ABSTRACT
Chimeric antigen receptors-based cell therapies have shown impressive preclinical and clinical success and revolutionized biomedicine. However, the link between science and invention, the impact of international cooperation, and the influence and prestige of CARs research have not been explored. This study analyzed the landscape of peer-reviewed articles and patents related to CARs. A total of 5,681 publications were analyzed using bibliometrics and machine learning-based text mining to assess publication metrics, subject areas, and research hotspots. 5,010 Inpadoc families were also analyzed for patent filing trends, priority countries, and applicant and inventor rankings. The results show that CARs research has the following distinctive features: high research prestige among research community; strong global geographical bias in both academic output and patenting patterns; strong links between science and invention, but significant differences among countries; and an inverse relationship between country size and international collaboration rates.
Introduction
Chimeric antigen receptors (CARs) are engineered receptors that graft a defined specificity and function onto an immune effector cell,Citation1 typically a T cell, and augment T-cell function to recognize tumor-associated antigens.Citation2,Citation3 The advent of genetic engineering technologies in the late 20th century provided the possibilities of engineering T lymphocytes.Citation4,Citation5 CAR-based cell therapies, including CAR-T, CAR-NK, and CAR-Treg, were looked upon as a versatile therapeutic engine to target virtually any tumor-associated cell-surface antigen.Citation6–9 It has shown great promise in treating acute lymphoid leukemia, B-Cell lymphomas, autoimmune diseases, cardiac fibrosis, or chronic hepatitis B or C virus infection, and has since revolutionized biomedicine.Citation10–16
Both academic and industrial research has made significant progress in the last decade.Citation17 2017 saw the first marketing of CAR-T cell therapies, with tisagenlecleucel (Kymriah, Novartis) and axicabtagene ciloleucel (Yescarta, Gilead) approved by the United States Food and Drug Administration (FDA).Citation18,Citation19 Several bibliometrics studies have been presented in previous works specifically on CAR-T cell therapy,Citation20,Citation21 while there is still a lack of comprehensive evaluation of academic literature and patent landscape of CARs. Previous studies have certain shortcomings, such as the wide use of bibliometric software (Citespace or VOSviewer), which relies heavily on direct citations without normalization (e.g., frequency of citations or the importance of citing journals) and simple word frequencies when examining research hotspots.Citation22,Citation23 A recent study proves that field normalization was a prerequisite for citation analysis.Citation24 Patent analysis of citations also mostly focuses on analyzing citation relationships, but using pooled citations may suffer from bias or overinflated significance levels, as citations made by patent examiners have not been separately reported.Citation25 What is more, a quantified discussion of international collaboration and the link between science and invention,Citation26 which were essential factors in modern scientific fields, were absent in the prior reviews.Citation27,Citation28
In this paper, we applied various bibliometrics indicators to explore the science and invention landscape of CARs and emphasize research excellence, prestige, international collaboration, and the link between science and invention.
Materials and methods
Peer-viewed papers and patents related to CAR T cell therapy were obtained from SciVal and Derwent Innovation (DI) database. The search formula used was as follows: TITLE-ABS-KEY (“Chimeric antigen receptor” OR “CAR-T” AND (cell OR therapy) OR “CAR T-cell” OR “CAR T cell” OR “CAR therapy”). SciVal uses a stemming algorithm that reduces words to their root form, so the singular, plural, and possessive forms of most keywords could be ignored, while it is not the case in patent searching. Cleanup was performed to remove irrelevant data. The search was limited to publication date up to 3 January 2021. Using this search formula, we retrieved 7,886 publications in the Scopus database, including 3,491 articles, 2,996 reviews, 424 notes, 302 editorial, 202 letters, 201 book Chapters, 116 surveys, 80 conference papers, 65 erratum, and six books. In SciVal, the search results in a total of 6,246 publications (1996–2020). After removing some unrelated technical fields, including agriculture, computer science, physics and astronomy, and environmental science, 5,681 publications remain, which are the bibliometric analysis subjects for this study. 5,010 Inpadoc families (17,498 records) were retrieved from DI database (the following analysis uses patent families). The current status of academic performance, including the distribution of countries, institutions, and scholars, is analyzed. The technical trends and research hotspots are revealed by text clustering of both peer-viewed papers and patents.
SciVal is a web-based analytics solution launched by Elsevier,Citation29 allows researchers to process over 50 million publication records from more than 22,000 journals of 5,000+ publishers, 14,000 research institutions and their associated researchers from 230 nations worldwide through more than 280 trillion metric values. Its potential as a robust tool to inform evidence-based research strategy and planning and drive performance has not been fully explored.
An overview of current academic research status, hotspots, and global technology trends of CAR-T cell therapies in the past ten years are presented using SciVal. Furthermore, the patent landscape is laid out following the WIPO guidelines for preparing patent landscape reports.
We employed many metrics (extracted from SciVal): Scholarly output, Field-Weighted Citation Impact (FWCI), Publications in Top Journal Percentiles, Outputs in Top Citation Percentiles, Patent-citations per scholarly output, Publications in Top Journal Percentiles. Each metric is explained below.
