- Postow MA, Callahan MK, Wolchok JD. Immune checkpoint blockade in cancer therapy. J Clin Onco. 2015;33:1974–17. doi:10.1200/jco.2014.59.4358. PMID: WOS:000355999800019.
- Ribas A, Wolchok JD. Cancer immunotherapy using checkpoint blockade. Science. 2018;359:1350–55. doi:10.1126/science.aar4060. PMID: WOS:000428043600036.
- Sharma P, Allison JP. The future of immune checkpoint therapy. Science. 2015;348:56–61. doi:10.1126/science.aaa8172. PMID: WOS:000352079500026.
- Okazaki T, Honjo T. PD-1 and PD-1 ligands: from discovery to clinical application. Int Immunol. 2007;19:813–24. doi:10.1093/intimm/dxm057. PMID: WOS:000249127900001.
- Callahan MK, Postow MA, Wolchok JD. Targeting T cell co-receptors for cancer therapy. Immun. 2016;44:1069–78. doi:10.1016/j.immuni.2016.04.023. PMID: WOS:000376478500008.
- Tan S, Chen D, Liu K, He M, Song H, Shi Y, Liu J, Zhang CWH, Qi J, Yan J, et al. Crystal clear: visualizing the intervention mechanism of the PD-1/PD-L1 interaction by two cancer therapeutic monoclonal antibodies. Protein Cell. 2016;7(12):866–77. doi:10.1007/s13238-016-0337-7. PMID: WOS:000391431600004.
- Wherry EJ. T cell exhaustion. Nat Immunol. 2011;12:492–99. doi:10.1038/ni.2035. PMID: WOS:000290707100007.
- Dong HD, Zhu GF, Tamada K, Chen LP. B7-H1, a third member of the B7 family, co-stimulates T-cell proliferation and interleukin-10 secretion. Nat Med. 1999;5:1365–69. doi:10.1038/70932. WOS:000084049700034. PMID: WOS:000084049700034.
- Ahmadzadeh M, Johnson LA, Heemskerk B, Wunderlich JR, Dudley ME, White DE, Rosenberg SA. Tumor antigen-specific CD8 T cells infiltrating the tumor express high levels of PD-1 and are functionally impaired. Blood. 2009;114:1537–44. doi:10.1182/blood-2008-12-195792. PMID: WOS:000269380300015.
- Hawkes EA, Grigg A, Chong G. Programmed cell death-1 inhibition in lymphoma. Lancet Oncol. 2015;16:E234–E45. doi:10.1016/s1470-2045(15)70103-8. PMID: WOS:000353908200025.
- Chen L, Han X. Anti–PD-1/PD-L1 therapy of human cancer: past, present, and future. J Clin Investigat. 2015;125(9):3384–91. PMID: WOS:000362303600013. doi:10.1172/jci80011.
- Okazaki T, Honjo T. The PD-1–PD-L pathway in immunological tolerance. Trends Immunol. 2006;27(4):195–201. PMID: WOS:000237145700008. doi:10.1016/j.it.2006.02.001.
- Herbst RS, Soria JC, Kowanetz M, Fine GD, Hamid O, Gordon MS, Sosman JA, McDermott DF, Powderly JD, Gettinger SN, et al. Predictive correlates of response to the anti-PD-L1 antibody MPDL3280A in cancer patients. Nature. 2014;515:563–67. doi:10.1038/nature14011. PMID: WOS:000346247600053.
- Schumacher TN, Kesmir C, van Buuren MM. Biomarkers in cancer immunotherapy. Cancer Cell. 2015;27:12–14. doi:10.1016/j.ccell.2014.12.004. PMID: WOS:000347906900006.
- Hoy SM. Sintilimab: first global approval. Drugs. 2019;79:341–46. doi:10.1007/s40265-019-1066-z. PMID: WOS:000459785400010.
- Keam SJ. Toripalimab: first global approval. Drugs. 2019;79:573–78. doi:10.1007/s40265-019-01076-2. PMID: WOS:000463813000009.
- Lee A. Serplulimab: first approval. Drugs. 2022;82:1137–41. doi:10.1007/s40265-022-01740-0. PMID: WOS:000825375200001.
- Lee AN, Keam SJ. Tislelizumab: first approval. Drugs. 2020;80:617–24. doi:10.1007/s40265-020-01286-z. PMID: WOS:000520675700002.
- Markham A. Zimberelimab: first approval. Drugs. 2021;81:2063–68. doi:10.1007/s40265-021-01628-5. PMID: WOS:000712334300001.
- Markham A, Duggan S. Cemiplimab: first global approval. Drugs. 2018;78(17):1841–46. PMID: WOS:000451587400008. doi:10.1007/s40265-018-1012-5.
- Markham A, Keam SJ. Camrelizumab: first global approval. Drugs. 2019;79(12):1355–61. PMID: WOS:000479057000008. doi:10.1007/s40265-019-01167-0.
- Paik J. Nivolumab plus relatlimab: first approval. Drugs. 2022;82(8):925–31. PMID: WOS:000793714400001. doi:10.1007/s40265-022-01723-1.
- Poole RM. Pembrolizumab: first global approval. Drugs. 2014;74(16):1973–81. PMID: WOS:000344619000008. doi:10.1007/s40265-014-0314-5.
- Dhillon S. Penpulimab: first approval. Drugs. 2021;81(18):2159–66. PMID: WOS:000721652600001. doi:10.1007/s40265-021-01640-9.
- Markham A. Dostarlimab: first approval. Drugs. 2021;81(10):1213–19. PMID: WOS:000659390700002. doi:10.1007/s40265-021-01539-5.
- Syed YY. Durvalumab: first global approval. Drugs. 2017;77(12):1369–76. PMID: WOS:000406276900009. doi:10.1007/s40265-017-0782-5.
- Rao A, Patel MR. A review of avelumab in locally advanced and metastatic bladder cancer. Ther Adv Urol. 2019;11:1756287218823485. doi:10.1177/1756287218823485. PMID: WOS:000457393900001.
- Markham A. Envafolimab: first approval. Drugs. 2022;82(2):235–40. PMID: WOS:000751583100001. doi:10.1007/s40265-022-01671-w.
- Markham A. Atezolizumab: first global approval. Drugs. 2016;76(12):1227–32. PMID: WOS:000380745400007. doi:10.1007/s40265-016-0618-8.
- Zak KM, Kitel R, Przetocka S, Golik P, Guzik K, Musielak B, Domling A, Dubin G, Holak TA. Structure of the complex of human programmed death 1, PD-1, and its ligand PD-L1. Struct. 2015;23:2341–48. doi:10.1016/j.str.2015.09.010. PMID: WOS:000366171500016.
- Pascolutti R, Sun XQ, Kao J, Maute RL, Ring AM, Bowman GR, Kruse AC. Structure and dynamics of PD-L1 and an ultra-high-affinity PD-1 receptor mutant. Struct. 2016;24:1719–28. doi:10.1016/j.str.2016.06.026. PMID: WOS:000386763800011.
