ABSTRACT
Rice false smut disease is one of the most significant rice diseases worldwide. Ustilaginoidea virens is the causative agent of this disease. Although several developmental and pathogenic genes have been identified and functionally analyzed, the pathogenic molecular mechanisms of U. virens remain elusive. The velvet family regulatory proteins are involved in fungal development, conidiation, and pathogenicity. In this study, we demonstrated the function of the VelC homolog UvVELC in U. virens. We identified the velvet family protein UvVELC and characterized its functions using a target gene deletion-strategy. Deletion of UvVELC resulted in conidiation failure and pathogenicity. The UvVELC expression levels during infection suggested that this gene might be involved in the early infection process. UvVELC is also important in resistance to abiotic stresses, the utilization efficiency of glucose, stachyose, raffinose, and other sugars, and the expression of transport-related genes. Moreover, UvVELC could physically interact with UvVEA in yeast, and UvVELC/UvVEA double-knockout mutants also failed in conidiation and pathogenicity. These results indicate that UvVELC play a critical role in the conidiation and pathogenicity in U. virens. Functional analysis indicated that UvVELC-mediated conidiation and nutrient acquisition from rice regulates the pathogenicity of U. virens. Understanding the function of the UvVELC homolog could provide a potential molecular target for controlling rice false smut disease.
Background
Rice false smut (RFS), one of the most important panicle disease worldwide, is an urgent problem to ensure food security [Citation1]. From 2008 to 2016, in China, the average annual RSF occurrence was 3.06 million hectares, and yield loss reached 158.6 million kilograms per year. In India, RFS cuases approximately 0.2–49 % yield loss, depending on the rice variety and disease intensity [Citation1]. Ustilaginoidea virens is the causative agent of this disease. U. virens infection transforms panicle spikelets to so-called smut balls surrounded by darkly-pigmented chlamydospores, which is the most visible trait of RFS. U. virens not only causes yield losses, but also produces mycotoxins that have inhibitory effects on the plants’ seedling growth and animals’ neural systems [Citation2–5]. Notably, infection only occurs during the booting stage of rice. Visible yellowish spore balls protrude from the spikelet after heading is the typical symptom [Citation1]. Although several developmental and pathogenic genes have been identified and functionally analysed, the pathogenic molecular mechanisms of the infection process remain elusive [Citation1,Citation6,Citation7].
Velvet family members, as conserved fungal regulatory proteins, are involved in development, conidiation, secondary metabolite production, and pathogenicity [Citation8,Citation9]. All family members (VeA, VelB, VelC, and VosA) contain the velvet domain. Compared to the roles of VeA, VelB, and VosA, which are better understood, research regarding VelC is relatively scarce [Citation10,Citation11]. In Penicillium chrysogenum, the ΔvelC mutant exhibits increased conidiation and reduced penicillin production [Citation12]. VelC is also involved in P. expansum’s patulin biosynthesis pathway [Citation13]. In Magnaporthe oryzae, MoVELC plays important roles in the production and development of conidia. The appressoria cell wall integrity of MoVELC deletion mutants is affected, resulting in its inability to infect rice [Citation14]. However, the ΔvelC mutant did not show significant phenotypic differences in A. nidulans, A. fumigatus, A. flavus and Fusarium oxysporum [Citation15–18], suggesting that VelC might play an auxiliary role in asexual development and conidia production in these fungi [Citation17,Citation18]. These studies indicate that the function of the VelC protein is diverse in different fungi.
In U. virens, distinct from most fungi, we identified only three members of the velvet protein family, UvVEA, UvVELB, and UvVELC. Previous studies have shown that UvVEA and UvVELB play crucial roles in conidial and chlamydospore production [Citation19]. In this study, we uncovered functions of the VelC homolog, UvVELC, in U. virens using a target gene knockout strategy. Functional analysis indicated that UvVELC-mediated conidiation and nutrient acquisition from rice regulate the virulence of U. virens. Understanding the functionality of the UvVELC homolog could provide a potential molecular target for RFS control.
Materials and methods
Strains and growth conditions
The wild-type strain Jt209, isolated from infected rice spikelets collected in Jiangsu, China, have strong pathogenicity to the rice cultivar “LYP9.” U. virens strains were cultured on potato sucrose agar (PSA: 200 g/L potato, 20 g/L sucrose, 15 g/L agar) or in potato sucrose (PSA without agar) medium at 28 °C in the dark [Citation19]. Mycelial plugs (5 mm in diameter) were cut and collected from the edge of the 10-day-old colony and used for phenotypic analysis.
Sequence analysis
The Hidden Markov model profiles and local BLAST searches (GenBank JHTR00000000.1) were performed to identify genes from the U. virens protein database [Citation20,Citation21]. CD-search was used to verify the typical velvet domains of predicted proteins (https://www.ncbi.nlm.nih.gov/Structure/cdd/wrpsb.cgi). InterPro website was used to perform the protein sequence analysis. cNLSmapper was used to search for nuclear localization signal sequences [Citation8].
Targeted gene deletion and complementation in U. virens
To generate UvVELC deletion mutants in U. virens, the hygromycin resistance cassette and approximately 1 kb of the target genes’ 5ʹ UTR and 3ʹ UTR regions were amplified and ligated into the pMD19 T-simple vector (TaKaRa, Japan) [Citation19]. We then generated gene deletion mutants as described in previous studies using the CRISPR/Cas9 system [Citation19,Citation22]. The method used to generate the double deletion mutants of UvVELC and UvVEA was consistent with the above description, except that the hygromycin-resistant fragment was changed to a glufosinate-resistant fragment (bar gene from Streptomyces hygroscopicus for resistance to glufosinate-ammonium herbicide). The initial strain, ΔUvVEA, for transformation was obtained from the previous study [Citation19]. To generate the complementary vector, a fragment comprising a 1.5 kb upstream promoter region, together with the full-length UvVELC gene sequence, was amplified and linked to the pKO1 vector [Citation23]. Agrobacterium tumefaciens strain AGL-1, containing the pKO1-UvVELC construct, was transformed via Agrobacterium tumefaciens-mediated transformation (ATMT) with a hyphal fragment of ΔUvVELC mutants [Citation24]. Geneticin-resistant transformants were confirmed using PCR. PCR primers (Table S1) used in this study were synthesized by Sangon Biotech (China).