Scholarly output indicates how many publications does this entity have indexed in Scopus. It should be used with field-normalized metrics such as publications in top journal percentiles or FWCI in comparing different entities.
A Topic is a dynamic collection of documents with a common focused intellectual interest. Topics are ranked by prominence percentile, and active topics give insights into the research planning process, help researchers stay abreast of the latest research trends. Topics were calculated by using direct citation analysis. Currently there are around 96,000 Topics and 1,500 Topic Clusters in SciVal, that have been formed through a method of direct citation linking. A publication can only belong to one Topic and one Topic Cluster. Each week new publications are allocated to the existing Topics and Topic Clusters.
Citations vary spatially and are affected by differences in research fields and need to be normalized. As an article-level field-standardized metric, FWCI benchmarks the prestige of entities regardless of differences in their size, disciplinary profile, age, and publication-type composition.Citation30 It indicates how the number of citations received by an entity’s publications compares with the average number of citations received in the data universe.
Two metrics, Publications in Top Journal Percentiles and Outputs in Top Citation Percentiles, are often used to illustrate research excellence.Citation31 Publications in Top Journal Percentiles indicate how an entity’s publications are present in the most-cited journals. Outputs in Top Citation Percentiles indicate the extent to which an entity’s publications are present in the most-cited publications. These metrics show how much of an institution’s publication output was good enough to rank among the world’s top publications.
The economic impact indicators can quantify the relationship between science and invention. Patent-citations per scholarly output is the patent citation counts divided by an entity’s total scholarly output for that period and multiplied by 1,000. It indicates how much, on average, research is being used to create products and provide information about the economic impact of research.
Publications in Top Journal Percentiles are used to benchmark the most influential, highly cited research fields. It is useful to benchmark the contributions of publications and distinguish their performances.
Other indicators used in this paper are self-explanatory: International Collaboration (%) (abbreviated as IC%); Academic-Corporate Collaboration (%) (abbreviated as ACC%); Publications in Top 10% Journal Percentiles by CiteScore Percentile (%) (abbreviated as JP10%); Output in Top 10% Citation Percentiles (%) (abbreviated as CP10%).
Results and discussion
CARs research focus and hotspots
Research hotspots are identified with text mining with Natural Language Processing techniques to mine publication titles, abstracts, and author keywords within the research area. As shown in , the main diseases treated with CARs include acute lymphoblastic leukemia, multiple myeloma, large cell lymphoma, acute myeloid leukemia, hematologic neoplasm, B lymphocyte, glioblastoma, and non-Hodgkin lymphoma. The main research focuses include natural killer cell, CD19 antigen, checkpoint, T-cell antigen receptor, bispecific antibody, tumor microenvironment, and CRISPR. The fastest-growing hotspots for research from 2015–2019 include B cell maturation antigen (1,350%), mesothelin (2,800%), neurotoxicity syndrome (1,933%), neurotoxicity (5,800%), and regulatory T lymphocyte (2,800%).
Figure 1. Top 50 key phrases by relevance, based on 4,907 publications.
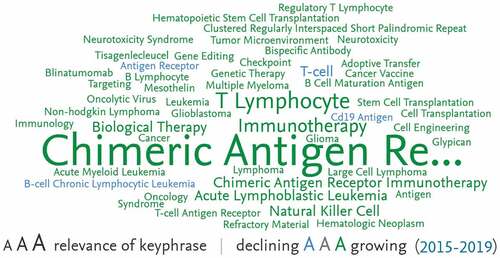
We also performed a cluster analysis of the patent texts in title, abstracts, and claims. Patents focus more on providing a complete and viable approach to constructing CAR-T engineered cells, including heavy and light chain, the variable domain, and the binding domain. The most intensively filed patent technologies were in the following directions: engineering of immune cells, infusion, expression, encoding and binding of cells, and breast, lung, and prostate cancer applications. We also found that the construction of peptide or MHC, culture medium or device, preparation of CAR-T, and moiety switch or conjugate are important technical spots. The application of Induced pluripotent stem cells (iPSCs) is also one of the latest research directions. These results show the technical focus of patents and their difference from basic research. Patent technology pays more attention to the integrity and practicability of technical solutions.
Screening of leading topics
Topic prominence indicates research excellence in funding (a correlation with the amount of funding per author), performance, and talent. It is calculated combining three metrics which indicate the momentum of the Topic: Citation Count in year n to papers published in n and n-1, Scopus View Count in year n to papers published in n and n-1, and average Journal CiteScore for year n. Prominence is calculated for each topic j in year n as:
Pj = 0.495 (Cj-mean(Cj))/stdev(Cj)+0.391 (Vj-mean(Vj))/stdev(Vj)+0.114 (CSj-mean(CSj))/stdev(CSj),
where cj is citation counts to articles in cluster j published in years n and n-1, vj is the Scopus views counts to articles in cluster j published in years n and n-1, and csj is the average CiteScore for articles in cluster j published in year n. These raw values are log-transformed into the values used in the formula as Cj = ln(cj + 1), Vj = ln(vj + 1), and CSj = ln(csj + 1). Prominence, as defined here, is a linear combination of citations, views and journal impact for a given topic, where each of these factors is normalized by the topic standard deviation.Citation32 The Prominence calculation model showed that the correlation between Prominence and future funding is 0.616, thus Prominence accounts for 38% (or 0.6162) of the variance of future funding.