- Lin DW, Tanaka Y, Iwasaki M, Gittis AG, Su HP, Mikami B, Okazaki T, Honjo T, Minato N, Garboczi DN. The PD-1/PD-L1 complex resembles the antigen-binding Fv domains of antibodies and T cell receptors. P Natl Acad Sci Usa. 2008;105:3011–16. doi:10.1073/pnas.0712278105. PMID: WOS:000253567900048.
- Horita S, Nomura Y, Sato Y, Shimamura T, Iwata S, Nomura N. High-resolution crystal structure of the therapeutic antibody pembrolizumab bound to the human PD-1. Sci Rep-Uk. 2016;6(1):35297. PMID: WOS:000385346100001. doi:10.1038/srep35297.
- Na Z, Yeo SP, Bharath SR, Bowler MW, Balikci E, Wang CI, Song H. Structural basis for blocking PD-1-mediated immune suppression by therapeutic antibody pembrolizumab. Cancer Res. 2017;27:147–50. doi:10.1038/cr.2016.77. PMID: WOS:000392277900014.
- Lee JY, Lee HT, Shin W, Chae J, Choi J, Kim SH, Lim H, Heo TW, Park KY, Ryu SE, et al. Structural basis of checkpoint blockade by monoclonal antibodies in cancer immunotherapy. Nat Commun. 2016;7. doi:10.1038/ncomms13354. PMID: WOS:000386515200001.
- Tan S, Zhang H, Chai Y, Song H, Tong Z, Wang Q, Qi J, Wong G, Zhu X, Liu WJ, et al. An unexpected N-terminal loop in PD-1 dominates binding by nivolumab. Nat Commun. 2017;8(1):14369. doi:10.1038/ncomms14369. PMID: WOS:000393375400001.
- Lu D, Xu Z, Zhang D, Jiang M, Liu K, He J, Ma D, Ma X, Tan S, Gao GF, et al. PD-1 N58-glycosylation-dependent binding of monoclonal antibody cemiplimab for immune checkpoint therapy. Front Immunol. 2022;13:826045. doi:10.3389/fimmu.2022.826045. PMID: WOS:000771576300001.
- Sang HL, Lee HT, Lim H, Kim Y, Heo YSJB, Communications BR. Crystal structure of PD-1 in complex with an antibody-drug tislelizumab used in tumor immune checkpoint therapy. Biochem Bioph Res Co. 2020;527(1):226–31. doi:10.1016/j.bbrc.2020.04.121.
- Hong Y, Feng Y, Sun H, Zhang B, Wu H, Zhu Q, Li Y, Zhang T, Zhang Y, Cui X, et al. Tislelizumab uniquely binds to the CC′ loop of PD-1 with slow-dissociated rate and complete PD-L1 blockage. FEBS Open Bio. 2021;11(3):782–92. doi:10.1002/2211-5463.13102. PMID: WOS:000618375800001.
- Liu K, Tan S, Jin W, Guan J, Wang Q, Sun H, Qi J, Yan J, Chai Y, Wang Z, et al. N-glycosylation of PD-1 promotes binding of camrelizumab. EMBO Rep. 2020;21(12):e51444. doi:10.15252/embr.202051444. PMID: WOS:000577337200001.
- Liu H, Guo L, Zhang J, Zhou Y, Zhou J, Yao J, Wu H, Yao S, Chen B, Chai Y, et al. Glycosylation-independent binding of monoclonal antibody toripalimab to FG loop of PD-1 for tumor immune checkpoint therapy. MAbs. 2019;11(4):681–90. doi:10.1080/19420862.2019.1596513. PMID: WOS:000476960300006.
- Issafras H, Fan S, Tseng CL, Cheng Y, Lin P, Xiao L, Huang YJ, Tu CH, Hsiao YC, Li M, et al. Structural basis of HLX10 PD-1 receptor recognition, a promising anti-PD-1 antibody clinical candidate for cancer immunotherapy. PLoS One. 2021;16(12):e0257972. doi:10.1371/journal.pone.0257972. PMID: WOS:000773555700007.
- Zhang F, Qi X, Wang X, Wei D, Wu J, Feng L, Cai H, Wang Y, Zeng N, Xu T, et al. Structural basis of the therapeutic anti-PD-L1 antibody atezolizumab. Oncotarget. 2017;8:90215–24. doi:10.18632/oncotarget.21652. PMID: WOS:000414097100066.
- Lee HT, Lee JY, Lim H, Lee SH, Moon YJ, Pyo HJ, Ryu SE, Shin W, Heo YS. Molecular mechanism of PD-1/PD-L1 blockade via anti-PD-L1 antibodies atezolizumab and durvalumab. Sci Rep-Uk. 2017;7:5532. doi:10.1038/s41598-017-06002-8. PMID: WOS:000405675400012.
- Tan S, Liu K, Chai Y, Zhang CWH, Gao S, Gao GF, Qi J. Distinct PD-L1 binding characteristics of therapeutic monoclonal antibody durvalumab. Protein Cell. 2018;9(1):135–39. PMID: WOS:000423135400011. doi:10.1007/s13238-017-0412-8.
- Liu K, Tan S, Chai Y, Chen D, Song H, Zhang CH, Shi Y, Liu J, Tan W, Lyu J, et al. Structural basis of anti-PD-L1 monoclonal antibody avelumab for tumor therapy. Cancer Res. 2017;27:151–53. doi:10.1038/cr.2016.102. PMID: WOS:000392277900015.
- Zhang F, Wei H, Wang X, Bai Y, Wang P, Wu J, Jiang X, Wang Y, Cai H, Xu T, et al. Structural basis of a novel PD-L1 nanobody for immune checkpoint blockade. Cell Discov. 2017;3(1):17004. doi:10.1038/celldisc.2017.4. PMID: WOS:000414903600001.
- Lazar-Molnar E, Yan QR, Cao E, Ramagopal U, Nathenson SG, Almo SC. Crystal structure of the complex between programmed death-1 (PD-1) and its ligand PD-L2. Proc Natl Acad Sci USA. 2008;105(30):10483–88. PMID: WOS:000258211600039. doi:10.1073/pnas.0804453105.
- Cheng XX, Veverka V, Radhakrishnan A, Waters LC, Muskett FW, Morgan SH, Huo JD, Yu C, Evans EJ, Leslie AJ, et al. Structure and interactions of the human programmed cell death 1 receptor. J Biol Chem. 2013;288(17):11771–85. doi:10.1074/jbc.M112.448126. PMID: WOS:000318157600014.
- Tan SG, Zhang CWH, Gao GF. Seeing is believing: anti-PD-1/PD-L1 monoclonal antibodies in action for checkpoint blockade tumor immunotherapy. Signal Transduct Tar. 2016;1(1):e16029. PMID: WOS:000454602900017. doi:10.1038/sigtrans.2016.29.
