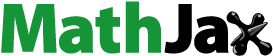
ABSTRACT
The concentration and sources of organic nitrogen (ON) in lake sediments affect lake nitrogen cycles. However, the factors influencing ON accumulation rate (ONAR) are unclear. We collected 3 sediment cores from northern, eastern, and southern Dianchi Lake (DC-N, DC-E, and DC-S, respectively) in July 2014, to study the effects of autochthonous and allochthonous sources of ON. The ON and ONAR increased 2.4–5.1 and 2.6–4.8 times, respectively, from 1900 to 2000, especially since the 1980s, when algal blooms have occurred more frequently. The ON decreased in the order DC-S > DC-N > DC-E, whereas the ONAR decreased in the order DC-N > DC-S > DC-E, suggesting that ONAR was influenced by ON content as well as the depositional environment. The total concentrations of n-alkanes (n-C12 to n-C34) ranged from 4719 to 61 960 ng g−1 in the 3 sediment cores, with proportions varying with vertical depth. The sources of ON were mainly allochthonous (soil erosion and terrestrial plants) and autochthonous (algal and aquatic plants) in DC-S and DC-N, respectively, and primarily mixed planktonic and terrestrial in DC-E. The stochastic impacts by regression on population, affluence, and technology (STIRPAT) model revealed that a 1% increase in air temperature and nitrogen fertilizer corresponded to an increase in ONAR by 23–33% and 20–79% in the Dianchi Lake basin, especially in DC-S and DC-E. However, a 1% increase in urban land area reduced ONAR by 2–11%, especially in DC-N. Our study suggests that the spatial and temporal ONAR in Dianchi Lake may increase in response to a warmer and wetter climate combined with increasing chemical nitrogen fertilizer application.
Introduction
Nitrogen is a limiting factor for the primary production of ecosystems and has become a major topic in climate change research over the past few decades (Galloway et al. Citation2008, Fowler et al. Citation2013). Lacustrine deposits play a vital role in overall nutrient cycling in lake ecosystems, especially regarding the nitrogen (N) cycle (Elser et al. Citation2007, Conley et al. Citation2009). Lacustrine N has many forms with different biogeochemical characteristics. Sedimentary organic nitrogen (ON), the main form of N (Zolitschka et al. Citation2015, Huang et al. Citation2017b), accumulates in the sediment and is easily converted to ammonium (NH4+), nitrite (NO2−), and nitrate (NO3−) via mineralization and nitrification (Wu et al. Citation2019, Wong et al. Citation2021). A part of the converted NH4+ and NO3− can be released into the overlying water, which may cause algal blooms (Galman et al. Citation2008, Yu et al. Citation2018). The effects of algal residues, considered important autochthonous sources, on organic matter (OM) burial have been widely studied (Anderson et al. Citation2014, Leithold et al. Citation2016). While carbon is a major element in OM and has been widely reported, research concerning N is limited (Anderson et al. Citation2013, Jiang et al. Citation2020).
Recent studies have proposed that the sources of sedimentary ON are complex and include municipal sewage, agricultural fertilizer, bacteria, algae, and terrestrial and aquatic plants (Leithold et al. Citation2016, Wang et al. Citation2020). In addition, most previous studies have found that the hydrological characteristics, including trophic state, climate, and vegetation, in lake watersheds affect OM accumulation in sediment (Downing et al. Citation2008, Anderson et al. Citation2013, Citation2014). For instance, Heathcote et al. (Citation2015) revealed that climate change could significantly increase organic carbon accumulation in northern lakes. However, most previous research has focused on boreal lakes while some has focused on temperate-zone lakes to determine the burial efficiency and influencing factors of OM burial over different time scales (Anderson et al. Citation2013, Citation2014, Heathcote et al. Citation2015, Fortino et al. Citation2016, Jiang et al. Citation2020). Dianchi Lake, the largest subtropical plateau lake in Southwest China, has not only been affected by a variety of climatic features (such as monsoons, high-altitude and low-latitude climates), but also by eutrophication and intensive human activities (such as deforestation, urbanization, and fertilization) since 1970 (Gao et al. Citation2015, Chen et al. Citation2020). Eutrophication is considered an important driver of increasing OM as a result of not only the enrichment of nutrients in lake water but also perennial climate change in this lake (Huang et al. Citation2017b, Chen et al. Citation2020). In addition, the sources of OM and the relative allochthonous and autochthonous contributions directly determine the ON burial efficiency and mineralization rate (Hockun et al. Citation2016). However, because of high spatial variability and limited monitoring of lake sediments, the composition of the increased sedimentary ON has not been determined. Therefore, investigating the effect of human activities on the spatial and temporal sediment ON accumulation rate (ONAR) by using multiple lake sediment samples could provide a comprehensive understanding of the lake N cycle under climate change.
n-alkanes, widely present in bacteria, algae, and aquatic and terrestrial plants, are considered ideal biomarkers for tracing the source of OM under environmental and climatic changes because of their degradation resistance (Mead et al. Citation2005, Fang et al. Citation2014, Liu and Liu Citation2016). The distribution of the n-alkanes carbon content in bacteria and algae ranges from n-C15 to n-C20. The n-alkanes distribution in aquatic macrophytes and crude oil is dominated by mid-chain alkanes (n-C20 to n-C25). Terrestrial higher plants are typically characterized by a high abundance of long-chain n-alkanes (n-C27 to n-C33; Ficken et al. Citation2000, Liu and Liu Citation2016). Complex relationships exist between climate change and human activities, which obscure the effect of individual factors on ON burial. The stochastic impacts by regression on population, affluence, and technology (STIRPAT) model can be used to statistically analyze the nonmonotonic or nonproportional impacts of driving factors on the environment (Dietz and Rose Citation1994, York et al. Citation2003). STIRPAT has been successfully utilized to explore the influence of human factors and analyze the effects of driving forces on a variety of environments, including carbon dioxide emissions and environmental changes (Wang et al. Citation2013, Zhou and Liu Citation2016).