Topics with the most momentum 2015–2020 are listed in by Prominence percentile. PD-1 (checkpoint) silencing augmented the potency CAR T cells’ anti-tumor activities, with a prominence percentile of 99.998%, is the cutting edge of CARs research.Citation33–35 The employment of CRISPR-Cas9 in CARs is another state-of-the-art technology.Citation36,Citation37 CARs against B-cell maturation antigen (BCMA) represent a promising therapy that has demonstrated antimyeloma activity in clinical and pre-clinical trials and improves patients’ overall survival.Citation38,Citation39 These results allow for the optimization of future research and funding strategies and the identification of commercialization targets.
Table 1. Top 20 topics by Prominence percentile 2015–2020
Status of global car academic performance
The overall performance of CARs research from 2010–2020 is shown in . The research performance is based on publications, citations, and collaboration. Researches on CARs began at the end of the last century, but until 2000, only a few pioneers were engaged in CARs research. 2000–2011 can be regarded as the nascent period of CARs research, characterized by prolonged growth in the number of authors, publications, and patents. CARs research formally entered the growth period after 2012, with the number of publications exceeding 100, and the number of authors and patents both exploded in parallel (). The number of global authors increased to 9,393, and scholarly output grew to 1,655 in 2020, while the number of patent families reached 1,026 in 2018, which shows that researchers worldwide have developed a keen interest in CARs after 2012. CARs have become one of the mainstream hotspots for academic research and industrial R&D.
Table 2. Research area overview and trends of global CARs academic performance
Figure 2. Patent landscape of CARs.
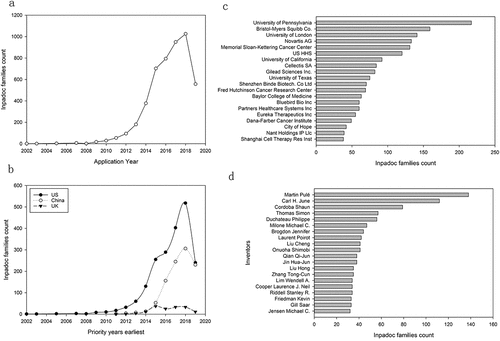
Citations indicate the impact or quality of publications. After 2012, CARs gained qualitative change in citations, growing to more than 10,000 and reaching 22,371 in 2018. The average FWCI for the CAR field from 2010–2019 was 3.04, which is 204% higher than the world average of 1.0. Comparing CARs with CRISPR can better elucidate the significance of this metric. According to our study, CRISPR gene-editing technology has an FWCI of 2.33 in the same period, but its scholarly output reached 15,657. The main difference between the two is that CRISPR, as an underlying general-purpose technology, has facilitated the development of many disciplines, while CARs are only used for disease treatment.
Patent citations per scholarly output reached 11,774 and 12,229 in 2010–2011, which indicates that essential discoveries were found in these years and thus received the most citations. This metric is also relatively high for other years, showing both a high economic impact and a strong link between academic and industrial research in CARs.
Publications in the top 1%, 5%, 10%, and 25% of the most-cited journals in CAR research are 6, 16.7, 26.2, and 46.4, respectively. Thus, the proportion of highly cited papers indicates that CARs research level is leading in biomedical sciences.
In summary, all metrics indicate that CARs research has become an undisputed research hotspot, a field with high research prestige, attracts talent, and interacts well with the industrial community, with the strong potential for even more groundbreaking discoveries in future research.
Global CAR research technology fields
The technical field of CARs academic output from 2015 to 2020 is shown in . Medicine accounted for 44.8% of the total papers, mainly in oncology, hematology, immunology and allergy. Biochemistry, Genetics and Biochemistry, Genetics and Molecular Biology accounted for 29.0% of the total papers, of which more than half belonged to cancer research. Immunology and pharmacology were also the focus of research. Medicine studies account for almost half of the total number of papers, suggesting that CARs research has a more pronounced character from basic laboratory studies to clinical translation.