- Tan SG, Chen DQ, Liu KF, He MN, Song H, Shi Y, Liu J, Zhang C, Qi JX, Yan JH, et al. Crystal clear: visualizing the intervention mechanism of the PD-1/PD-L1 interaction by two cancer therapeutic monoclonal antibodies. Protein Cell. 2016;7(12):866–77. doi:10.1007/s13238-016-0337-7. PMID: WOS:000391431600004.
- Shin J, Phelan PJ, Gjoerup O, Bachovchin W, Bullock PA. Characterization of a single chain variable fragment of nivolumab that targets PD-1 and blocks PD-L1 binding. Protein Expres Purif. 2021;177:105766. doi:10.1016/j.pep.2020.105766. PMID: WOS:000579451300016.
- Sahu M, Zimberelimab CJ. Anti-programmed cell death protein 1 (PD-1) monoclonal antibody, Treatment of hodgkin lymphoma, treatment of advanced solid tumors. Drugs Fut. 2022;47(2):115–21. PMID: WOS:000759046000001. doi:10.1358/dof.2022.47.2.3400575.
- Mittal L, Srivastava M, Kumari A, Tonk RK, Awasthi A, Asthana S. Interplay among structural stability, plasticity, and energetics determined by conformational attuning of flexible loops in PD-1. J Chem Inf Model. 2021;61(1):358–84. PMID: WOS:000613719400033. doi:10.1021/acs.jcim.0c01080.
- Lu D, Xu ZP, Zhang D, Jiang M, Liu KF, He JH, Ma DL, Ma XP, Tan SG, Gao GF, et al. PD-1 N58-gycosylation-dependent binding of monoclonal antibody cemiplimab for immune checkpoint therapy. Front Immunol. 2022;13:826045. doi:10.3389/fimmu.2022.826045. PMID: WOS:000771576300001.
- Liu KF, Tan SG, Jin WJ, Guan JW, Wang QL, Sun H, Qi JX, Yan JH, Chai Y, Wang ZF, et al. N-glycosylation of PD-1 promotes binding of camrelizumab. EMBO Rep. 2020;21(12):e51444. doi:10.15252/embr.202051444. PMID: WOS:000577337200001.
- Liu HC, Guo LJ, Zhang J, Zhou YH, Zhou JW, Yao J, Wu H, Yao S, Chen B, Chai Y, et al. Glycosylation-independent binding of monoclonal antibody toripalimab to FG loop of PD-1 for tumor immune checkpoint therapy. MAbs. 2019;11(4):681–90. doi:10.1080/19420862.2019.1596513. PMID: WOS:000476960300006.
- Lee HT, Lee SH, Heo YS. Molecular interactions of antibody drugs targeting PD-1, PD-L1, and CTLA-4 in immuno-oncology. Molecules. 2019;24:1190. doi:10.3390/molecules24061190. PMID: WOS:000465503800152.
- Issafras H, Fan SL, Tseng CL, Cheng YC, Lin PH, Xiao LS, Huang YJ, Tu CH, Hsiao YC, Li M, et al. Structural basis of HLX10 PD-1 receptor recognition, a promising anti-PD-1 antibody clinical candidate for cancer immunotherapy. PLoS One. 2021;16(12):e0257972. doi:10.1371/journal.pone.0257972. PMID: WOS:000773555700007.
- Ferris RL, Blumenschein G Jr., Fayette J, Guigay J, Colevas AD, Licitra L, Harrington K, Kasper S, Vokes EE, Even C, et al. Nivolumab for recurrent squamous-cell carcinoma of the head and neck. N Engl J Med. 2016;375(19):1856–67. doi:10.1056/NEJMoa1602252. PMID: WOS:000387534200009.
- Feng YC, Hong Y, Sun HZ, Zhang B, Wu HF, Li K, Liu XS, Liu Y. The molecular binding mechanism of tislelizumab, an investigational anti-PD-1 antibody, is differentiated from pembrolizumab and nivolumab. Cancer Res. 2019;79(13_Supplement):2383–2383. PMID: WOS:000488279400352. doi:10.1158/1538-7445.Am2019-2383.
- Zhang F, Wei HD, Wang XX, Bai Y, Wang PL, Wu JW, Jiang XY, Wang YG, Cai HY, Xu T, et al. Structural basis of a novel PD-L1 nanobody for immune checkpoint blockade. Cell Discov. 2017;3(1):17004. doi:10.1038/celldisc.2017.4. PMID: WOS:000414903600001.
- Tan SG, Liu KF, Chai Y, Zhang CWH, Gao S, Gao G, Qi JX. Distinct PD-L1 binding characteristics of therapeutic monoclonal antibody durvalumab. Protein Cell. 2018;9(1):135–39. PMID: WOS:000423135400011. doi:10.1007/s13238-017-0412-8.
- Liu KF, Tan SG, Chai Y, Chen DQ, Song H, Zhang CWH, Shi Y, Liu J, Tan WJ, Lyu JX, et al. Structural basis of anti-PD-L1 monoclonal antibody avelumab for tumor therapy. Cancer Res. 2017;27(1):151–53. doi:10.1038/cr.2016.102. PMID: WOS:000392277900015.
- Cordova-Bahena L, Velasco-Velazquez MA. Anti-PD-1 and anti-PD-L1 antibodies as imminotherapy against cancer: a structural perspective. Rev Invest Clin. 2021;73:8–16. doi:10.24875/ric.20000341. PMID: WOS:000662706100003.
- Makaremi S, Asadzadeh Z, Hemmat N, Baghbanzadeh A, Sgambato A, Ghorbaninezhad F, Safarpour H, Argentiero A, Brunetti O, Bernardini R, et al. Immune checkpoint inhibitors in colorectal cancer: challenges and future prospects. Biomed. 2021;9(9):1075. doi:10.3390/biomedicines9091075. PMID: WOS:000699157400001.
- Lin X, Lu X, Luo GS, Xiang H. Progress in PD-1/PD-L1 pathway inhibitors: from biomacromolecules to small molecules. Eur J Med Chem. 2020;186:111876. doi:10.1016/j.ejmech.2019.111876. PMID: WOS:000509616800004.
- Mittal L, Tonk R, Awasthi A, Asthana S. Traversing through the dynamic protein–protein interaction landscape and conformational plasticity of PD-1 for small-molecule discovery. J Med Chem. 2022;65(8):5941–53. PMID: WOS:000797573100006. doi:10.1021/acs.jmedchem.2c00176.
- Park UB, Jeong TJ, Gu N, Lee HT, Heo YS. Molecular basis of PD-1 blockade by dostarlimab, the FDA-approved antibody for cancer immunotherapy. Biochem Bioph Res Co. 2022;599:31–37. doi:10.1016/j.bbrc.2022.02.026. PMID: WOS:000755175000005.