Our hypotheses were that (1) sedimentary ON accumulation rates were highly spatial and temporal variation in Dianchi Lake because of the different autochthonous and allochthonous OM sources, and (2) over the past hundred years, the human activities from chemical N fertilizer and urban land have played an increasingly important role of ONAR under climate change. Therefore, we combined sediment chronology and n-alkanes indicators to explore the contributions of autochthonous and allochthonous OM to ON sources from 3 sediment cores in Dianchi Lake spanning the last century. In addition, we quantitatively evaluated the effects of climate change and human activities on sedimentary ONAR in different regions of Dianchi Lake by using the STIRPAT model.
Materials and methods
Study area
Dianchi Lake (24°40′–25°02′N, 102°36′–102°47′E) in Yunnan Province, southwest China, has an average altitude of 1886 m. It is the largest lake in the Yunnan Province and the sixth-largest freshwater lake in China. More than 20 rivers flow into Dianchi Lake while only 1 river flows out. The climate is subtropical, humid, and monsoonal. The annual mean air temperature is 14.8 °C. The rainy season occurs from May to October, contributing an average annual rainfall of 1000 mm. Red and purple soils are the main soil types in Dianchi Lake basin. The average and maximum water depths in Dianchi Lake are 5.1 and 10.9 m, respectively, its storage capacity is 1.57 billion m3, and the total area is 309 km2. Land use of the 2800 km2 lake basin is mainly forest (36%), agriculture (23%), and urban land (29%).
Sample collection and analysis of nitrogen and carbon
Three sediment cores were collected with a gravity corer in July 2014 from 3 diverse locations in southern, eastern, and northern Dianchi Lake: DC-S (30 cm), DC-E (27 cm), and DC-N (22 cm), respectively (). For each core, sediments were subsampled at 1 cm intervals, frozen, and then freeze-dried.
Figure 2. (a) Historical variations of air temperature and rainfall in Kunming City, (b) chemical nitrogen fertilizer application (1 Mg = 106 g) and urban land area in Kunming, and (c) total phosphorus (TP) and total nitrogen (TN) concentrations in Dianchi Lake.

Total nitrogen (TN) was determined after digestion using persulfate solution (K2S2O4 + NaOH) at 121 °C for 30 min. To determine the NH4-N and NO3-N contents, 2 mol L−1 KCl was added to the samples and shaken for 30 min. The filtrate was then extracted using a 0.45 μm membrane filter. NO3-N was directly determined using a UV-3600 spectrophotometer (Shimadzu Corp., Japan). The NH4-N content was analyzed using salicylic acid and hypochlorous acid salt spectrophotometry. The ON concentration was obtained by subtracting the inorganic N content, which includes NH4-N and NO3-N, from the TN content. Total organic carbon (TOC) was measured using a TOC analyzer (Shimadzu Corp., Japan).
n-alkanes determination and source identification
n-alkanes were extracted from a 2 g subsample using microwave extraction (100 °C, 10 min) with a dichloromethane-methanol mixed solution (93:7, v:v; Mead et al. Citation2005, Fang et al. Citation2014). The supernatant was obtained after extraction from 3 centrifugations. The solvent was replaced with n-hexane and concentrated to 1 mL 3 times via rotary evaporation at 40 °C. The concentrated solution was separated and purified by solid-phase extraction and concentrated to 1 mL via rotary evaporation. The n-alkanes were quantified by measuring the concentration of the fluent via gas chromatography-mass spectrometry (GC/MS-QP2010 Ultra, Shimadzu Corp., Japan). During this process the injection port temperature was gradually increased to 300 °C at a rate of 10 °C min−1 from its starting temperature of 50 °C.
The carbon preference index (CPI) and proxy of aquatic macrophyte input (Paq) are useful for identifying the sources of n-alkanes (Ficken et al. Citation2000, Sawada et al. Citation2020). CPI is widely used as a source indicator of n-alkanes, whereas Paq represents the proportion of n-alkanes in submerged plants among the mid-chain and long-chain n-alkanes (Hockun et al. Citation2016, Liu and Liu Citation2016). Their calculations are as follows:
(1)
(1)
(2)
(2)
(3)
(3) Cn represents different carbon numbers of n-alkanes. n-alkanes derived from land plants showed a predominance of odd-numbered carbon chains with CPI2 (CPI25∼33) while CPI1 (CPI15∼23) values close to 1 with the preference of n-C15 or n-C17 may indicate a greater input from floating aquatic plants (Liu and Liu Citation2016, Wang et al. Citation2019). Paq ≤ 0.1, 0.1 ≤ Paq < 0.4, and 0.4 ≤ Paq < 1 suggested OM sources mainly from terrestrial higher plants, mixed terrestrial higher plants and emergent aquatic plants, and incorporated submerged plants with macrophytoplankton, respectively (Ficken et al. Citation2000, Zhou et al. Citation2010).
Chronology and accumulation rates of ON
The 210Pb radiometric technique has been used to analyze sediment chronologies (Smith Citation2001, Sanchez-Cabeza and Ruiz-Fernandez Citation2012). Here, a high-resolution HPGe γ-spectrometer (EG&GORTEC, GWL-120-15, USA) was used to determine the activities of 226Ra and 210Pb at a 40 000 s determination time. The difference between 226Ra and 210Pb is the activity of excess 210Pb (210Pbex). After determination, a constant rate of 210Pb supply model was applied to date the sediment cores (equation 4). The distribution of Pbex and the dating of the 3 sediment cores are detailed in Huang et al. (Citation2017b).