Geographical distribution of car research output
The geographical distribution of CARs research 2015–2020 is shown in . The US has 2,473 scholarly outputs in the first place, and China is in second place with 958 scholarly outputs. Germany is in third place with 465 scholarly outputs, and the UK, France, and Italy with just over 200 scholarly outputs, followed by relatively weaker countries in terms of scholarly outputs. According to clinicaltrials.gov, the US continues to have the largest overall sample size of subjects and number of centers in clinical trials.Citation40 In contrast, China has the most CAR T clinical trials registered at over 350, compared to just over 150 for all clinical trials outside China.Citation41
Table 3. Global geographical distribution of CARs 2015–2020
Combining the FWCI, JP10%, and CP10% to judge the quality of research, Canada and Australia have high research quality but small research output. The US has high research quality, and its lower International Collaboration may be because US institutions have more quantity and quality to meet their own research needs. China’s research quality is lower, with an FWCI of 1.4, and both JP10% and CP10% are the lowest in . Except for Japan, all other countries present high international collaboration, showing excellent research ability and academic performance.
Regarding academic research’s institutional cooperation, the international collaboration rate was 20.4%, with an FWCI of 3.66. In comparison, the domestic collaboration rate was 38.2%. It has an FWCI of only 1.59, which indicates that international collaboration can promote papers’ citation and improve research results and researchers’ international prestige significantly. Moreover, among the papers published during this period, the UK, Switzerland, and Austria have the highest academic-corporate collaboration rate.
shows the geographical distribution of patent families according to the earliest priority years. Most patents were filed after 2012, and the US has been leading the way with 1,973 patents and is committed to filing homologation patents in countries worldwide. China with 1,156 patents mainly files patents domestically, with barely a few dozen patents applied globally. The UK filed 157 patents, and subsequent countries filed even fewer, with European countries (151 patents), WO (84 patents), Japan (53 patents), the Republic of Korea (43 patents), Denmark (35 patents), Australia (18 patents), Singapore (10 patents).
In summary, we recommend that countries outside the US strengthen collaboration and communication with the US to promote CARs research in their countries.
Global distribution of research institutions
lists research institutions according to the scholarly output during 2015–2020. 15 of the top 20 institutions are from the US, with the only Institut national de la santé et de la recherche médicale (ISERM) from France, German Cancer Research Center from Germany, University College London from the UK, and Zhengzhou University and General Hospital of People’s Liberation Army from China. The US institutions have excellent performance in many indicators, among which the University of Pennsylvania ranks first in the scholarly output of 350, FWCI of 6.77. Its academic-corporate collaboration rate is 16%, which is the highest among US institutions. French institutions lag in all indicators, while two Chinese institutions have no Academic-Corporate Collaboration, but University College London has a high Academic-Corporate Collaboration rate of 28.2%. Among institutions with high FWCI after the top 20, Novartis (including Novartis USA) has excellent academic performance and prestige as a company and maintains close collaboration with academic institutions. Some institutions outside the US, such as the University of Würzburg, Goethe University Frankfurt, Karolinska Institutet of Sweden, University of Toronto, Tel Aviv University, and the University of Oslo, all have a robust academic prestige and research influence, although with a smaller research output volume.
Table 4. Institutional distribution in the field of CARs 2015–2020
As shown in , the University of Pennsylvania, the University of London, Memorial Sloan-Kettering Cancer Center, the University of California, the University of Texas, and the Fred Hutchinson Cancer Research Center are both leaders in academic research and patent filings. Two Chinese companies were among the top 20 patent filers, Shenzhen Binde Biotech with 70 patents and Shanghai Cell Therapy Res Inst with 38, with the rest being U.S. research institutions and companies. In terms of corporate patent filing ranking, Bristol-Myers Squibb Co., Novartis AG, Cellectis SA, Gilead Sciences Inc., Shenzhen Binde Biotech, Bluebird Bio Inc, Eureka Therapeutics Inc, and Shanghai Cell Therapy Res Inst are leading patent owners.
Global distribution of scholars
lists the top 20 scholars by scholarly output for the period 2015–2020. Most scholars are from the US, and all have high FWCI. The highest being Bruce L. Levine reaching 20.76, and most other scholars are above 10. The lowest being Han Weidong, who is the only scholar from outside the US but also reaches 3.29. Both JP10% and CP10% are high for them, generally getting over 50%, many even going above 70%. It is, undoubtedly, a glory of CARs research and rarely seen in other research areas.
Table 5. Top scholars in the field of CARs 2015–2020
US scholars generally have some degree of corporate collaboration to transform their academic results into clinical research and industrial applications, which is worth learning for other countries and regions. Of the top 20 scholars with the highest scholarly output, seven are from the University of Pennsylvania, two from Memorial Sloan-Kettering Cancer Center, and two from Fred Hutchinson Cancer Research Center. As a pioneer in immunotherapy, Carl H. June is in the first place. He is most widely known for the development of T-cell therapies, tested the capacity of genetically modified CAR-T cells in humans, and led to the development and commercialization of Kymriah, the first FDA-approved treatment in the US. University of Pennsylvania and Novartis have established themselves as leaders in CAR research under his leadership. Given that CAR-T cell therapy has gained academic attention as a frontier discipline in immunotherapy for only about a decade, these scholars will be strong contenders for the Nobel Prize if enhanced CAR-T cell therapy makes breakthroughs in solid tumors in the future.Citation42–44
Analyzing the inventors of CAR-T patents (), we find that Carl H. June has successfully translated his scientific results into patented technologies, with a patent family of 112. Here, the Academic-Corporate Collaboration analyzed above was transformed into inventions. The collaboration analysis proves relevant for analyzing the association between science and invention. Other academics with outstanding scientific research have not been as successful in translating their results. For example, Martin Pulé of University College London published only 16 papers in 2015–2020. The number of patent applications of other highly productive scholars is far less than their papers.