Vegetative growth and conidiation
For growth, mycelial plugs were cultured on fresh YTA medium (1 g/L yeast extract, 1 g/L tryptone, 1 g/L sucrose, and 15 g/L agar) at 28 °C, with or without rice tissue. Vegetative mycelia radial growth was measured after 12 days incubation in the dark. For conidiation tests, five mycelial plugs were incubated in 50 mL YT (YTA without agar) medium, with or without rice tissue. Media containing rice tissue was prepared as previously described [Citation25]. In brief, flag leaves and panicles of rice cultivars “LYP9” were collected at the late booting stage. Subsequently, 20 g rice tissue was crushed for 30 s using a mixer in 1 L of YT medium. The medium was then autoclaved at 121°C for 30 min, and filtered using three layers of sterile gauze. Filtrate with 15 g/L agar was used as the rice tissue medium for growth tests. Filtrate without agar was used as the rice tissue medium for conidiation tests. Production was measured using a hemocytometer after shaking at 160 rpm for 7 d. Filtered vegetative hyphae were collected for RNA extraction. Each treatment was repeated thrice.
Stress response and sugar utilization assays
For stress response assays, mycelial plugs were cultured on YTA plates with different stress agents, including 0.5 M KCl, 0.3 M NaCl, 0.7 M sorbitol, 0.005% (w/v) sodium dodecylsulphate (SDS), 70 μg/ml Congo red (CR), 500 μg/ml calcofluor white (CFW) or 3 mM H2O2, respectively. For sugar utilization, mycelial plugs were transferred to YTA with different sugar sources (10 g/L), including glucose, sucrose, maltose, raffinose, stachyose, trehalose, fructose, and stachyose. Plates were incubated in the dark with five replicates for each group. Each diameter of colony was measured after 12 days of cultivation [Citation19]. Each treatment was repeated thrice.
Plant infection assays
The virulence of U. virens strains was tested based on a previously described method [Citation19]. Mycelial plugs of Jt209, ΔUvVELC mutants, complementation strains, and ΔUvVELC/ΔUvVEA double-deletion mutants were placed into PS media for 7 days, respectively. Cultures without conidia were homogenized in a high-speed blender. The susceptible rice cultivar LYP9 was inoculated using a 2 mL mixture in the middle of panicles at the booting stage. Three weeks after inoculation, injected panicles were photographed, and the number of false smut balls per panicle were calculated. Twenty rice panicles were inoculated in each treatment. For UvVELC expression assays during infection, inoculated rice panicles were sampled at 0, 1, 2, 3, 5, 7, 9, and 11 days post-inoculation (dpi) for qRT-PCR. GFP-tagged U. virens strains were obtained to discover the expansion of infection hyphae. The binary vector pKD2-GFP was transformed into Jt209 and ΔUvVELC mutants using ATMT [Citation23]. GFP was randomly inserted into the U. virens genome. The infection’s hyphal expansion was observed inside the panicles and spikelets at an early stage of infection using a stereo-fluorescence microscope (Olympus IX71, Japan). Each treatment was repeated thrice.
Nucleic acid manipulations and qRT-qPCR
The CTAB protocol was used to extract genomic DNA from vegetative hyphae [Citation7]. Total RNA was extracted from the mycelia of infected rice spikelets using a Total RNA Extraction Kit (Biotech, China). The PrimeScript RT reagent Kit with gDNA Eraser (TaKaRa, Japan) was used for cDNA synthesis. The SYBR Premix Ex TaqTM II Kit (TaKaRa, Japan) was used for qRT-PCR assays, performed to detect the expression levels of UvVELC or genes involved in transmembrane transport, cell wall-degrading enzymes, conidiation, and polyketide synthase [Citation7,Citation19,Citation26]. The β-tubulin gene was used as the internal control [Citation27]. Relative mRNA amounts were calculated using the −2ΔΔCt method [Citation28]. PCR primers are listed in Supplementary Table S1.
Yeast two-hybrid assay
The full-length cDNA of UvVELC was amplified from the cDNA of wild-type strain Jt209, and cloned into the binding domain vector pGBKT7 and the activation domain vector pGADT7, respectively [Citation19]. The plasmids were then cotransformed into the Saccharomyces cerevisiae reporter strain Y2H gold, following the yeast two-hybrid kit (Clontech, San Francisco, CA, USA). A similar approach was used to generate bait and prey vectors for UvVEA and UvVELB. The transformants were isolated in SD/-Leu/-Trp medium and confirmed in SD/-Ade/-His/-Leu/-Trp medium. The experiments were independently repeated thrice to confirm the results.
Results
Identification of UvVELC ortholog in U. virens
The HMM profile and a BLAST_P search were performed to identify velvet family proteins in U. virens. Unlike most ascomycetes, only three velvet proteins were found: UV8b_01329 (identified as UvVEA), UV8b_04876 (identified as UvVELB) [Citation19], and UV8b_03692. As a phylogenetic analysis of protein sequences revealed that UV8b_03692 had a closer relationship with VelC homologs in other filamentous fungi, we named this homolog UvVELC in U. virens (Figure S1). The VosA homolog was not identified by searches using AnVosA of A. nidulans and MoVosA of M. oryzae as queries in the U. virens genome database.
To verify the transcript, we sequenced the cDNA fragment of Jt209. The results identified UvVELC as a predicted 235 amino acid protein (based on its cDNA) (GAO13277), 19 amino acids longer than previously predicted (UV8b_03692). The UvVELC sequence included only one exon without an intron, and carried a velvet factor-conserved domain between amino acids 15–200 and a NLS between amino acids 226–235 ().
Figure 1. The mutiple alignment of amino acid sequences of UvVELC, and VelC homologue in other fungi. Deduced VelC-like proteins of fusarium graminearum, magnaporthe oryzae, neurospora crassa, Aspergillus nidulans, Aspergillus flavus, and U. virens, were aligned by DNASTAR using ClustalW multiple sequence alignment. The velvet domain predicted by InterPro website for the UvVELC protein is depicted in light blue according to IPR037525. The predicted NLS is shown in purple.