(4)
(4) where T0 is the sampling year (2014), λ is the decay constant of 210Pb (0.03114 yr−1), and A0 and Ah are the inventories of 210Pbex for the entire core and the section below depth h (Bq cm−2), respectively. The ONAR (g cm−2 yr−1) was calculated as follows:
(5)
(5) where ρ is the dry bulk density (g cm−3), Z is the depth (cm), t denotes time (yr), and C is the concentration of ON (mg g−1).
Supplemental data
Data on N fertilizer use and land area under urban land were collected from the National Bureau of Statistics (http://data.cnki.net/). We downloaded the air temperature and rainfall data from the Chinese Meteorological Data Sharing Service System (http://cdc.cma.gov.cn). The concentrations of TN and total phosphorus (TP) in the overlying water were collected from previous studies that conducted in situ measurements (He et al. Citation2015, Zhou et al. Citation2016; Fig. 2).
STIRPAT model
The STIRPAT model, originally named IPAT, was proposed by Ehrlich and Holdren (Citation1971) and used to describe the effects of human activities on the environment in 1994 (Dietz and Rose Citation1994). To overcome various model weaknesses, the model was reformulated using reasonable equations and became the STIRPAT model. Because all variables were in logarithmic form to facilitate estimation and hypothesis testing, they modified the STIRPAT model to a logarithmic regression model (York et al. Citation2003), thereby developing the current model:
(6)
(6) where Fit represents relevant factors (e.g., population size, gross domestic product, and technology), α is a constant, εit is an error term, and ait denotes the elasticity of the corresponding parameters, wherein the elasticity value indicates that a 1% increase in F induces a certain percent change in Iit. In some studies, the model can be extended by adding other factors with a logical correlation to a specific environment (Wang et al. Citation2013). On this basis, to comprehensively examine the relevant factors driving ON loads entering the lake, the extended STIRPAT model was amended by adding meteorological factors (e.g., air temperature). In this study, an ordinary least squares regression was employed after testing for an absence of multicollinearity in the variables. If multicollinearity occurred, a ridge regression was selected to increase the significance of the model.
Results
Sedimentary ON concentration and accumulation rate
The concentration of ON increased gradually over the study period. Specifically, the ON contents increased from 596 to 6140 mg kg−1, 347.1 to 2848 mg kg−1, and 298 to 2752.4 mg kg−1 in DC-S, DC-E, and DC-N, respectively (a). Overall, the ON contents (standard deviation in brackets) increased from 376 (232) mg kg−1 in 1900 to 4537 (1735) mg kg−1 in 2012 over a 112 yr period. Significant increases in ON were observed after 1960 in DC-N and DC-S, and after 1980 in DC-E. Since 1960, the mean values of ON declined in the order DC-S (2630 [1605] mg kg−1) > DC-N (1575 [978] mg kg−1) > DC-E (1084 [913] mg kg−1). The highest ON content (6140 mg kg−1) was observed in DC-S in 2000.
Figure 3. Profiles of the organic nitrogen (ON) concentration and organic nitrogen accumulation rates (ONAR) for 3 sediment cores from northern, eastern, and southern Dianchi Lake (DC-N, DC-E, and DC-S, respectively).

ONAR gradually decreased with vertical depth. It changed little before the 1970s, with mean values of 0.101 mg cm−2 yr−1, but significantly increased after, with mean values of 0.302 mg cm−2 yr−1 (b). The highest ONAR occurred on the surface in all 3 sediment cores, which from DC-S (1.21 mg cm−2 yr−1) is almost 2 times higher than DC-N (0.53 mg cm−2 yr−1) and DC-E (0.54 mg cm−2 yr−1). After the 1970s, the increase in the ONAR followed the order DC-N (0.38 mg cm−2 yr−1) > DC-S (0.28 mg cm−2 yr−1) > DC-E (0.24 mg cm−2 yr−1).
Distributions of n-alkanes
Variations in the n-alkanes characteristics were different in each of the 3 sediment cores (). The total concentrations of n-alkanes (TNA; n-C12 to n-C34) were 22 210 (9022–61 960) ng g−1, 10 618 (4719–18 240) ng g−1, and 15 277 (5770–28 091) ng g−1 in DC-S, DC-N, and DC-E, respectively. For DC-S, the aliphatic hydrocarbon fractions were mainly from allochthonous-derived long-chain n-C27 to n-C31 alkanes in the surface 10 cm and then became autochthonous-derived short-chain n-C17 alkanes at 10–20 cm. Meanwhile, DC-E exhibited bimodal behavior, with long-chain n-C27 to n-C31 alkanes and short-chain n-C17 alkanes on its surface, which declined with depth. After 1980 (0–10 cm), short- and mid-chain n-alkanes (n-C14 to n-C25), especially n-C17, tended to be larger contributors, suggesting the sources of OM varied (between mixed planktonic and terrestrial sources) and the phytoplankton contribution increased from the bottom to the top of the sediment core. For DC-N, only one clear peak with short-chain n-C17 alkanes was found on the surface, which gradually decreased with depth. In the lower section (1906–1965 AD), the TNA value was relatively low, exhibiting a bimodal distribution (n-C16 and n-C18). It then decreased gradually from 0 to 13 cm, corresponding to 2012 and 1965, respectively. The n-alkanes distribution indicated an abundance of short-chain n-alkanes (n-C14 to n-C20) with a peak value at C17. Overall, before 1958 the TNA values were low, whereas after 1958 (16 cm) the TNA values increased, and the n-alkanes distribution showed an abundance of short-chain n-alkanes (n-C14 to n-C20) with a peak value at C17, indicating the n-alkanes in the north came predominantly from bacteria or fungal lipids and fossil fuel combustion.