These findings indicate that the diffusion of CARs technology to research communities outside the US needs to be further accelerated to promote this revolutionary technology further.
Highly cited publications
The field of CARs research is rich in citations, with 12 articles with more than 1,000 citations, 21 articles with 500–999 citations, 322 articles with 100–499 citations, 2,321 articles between 10–99 citations, 1,006 articles with 5–9 citations, and 1,963 articles with 1–4 citations. Encouragingly, not all articles with the highest citation counts were distributed in earlier years; there is also a lot of highly cited literature in recent years. Citation activity shows a thriving CARs research community.
The New England Journal of Medicine is the most popular journal for highly cited authors to publish in. Before 2015, CAR-T cells were mainly for chronic or acute lymphoid leukemia,Citation45 antitumor memory in advanced leukemia,Citation46 adverse event,Citation47 and sustained remissions in leukemia.Citation48 After 2015, B-cell lymphoma caught researchers’ attention.Citation49,Citation50 Remained a research hotspot since their emergence in the 2010s, CD19 CAR therapies have shown efficacy in treating acute or chronic lymphocytic leukemia, B-cell lymphomas and relapsed or refractory diffuse large B-cell lymphoma,Citation18 and the promising results led to the landmark approvals of two CD19-specific CAR T-cell products.Citation51–53
Conclusion
In this paper, we conducted a bibliometric analysis of academic research and patent landscape of CARs, focusing on the link between science and invention, the impact of international cooperation, and the influence and prestige of academic research. Through the technology life-cycle analysis, we found that researches on CARs began at the end of the last century. However, researchers worldwide did not develop a keen interest in CARs until after 2012. Since then, all bibliometric indexes indicate that CARs research has become an undisputed research hotspot, a field with high research prestige, attracts talent, and interacts well with the industrial community. The US has been leading the way in academic research and patented technologies. China trails in second place, while Germany, the UK, France, and Italy follow behind. Almost all European countries present high international collaboration, especially with the US. However, research performance outside of the US and Europe shows a considerable gap.
The CARs research community has a high academic-corporate collaboration rate, which greatly contributed to global scientific progress. This link is close among scholars in the US, closer in the UK, Austria, Belgium, and Norway, but sparser in France, Italy, and China. It is worth noting that US scholars generally have a high degree of corporate collaboration to transform their academic results into clinical research and industrial applications. The best example of this is Professor Carl H. June, who has successfully translated his scientific works into patented technologies.
An analysis of international collaboration in CARs reveals that international collaboration is inversely proportional to country size/research volume. European countries such as Sweden, Switzerland, and Belgium have the highest international collaboration, followed by Germany, the UK, and Australia. The US and China have the lowest. From the results, the impact of international cooperation on FWCI is generally positive, and the higher the proportion of international cooperation, the higher the research prestige indicated by FWCI.
Currently, CARs have been developed mainly by scholars and companies in the US and China. Over the past decade, both academia and industry have made significant advances in CARs. Researchers enjoy high research prestige, which indicates high research quality and academic impact. This revolutionary technology has significantly advanced the cure for various diseases. However, the diffusion of CARs technology to research communities outside the US needs to be further accelerated to promote this revolutionary technology further. Another issue to note is the high prices of current CAR T-cell therapies pose a financial burden on patients and the healthcare systems and thus undermine its impact. In addition, some technical issues remain, especially for solid tumors, where progress is slow, and breakthroughs are yet to be made. New CARs cell therapies, such as CAR-NK therapy, are still in their infancy. Generic CAR-T platforms with multi target or combination strategies of CARs with other therapies such as checkpoint silencing are among the most promising directions for the next generation of cancer therapy. The future of biomedical science will revolutionize as more advanced technologies mentioned above become available.
Disclosure of potential conflicts of interest
No potential conflicts of interest were disclosed.
Additional information
Funding
References
- June CH, Sadelain M. Chimeric antigen receptor therapy. N Engl J Med. 2018;379:64–73. doi:10.1056/NEJMra1706169.
- Sadelain M, Rivière I, Riddell S. Therapeutic T cell engineering. Nature. 2017;545:423–31. doi:10.1038/nature22395.
- Hong M, Clubb JD, Chen YY. Engineering CAR-T cells for next-generation cancer therapy. Cancer Cell. 2020;38:473–88. doi:10.1016/j.ccell.2020.07.005.
- Ren J, Liu X, Fang C, Jiang S, June CH, Zhao Y. Multiplex genome editing to generate universal CAR T cells resistant to PD1 inhibition. Clin Cancer Res. 2017;23:2255–66. doi:10.1158/1078-0432.CCR-16-1300.