- Tang SG, Kim PS. A high-affinity human PD-1/PD-L2 complex informs avenues for small-molecule immune checkpoint drug discovery. P Natl Acad Sci Usa. 2019;116:24500–06. doi:10.1073/pnas.1916916116. PMID: WOS:000500804600026.
- Lee SH, Lee HT, Lim H, Kim Y, Park UB, Heo YS. Crystal structure of PD-1 in complex with an antibody-drug tislelizumab used in tumor immune checkpoint therapy. Biochem Bioph Res Co. 2020;527:226–31. doi:10.1016/j.bbrc.2020.04.121. PMID: WOS:000535964300034.
- Vidarsson G, Dekkers G, Rispens T. IgG subclasses and allotypes: from structure to effector functions. Front Immunol. 2014;5:520. doi:10.3389/fimmu.2014.00520. PMID: WOS:000354496200001.
- Kim DW, Zager JS, Eroglu Z. Improving clinical outcomes with pembrolizumab in patients with advanced melanoma. Chin Clin Oncol. 2017;6:2–2. doi:10.21037/cco.2017.01.04. PMID: MEDLINE:28285535.
- Reck M, Rodriguez-Abreu D, Robinson AG, Hui R, Csoszi T, Fulop A, Gottfried M, Peled N, Tafreshi A, Cuffe S, et al. Pembrolizumab versus chemotherapy for PD-L1-positive non-small-cell lung cancer. New Engl J Med. 2016;375:1823–33. doi:10.1056/NEJMoa1606774. PMID: WOS:000387534200006.
- Burtness B, Harrington KJ, Greil R. Pembrolizumab alone or with chemotherapy versus cetuximab with chemotherapy for recurrent or metastatic squamous cell carcinoma of the head and neck (KEYNOTE-048): a randomised, open-label, phase 3 study (vol 394, pg 1915, 2019). Lancet. 2020;395:564–564. WOS:000514849400026. PMID: WOS:000514849400026.
- Riano I, Patel SR, Liu SV, Duma N. Evidence to date: evaluating pembrolizumab in the treatment of extensive-stage small-cell lung cancer. Clinics Pract. 2021;11:441–54. doi:10.3390/clinpract11030059. PMID: MEDLINE:34287275.
- Lyu MM, Shen Y, Beharee N, Lu J, Deng F, Wang JH. The combined use of chemotherapy and radiotherapy with PD- I inhibitor, pembrolizumab, in advanced cervical cancer: a case report. OncoTargets Ther. 2020;13:4465–71. doi:10.2147/ott.S245190. PMID: WOS:000534220600001.
- Asmar R, Yang J, Carvajal RD. Clinical utility of nivolumab in the treatment of advanced melanoma. Ther Clin Risk Manag. 2016;12:313–25. doi:10.2147/tcrm.S78039. PMID: WOS:000370939200001.
- Cortinovis DL, Canova S, Abbate M, Colonese F, Bidoli P. Focus on nivolumab in NSCLC. Front Med-Lausanne. 2016;3:67. doi:10.3389/fmed.2016.00067. PMID: WOS:000407067900001.
- Ansell SM, Lesokhin AM, Borrello I, Halwani A, Scott EC, Gutierrez M, Schuster SJ, Millenson MM, Cattry D, Freeman GJ, et al. PD-1 blockade with nivolumab in relapsed or refractory Hodgkin’s lymphoma. New Engl J Med. 2015;372:311–19. doi:10.1056/NEJMoa1411087. PMID: WOS:000348204500006.
- Song Y, Gao Q, Zhang H, Fan L, Zhou J, Zou D, Li W, Yang H, Liu T, Wang Q, et al. Tislelizumab (BGB-A317) for relapsed/refractory classical Hodgkin lymphoma: preliminary efficacy and safety results from a phase 2 study. Blood. 2018;132(Supplement 1):682–682. doi:10.1182/blood-2018-99-117848. PMID: WOS:000454837602060.
- Ye D, Liu J, Zhou A, Zou Q, Li H, Fu C, Hu H, Huang J, Zhu S, Jin J, et al. Tislelizumab in Asian patients with previously treated locally advanced or metastatic urothelial carcinoma. Cancer Sci. 2021;112:305–13. doi:10.1111/cas.14681. PMID: WOS:000585909300001.
- Liu SY, Wu YL. Tislelizumab: an investigational anti-PD-1 antibody for the treatment of advanced non-small cell lung cancer (NSCLC). Expert Opin Inv Drug. 2020;29(12):1355–63. doi:10.1080/13543784.2020.1833857. PMID:WOS:000580619700001.
- Kudo M. Systemic therapy for hepatocellular carcinoma: latest advances. Cancers. 2018;10:412. doi:10.3390/cancers10110412. PMID: WOS:000451307700019.
- Song YQ, Wu JQ, Chen XC, Lin TY, Cao JN, Liu YY, Zhao YZ, Jin J, Huang HW, Hu JD, et al. A single-arm, multicenter, phase II study of camrelizumab in relapsed or refractory classical Hodgkin lymphoma. Clin Cancer Res. 2019;25:7363–69. doi:10.1158/1078-0432.Ccr-19-1680. PMID: WOS:000509983400013.
- Qin SK, Ren ZG, Meng ZQ, Chen ZD, Chai XL, Xiong JP, Bai YX, Yang L, Zhu H, Fang WJ, et al. Camrelizumab in patients with previously treated advanced hepatocellular carcinoma: a multicentre, open-label, parallel-group, randomised, phase 2 trial. Lancet Oncol. 2020;21:571–80. doi:10.1016/s1470-2045(20)30011-5. PMID: WOS:000522625000033.
- Huang J, Xu JM, Chen Y, Zhuang W, Zhang YP, Chen ZD, Chen J, Zhang HL, Niu ZX, Fan QX, Lin, L., et al. Camrelizumab versus investigator’s choice of chemotherapy as second-line therapy for advanced or metastatic oesophageal squamous cell carcinoma (ESCORT): a multicentre, randomised, open-label, phase 3 study. Lancet Oncol. 2020;21(6):832–42. doi:10.1016/s1470-2045(20)30110-8. PMID: WOS:000544057400034.
- Fang WF, Yang YP, Ma YX, Hong SD, Lin LZ, He XH, Xiong JP, Li P, Zhao HY, Huang Y, et al. Camrelizumab (SHR-1210) alone or in combination with gemcitabine plus cisplatin for nasopharyngeal carcinoma: results from two single-arm, phase 1 trials. Lancet Oncol. 2018;19:1338–50. doi:10.1016/s1470-2045(18)30495-9. PMID: WOS:000446052800054.