C:N and n-alkanes ratios
The C:N ratio of 3 sediment cores ranged from 4.1 to 18.6 from 1900 to 2012 (). For DC-S and DC-E, the C:N ratio variation was small, mostly fluctuating from 8.3 to 15.4 and 4.1 to 10.3, respectively. For DC-N, before the 1960s, the C:N ratio was constant at ∼8, whereas it significantly increased to 18.6 from the 1960s to the 1980s and then decreased to ∼12 until 2012. The fluctuating increasing trend of CPI1 and CPI2 values were similar with the same C:N ratio. The mean CPI1 values varied from 0.76 (0.31), to 0.64 (0.34), and 0.66 (0.14) in DC-S, DC-N, and DC-E, respectively. Notably, the mean CPI1 values were ∼0.5 before the 2000s, whereas they increased to 1.3 from DC-S. The mean CPI2 values varied between 0.5 and 3.1, with average values of 2.1 (0.8) for DC-S, 1.4 (0.5) for DC-N, and 1.1 (0.6) for DC-E, respectively, and were >1.0 in all samples from DC-S. The mean Paq were similar between DC-S (0.61 [0.27]) and DC-N (0.60 [0.21]) and higher than DC-E (0.43 [0.26]).
Figure 5. Organic nitrogen (ON) concentration, C:N ratio, and n-alkane source diagnostic ratio profiles for 3 sediment cores in Dianchi Lake where: CPI1 = 1/2 × [∑odd(C15-C23)/∑even(C14-C22) + ∑odd(C15-C23)/∑even(C16-C24)], CPI2 = 1/2 × [∑odd(C25-C33)/∑even(C24-C32) + ∑odd(C25-C33)/∑even(C26-C34)], and Paq = (C23 + C25)/(C23 + C25 + C29 + C31). A C:N ratio of 4–10 indicates that the organic matter (OM) mainly originated from phytoplankton and algae. When the C:N ratio is ≥12, the OM is mainly from terrestrial higher plants. Paq ≤ 0.1, 0.1 ≤ Paq < 0.4, and 0.4 ≤ Paq < 1 suggests OM sources are mainly derived from terrestrial higher plants, mixed terrestrial higher plants and emergent aquatic plants, and incorporated submerged plants with macrophytoplankton, respectively.
![Figure 5. Organic nitrogen (ON) concentration, C:N ratio, and n-alkane source diagnostic ratio profiles for 3 sediment cores in Dianchi Lake where: CPI1 = 1/2 × [∑odd(C15-C23)/∑even(C14-C22) + ∑odd(C15-C23)/∑even(C16-C24)], CPI2 = 1/2 × [∑odd(C25-C33)/∑even(C24-C32) + ∑odd(C25-C33)/∑even(C26-C34)], and Paq = (C23 + C25)/(C23 + C25 + C29 + C31). A C:N ratio of 4–10 indicates that the organic matter (OM) mainly originated from phytoplankton and algae. When the C:N ratio is ≥12, the OM is mainly from terrestrial higher plants. Paq ≤ 0.1, 0.1 ≤ Paq < 0.4, and 0.4 ≤ Paq < 1 suggests OM sources are mainly derived from terrestrial higher plants, mixed terrestrial higher plants and emergent aquatic plants, and incorporated submerged plants with macrophytoplankton, respectively.](/cms/asset/2b2710fa-c34d-4a30-987b-084a2d0a2de0/tinw_a_2208515_f0005_oc.jpg)
Elasticity of driving factors
Our previous result showed that air temperature, land use change, economic development, and population growth were strongly related to sedimentary OM variations (Huang et al. Citation2017b). On this basis, we selected 2 natural factors (air temperature, rainfall) and 2 anthropogenic factors (chemical N fertilizer, urban land) to investigate their effect on sedimentary ON variations in different regions of Dianchi Lake using the STIRPAT model (). Rainfall was the primary influencing factor in DC-S and DC-N, whereas chemical N fertilizer was the most important factor in DC-E. A 1% increase in air temperature was correlated with 33%, 23%, and 31% increases in ONAR from DC-S, DC-E, and DC-N, respectively. A 1% increase in rainfall increased ONAR by 50% and 57% in DC-S and DC-N, respectively, whereas it reduced by 0.1% in DC-E. In addition, a 1% increase in chemical N fertilization was correlated with an increase in ONAR of 34%, 79%, and 20% in DC-S, DC-E, and DC-N, respectively. Conversely, a 1% increase in urban land area was correlated with a reduced ONAR of 11%, 9%, and 2% in DC-S, DC-E, and DC-N, respectively.
Figure 6. Elasticities of organic nitrogen (ON) contents regarding air temperature, rainfall, nitrogen fertilizer, and urban land. Bars denote the estimated elasticity for ON while error bars show 95% confidence interval values obtained using the stochastic impacts by regression on population, affluence, and technology (STIRPAT) model. Elasticities indicate the effect on the ON content (%) of a 1% increase in the independent variables.