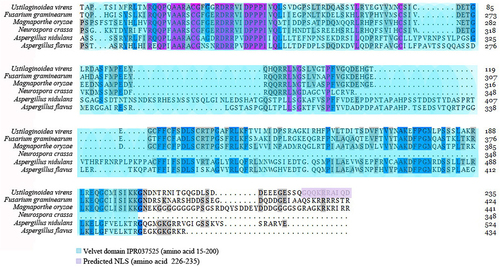
Deletion of UvVELC in U. virens
To investigate the functional role of UvVELC in U. virens, we deleted the UvVELC gene using homologous recombination, replacing the open reading frames with a hygromycin resistance cassette (HYG) (). ΔUvVELC null mutants were confirmed via PCR using primer sets flanking the gene and qRT-PCR. Among 25 hygromycin-resistant transformants, HYG replaced the ORF of UvVELC in three deletion mutants (ΔUvVELC −2, −3, and −7). The three mutants exhibited similar phenotypic characteristics. Complemented transformants CΔUvVELC (−1 and −2), in which a genomic copy of the UvVELC gene was introduced into the ΔUvVELC-7 mutants (), were obtained to verify the phenotypic defects of the knockout mutants.
Figure 2. Targeted knockout and complement assay of UvVELC in U. virens. (a) The UvVELC coding region was replaced with the hygromycin phosphotransferase gene cassette (HYG) by homologous recombination. (b) PCR amplify of the UvVELC in the genome of WT, ΔUvVELC, and CΔUvVELC strains. actin gene was used as the control gene. (c) qRT-PCR analysis of the expression of UvVELC in the Jt209, ΔUvVELC, and CΔUvVELC strains. β-tubulin gene was used as the endogenous reference gene. (d) Sanger sequencing traces of junction regions confirmed that UvVELC was replaced with HYG in mutants. Inserted sequences are indicated in red.
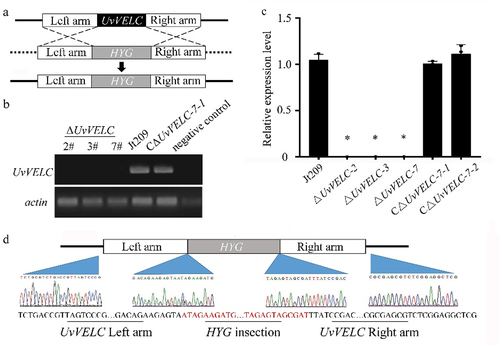
UvVELC is involved in conidiation in U. virens
Quantitative analyses of the number of conidia produced using YT medium demonstrated that Jt209 and CΔUvVELC produced conidia after shaking for 7 days, whereas ΔUvVELC mutants lost the ability to produce conidia (). As the rice tissue medium could promote the conidiation of U. virens conidiation-defective isolates [Citation25], we further induced mutants’ conidia using this medium. The number of conidia increased by 19.9- and 27.6-fold in Jt209 and CΔUvVELC, respectively, compared with those in YT medium. However, consistent with YT medium results, ΔUvVELC mutants still failed to produce conidia (). These results indicate that UvVELC acts as a regulator of conidiation in U. virens.
Figure 3. UvVELC involves in mycelial growth and conidiation in U. virens. (a) Statistical analysis of conidiation in YT medium. The wild-type strain Jt209, ΔUvVELC-3, ΔUvVELC-7, and CΔUvVELC strains were cultured in YT medium for 7 d. (b, c) expression pattern of central regulatory pathway genes in vegetative grown cultures in YT medium without (b) or with (c) rice tissue. Mean values and SDs were derived from three independent biological replicates with similar results. * represents significant differences between the mutants and the wild-type strain as subjected to Duncan’s test (p < 0.05).
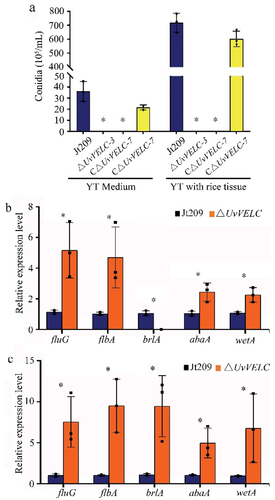
We further investigated the expression levels of central regulatory pathway genes (brlA, abaA and wetA) and pathway activators (fluG and flbA) in ΔUvVELC mutants. In accordance with conidiation, the mRNA level of the central regulator brlA was significantly reduced in the YT medium (). Unexpectedly, brlA’s expression level was significantly induced in the rice tissue medium (), suggesting that the UvVELC target genes might be important in the conidiation and downstream of brlA in the conidial production pathway.
UvVELC is involved in vegetative growth and responses to various stresses in U. virens
We also measured mycelial growth rates of the wild-type strain Jt209, ΔUvVELC mutants, and complementation strains CΔUvVELC on YTA medium. Deletion mutants showed significantly increased radial growth compared with Jt209 and CΔUvVELC ().
Figure 4. UvVELC plays roles in responses to various stresses in U. virens. (a) The Jt209, ΔUvVELC-3, ΔUvVELC-7, and CΔUvVELC-7 were cultured on YT plates with 0.5 M KCl, 0.3 M NaCl, 0.7 M sorbitol, 0.005% (w/v) SDS (sodium dodecyl sulfate), 70 μg/ml CR (Congo red), 500 μg/ml CFW (calcofluor white), or 3 mM H2O2. The photograph of the plates were captured 12 d post-incubation. (b) The diameters of colonies were measured under various stress conditions. The mean values and SDs of diameters from three independent biological experiments with similar results were statistically analyzed. * denotes significant differences between the mutants and the wild-type strain, as determined by Duncan’s test (p < 0.05).
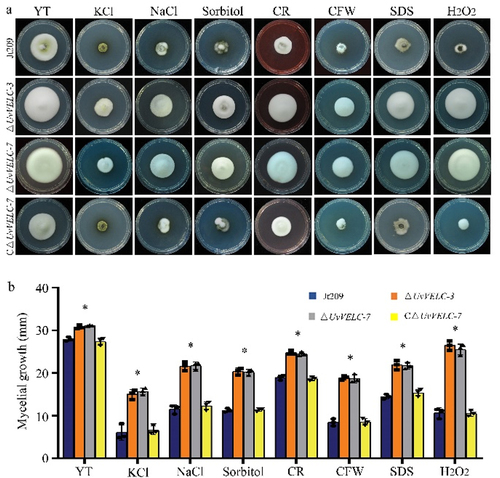
We then tested the effects of various stresses on the ΔUvVELC mutants on YTA medium supplemented with 0.5 M KCl, 0.3 M NaCl, or 0.7 M sorbitol. In the presence of hyperosmotic stress, the mycelial growth rates of all strains decreased, and ΔUvVELC mutants displayed less sensitivity than WT and CΔUvVELC strains (). These results indicate that UvVELC might play a negative role in response to salt stress in U. virens.