- Uherek C, Tonn T, Uherek B, Becker S, Schnierle B, Klingemann H-G, Wels W. Retargeting of natural killer-cell cytolytic activity to ErbB2-expressing cancer cells results in efficient and selective tumor cell destruction. Blood. 2002;100:1265–73. doi:10.1182/blood.V100.4.1265.h81602001265_1265_1273.
- Boardman DA, Philippeos C, Fruhwirth GO, Ibrahim MAA, Hannen RF, Cooper D, Marelli-Berg FM, Watt FM, Lechler RI, Maher J, et al. Expression of a chimeric antigen receptor specific for donor HLA class I enhances the potency of human regulatory T cells in preventing human skin transplant rejection. Am J Transplant. 2017;17:931–43. doi:10.1111/ajt.14185.
- Demaria O, Cornen S, Daëron M, Morel Y, Medzhitov R, Vivier E. Harnessing innate immunity in cancer therapy. Nature. 2019;574:45–56.
- Basar R, Daher M, Rezvani K. Next-generation cell therapies: the emerging role of CAR-NK cells. Hematol (United States). 2020;20:570–78.
- Daher M, Rezvani K. Outlook for new car-based therapies with a focus on car nk cells: what lies beyond car-engineered t cells in the race against cancer. Cancer Discov. 2021;11:45–58. doi:10.1158/2159-8290.CD-20-0556.
- Torikai H, Reik A, Liu P-Q, Zhou Y, Zhang L, Maiti S, Huls H, Miller JC, Kebriaei P, Rabinovitch B, et al. A foundation for universal T-cell based immunotherapy: t cells engineered to express a CD19-specific chimeric-antigen-receptor and eliminate expression of endogenous TCR. Blood. 2012;119:5697–705. doi:10.1182/blood-2012-01-405365.
- Barrett DM, Singh N, Porter DL, Grupp SA, June CH. Chimeric antigen receptor therapy for cancer. Annu Rev Med. 2014;65:333-47. doi:10.1146/annurev-med-060512-150254.
- Ellebrecht CT, Bhoj VG, Nace A, Choi EJ, Mao X, Cho MJ, Di Zenzo G, Lanzavecchia A, Seykora JT, Cotsarelis G, et al. Reengineering chimeric antigen receptor T cells for targeted therapy of autoimmune disease. Science. 2016;353:179–84. doi:10.1126/science.aaf6756.
- Aghajanian H, Kimura T, Rurik JG, Hancock AS, Leibowitz MS, Li L, Scholler J, Monslow J, Lo A, Han W, et al. Targeting cardiac fibrosis with engineered T cells. Nature. 2019;573:430–33. doi:10.1038/s41586-019-1546-z.
- Mao Y, Zhao C, Zheng P, Zhang X, Xu J. Current status and future development of anti-HIV chimeric antigen receptor T-cell therapy. Immunotherapy. 2021;13:177–84. doi:10.2217/imt-2020-0199.
- Wang Y, Liu Y, Tan X, Pan B, Ge J, Qi K, Cheng H, Cao J, Shi M, Yan Z, et al. Safety and efficacy of chimeric antigen receptor (CAR)-T-cell therapy in persons with advanced B-cell cancers and hepatitis B virus-infection. Leukemia. 2020;34:2704–07. doi:10.1038/s41375-020-0936-4.
- Strati P, Nastoupil LJ, Fayad LE, Samaniego F, Adkins S, Neelapu SS. Safety of CAR T-cell therapy in patients with B-cell lymphoma and chronic hepatitis B or C virus infection. Blood. 2019;133:2800–02. doi:10.1182/blood.2019000888.
- Dotti G, Savoldo B, Brenner M. Fifteen years of gene therapy based on chimeric antigen receptors: are we nearly there yet? Hum Gene Ther. 2009;20:1229–39. doi:10.1089/hum.2009.142.
- Maude SL, Laetsch TW, Buechner J, Rives S, Boyer M, Bittencourt H, Bader P, Verneris MR, Stefanski HE, Myers GD, et al. Tisagenlecleucel in children and young adults with B-cell lymphoblastic leukemia. N Engl J Med. 2018;378:439–48. doi:10.1056/NEJMoa1709866.
- Lyu L, Feng Y, Chen X, Hu Y. The global chimeric antigen receptor T (CAR-T) cell therapy patent landscape. Nat Biotechnol. 2020;38:1387–94.
- Picanco-Castro V, Gonçalves Pereira C, Swiech K, Ribeiro Malmegrim KC, Tadeu Covas D, Silveira Porto G. Emerging CAR T cell therapies: clinical landscape and patent technological routes. Hum Vaccines Immunother. 2020;16:1424–33. doi:10.1080/21645515.2019.1689744.