Discussion
Sources of organic nitrogen
In general, the sources of ON in lake sediments are complex, but mainly include autochthonous (e.g., algae, aquatic plants, and animals) and allochthonous (e.g., soil erosion, atmospheric deposition, and living or aquaculture wastewater) sources. Therefore, the sources of ON in the 3 sediment cores varied. In the DC-S core, the correlations between the short-chain n-alkanes and ON at the upper part of the core (1–14 cm, 2012–1981) were negative (−0.80 to −0.36), whereas at the bottom core (15–32 cm, 1978–1903) they were positive (0.86–0.87). In addition, the correlations were positive between ON and the mid-chain (0.15–0.72) and long-chain (0.03–0.44) n-alkanes. The CPI2 values ranged from 1.20 to 2.95 with a mean of 1.87 (), indicating a greater input from microorganisms, recycled OM, and petroleum (Choi and Lee Citation2013, Liu and Liu Citation2016). Meanwhile, Paq values were higher (0.54 [0.10]), indicating that the ON was primarily derived from allochthonous microorganisms and floating aquatic plants in the southern lake (Han and Calvin Citation1969, Jeng Citation2006). More recently, the contribution to ON content was mostly by sources different from those associated with short-chain n-alkanes, consistent with the replacement of a large amount of forest land by farmland in the last 3 decades and its higher risk of soil erosion (Quinton et al. Citation2010, Huang et al. Citation2014). In addition, the proportion of N fertilizer utilization by crops was estimated at only 30–40% (Ju et al. Citation2009), suggesting a large amount of N fertilizer directly discharged into DC-S.
In DC-E, ON exhibited a positive correlation with short-chain n-alkanes, mainly from bacteria and algae (Mead et al. Citation2005, Fang et al. Citation2014). In addition, the relevant Paq (0.37 [0.05]), CPI1 (0.66 [0.14]), and CPI2 (1.51 [0.34]) values for DC-E were lower than those in other cores, suggesting that n-alkanes in DC-E were derived from a mixed OM source of submerged and floating aquatic plants and terrigenous higher plants (; Wang et al. Citation2014, Sawada et al. Citation2020). This finding is consistent with previous cluster analysis results showing that flower and vegetable cultivation with high fertilization increased the contribution of exogenous N to DC-E (Huang et al. Citation2017a). The correlation between ON and the mid- and short-chain n-alkanes was most significant in DC-N, especially for C17 (R2 = 0.87). Thus, the main sources of ON were short-chain n-alkanes, which are mainly derived from endogenous biomass and allochthonous origins (Wang et al. Citation2014, Zhan et al. Citation2020). In addition, the mean CPI1 (0.91 [0.14]) was close to 1, with mostly n-C17, indicating the short-chain n-alkanes were mainly derived from endogenous algae and phytoplankton (Hockun et al. Citation2016, Liu and Liu Citation2016). Furthermore, the C:N ratio increased from 6.6 (2.9) to 12.4 (3.4) in the last 4 decades, suggesting the contribution of allochthonous organic matter brought by human activities (such as sewage, soil erosion) is gradually increasing in recent years. The north side of Dianchi Lake is near Kunming City and is thus heavily influenced by human activities such as sewage and industrial effluent processes (He et al. Citation2015). Previous studies have indicated that eutrophication first observed in DC-N in 1970 was a result of rapid economic development and urbanization of Kunming City (Huang et al. Citation2014, Chen et al. Citation2020). Overall, endogenous algae and phytoplankton are the primary contributors to the source of ON in DC-N.
Influencing factors on the organic nitrogen burial
Dianchi Lake is a typical plateau-type hydrostatic lake, with a long water residence of 3.9 years (Huang et al. Citation2018). A previous study indicated that only ∼20% of pollutants are removed by different processes in Dianchi Lake and via the outflow while the rest remain in the sediment, allowing them to be released into the overlying water under appropriate conditions (He et al. Citation2015). This phenomenon is one reason Dianchi Lake sediment has higher ON content (294–6140 mg kg−1) than other eutrophic lake sediments, such as Chaohu Lake (195–1076 mg kg−1) and Taihu Lake (278–4687 mg kg−1; Yu et al. Citation2018, Wu et al. Citation2019). In addition, the specific conditions of the sediment core sampling region affect ON burial. For instance, one reason the ON concentration in the DC-E sediment is lowest of the observed cores is because the water depth in DC-E is often <30 cm, causing it to be easily affected by the prevailing southwest winds (a; Chen et al. Citation2007). Therefore, the formation type and hydrological characteristics of lakes are some of the most important factors affecting ON burial.
A previous Taihu Lake study indicated that increasing precipitation intensity and frequency could cause more nutrients to flow into the lake (Paerl et al. Citation2011). For our study region, the annual precipitation gradually decreased over the last 3 decades, according to the Kunming Meteorological Bureau record (a). Moreover, the discharge of industrial wastewater from Kunming City decreased from 152 million tonnes in 1990 to 31 million tonnes in 2010 as a result of strict water pollution management (Huang et al. Citation2014, He et al. Citation2015). However, the ONAR values of the Dianchi Lake sediment cores significantly increased from 0.37 mg kg−1 in 2000 to 0.76 mg kg−1 in 2010 (b), suggesting they might be affected by other environmental conditions, such as air temperature. Increasing air temperature enhances gross primary productivity as well as terrestrial and aquatic vegetation via photosynthetic rate acceleration and growing season extension (Larsen et al. Citation2011, Chen et al. Citation2020). The results obtained are consistent with those of previous studies, revealing that TP decreased in Dianchi Lake between 2000 and 2010, when algal blooms began to occur frequently (c; Wang et al. Citation2020).