We also assayed the effects of cell membrane and oxidative stress on ΔUvVELC mutants. In the presence of 0.005% (w/v) SDS, 70 μg/ml CR, or 500 μg/ml CFW, which mimicked the cytoplasmic membrane and cell wall stresses, Jt209, and CΔUvVELC strains displayed significantly reduced growth compared to those of ΔUvVELC mutants (). Similar results were obtained when ΔUvVELC mutants were grown on YTA medium with 3 mM H2O2. Therefore, these results indicate that UvVELC is involved in the responses to membrane and cell wall stresses in U. virens, as well as oxidative stress.
UvVELC plays crucial roles in virulence in U.Virens
To further investigate UvVELC functions during infection, we evaluated its expression profiles during the spikelet infection stages (at 0, 1, 2, 3, 5, 7, 9, and 11 dpi) using qRT-PCR. The expression of UvVELC was significantly up-regulated during the early stages of inoculation, with the highest value on the fifth day, which was 3.5-fold higher than it was at the initial inoculation. Subsequently, the UvVELC expression level showed a downward trend. This result suggests that UvVELC might play a role in the early infection stages of U. virens ().
Figure 5. UvVELC plays crucial roles in pathogenicity of U.Virens. (a) qRT-qPCR evaluated the expression profiles of UvVELC during spikelet infection stage (at 0, 1, 2, 3, 5, 7, 9, and 11 dpi). (b) Disease symptoms caused by the wild type strain Jt209, ΔUvVELC-3, ΔUvVELC-7, and CΔUvVELC-7 strains three weeks after inoculation. (c) Statistical analysis conducted on the mean number of false smut balls per spikelet. Three replicates with at least 20 inoculated panicles in each trial. The mean values and SDs from three independent biological experiments with similar results statistically analyzed. * denotes significant differences between mutants and the wild-type strain, as determined by Duncan’s test (p < 0.05). (d) Mycelial extension of Jt209-GFP and ΔUvVELC-GFP inside the spikelets were observed at 2, 4, 7 dpi. bar, 100 μm.
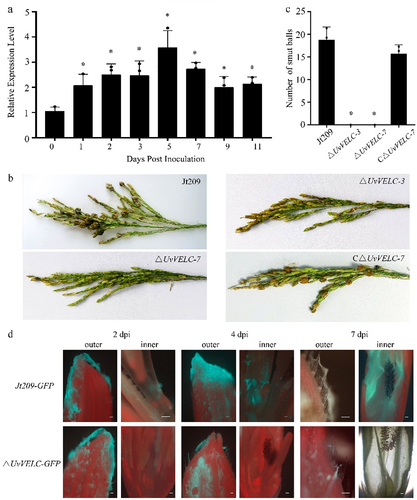
For rice inoculation tests, due to the conidiation failure observed in ΔUvVELC mutants, we utilized a mycelial suspension of these mutants for inoculation, as well as the Jt209 and CΔUvVELC strains. Three weeks post-inoculation, both Jt209 and CΔUvVELC strains caused the typical symptoms of false smut balls, whereas ΔUvVELC failed to develop false smut balls ().
To verify the pathogenicity defect of the mutants in vivo, Jt209 and ΔUvVELC strains tagged with GFP were inoculated into rice panicles. The inoculated rice was monitored for U. virens infection for 7 days (). In Jt209-GFP strains, hyphae extended into the interior of spikelets and reached the surface of filaments by 2 dpi. At 4 dpi, hyphae extended along the filaments and onto the stamen anthers. At 7 dpi, massive filaments enveloped the internal organs, including anthers, filaments, and lodicules. For ΔUvVELC-GFP strains, hyphae were elongated and enveloped the surface of spikelets during 1–5 dpi, similar to that of the Jt209-GFP strains. However, at the rice-breaking stage (6–7 dpi), mycelia lost fluorescence and failed to grow further. Furthermore, no hyphae extended into spikelets until 7 dpi. This confirms that UvVELC plays an important role in the colonization and extension of U. virens.
UvVELC involves in carbon sources utilization, and transmembrane transport
As a nonobligate biotrophic fungus, U. virens acquires carbohydrates as a carbon source from the apoplasts of rice flower cells. We hypothesized that the impaired acquisition of nutrients was responsible for the ΔUvVELC defect of extension into the interior of spikelets. Therefore, we first detected expression levels of pectinase (UvPe1 and UvPe2), cellulose (UvCel), and cutinase (UvCut1, UvCut2, and UvCut3) genes [Citation26]. In ∆UvVELC mutants, the expression of UvCel was significantly upregulated, whereas UvCut1 and UvCut2 were significantly downregulated compared to Jt209 (). We further analyzed the expression levels of these genes in ∆UvVELC during the rice infection process, and found that UvPe1, UvPe2, Uvcut1, and Uvcut3 were significantly downregulated (). Consistently, as shown in , the growth rates of the mutants significantly decreased compared to the wild-type in the rice medium. These results suggest that UvVELC participates in regulating the ability of U. virens to decompose some host cell wall components, such as pectinase, cellulose, and cutinase.