- Liu M, Gao Y, Yuan Y, Shi S, Yang K, Wu J, Zhang J, Tian J. Global hotspots and future prospects of chimeric antigen receptor T-cell therapy in cancer research: a bibliometric analysis. Futur Oncol. 2020;16:597–612. doi:10.2217/fon-2019-0810.
- Callaham M, Wears RL, Weber E. Journal prestige, publication bias, and other characteristics associated with citation of published studies in peer-reviewed journals. J Am Med Assoc. 2002;287:2847–50. doi:10.1001/jama.287.21.2847.
- Boyack KW, Klavans R. Co-citation analysis, bibliographic coupling, and direct citation: which citation approach represents the research front most accurately? J Am Soc Inf Sci Technol. 2010;61:2389–404. doi:10.1002/asi.21419.
- Bornmann L, Haunschild R, Mutz R. Should citations be field-normalized in evaluative bibliometrics? An empirical analysis based on propensity score matching. J Informetr. 2020;14:101098. doi:10.1016/j.joi.2020.101098.
- Alcácer J, Gittelman M. Patent citations as a measure of knowledge flows: the influence of examiner citations. Rev Econ Stat. 2006;88:774–79. doi:10.1162/rest.88.4.774.
- Ahmadpoor M, Jones BF. The dual frontier: patented inventions and prior scientific advance. Science. 2017;357:583–87. doi:10.1126/science.aam9527.
- Coccia M, Wang L. Evolution and convergence of the patterns of international scientific collaboration. Proc Natl Acad Sci U S A. 2016;113:2057–61. doi:10.1073/pnas.1510820113.
- Gittelman M, Kogut B. Does good science lead to valuable knowledge? Biotechnology firms and the evolutionary logic of citation patterns. Manage Sci. 2003;49:366–82. doi:10.1287/mnsc.49.4.366.14420.
- Lancho-Barrantes BS, Cantu-Ortiz FJ. Quantifying the publication preferences of leading research universities. Scientometrics. 2021;126:2269–310. doi:10.1007/s11192-020-03790-1.
- Purkayastha A, Palmaro E, Falk-Krzesinski HJHJ, Baas J. Comparison of two article-level, field-independent citation metrics: Field-Weighted Citation Impact (FWCI) and Relative Citation Ratio (RCR). J Informetr. 2019;13:635–42. doi:10.1016/j.joi.2019.03.012.
- Rodríguez-Navarro A, Brito R. Like-for-like bibliometric substitutes for peer review: advantages and limits of indicators calculated from the ep index. Res Eval. 2020;29:215–30. doi:10.1093/reseval/rvaa002.
- Klavans R, Boyack KW. Research portfolio analysis and topic prominence. J Informetr. 2017;11:1158–74. doi:10.1016/j.joi.2017.10.002.
- Cherkassky L, Morello A, Villena-Vargas J, Feng Y, Dimitrov DS, Jones DR, Sadelain M, Adusumilli PS. Human CAR T cells with cell-intrinsic PD-1 checkpoint blockade resist tumor-mediated inhibition. J Clin Invest. 2016;126:3130–44. doi:10.1172/JCI83092.
- Rafiq S, Yeku OO, Jackson HJ, Purdon TJ, van Leeuwen DG, Drakes DJ, Song M, Miele MM, Li Z, Wang P, et al. Targeted delivery of a PD-1-blocking scFV by CAR-T cells enhances anti-tumor efficacy in vivo. Nat Biotechnol. 2018;36:847–58. doi:10.1038/nbt.4195.
- Yuan X, Sun Z, Yuan Q, Hou W, Liang Q, Wang Y, Mo W, Wang H, Yu M. Dual-function chimeric antigen receptor T cells targeting c-Met and PD-1 exhibit potent anti-tumor efficacy in solid tumors. Invest New Drugs. 2021;39:34–51. doi:10.1007/s10637-020-00978-3.
- Jung I-Y, Kim -Y-Y, Yu H-S, Lee M, Kim S, Lee J. CRISPR/Cas9-mediated knockout of DGK improves antitumor activities of human T cells. Cancer Res. 2018;78:4692–703. doi:10.1158/0008-5472.CAN-18-0030.
- Georgiadis C, Preece R, Nickolay L, Etuk A, Petrova A, Ladon D, Danyi A, Humphryes-Kirilov N, Ajetunmobi A, Kim D, et al. Long terminal repeat CRISPR-CAR-Coupled “Universal” T cells mediate potent anti-leukemic effects. Mol Ther. 2018;26:1215–27. doi:10.1016/j.ymthe.2018.02.025.
- Raje N, Berdeja J, Lin Y, Siegel D, Jagannath S, Madduri D, Liedtke M, Rosenblatt J, Maus MV, Turka A, et al. Anti-BCMA CAR T-cell therapy bb2121 in relapsed or refractory multiple myeloma. N Engl J Med. 2019;380:1726–37. doi:10.1056/NEJMoa1817226.