- Sun GG, Jia JH, Gao P, Yao XM, Chen MD, Yao WN, Sun L, Wang W. Activity and safety of camrelizumab, an anti-PD-1 immune checkpoint inhibitor, for patients with advanced non-small-cell lung cancer. J ImmunoTher Cancer. 2020;8:A267–A68. doi:10.1136/jitc-2020-SITC2020.0440. PMID: WOS:000616665300428.
- Tang BX, Chi ZH, Guo J. Toripalimab for the treatment of melanoma. Expert Opin Biol Th. 2020;20:863–69. doi:10.1080/14712598.2020.1762561. PMID: WOS:000534142800001.
- Wang FH, Wei XL, Feng JF, Li Q, Xu N, Hu XC, Liao WJ, Jiang Y, Lin XY, Zhang QY, et al. Efficacy, safety, and correlative biomarkers of toripalimab in previously treated recurrent or metastatic nasopharyngeal carcinoma: a phase II clinical trial (POLARIS-02). J Clin Onco. 2021;39:704–12. doi:10.1200/jco.20.02712. PMID: WOS:000635371200003.
- Zan N, Zhang X, Du LY, Lin ZY, Yu DF, Liu J, Gou FS. Case report: toripalimab combined with anlotinib in a patient with metastatic upper tract urothelial carcinoma after pembrolizumab failure. Front Oncol. 2022;12(796407):796407. doi:10.3389/fonc.2022.796407. PMID: WOS:000770970400001.
- Poh A. Assessing toripalimab in NSCLC. Cancer Discov. 2022;12:1176–77. doi:10.1158/2159-8290.Cd-nb2022-0022. PMID: WOS:000795608300005.
- Ghidini A, Santangelo D, Vaccaro G, Chillura M, Petrelli F. Cemiplimab in cutaneous squamous cell carcinomas (SCC): an overview and a clinical case. Oral Oncol. 2022;128:105847. doi:10.1016/j.oraloncology.2022.105847. PMID: WOS:000792738900003.
- Naik PP. Cemiplimab in advanced cutaneous squamous cell carcinoma. Dermatol Ther. 2021;34:e15184. doi:10.1111/dth.15184. PMID: WOS:000715489200001.
- Ren Z, Shao G, Shen J, Zhang L, Zhu X, Fang W, Sun G, Bai Y, Wu J, Liu L, et al. Phase 2 study of the PD-1 inhibitor serplulimab plus the bevacizumab biosimilar HLX04 in patients with previously treated advanced hepatocellular carcinoma. Liver Cancer. 2022;12(2):116–28. doi:10.1159/000526638. PMID: WOS:000848468700001.
- Oaknin A, Tinker AV, Gilbert L, Samouelian V, Mathews C, Brown J, Barretina-Ginesta MP, Moreno V, Gravina A, Abdeddaim C, et al. Clinical activity and safety of the anti-PD-1 monoclonal antibody dostarlimab for patients with recurrent or advanced dMMR endometrial cancer. Future Oncol. 2021;17:3781–85. doi:10.2217/fon-2021-0598. PMID: WOS:000687857400001.
- Liu XH, Yi Y. Sintilimab plus sorafenib: a novel regimen for hepatocellular carcinoma. Immunotherapy-Uk. 2021;13:1387–93. doi:10.2217/imt-2021-0062. PMID: WOS:000708528700001.
- Lv HL, Tian Y, Li JC, Huang C, Sun BK, Gai CY, Li ZH, Tian ZQ. Neoadjuvant sintilimab plus chemotherapy in resectable locally advanced esophageal squamous cell carcinoma. Front Oncol. 2022;12:864533. doi:10.3389/fonc.2022.864533. PMID: WOS:000795234900001.
- Zhang L, Lin WH, Tan FW, Li N, Xue Q, Gao SG, Gao YB, He J. Sintilimab for the treatment of non-small cell lung cancer. Biomark Res. 2022;10:23. doi:10.1186/s40364-022-00363-7. PMID: WOS:000786459400001.
- Deng M. The approval of sintilimab for classical Hodgkin’s lymphoma: views and perspectives of anti-PD-1/PD-L1 antibodies in China. Antib Ther. 2019;2:54–55. doi:10.1093/abt/tbz005. PMID: MEDLINE:33928222.
- Haddley K. Dostarlimab anti-PD-1 monoclonal antibody treatment of advanced solid tumors. Drug Future. 2019;44:527–34. doi:10.1358/dof.2019.044.07.3023389. PMID: WOS:000478690100002.
- Song Y, Zhou K, Jin C, Qian Z, Hou M, Fan L, Li F, Ding K, Zhou H, Li X, et al. A phase II study of penpulimab, an anti-PD-1 antibody, in patients with relapsed or refractoryclassic Hodgkin lymphoma (cHL). J Clin Onco. 2021;39(15_suppl):7529–7529. doi:10.1200/JCO.2021.39.15_suppl.7529. PMID: WOS:000708120604126.
- Lin NJ, Zhang MZ, Bai H, Liu H, Cui J, Ke XY, Zhang HL, Liu LH, Yan DM, Jiang YS, et al. Efficacy and safety of GLS-010 (zimberelimab) in patients with relapsed or refractory classical Hodgkin lymphoma: A multicenter, single-arm, phase II study. Eur J Cancer. 2022;164:117–26. doi:10.1016/j.ejca.2021.07.021. PMID: WOS:000792600200013.
- Crist M, Balar A. Atezolizumab in invasive and metastatic urothelial carcinoma. Expert Rev Clin Phar. 2017;10:1295–301. doi:10.1080/17512433.2017.1389275. PMID: WOS:000418037400002.
- Rittmeyer A, Barlesi F, Waterkamp D, Park K, Ciardiello F, von Pawel J, Gadgeel SM, Hida T, Kowalski DM, Dols MC, et al. Atezolizumab versus docetaxel in patients with previously treated non-small-cell lung cancer (OAK): a phase 3, open-label, multicentre randomised controlled trial. Lancet. 2017;389:255–65. doi:10.1016/s0140-6736(16)32517-x. PMID: WOS:000392801200027.
- McDermott DF, Huseni MA, Atkins MB, Motzer RJ, Rini BI, Escudier B, Fong L, Joseph RW, Pal SK, Reeves JA, et al. Clinical activity and molecular correlates of response to atezolizumab alone or in combination with bevacizumab versus sunitinib in renal cell carcinoma. Nat Med. 2018;24:749–57. doi:10.1038/s41591-018-0053-3. PMID: WOS:000434281300020.
- Finkelmeier F, Waidmann O, Trojan J. Nivolumab for the treatment of hepatocellular carcinoma. Expert Rev Anticanc. 2018;18:1169–75. doi:10.1080/14737140.2018.1535315. PMID: WOS:000450563700002.
- Schmid P, Adams S, Rugo HS, Schneeweiss A, Barrios CH, Iwata H, Dieras V, Hegg R, Im SA, Wright GS, et al. Atezolizumab and nab-paclitaxel in advanced triple-negative breast cancer. New Engl J Med. 2018;379:2108–21. doi:10.1056/NEJMoa1809615. PMID: WOS:000451402500006.