Figure 6. UvVELC was involved in carbon sources utilization, and transmembrane transport. (a) qRT-qPCR evaluated the expression of pectinase genes (UvPe1, UvPe2), cellulase genes (UvCel), and cutinase genes (UvCut1, UvCut2, and UvCut3) in the wild-type strain Jt209 and ΔUvVELC mutants after 5 d of during the rice infection process. (b) qRT-qPCR evaluated the expression of genes in the wild-type strain Jt209 and ΔUvVELC mutants after 5 d of inoculation on rice. (c) The diameter of colonies was measured after 12 d of incubation on YTA plates with or without rice tissues. (d) The diameter of colonies was measured after 12 d of incubation on plates containing sucrose, raffinose, soluble starch, trehalose, maltose, glucose, stachyose, and fructose, respectively. (e) qRT-PCR evaluated the expression level of genes related to transmembrane transport. Uv8b_5286, putative sugar transporter; Uv8b_827, oligopeptide transporter; Uv8b_7122, MFS sugar transporter; Uv8b_601, putative sugar transport protein; Uv8b_6977, sugar transporter family protein; Uv8b_654, putative iron transferase; Uv8b_1682, putative methionine permease; Uv8b_6013, MFS multidrug transporter; Uv8b_6685, MFS multidrug transporter; Uv8b_6649, MFS transporter; Uv8b_121, choline transport protein; Uv8b_144, carboxylic acid transport protein. The mean values and SDs from three independent biological experiments with similar results were statistically analyzed. * denotes significant differences between the mutant and the wild-type strain, as determined by Duncan’s test (p < 0.05).
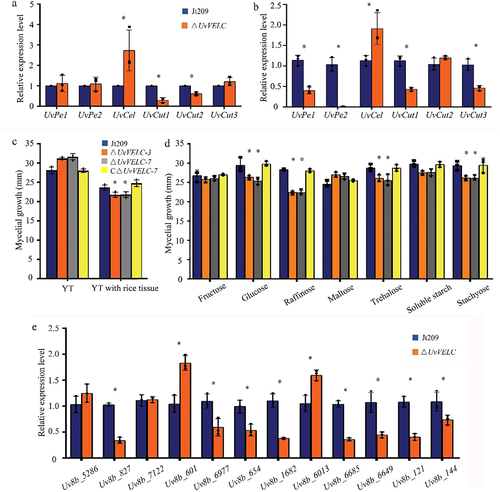
Then, the carbohydrate utilization preferences of ΔUvVELC with Jt209 and CΔUvVELC strains were compared, the growth rates of the ΔUvVELC had significantly decreased on media containing raffinose, trehalose, glucose, or stachyose, suggesting ΔUvVELC defects in carbon source utilization ().
To further study the role of UvVELC in carbon source utilization and transmembrane transport, the expression levels of related genes during the rice infection process were assessed (). qRT-PCR results demonstrated that the expression levels of some specific genes were significantly decreased in ΔUvVELC, including Uv8b_827 (oligopeptide transporter), Uv8b_6977 (Sugar transporter family protein), Uv8b_654 (putative iron transferase), Uv8b_1682 (putative methionine permease), Uv8b_6685 (MFS multidrug transporter), and Uv8b_6649 (MFS transporter), Uv8b_121 (choline transport protein), Uv8b_144 (carboxylic acid transport protein). Genes related to sugar transporters were significantly upregulated in the mutants, including Uv8b_601 (putative sugar transport protein) and Uv8b_6013 (MFS multidrug transporter) [Citation7]. The results indicated that UvVELC plays a critical role in carbon source utilization and transmembrane transport in U. virens. ΔUvVELC mutants might have defects regarding transporting rice nutrients for carbohydrate utilization, which prevent mutants from extending and forming smut balls.
Interaction of UvVELC with UvVEA
In several fungal species, such as A. nidulans and Botrytis cinerea, velvet proteins form specific complexes that regulate various processes [Citation17]. Therefore, Yeast Two-hybrid (Y2H) assays were performed to test whether these three velvet protein interactions also occur in U. virens. Results showed that UvVEA could physically interact with UvVELC (). Further, we generated UvVELC and UvVEA double deletion mutants (Figure S2) and compared their phenotypes, including conidiation and pathogenicity, with Jt209, ΔUvVELC, and ΔUvVEA single mutants. ΔUvVELC/ΔUvVEA double mutants behaved almost identically to ΔUvVELC mutants, and completely lost conidiation (Figure S3c) and pathogenicity (Figure S3a, b), which was completely different from that of ΔUvVEA [Citation19]. Similarly, conidiation failed in double mutants, whereas it increased in ΔUvVEA single mutants compared to Jt209 (Figure S3c). These results suggest that the interaction between UvVELC and UvVEA is not required for conidiation or pathogenicity in U. virens.
Discussion
In the present study, we identified the velvet family protein, UvVELC, in U.virens. The deletion of UvVELC resulted in failure of conidiation and pathogenicity. UvVELC is also important in the utilization efficiency of glucose, stachyose, raffinose, and other sugars, as well as expression levels of pectinase, cellulase, cutinase, and transport-related genes. We further showed that UvVELC physically interacts with UvVEA in yeast. Our findings provide insights into the mechanism of UvVELC; that is, in U. virens, the pathogenic defects of ΔUvVELC mutants are mediated by gene regulation in conidiation and nutrient acquisition.
Conidial formation is regulated by three key transcription factors, brlA, abaA, and wetA [Citation29]. Velvet family proteins also control the regulatory circuits of downstream transcriptional networks for conidiation, elaborating on conidia formation and maturation mechanism. In A. nidulans, VosA inhibits the premature induction of brlA [Citation30]. VosA/VelB functions as global repressors, mediating the expression of McrA. In ΔmcrA mutants, conidiation was reduced as well as the mRNA levels of brlA [Citation29]. In this study, ΔUvVELC mutants also showed a defect in conidiation and consistently reduced mRNA levels of brlA. However, brlA can be induced by rice, and has no effect on the defect in conidiation in the mutants. Qin et al. [Citation31] found that brlA expression was required, but not sufficient, for conidiation in Penicillium decumbens. When P. decumbens was cultured in either nutrient-rich or nutrient-poor liquid media, no conidiation was observed in either medium. However, the expression of brlA was low in nutrient-rich medium, but was high in nutrient-low medium. It is therefore proposed that in ΔUvVELC mutants, although the loss of conidiation caused by the UvVELC gene deletion did not change, the brlA gene’s upregulated expression might have been induced by changes in the culture environment. This suggests that UvVELC participates in conidiation by regulating the expression of its target genes downstream of brlA in the conidial production pathway. Therefore, identifying target genes and revealing the UvVELC regulatory mechanisms in conidiation requires further study.