- Ali SA, Shi V, Maric I, Wang M, Stroncek DF, Rose JJ, Brudno JN, Stetler-Stevenson M, Feldman SA, Hansen BG, et al. T cells expressing an anti-B-cell maturation antigen chimeric antigen receptor cause remissions of multiple myeloma. Blood. 2016;128:1688–700. doi:10.1182/blood-2016-04-711903.
- Gou L, Gao J, Yang H, Gao C. The landscape of CAR T-cell therapy in the United States and China: a comparative analysis. Int J Cancer. 2019;144:2043–50. doi:10.1002/ijc.31924.
- Wei J, Guo Y, Wang Y, Wu Z, Bo J, Zhang B, Zhu J, Han W. Clinical development of CAR T cell therapy in China: 2020 update. Cell Mol Immunol. 2021 Apr;18(4):792–804. doi:10.1038/s41423-020-00555-x
- Martinez M, Moon EK. CAR T cells for solid tumors: new strategies for finding, infiltrating, and surviving in the tumor microenvironment. Front Immunol. 2019;10. doi:10.3389/fimmu.2019.00128.
- Chen J, López-Moyado IF, Seo H, Lio C-WJ, Hempleman LJ, Sekiya T, Yoshimura A, Scott-Browne JP, Rao A. NR4A transcription factors limit CAR T cell function in solid tumours. Nature. 2019;567:530–34. doi:10.1038/s41586-019-0985-x.
- Ma L, Dichwalkar T, Chang JYH, Cossette B, Garafola D, Zhang AQ, Fichter M, Wang C, Liang S, Silva M, et al. Enhanced CAR–T cell activity against solid tumors by vaccine boosting through the chimeric receptor. Science. 2019;365:162–68. doi:10.1126/science.aav8692.
- Grupp SA, Kalos M, Barrett D, Aplenc R, Porter DL, Rheingold SR, Teachey DT, Chew A, Hauck B, Wright JF, et al. Chimeric antigen receptor-modified T cells for acute lymphoid leukemia. N Engl J Med. 2013;368:1509–18. doi:10.1056/NEJMoa1215134.
- Kalos M, Levine BL, Porter DL, Katz S, Grupp SA, Bagg A, June CH. T cells with chimeric antigen receptors have potent antitumor effects and can establish memory in patients with advanced leukemia. Sci Transl Med. 2011;3(95):95ra73. doi:10.1126/scitranslmed.3002842.
- Morgan RA, Yang JC, Kitano M, Dudley ME, Laurencot CM, Rosenberg SA. Case report of a serious adverse event following the administration of t cells transduced with a chimeric antigen receptor recognizing ERBB2. Mol Ther. 2010;18:843–51. doi:10.1038/mt.2010.24.
- Maude SL, Frey N, Shaw PA, Aplenc R, Barrett DM, Bunin NJ, Chew A, Gonzalez VE, Zheng Z, Lacey SF, et al. Chimeric antigen receptor T cells for sustained remissions in leukemia. N Engl J Med. 2014;371:1507–17. doi:10.1056/NEJMoa1407222.
- Neelapu SS, Locke FL, Bartlett NL, Lekakis LJ, Miklos DB, Jacobson CA, Braunschweig I, Oluwole OO, Siddiqi T, Lin Y, et al. Axicabtagene ciloleucel CAR T-cell therapy in refractory large B-Cell lymphoma. N Engl J Med. 2017;377:2531–44. doi:10.1056/NEJMoa1707447.
- Schuster SJ, Bishop MR, Tam CS, Waller EK, Borchmann P, McGuirk JP, Jäger U, Jaglowski S, Andreadis C, Westin JR, et al. Tisagenlecleucel in adult relapsed or refractory diffuse large B-cell lymphoma. N Engl J Med. 2019;380:45–56. doi:10.1056/NEJMoa1804980.
- Park JH, Rivière I, Gonen M, Wang X, Sénéchal B, Curran KJ, Sauter C, Wang Y, Santomasso B, Mead E, et al. Long-term follow-up of CD19 CAR therapy in acute lymphoblastic leukemia. N Engl J Med. 2018;378:449–59. doi:10.1056/NEJMoa1709919.
- Kochenderfer JN, Dudley ME, Kassim SH, Somerville RPT, Carpenter RO, Maryalice -S-S, Yang JC, Phan GQ, Hughes MS, Sherry RM, et al. Chemotherapy-refractory diffuse large B-cell lymphoma and indolent B-cell malignancies can be effectively treated with autologous T cells expressing an anti-CD19 chimeric antigen receptor. J Clin Oncol. 2015;33:540–49. doi:10.1200/JCO.2014.56.2025.
- Lee DW, Kochenderfer JN, Stetler-Stevenson M, Cui YK, Delbrook C, Feldman SA, Fry TJ, Orentas R, Sabatino M, Shah NN, et al. T cells expressing CD19 chimeric antigen receptors for acute lymphoblastic leukaemia in children and young adults: a phase 1 dose-escalation trial. Lancet. 2015;385:517–28. doi:10.1016/S0140-6736(14)61403-3.