- Rico GT, Price TJ. Atezolizumab for the treatment of colorectal cancer: the latest evidence and clinical potential. Expert Opin Biol Th. 2018;18:449–57. doi:10.1080/14712598.2018.1444024. PMID: WOS:000428249400009.
- Barlesi F, Vansteenkiste J, Spigel D, Ishii H, Garassino M, de Marinis F, Ozguroglu M, Szczesna A, Polychronis A, Uslu R, et al. Avelumab versus docetaxel in patients with platinum-treated advanced non-small-cell lung cancer (JAVELIN lung 200): an open-label, randomised, phase 3 study. Lancet Oncol. 2018;19:1468–79. doi:10.1016/s1470-2045(18)30673-9. PMID: WOS:000449100300044.
- Motzer RJ, Penkov K, Haanen J, Rini B, Albiges L, Campbell MT, Venugopal B, Kollmannsberger C, Negrier S, Uemura M, et al. Avelumab plus axitinib versus sunitinib for advanced renal-cell carcinoma. New Engl J Med. 2019;380:1103–15. doi:10.1056/NEJMoa1816047. PMID: WOS:000461898400006.
- Roviello G, D’Angelo A, Generali D, Pittacolo M, Ganzinelli M, Iezzi G, de Manzini N, Sobhani N. Avelumab in gastric cancer. Immunotherapy-Uk. 2019;11:759–68. doi:10.2217/imt-2019-0011. PMID: WOS:000469496800002.
- Antonia S, Goldberg SB, Balmanoukian A, Chaft JE, Sanborn RE, Gupta A, Narwal R, Steele K, Gu Y, Karakunnel JJ, et al. Safety and antitumour activity of durvalumab plus tremelimumab in non-small-cell lung cancer: a multicentre, phase 1b study. Lancet Oncol. 2016;17:299–308. doi:10.1016/s1470-2045(15)00544-6. PMID: WOS:000371234900043.
- Ferris RL, Haddad R, Even C, Tahara M, Dvorkin M, Ciuleanu TE, Clement PM, Mesia R, Kutukova S, Zholudeva L, et al. Durvalumab with or without tremelimumab in patients with recurrent or metastatic head and neck squamous cell carcinoma: EAGLE, a randomized, open -label phase III study. Ann Oncol. 2020;31:942–50. doi:10.1016/j.annonc.2020.04.001. PMID: WOS:000540695500017.
- Siu LL, Even C, Mesia R, Remenar E, Daste A, Delord JP, Krauss J, Saba NF, Nabell L, Ready NE, et al. Safety and efficacy of durvalumab with or without tremelimumab in patients with PD-L1-low/negative recurrent or metastatic HNSCC the phase 2 CONDOR randomized clinical trial. JAMA Oncol. 2019;5:195–203. doi:10.1001/jamaoncol.2018.4628. PMID: WOS:000458630000015.
- Shimizu T, Nakajima TE, Yamamoto N, Yonemori K, Koyama T, Kondo S, Sunakawa Y, Izawa N, Horie Y, Xiang SL, et al. Phase I study of envafolimab (KN035), a novel subcutaneous single-domain anti-PD-L1 monoclonal antibody, in Japanese patients with advanced solid tumors. Invest New Drug. 2022;40:1021–31. doi:10.1007/s10637-022-01287-7. PMID: WOS:000836752400001.
- Shen L, Li J, Deng YH, Zhang WJ, Zhou AP, Guo WJ, Yang JW, Yuan Y, Zhu LJ, Qin SK, et al. Envafolimab (KN035) in advanced tumors with mismatch-repair deficiency. J Clin Onco. 2020;38(15_suppl):3021–3021. doi:10.1200/JCO.2020.38.15_suppl.3021. WOS:000560368301283. PMID: WOS:000560368301283.
- Li J, Deng YH, Zhang WJ, Zhou AP, Guo WJ, Yang JW, Yuan Y, Zhu LJ, Qin SK, Xiang SL, et al. Subcutaneous envafolimab monotherapy in patients with advanced defective mismatch repair/microsatellite instability high solid tumors. J Hematol Oncol. 2021;14:95. doi:10.1186/s13045-021-01095-1. PMID: WOS:000664109200001.
- Dhillon S, Duggan S. Sugemalimab: first approval. Drugs. 2022;82:593–99. doi:10.1007/s40265-022-01693-4. PMID: WOS:000770214800002.
- Ribatti D. Edelman’s view on the discovery of antibodies. Immunol Lett. 2015;164(2):72–75. doi:10.1016/j.imlet.2015.02.005. PMID: WOS:000353094900003.
- Siegel DL. Recombinant monoclonal antibody technology. Transfus Clin Biol. 2002;9:15–22. doi:10.1016/s1246-7820(01)00210-5. PMID: WOS:000174094300003.
- Ahmad ZA, Yeap SK, Ali AM, Ho WY, Alitheen NBM, Hamid M. scFv antibody: principles and clinical application. Clin Dev Immunol. 2012;2012:1–15. doi:10.1155/2012/980250. PMID: WOS:000302595700001.
- Wen BY, Zhao L, Wang YC, Qiu CN, Xu ZM, Huang KL, Zhu H, Li ZM, Li HJ. Nanobodies targeting the interaction interface of programmed death receptor 1 (PD-1)/PD-1 ligand 1 (PD-1/PD-L1). Prep Biochem Biotech. 2020;50:252–59. doi:10.1080/10826068.2019.1692217. PMID: WOS:000500447300001.
- Lim H, Chun J, Jin X, Kim J, Yoon J, No KT. Investigation of protein-protein interactions and hot spot region between PD-1 and PD-L1 by fragment molecular orbital method. Sci Rep-Uk. 2019;9:16727. doi:10.1038/s41598-019-53216-z. PMID: WOS:000496135600005.
- Chen LP. Co-inhibitory molecules of the B7-CD28 family in the control of T-cell immunity. Nat Rev Immunol. 2004;4:336–47. doi:10.1038/nri1349. PMID: WOS:000221183300012.
- Xu JM, Shen J, Gu SZ, Zhang Y, Wu LH, Wu J, Shao GL, Zhang YQ, Xu L, Yin T, et al. Camrelizumab in combination with apatinib in patients with advanced hepatocellular carcinoma (RESCUE): a for nonrandomized, open-label, phase II trial. Clin Cancer Res. 2021;27:1003–11. doi:10.1158/1078-0432.Ccr-20-2571. PMID: WOS:000620168400015.
- Lu M, Zhang PP, Zhang YQ, Li ZW, Gong JF, Li J, Li J, Li Y, Zhang XT, Lu ZH, et al. Efficacy, safety, and biomarkers of toripalimab in patients with recurrent or metastatic neuroendocrine neoplasms: a multiple-center phase Ib trial. Clin Cancer Res. 2020;26:2337–45. doi:10.1158/1078-0432.Ccr-19-4000. PMID: WOS:000535265900008.