In addition to climate change, human activities (e.g., tillage, fertilization, and land-use change) are also considered important factors influencing ON burial in lacustrine sediments. The average ONAR increased 2.7–4.6 times from 1995 to 2012 in the 3 sediment cores (b), corresponding to chemical N fertilizer use, which increased from 5 × 104 Mg (1 Mg = 106 g) to 9 × 104 Mg (b). Inappropriate/excess fertilization reportedly results in a high risk of N fertilizer loss into the lake via precipitation runoff and leaching, which is then rapidly utilized by algae and aquatic plants (Gao et al. Citation2015). In addition, the ON content from crop residue could increase because of N fertilizer application, which indirectly stimulates the ONAR burial in the lake sediments. Moreover, urban land in the Dianchi Lake basin increased sharply from 137 km2 in 1995 to 842 km2 in 2012 (b), accompanied by a large amount of topsoil erosion due to anthropogenic activity (Guzman et al. Citation2013, Huang et al. Citation2017a). A previous study revealed that fluvial sediment yield in western China's rivers increased 1.9 times compared with the background value because of agricultural land use (Schmidt et al. Citation2018). Therefore, high-intensity human activities, such as agricultural production and urbanization, significantly stimulate ONAR.
Overall, air temperature could significantly stimulate ONAR in all 3 sediment cores, especially in DC-S, where a 1% increase in air temperature resulted in a 33% increase in ONAR, demonstrating that warmer temperatures improve biological productivity and promote N deposition in lakes by increasing phytoplankton and microbial activity (Larsen et al. Citation2011, Chen et al. Citation2020). In addition, rainfall significantly increased ONAR values in the DC-S and DC-N cores but was only weakly reduced in DC-E because DC-E is too shallow to deposit ON, which may cause precipitation to have a dilution effect on the ON intensity. Conversely, a higher ON content occurred in DC-N as a result of rainfall, wherein sewage and domestic pollutants flow into the lake because of the high proportion of urban land in Kunming City (Gao et al. Citation2015, Chen et al. Citation2020). However, the elasticity of chemical N fertilizer was higher than that of other factors in DC-E, in which a 1% increase in the amount of N fertilizer increased the ON burial by 106%. In this region, flower and vegetable cultivation is the main land use type, which consumes a large amount of chemical N fertilizer (Huang et al. Citation2014, Gao et al. Citation2015). Furthermore, the elasticities of the urban land area were negative but insignificant for DC-E (). In general, increasing urban land could decrease effluent because the urban-built area significantly reduces the density of urban pollution and dilutes the concentration of pollutant discharge over a given period (Rickson Citation2014).
Conclusions
The ON content and burial in the sediments of Dianchi Lake showed significant spatial and temporal variation. Specifically, ON content declined in the order DC-S > DC-N > DC-E, whereas ONAR followed the order DC-N > DC-S > DC-E. In addition, ON content significantly increased in the 1960s, whereas the ONAR increased beginning in the 1980s. The ON sources were found to be both allochthonous and autochthonous and exhibited significant spatial and temporal variation. In addition to the characteristics of lakes, air temperature, rainfall, chemical N fertilization, and increasing urban land area are also important factors affecting the ONAR. Over the past 4 decades, the dominant contribution of chemical N fertilization to the ONAR was mainly apparent in the east and south of Dianchi Lake, whereas in the last 2 decades, warmer and wetter climate was the main contributor to the ONAR in the south and north of Dianchi Lake. Moreover, 1% increases in air temperature and N fertilizer could be correlated with an increase in ONAR by 74–86% and 73–151%, respectively, in all sediments, whereas a 1% increase in urban land area could be correlated with a reduction in the amount of ONAR by 2%–14%. In summary, climate change and human activities both contribute to the eutrophication of Dianchi Lake, directly and indirectly affecting the sedimentary ONAR variation. To better manage eutrophication in the Dianchi Lake basin, the administration should focus on chemical N fertilizer management under global warming and frequent rainfall, especially in eastern areas with a higher proportion of farmland. However, the process of how human activities and climate change affected lacustrine sedimentary ONAR is complex, with a number of processes concurrently operative, such as changes in rates of sediment and nutrient deposition, variations in water residence time, and rates of sediment resuspension. The effects of sedimentary ONAR burial on the N concentration in overlying water and the role of ONAR burial in the regional N cycle is still unclear. Therefore, more research is needed to understand the effect of the N composition of allochthonous and autochthonous organic matter sources in eutrophic lakes.
Acknowledgements
We thank Editage (www.editage.cn) for English language editing. We also sincerely thank the 2 anonymous reviewers and Associate Editor Dr. Grant Douglas for their constructive comments to improve the readability and scientific presentation of this manuscript.
Disclosure statement
No potential conflict of interest was reported by the author(s).
Additional information
Funding
References
- Anderson NJ, Bennion H, Lotter AF. 2014. Lake eutrophication and its implications for organic carbon sequestration in Europe. Global Change Biol. 20:2741–2751.
- Anderson NJ, Dietz RD, Engstrom DR. 2013. Land-use change, not climate, controls organic carbon burial in lakes. Proc R Soc B. 280(1769):20131278.
- Chen QY, Ni ZK, Wang SR, Guo Y, Liu SR. 2020. Climate change and human activities reduced the burial efficiency of nitrogen and phosphorus in sediment from Dianchi Lake, China. J Clean Prod. 274:122839.
- Chen YC, Tang L, Zhang DG, Li J, Zhou J, Guan XP. 2007. Spatial and temporal dynamic variation of nitrogen in sediment of Dianchi Lake. Soils. 39:879–883.
- Choi YJ, Lee SY. 2013. Microbial production of short-chain alkanes. Nature. 502:571–574.