UvVELC was expressed in the early stage of the interaction and showed an upward trend during the first five days. These results suggested that UvVELC played a role in fungal pathogenesis. During U. virens infection, hyphae diffuse on the outer surfaces of the spikelets and expand into their inner spaces [Citation1]. Massive and rapid conidiation might promote pathogen dispersal, subsequently leading to an increase in the occurrence of rice smut disease [Citation32,Citation33]. In ΔUvVELC mutants, failure to produce conidia might affect the pathogen dispersal by extending into the spikelets, thereby reducing the disease’s ability to progress. Moreover, as a nonobligate biotrophic fungus, U. virens acquires carbohydrates as a carbon source from apoplasts of rice flower cells for vegetative growth and pathogenicity [Citation1]. In our study, we found that the deletion of UvVELC led to a decrease in the nutrition utilization efficiency from rice tissue. This phenomenon might first be due to the significantly downregulated expression of pectinase, cellulase, and cutinase in ΔUvVELC mutants during infection. Cutinase plays an important role in degrading the surface layer of the stamen filaments during the early stage of infection by U. virens [Citation26]. On the other hand, we assessed expression levels of genes related to carbon source utilization and transmembrane transport and found that the expression of several genes was significantly decreased in ΔUvVELC mutants. Correspondingly, the utilization of sugars, such as glucose and stachyose, decreased in ΔUvVELC. In the ΔUvCCHC5 mutants of U.virens, it also impaired transmembrane transport, resulting in nutrient deficiencies and preventing mutants from using nutrients from rice to form smut balls [Citation7]. Collectively, changes in the usage and absorption of nutrients in ΔUvVELC, which prevented it from obtaining sufficient nutrients from rice during the infection process, might also explain the loss of pathogenicity in U. virens.
In U. virens genome, we identified only three velvet proteins in the U. virens genome: UvVEA, UvVELB, and UvVELC. The lack of VosA orthologs appeared similar to those of F. graminearum, F. verticillioides, and F. solani, whereas ascomycetes such as Neurospora crassa, A. nidulans, and M. oryzae all predicted VosA proteins [Citation18]. In N. crassa, Ve-2 can form heterodimers with VosA, whhereas VE-3, the VelC homologous protein, appears to have a truncated velvet domain and is not part of the velvet complex [Citation34]. VosA is a key regulator for conidiation viability, while VelC does not appear to play a significant role in conidiation A. flavus [Citation35,Citation36]. It is unclear whether the specific functions of VosA orthologs have been replaced by those of other velvet complex proteins, such as UvVELC in U. virens. In this study, we found that, unlike VelB and VosA, which interact in other fungi, UvVELB and UvVELC do not interact in U. virens. However, UvVELC was involved in conidiation and pathogenicity, similar to function of VosA in A. flavus. Therefore, we speculated that UvVELC might partially replace the function of VosA in U. virens.
Previous studies have shown that velvet family proteins are related to the secondary metabolisms of various fungal species, including P. chrysogenum, F. oxysporum, and B. cinerea [Citation18,Citation37,Citation38]. Knockout of the VeA homolog in A. nidulans, A. flavus, and A. parasiticus results in the failure of aflatoxin or aflatoxin precursor production [Citation10]. The ΔFvVE1 mutants of F. verticilloides failed to produce the deleterious mycotoxins fumonisin and fusarins [Citation39]. The velB mutants were deficient in pigment and sterigmatocystin production in A. nidulans. In F. graminearum, ΔFgVELB mutants showed a yellow pigment rather than a pink pigment, with a dramatic impairment in DON production [Citation40,Citation41]. Deletion of PeVelC significantly reduces patulin production in P. expansum [Citation13]. In U. virens, our data showed that deletion of UvVELC led to defects in pigmentation compared to Jt209 (Figure S4a). Ustilaginoidins, one of the main mycotoxins produced by U. virens, are toxic to plants, animals, and humans [Citation42]. Moreover, in comparison with Jt209, expressions of gene clusters containing polyketide synthase genes were downregulated or upregulated in ΔUvVELC (Figure S4b). It has been speculated that UvVELC is also involved in regulating the secondary metabolism of U. virens. However, when treated with culture filtrates of Jt209, ΔUvVELC, or CΔUvVELC strains, there were no significant differences in the shoots and roots of the rice, suggesting that the loss of pathogenicity might not depend on the effect of the ΔUvVELC mutant on reducing toxicity to rice (Figure S4c).
Velvet family proteins regulate gene expression through interactions among family members. In Aspergillus spp., VelB physically interacts with VeA and the putative methyltransferase LaeA to regulate secondary metabolites [Citation43]. In F. oxysporum, VeA-VelB complexes have positive roles in conidia size, and VeA-VelC plays the opposite role in determining conidia size [Citation18]. VelC interacts with other velvet regulators. In P. chrysogenum, VelC can form a polymer with VelA and VosA [Citation44]. VosA interacts with VelC in A. nidulans, and is the epistasis of VelC in most biological processes [Citation17]. In U. virens, UvVELC interacted with UvVEA in yeast. The phenotype of the single mutants indicated that UvVELC acts as an activator, and UvVEA as a repressor of conidiation, playing opposite roles during asexual development and pathogenicity [Citation19]. The opposing regulatory role of proteins has been described in the previous study (e.g., PcVelB and PcVelC in the velvet complex in P. chrysogenum conidiation); however, the regulatory mechanism is unclear [Citation12]. In A. nidulans, the well-conserved tetrameric HapB-HapC-HapE-HapX complex AnCF, and the basic-region helix-loop-helix transcription factor AnBH1, showed alternative counteracting roles in binding to the CCAAT motif in the promoter of penicillin biosynthesis genes to regulate the expression of penicillin [Citation45,Citation46]. In this study, functional characterization of single- and double-knockout mutants led us to conclude that UvVELC, as a member of the complex, plays an alternative regulatory role in binding to the promoter of conidiation genes in U. virens. Future research regarding velvet complexes will provide further evidence of their predicted function, and thus promote our understanding of their mechanistic role.
Conclusions
In summary, we report the involvement of UvVELC, from the velvet family, in conidiation and pathogenicity of U. virens. These results will enrich research on the pathogenic mechanisms of U. virens. As velvet family proteins have been found only in ascomycetes, UvVELC represents a promising potential molecular target for developing novel control strategies for U. virens. Therefore, we are using the UvVELC-flag fusion protein to identify the direct targets of UvVELC via ChIP-seq, to elucidate the molecular mechanisms underlying the regulatory functions mediated by UvVELC.