- Tang BX, Yan XQ, Sheng XA, Si L, Cui CL, Kong Y, Mao LL, Lian B, Bai X, Wang X, et al. Safety and clinical activity with an anti-PD-1 antibody JS001 in advanced melanoma or urologic cancer patients. J Hematol Oncol. 2019;12:7. doi:10.1186/s13045-018-0693-2. PMID: WOS:000455639900001.
- Yang JL, Dong LH, Yang S, Han XH, Han Y, Jiang SY, Yao JR, Zhang ZS, Zhang SX, Liu P, et al. Safety and clinical efficacy of toripalimab, a PD-1 mAb, in patients with advanced or recurrent malignancies in a phase I study. Eur J Cancer. 2020;130:182–92. doi:10.1016/j.ejca.2020.01.028. PMID: WOS:000535711100019.
- Motzer RJ, Escudier B, McDermott DF, George S, Hammers HJ, Srinivas S, Tykodi SS, Sosman JA, Procopio G, Plimack ER, et al. Nivolumab versus everolimus in advanced renal-cell carcinoma. New Engl J Med. 2015;373:1803–13. doi:10.1056/NEJMoa1510665. PMID: WOS:000364144000004.
- Brahmer J, Reckamp KL, Baas P, Crino L, Eberhardt WEE, Poddubskaya E, Antonia S, Pluzanski A, Vokes EE, Holgado E, et al. Nivolumab versus docetaxel in advanced squamous-cell non-small-cell lung cancer. New Engl J Med. 2015;373:123–35. doi:10.1056/NEJMoa1504627. PMID: WOS:000357598600005.
- Borghaei H, Paz-Ares L, Horn L, Spigel DR, Steins M, Ready NE, Chow LQ, Vokes EE, Felip E, Holgado E, et al. Nivolumab versus docetaxel in advanced nonsquamous non-small-cell lung cancer. New Engl J Med. 2015;373:1627–39. doi:10.1056/NEJMoa1507643. PMID: WOS:000363317800008.
- Hellmann MD, Ciuleanu TE, Pluzanski A, Lee JS, Otterson GA, Audigier-Valette C, Minenza E, Linardou H, Burgers S, Salman P, et al. Nivolumab plus ipilimumab in lung cancer with a high tumor mutational burden. New Engl J Med. 2018;378:2093–104. doi:10.1056/NEJMoa1801946. PMID: WOS:000433428000007.
- Overman MJ, McDermott R, Leach JL, Lonardi S, Lenz H-J, Morse MA, Desai J, Hill A, Axelson M, Moss RA, et al. Nivolumab in patients with metastatic DNA mismatch repair-deficient or microsatellite instability-high colorectal cancer (CheckMate 142): an open-label, multicentre, phase 2 study. Lancet Oncol. 2017;18:1182–91. doi:10.1016/s1470-2045(17)30422-9. PMID: WOS:000408873500042.
- El-Khoueiry AB, Sangro B, Yau T, Crocenzi TS, Kudo M, Hsu C, Kim TY, Choo SP, Trojan J, Welling TH III, et al. Nivolumab in patients with advanced hepatocellular carcinoma (CheckMate 040): an open-label, non-comparative, phase 1/2 dose escalation and expansion trial. Lancet. 2017;389:2492–502. doi:10.1016/s0140-6736(17)31046-2. PMID: WOS:000403901800028.
- Johnson D, Balko J, Compton M, Chalkias S, Gorham J, Xu Y, Hicks M, Puzanov I, Alexander M, Bloomer T, et al. Fulminant myocarditis with combination immune checkpoint blockade. New Engl J Med. 2016;375:1749–55. doi:10.1056/NEJMoa1609214. PMID: WOS:000387007300008.
- Valsecchi ME. Combined nivolumab and ipilimumab or monotherapy in untreated melanoma. New Engl J Med. 2015;373:1270–1270. doi:10.1056/NEJMc1509660. PMID: WOS:000361635200016.
- Garon EB, Rizvi NA, Hui R, Leighl N, Balmanoukian AS, Eder JP, Patnaik A, Aggarwal C, Gubens M, Horn L, et al. Pembrolizumab for the treatment of non-small-cell lung cancer. New Engl J Med. 2015;372:2018–28. doi:10.1056/NEJMoa1501824. PMID: WOS:000354809300008.
- Zhu AX, Finn RS, Edeline J, Cattan S, Ogasawara S, Palmer D, Verslype C, Zagonel V, Fartoux L, Vogel A, et al. Pembrolizumab in patients with advanced hepatocellular carcinoma previously treated with sorafenib (KEYNOTE-224): a non-randomised, open-label phase 2 trial. Lancet Oncol. 2018;19:940–52. doi:10.1016/s1470-2045(18)30351-6. PMID: WOS:000437342400053.
- Nanda R, Chow LQM, Dees EC, Berger R, Gupta S, Geva R, Pusztai L, Pathiraja K, Aktan G, Cheng JD, et al. Pembrolizumab in patients with advanced triple-negative breast cancer: phase Ib KEYNOTE-012 study. J Clin Onco. 2016;34:2460–67. doi:10.1200/jco.2015.64.8931. PMID: WOS:000381497000005.
- Bellmunt J, de Wit R, Vaughn DJ, Fradet Y, Lee JL, Fong L, Vogelzang NJ, Climent MA, Petrylak DP, Choueiri TK, et al. Pembrolizumab as second-line therapy for advanced urothelial carcinoma. New Engl J Med. 2017;376:1015–26. doi:10.1056/NEJMoa1613683. PMID: WOS:000396403700006.
- Adams S, Schmid P, Rugo HS, Winer EP, Loirat D, Awada A, Cescon DW, Iwata H, Campone M, Nanda R, et al. Pembrolizumab monotherapy for previously treated metastatic triple-negative breast cancer: cohort a of the phase II KEYNOTE-086 study. Ann Oncol. 2019;30:397–404. doi:10.1093/annonc/mdy517. PMID: WOS:000465084000012.
- Scapin G, Yang X, Prosise WW, McCoy M, Reichert P, Johnston JM, Kashi RS, Strickland C. Structure of full-length human anti-PD1 therapeutic IgG4 antibody pembrolizumab. Nat Struct Molecul Biol. 2015;22:953–58. doi:10.1038/nsmb.3129. PMID: WOS:000366152400007.
- Zhang T, Song X, Xu L, Ma J, Zhang Y, Gong W, Zhang Y, Zhou X, Wang Z, Wang Y, et al. The binding of an anti-PD-1 antibody to FcγRΙ has a profound impact on its biological functions. Cancer Immunol Immun. 2018;67(7):1079–90. doi:10.1007/s00262-018-2160-x. PMID: WOS:000435591800005.