- Conley DJ, Paerl HW, Howarth RW, Boesch DF, Seitzinger SP, Havens KE, Lancelot C, Likens GE. 2009. Controlling eutrophication: nitrogen and phosphorus. Science. 323:1014–1015.
- Dietz TR, Rose EA. 1994. Rethinking the environmental impacts of population, affluence and technology. Human Ecol Rev. 1(2):277–300.
- Downing JA, Cole JJ, Middelburg JJ, Striegl RG, Duarte CM, Kortelainen P, Prairie YT, Laube KA. 2008. Sediment organic carbon burial in agriculturally eutrophic impoundments over the last century. Global Biogeochem Cy. 22:1–10.
- Ehrlich PR, Holdren JP. 1971. Impact of population growth. Science. 171:1212–1217.
- Elser JJ, Bracken MES, Cleland EE, Gruner DS, Harpole WS, Hillebrand H, Ngai JT, Seabloom EW, Shurin JB, Smith JE. 2007. Global analysis of nitrogen and phosphorus limitation of primary producers in freshwater, marine and terrestrial ecosystems. Ecol Lett. 10:1135–1142.
- Fang J, Wu F, Xiong Y, Li F, Du X, An D, Wang L. 2014. Source characterization of sedimentary organic matter using molecular and stable carbon isotopic composition of n-alkanes and fatty acids in sediment core from Lake Dianchi, China. Sci Total Environ. 473–474:410–421.
- Ficken KJ, Li B, Swain DL, Eglinton G. 2000. An n-alkane proxy for the sedimentary input of submerged/floating freshwater aquatic macrophytes. Org Geochem. 31:745–749.
- Fortino K, Whalen SC, Smoak JM. 2016. Patterns in the percent sediment organic matter of arctic lakes. Hydrobiologia. 777:149–160.
- Fowler D, Pyle JA, Raven JA, Sutton MA. 2013. The global nitrogen cycle in the twenty-first century: introduction. Philos Trans R Soc B. 368:20130165.
- Galloway JN, Townsend AR, Erisman JW, Bekunda M, Cai Z, Freney JR, Martinelli LA, Seitzinger SP, Sutton MA. 2008. Transformation of the nitrogen cycle: recent trends, questions, and potential solutions. Science. 320:889–892.
- Galman V, Rydberg J, de-Luna SS, Bindler R, Renberg I. 2008. Carbon and nitrogen loss rates during aging of lake sediment: changes over 27 years studied in varved lake sediment. Limnol Oceanogr. 53:1076–1082.
- Gao W, Howarth RW, Swaney DP, Hong B, Guo HC. 2015. Enhanced N input to Lake Dianchi Basin from 1980 to 2010: drivers and consequences. Sci Total Environ. 505:376–384.
- Guzman G, Quinton JN, Nearing MA, Mabit L, Gomez JA. 2013. Sediment tracers in water erosion studies: current approaches and challenges. J Soil Sediment. 13:816–833.
- Han J, Calvin M. 1969. Hydrocarbon distribution of algae and bacteria, and microbiological activity in sediments. P Natl Acad Sci USA. 64:436–443.
- He J, Xu X, Yang Y, Wu X, Wang L, Li S, Zhou H. 2015. Problems and effects of comprehensive management of water environment in Lake Dianchi. J Lake Sci. 27:195–199.
- Heathcote AJ, Anderson NJ, Prairie YT, Engstrom DR, del Giorgio PA. 2015. Large increases in carbon burial in northern lakes during the Anthropocene. Nat Commun. 6:10016.
- Hockun K, Mollenhauer G, Ho SL, Hefter J, Ohlendorf C, Zolitschka B, Mayr C, Luecke A, Schefuss E. 2016. Using distributions and stable isotopes of n-alkanes to disentangle organic matter contributions to sediments of Laguna Potrok Aike, Argentina. Org Geochem. 102:110–119.
- Huang C, Zhang L, Li Y, Lin C, Huang T, Zhang M, Zhu AX, Yang H, Wang X. 2018. Carbon and nitrogen burial in a plateau lake during eutrophication and phytoplankton blooms. Sci Total Environ. 616–617:296–304.
- Huang CC, Wang XL, Yang H, Li YM, Wang YH, Chen X, Xu LJ. 2014. Satellite data regarding the eutrophication response to human activities in the plateau lake Dianchi in China from 1974 to 2009. Sci Total Environ. 485–486:1–11.
- Huang CC, Yang H, Li Y, Zhang ML, Lv H, Zhu AX, Yu Y, Luo Y, Huang T. 2017a. Quantificational effect of reforestation to soil erosion in subtropical monsoon regions with acid red soil by sediment fingerprinting. Environ Earth Sci. 76:34–49.
- Huang CC, Yao L, Zhang Y, Huang T, Zhang ML, Zhu AX, Yang H. 2017b. Spatial and temporal variation in autochthonous and allochthonous contributors to increased organic carbon and nitrogen burial in a plateau lake. Sci Total Environ. 603:390–400.
- Jeng WL. 2006. Higher plant n-alkane average chain length as an indicator of petrogenic hydrocarbon contamination in marine sediments. Mar Chem. 102:242–251.
- Jiang Q, Li S, Chen ZL, Huang CC, Wu W, Wan H, Hu Z, Han C, Zhang Z, Yang H, Huang T. 2020. Disturbance mechanisms of lacustrine organic carbon burial: case study of Cuopu Lake, Southwest China. Sci Total Environ. 746:140615.