Authors’ contributions
YFL and WDL designed the study. MNY, TQS, JJY, HJC, XYP, ZQQ, and YD performed methodology, validation, investigation, and data curation. MNY was a major contributor to writing the manuscript. YFL performed the reviewed and edited the manuscript. All authors have read and approved the final manuscript.
Availability of data and material
The datasets supporting the conclusions of this study are included within the article and its additional files.
List of abbreviations
CFW, calcofluor white; CR, Congo red; dpi, days post-inoculation; HYG, hygromycin resistance cassette; NLS: Nuclear localization signals; PS, potato sucrose media; qRT-PCR, real-time quantitative PCR; RFS, rice false smut; SDS, sodium dodecyl sulphate
Table S1. The primers used in this study.docx
Download MS Word (40.8 KB)Supplementary Figure.docx
Download MS Word (901.9 KB)Disclosure statement
No potential conflict of interest was reported by the author(s).
Supplementary material
Supplemental data for this article can be accessed online at https://doi.org/10.1080/21505594.2023.2301243
Additional information
Funding
References
- Sun W, Fan J, Fang A, et al. Ustilaginoidea virens: insights into an emerging rice pathogen. Annu Rev Phytopathol. 2020;58(1):363–13. doi: 10.1146/annurev-phyto-010820-012908
- Lai D, Meng J, Xu D, et al. Determination of the absolute configurations of the stereogenic centers of ustilaginoidins by studying the biosynthetic monomers from a gene knockout mutant of villosiclava virens. Sci Rep. 2019;9(1):1855. doi: 10.1038/s41598-018-37941-5
- Wang G, He D, Zhao F, et al. Extraction and purification of ustiloxin a from rice false smut balls by a combination of macroporous resin and high-speed countercurrent chromatography. Food Prod Process And Nutr. 2020;2:29. doi: 10.1186/s43014-020-00043-9
- Kumar A, Pathak H, Bhadauria S, et al. Aflatoxin contamination in food crops: causes, detection, and management: a review. Food Prod Process And Nutr. 2021;3:17. doi: 10.1186/s43014-021-00064-y
- Zhang X, Xu D, Hou X, et al. UvSorA and UvSorB involved in sorbicillinoid biosynthesis contribute to fungal development, stress response and phytotoxicity in Ustilaginoidea virens. Int J Mol Sci. 2022;23(19):11056.
- Song T, Zhang Y, Zhang Q, et al. The N-terminus of an ustilaginoidea virens ser-thr-rich glycosylphosphatidylinositol-anchored protein elicits plant immunity as a MAMP. Nat Commun. 2021;12(1):2451. doi: 10.1038/s41467-021-22660-9
- Chen X, Pei Z, Peng L, et al. Genome-wide identification and functional characterization of CCHC-type zinc finger genes in Ustilaginoidea virens. J Fungi (Basel). 2021;7(11):947.
- Höfer AM, Harting R, Aßmann NF, et al. The velvet protein Vel1 controls initial plant root colonization and conidia formation for xylem distribution in verticillium wilt. PloS Genet. 2021;17(3):e1009434.
- Liu H, Yao X, Ke W, et al. A velvet transcription factor specifically activates mating through a novel mating-responsive protein in the human fungal pathogen Cryptococcus deneoformans. Microbiol Spectr. 2022;10(3):e0265321.
- Wang G, Ran H, Fan J, et al. Fungal-fungal cocultivation leads to widespread secondary metabolite alteration requiring the partial loss-of-function VeA1 protein. Sci Adv. 2022;8(17):eabo6094.
- Lan N, Yue Q, An Z, et al. Apc.LaeA and Apc.VeA of the velvet complex govern secondary metabolism and morphological development in the echinocandin-producing fungus aspergillus pachycristatus. J Ind Microbiol Biotechnol. 2020;47(1):155–168.
- Kopke K, Hoff B, Bloemendal S, et al. Members of the penicillium chrysogenum velvet complex play functionally opposing roles in the regulation of penicillin biosynthesis and conidiation. Eukaryot Cell. 2013;12(2):299–310. doi: 10.1128/EC.00272-12
- Li B, Chen Y, Zong Y, et al. Dissection of patulin biosynthesis, spatial control and regulation mechanism in penicillium expansum. Environ Microbiol. 2019;21(3):1124–1139.
- Kim HJ, Han JH, Kim KS, et al. Comparative functional analysis of the velvet gene family reveals unique roles in fungal development and pathogenicity in magnaporthe oryzae. Fungal Genet Biol. 2014;66:33–43. doi: 10.1016/j.fgb.2014.02.011
- Sarikaya Bayram O, Bayram O, Valerius O, et al. LaeA control of velvet family regulatory proteins for light-dependent development and fungal cell-type specificity. PloS Genet. 2010;6(12):e1001226. doi: 10.1371/journal.pgen.1001226
- Park HS, Bayram O, Braus GH, et al. Characterization of the velvet regulators in aspergillus fumigatus. Mol Microbiol. 2012;86(4):937–953. doi: 10.1111/mmi.12032
- Park HS, Nam TY, Han KH, et al. VelC positively controls sexual development in Aspergillus nidulans. PloS One. 2014;9(2):e89883. doi: 10.1371/journal.pone.0089883
- López-Berges MS, Hera C, Sulyok M, et al. The velvet complex governs mycotoxin production and virulence of Fusarium oxysporum on plant and mammalian hosts. Mol Microbiol. 2013;87(1):49–65.
- Yu M, Yu J, Cao H, et al. The velvet protein UvVEA regulates conidiation and chlamydospore formation in Ustilaginoidea virens. J Fungi (Basel). 2022;8(5):479.