- Zhang T, Song XM, Xu LL, Ma J, Zhang YJ, Gong WF, Zhang YL, Zhou XS, Wang ZB, Wang YL, et al. The binding of an anti-PD-1 antibody to FcγRΙ has a profound impact on its biological functions. Cancer Immunol Immun. 2018;67(7):1079–90. doi:10.1007/s00262-018-2160-x. PMID: WOS:000435591800005.
- Yu R, Wang W, Li T, Li J, Zhao K, Wang W, Liang L, Wu H, Ai T, Huang W, et al. RATIONALE 311: tislelizumab plus concurrent chemoradiotherapy for localized esophageal squamous cell carcinoma. Future Oncol. 2021;17(31):4081–89. doi:10.2217/fon-2021-0632. PMID: WOS:000674048800001.
- Song Y, Gao Q, Zhang H, Fan L, Zhou J, Zou D, Li W, Yang H, Liu T, Wang Q, et al. Treatment of relapsed or refractory classical Hodgkin lymphoma with the anti-PD-1, tislelizumab: results of a phase 2, single-arm, multicenter study. Leukemia. 2020;34:533–42. doi:10.1038/s41375-019-0545-2. PMID: WOS:000523481800020.
- Socinski MA, Jotte RM, Cappuzzo F, Orlandi F, Stroyakovskiy D, Nogami N, Rodriguez-Abreu D, Moro-Sibilot D, Thomas CA, Barlesi F, et al. Atezolizumab for first-line treatment of metastatic nonsquamous NSCLC. New Engl J Med. 2018;378:2288–301. doi:10.1056/NEJMoa1716948. PMID: WOS:000435099900006.
- Rosenberg JE, Hoffman-Censits J, Powles T, van der Heijden MS, Balar AV, Necchi A, Dawson N, O’Donnell PH, Balmanoukian A, Loriot Y, et al. Atezolizumab in patients with locally advanced and metastatic urothelial carcinoma who have progressed following treatment with platinum-based chemotherapy: a single-arm, multicentre, phase 2 trial. Lancet. 2016;387:1909–20. doi:10.1016/s0140-6736(16)00561-4. PMID: WOS:000375374200035.
- Horn L, Mansfield AS, Szczesna A, Havel L, Krzakowski M, Hochmair MJ, Huemer F, Losonczy G, Johnson ML, Nishio M, et al. First-line atezolizumab plus chemotherapy in extensive-stage small-cell lung cancer. New Engl J Med. 2018;379:2220–29. doi:10.1056/NEJMoa1809064. PMID: WOS:000452259200007.
- Herbst RS, Giaccone G, de Marinis F, Reinmuth N, Vergnenegre A, Barrios CH, Morise M, Felip E, Andric Z, Geater S, et al. Atezolizumab for first-line treatment of PD-L1-selected patients with NSCLC. New Engl J Med. 2020;383:1328–39. doi:10.1056/NEJMoa1917346. PMID: WOS:000575616600009.
- Emens LA, Cruz C, Eder JP, Braiteh F, Chung C, Tolaney SM, Kuter I, Nanda R, Cassier PA, Delord JP, et al. Long-term clinical outcomes and biomarker analyses of atezolizumab therapy for patients with metastatic triple-negative breast cancer a phase 1 study. JAMA Oncol. 2019;5:74–82. doi:10.1001/jamaoncol.2018.4224. PMID: WOS:000455776100015.
- De Meyer T, Muyldermans S, Depicker A. Nanobody-based products as research and diagnostic tools. Trends Biotechnol. 2014;32:263–70. doi:10.1016/j.tibtech.2014.03.001. PMID: WOS:000335625700006.
- De Genst E, Silence K, Decanniere K, Conrath K, Loris R, Kinne R, Muyldermans S, Wyns L. Molecular basis for the preferential cleft recognition by dromedary heavy-chain antibodies. P Natl Acad Sci Usa. 2006;103:4586–91. doi:10.1073/pnas.0505379103. PMID: WOS:000236362600048.
- Nguyen VK, Su C, Muyldermans S, van der Loo W. Heavy-chain antibodies in camelidae; a case of evolutionary innovation. Immunogenet. 2002;54:39–47. doi:10.1007/s00251-002-0433-0. PMID: WOS:000175617900005.
- Barderas R, Desmet J, Timmerman P, Meloen R, Casal JI. Affinity maturation of antibodies assisted by in silico modeling. Proc Natl Acad Sci USA. 2008;105(26):9029–34. doi:10.1073/pnas.0801221105. PMID: WOS:000257354400042.
- Lippow SM, Wittrup KD, Tidor B. Computational design of antibody-affinity improvement beyond in vivo maturation. Nat Biotechnol. 2007;25:1171–76. doi:10.1038/nbt1336. PMID: WOS:000250226600029.
- Barderas R, Shochat S, Timmerman P, Hollestelle MJ, Martinez-Torrecuadrada JL, Hoeppener JWM, Altschuh D, Meloen R, Casal JI. Designing antibodies for the inhibition of gastrin activity in tumoral cell lines. Int J Cancer. 2008;122(10):2351–59. doi:10.1002/ijc.23395. PMID: WOS:000254983100024.
- Lippow SM, Tidor B. Progress in computational protein design. Curr Opin Biotechnol. 2007;18:305–11. doi:10.1016/j.copbio.2007.04.009. PMID: WOS:000249980400003.
- Shin J, Raissi S, Phelan P, Bullock PA. Rational design of a nivolumab-based ANTI-PD-1 single chain variable fragment that blocks the interaction between PD-1 expressed on T-CELLS and PD-L1 on CHO cells. Protein Expres Purif. 2023;202:106196. doi:10.1016/j.pep.2022.106196. PMID: WOS:000917702900001.
- Radaev S, Sun P. Recognition of immunoglobulins by Fcγ receptors. Molecular Immunol. 2002;38(14):1073–83. doi:10.1016/s0161-5890(02)00036-6. PMID: WOS:000175986600008.
- Yamaguchi Y, Barb AW. (2020). A synopsis of recent developments defining how N-glycosylation impacts immunoglobulin G structure and function. Glycobiology, 30(4):214–225. doi:10.1093/glycob/cwz068.
- Yi M, Zheng XL, Niu MK, Zhu SL, Ge H, Wu KM. Combination strategies with PD-1/PD-L1 blockade: current advances and future directions. Mol Cancer. 2022;21:28. doi:10.1186/s12943-021-01489-2. PMID: WOS:000745440900002.
- Wang Q, Cao YL, Shen LJ, Xiao TR, Cao RY, Wei SK, Tang M, Du LY, Wu HY, Wu B, et al. Regulation of PD-L1 through direct binding of cholesterol to CRAC motifs. Sci Adv. 2022;8(34):eabq4722. doi:10.1126/sciadv.abq4722. PMID: WOS:000847345000038.