- Ju XT, Xing GX, Chen XP, Zhang SL, Zhang LJ, Liu XJ, Cui ZL, Yin B, Christie P, Zhu ZL, Zhang FS. 2009. Reducing environmental risk by improving N management in intensive Chinese agricultural systems. P Natl Acad Sci USA. 106:3041–3046.
- Larsen S, Andersen T, Hessen DO. 2011. Climate change predicted to cause severe increase of organic carbon in lakes. Global Change Biol. 17:1186–1192.
- Leithold EL, Blair NE, Wegmann KW. 2016. Source-to-sink sedimentary systems and global carbon burial: A river runs through it. Earth-Sci Rev. 153:30–42.
- Liu H, Liu W. 2016. n-alkane distributions and concentrations in algae, submerged plants and terrestrial plants from the Qinghai-Tibetan plateau. Org Geochem. 99:10–22.
- Mead R, Xu YP, Chong J, Jaffe R. 2005. Sediment and soil organic matter source assessment as revealed by the molecular distribution and carbon isotopic composition of n-alkanes. Org Geochem. 36:363–370.
- Paerl HW, Xu H, McCarthy MJ, Zhu G, Qin B, Li Y, Gardner WS. 2011. Controlling harmful cyanobacterial blooms in a hyper-eutrophic lake (Lake Taihu, China): the need for a dual nutrient (N & P) management strategy. Water Res. 45:1973–1983.
- Quinton JN, Govers G, Van Oost K, Bardgett RD. 2010. The impact of agricultural soil erosion on biogeochemical cycling. Nat Geosci. 3:311–314.
- Rickson RJ. 2014. Can control of soil erosion mitigate water pollution by sediments? Sci Total Environ. 468:1187–1197.
- Sanchez-Cabeza JA, Ruiz-Fernandez AC. 2012. 210Pb sediment radio chronology: an integrated formulation and classification of dating models. Geochim Cosmochim Acta. 82:183–200.
- Sawada K, Ono M, Nakamura H, Tareq SM. 2020. Reurban land of Holocene Optimum paleoclimatic variations using long-chain n-alkanes and alkenones in sediments from Dabusu Lake, northeastern China. Quat Int. 550:27–38.
- Schmidt AH, Gonzalez VS, Bierman PR, Neilson TB, Rood DH. 2018. Agricultural land use doubled sediment loads in western China’s rivers. Anthropocene. 21:95–106.
- Smith JN. 2001. Why should we believe Pb-210 sediment geochronologies? J Environ Rad. 55:121–123.
- Wang BC, Yang J, Jiang HC, Zhang GJ, Dong HL. 2019. Chemical composition of n-alkanes and microbially mediated n-alkane degradation potential differ in the sediments of Qinghai-Tibetan lakes with different salinity. Chem Geol. 524:37–48.
- Wang JH, Wang YN, Dao GH, Du JS, Han YP, Hu HY. 2020. Decade-long meteorological and water quality dynamics of northern Lake Dianchi and recommendations on algal bloom mitigation via key influencing factors identification. Ecol Indic. 115:106425.
- Wang N, Zong YQ, Brodie CR, Zheng Z. 2014. An examination of the fidelity of n-alkanes as a palaeoclimate proxy from sediments of Palaeolake Tianyang, South China. Quat Int. 333:100–109.
- Wang P, Wu WS, Zhu BZ, Wei YM. 2013. Examining the impact factors of energy-related CO2 emissions using the STIRPAT model in Guangdong Province, China. Appl Energy. 106:65–71.
- Wong WW, Greening C, Shelley G, Lappan R, Leung PM, Kessler A, Winfrey B, Poh SC, Cook P. 2021. Effects of drift algae accumulation and nitrate loading on nitrogen cycling in a eutrophic coastal sediment. Sci Total Environ. 790:147749–147749.
- Wu TF, Qin BQ, Brookes JD, Yan WM, Ji XY, Feng J. 2019. Spatial distribution of sediment nitrogen and phosphorus in Lake Taihu from a hydrodynamics-induced transport perspective. Sci Total Environ. 650:1554–1565.
- York R, Rosa EA, Dietz T. 2003. STIRPAT, IPAT and ImPACT: analytic tools for unpacking the driving forces of environmental impacts. Ecol Econ. 46:351–365.
- Yu QB, Wang F, Yan WJ, Zhang FS, Lv SC, Li YQ. 2018. Carbon and nitrogen burial and response to climate change and anthropogenic disturbance in Chaohu Lake, China. Int J Env Res Pub Health. 15:2734–2752.
- Zhan S, Wu JL, Wang JZ, Jing M. 2020. Distribution characteristics, sources identification and risk assessment of n-alkanes and heavy metals in surface sediments, Tajikistan, Central Asia. Sci Total Environ. 709:136278.
- Zhou Q, Zhang Y, Lin D, Shan K, Luo Y, Zhao L, Tan Z, Song L. 2016. The relationships of meteorological factors and nutrient levels with phytoplankton biomass in a shallow eutrophic lake dominated by cyanobacteria, Lake Dianchi from 1991 to 2013. Environ Sci Pollut Res. 23(15):15616–15626.
- Zhou WJ, Zheng YH, Meyers PA, Jull AJT, Xie SC. 2010. Postglacial climate-change record in biomarker lipid compositions of the Hani peat sequence, northeastern China. Earth Planet Sci Lett. 294:37–46.
- Zhou Y, Liu Y. 2016. Does population have a larger impact on carbon dioxide emissions than income? Evidence from a cross-regional panel analysis in China. Appl Energ. 180:800–809.
- Zolitschka B, Francus P, Ojala AEK, Schimmelmann A. 2015. Varves in lake sediments – a review. Quat Sci Rev. 117:1–41.