- Zhang Y, Zhang K, Fang A, et al. Specific adaptation of Ustilaginoidea virens in occupying host florets revealed by comparative and functional genomics. Nat Commun. 2014;5:3849. doi: 10.1038/ncomms4849
- Yu M, Yu J, Cao H, et al. Genome-wide identification and analysis of the GATA transcription factor gene family in Ustilaginoidea virens. Genome. 2019;62(12):807–816. doi: 10.1139/gen-2018-0190
- Liang Y, Han Y, Wang C, et al. Targeted deletion of the USTA and UvSLT2 genes efficiently in Ustilaginoidea virens with the CRISPR-Cas9 system. Front Plant Sci. 2018;9:699. doi: 10.3389/fpls.2018.00699
- Cao H, Zhang J, Yong M, et al. The cyclase-associated protein UvCap1 is required for mycelial growth and pathogenicity in the rice false smut fungus. Phytopathol Res. 2021;3(5). doi: 10.1186/s42483-021-00083-0
- Yu M, Yu J, Hu J, et al. Identification of pathogenicity-related genes in the rice pathogen ustilaginoidea virens through random insertional mutagenesis. Fungal Genet Biol. 2015;76:10–9. doi: 10.1016/j.fgb.2015.01.004
- Wang Y, Wang F, Xie S, et al. Development of rice conidiation media for Ustilaginoidea virens. PloS One. 2019;14(10):e0217667. doi: 10.1371/journal.pone.0217667
- Tang JT. Studies on transcriptome of infection process, long non-coding RNA identification, and species-specific molecular detection of Ustilaginoidea virens. Ph D. Dissertation, Wuhan, China: Huazhong Agricultural University; 2019
- Fan J, Guo XY, Li L, et al. Infection of ustilaginoidea virens intercepts rice seed formation but activates grain-filling-related genes. J Integr Plant Biol. 2015;57(6):577–590.
- Livak KJ, Schmittgen TD. Analysis of relative gene expression data using realtime quantitative PCR and the 2−ΔΔCt method. Methods. 2001;25:402–408. doi: 10.1006/meth.2001.1262
- Lee MK, Son YE, Park HS, et al. Velvet activated McrA plays a key role in cellular and metabolic development in Aspergillus nidulans. Sci Rep. 2020;10(1):15075. doi: 10.1038/s41598-020-72224-y
- Thieme KG, Gerke J, Sasse C, et al. Velvet domain protein VosA represses the zinc cluster transcription factor SclB regulatory network for Aspergillus nidulans asexual development, oxidative stress response and secondary metabolism. PloS Genet. 2018;14(7):e1007511.
- Qin Y, Bao L, Gao M, et al. Penicillium decumbens BrlA extensively regulates secondary metabolism and functionally associates with the expression of cellulase genes. Appl Microbiol Biotechnol. 2013;97(24):10453–10467. doi: 10.1007/s00253-013-5273-3
- Fan J, Guo XY, Huang F, et al. Epiphytic colonization of ustilaginoidea virens on biotic and abiotic surfaces implies the widespread presence of primary inoculum for rice false smut disease. Plant Pathol. 2013;63:937–945. doi: 10.1111/ppa.12167
- Meng S, Xiong M, Jagernath JS, et al. UvAtg8-mediated autophagy regulates fungal growth, stress responses, conidiation, and pathogenesis in Ustilaginoidea virens. Rice (N Y). 2020;13(1):56. doi: 10.1186/s12284-020-00418-z
- Bayram ÖS, Dettmann A, Karahoda B, et al. Control of development, secondary metabolism and light-dependent carotenoid biosynthesis by the velvet complex of neurospora crassa. Genetics. 2019;212(3):691–710. doi: 10.1534/genetics.119.302277
- Eom TJ, Moon H, Yu JH, et al. Characterization of the velvet regulators in aspergillus flavus. J Microbiol. 2018;56(12):893–901. doi: 10.1007/s12275-018-8417-4
- Cho HJ, Son SH, Chen W, et al. Regulation of conidiogenesis in Aspergillus flavus. Cells. 2022;11(18):2796. doi: 10.3390/cells11182796
- Kamerewerd J, Zadra I, Kürnsteiner H, et al. PcchiB1, encoding a class V chitinase, is affected by PcVelA and PcLaeA, and is responsible for cell wall integrity in penicillium chrysogenum. Microbiology (Reading). 2011;157(Pt 11):3036–3048. doi: 10.1099/mic.0.051896-0
- Yang Q, Chen Y, Ma Z. Involvement of BcVeA and BcVelB in regulating conidiation, pigmentation and vi rulence in botrytis cinerea. Fungal Genet Biol. 2013;50:63–71. doi: 10.1016/j.fgb.2012.10.003
- Li S, Myung K, Guse D, et al. FvVE1 regulates filamentous growth, the ratio of microconidia to macroconidia and cell wall formation in Fusarium verticillioides. Mol Microbiol. 2006;62(5):1418–32.
- Jiang J, Yun Y, Liu Y, et al. FgVELB is associated with vegetative differentiation, secondary metabolism and virulence in fusarium graminearum. Fungal Genet Biol. 2012;49(8):653–62.
- Lee J, Myong K, Kim JE, et al. FgVelB globally regulates sexual reproduction, mycotoxin production and pathogenicity in the cereal pathogen Fusarium graminearum. Microbiology (Reading). 2012;158(Pt 7):1723–1733. doi: 10.1099/mic.0.059188-0
- Li Y, Wang M, Liu Z, et al. Towards understanding the biosynthetic pathway for ustilaginoidin mycotoxins in Ustilaginoidea virens. Environ Microbiol. 2019;21(8):2629–2643.
- Bayram O, Krappmann S, Ni M, et al. VelB/VeA/LaeA complex coordinates light signal with fungal development and secondary metabolism. Science. 2008;320(5882):1504–6.
- Becker K, Ziemons S, Lentz K, et al. Genome-wide chromatin immunoprecipitation sequencing analysis of the penicillium chrysogenum velvet protein PcVelA identifies methyltransferase PcLlmA as a novel downstream regulator of fungal development. mSphere. 2016;1(4):e00149–16. doi: 10.1128/mSphere.00149-16
- Caruso ML, Litzka O, Martic G, et al. Novel basic-region helix-loop-helix transcription factor (AnBH1) of aspergillus nidulans counteracts the CCAAT-binding complex AnCF in the promoter of a penicillin biosynthesis gene. J Mol Biol. 2002;323(3):425–439. doi: 10.1016/s0022-2836(02)00965-8
- Hortschansky P, Eisendle M, Al-Abdallah Q, et al. Interaction of HapX with the CCAAT-binding complex–a novel mechanism of gene regulation by iron. EMBO J. 2007;26(13):3157–3168. doi: 10.1038/sj.emboj.7601752