ABSTRACT
Vaccines offer a promising prophylactic and therapeutic intervention to counteract opioid use disorders (OUD) and fatal overdoses. Vaccines generate opioid-specific antibodies that bind the target opioid, reducing drug distribution to the brain and preventing drug-induced behavioral and pharmacological effects. Due to their selectivity, anti-opioid vaccines can be administered in combination with FDA-approved medications. Because patients with OUD or other substance use disorders may be affected by other multifactorial co-morbidities, such as infection or depression, it is important to test whether vaccine efficacy is modified by factors that may impact individual innate or adaptive immunity. To that end, this study tested whether housing conditions would affect the efficacy of two lead vaccine formulations targeting oxycodone and fentanyl in male mice and rats, and further analyzed whether differences in the gastrointestinal (GI) microbiome would be correlated with either vaccine efficacy or housing conditions. Results showed that housing mice and rats in either conventional (non-controlled) or specific pathogen-free (SPF, sterile barrier maintained) environment did not affect vaccine-induced antibody responses against oxycodone and fentanyl, nor their efficacy against oxycodone- and fentanyl-induced antinociception, respiratory depression, and bradycardia. Differences in the GI microbiome detected via 16S rRNA gene sequencing were related to the housing environment. This study supports use of anti-opioid vaccines in clinical populations that may display deficits in microbiome function.
Introduction
The epidemic of opioid-related fatal overdoses constitutes a significant public health burden. An estimated 2 million people in the US suffer from opioid use disorders (OUD)Citation1 and two out of three drug overdoses in 2018 involved opioids.Citation2 Synthetic opioids such as fentanyl and its analogs are increasingly prevalent in the community and have proven especially deadly. Synthetic opioids were responsible for 67% of opioid-related deaths in 2018.Citation2 During the COVID-19 pandemic, the incidence of opioid-related fatal overdoses further increased, highlighting the complex interplay of OUD and other public health threats.Citation3 Current treatments for OUD include medication-assisted therapy (MAT) with drugs such as methadone, buprenorphine, and naltrexone, as well as overdose reversal using naloxone. However, these medications either display potential abuse liability or can complicate necessary pain management. Vaccines have been explored as a viable addition to the OUD-treatment repertoire and have displayed efficacy against several opioids in mice, rats, dogs, and nonhuman primates.Citation4–12 Upon active immunization, vaccines elicit drug-specific polyclonal antibodies that bind the target drug preventing the distribution of free (unbound) drug across the blood–brain barrier, and reduce drug-induced pharmacological and behavioral effects.Citation8 Vaccine efficacy varies across formulations containing different components, such as haptens, carrier proteins, and adjuvants.Citation9,Citation12–22 Individual variability in vaccine efficacy has been shown to relate to the preexisting antigen-specific B and T cell repertoire, processes involved in CD4+ T cell-dependent B cell activation, and selected cytokine pathways.Citation13,Citation15,Citation23,Citation24 Additionally, pre-clinical studies in mice have shown that sex and strain differences may have an impact on the efficacy of vaccines against heroin,Citation25 oxycodone,Citation15 and cocaine.Citation26 Outside of the laboratory, there are many factors that may impact translation such as age, sex, genetics, and environmental influences. While some of these factors have been explored in the context of OUD vaccines, many others have not.
One such factor is the link between environmental conditions that may impact the gastrointestinal (GI) microbiome and the body’s immune response to vaccines. Our knowledge of the relationship between the environment, GI microbiome, and our health continues to grow as more studies investigate this connection. In the context of vaccines, the composition of the GI microbiota has been shown to affect innate and adaptive immune responses to vaccines, and vaccine efficacy in the clearance of pathogens in both pre-clinical and clinical studies.Citation27–30 Given that opioid use is linked to an altered GI microbiome,Citation31–35 the potential for gut dysbiosis in the target population to receive OUD vaccines is high. While pre-clinical studies involving vaccines against OUD or other substance use disorders involve immunization of laboratory animals in either conventional or specific pathogen-free (SPF) housing conditions, previous literature indicates that the abnormally hygienic environment of laboratory mice may impact their immune system. This was demonstrated by studies of “dirty mice” or “pet store mice,” which were bred outside of the more controlled microbial environment (e.g., SPF) of most research animals, which resulted in more robust immune responses to infection.Citation36 Therefore, it is essential to test whether different housing conditions would affect vaccine efficacy against OUD, and whether housing conditions would differentially affect the GI microbiome in vaccinated and control subjects. Such information will help to interpret pre-clinical data across laboratories using mice and rats housed under different conditions, and to ensure validity of findings across academic labs and contract research organizations (CROs) conducting GLP studies to support IND filing. Additionally, understanding the contribution that housing or the microbiome may have on therapeutic outcomes will inform clinical management of OUD patients, who may have altered flora based upon the effects of opioids on the GI tract.
In this study, we tested the hypothesis that the microbial environment associated with different housing conditions would alter responses to OUD vaccines. Mice and rats were housed in either SPF conditions (wherein a sterile barrier is kept throughout the study) or non-sterile housing, where no such barrier is maintained (termed “conventional housing” in many academic and commercial vivaria, and for the remainder of this manuscript). We then assessed the effect of these housing conditions on the efficacy of vaccines against oxycodone and fentanyl through examination of parameters such as the generation of drug-specific antibodies, inhibition of opioid-induced behaviors, and blockage of drug distribution to the brain in mice and rats. Finally, we tested mice housed under different conditions for changes in the microbiome that may be related to either differential housing or administration of vaccines.
Materials and methods
Drugs and reagents
Oxycodone and fentanyl were obtained from the University of Minnesota Pharmacy. Drug doses and concentrations are expressed as weight of the free base. Subunit keyhole limpet hemocyanin (sKLH) was obtained as GMP-grade source (Biosyn, Carlsbad, CA).
Animals
Male BALB/c mice (Jackson Laboratories, Bar Harbor, ME) and male Sprague–Dawley rats (Envigo, Madison, WI) were housed in a 14/10 hour light/dark cycle and fed ad libitum. Mice were 6 weeks old on arrival, while rats were 2 months old on arrival. Specified pathogen-free (SPF) animals were handled in a laminar flow hood under sterile conditions for the duration of the experiment. Specific Pathogen-Free facilities are regularly tested by RAR to ensure maintenance of SPF status. Conventionally housed animals were kept in a separate facility where no sterile barrier was maintained. Mice and rats were tested in the same room for vaccine efficacy against drug-induced behavioral effects. All testing occurred in the light cycle.
Vaccine formulation
The anti-oxycodone conjugate vaccine consists of an oxycodone-based hapten (OXY) conjugated to the subunit keyhole limpet hemocyanin carrier protein (sKLH) through a tetraglycine linker. The OXY-sKLH has been extensively described in mice and rats, and it has shown efficacy against oxycodone-induced behavioral, pharmacological, and toxic effects.Citation6,Citation17,Citation37,Citation38 The anti-fentanyl conjugate vaccine consists of a fentanyl-based hapten (F) conjugated to the sKLH through a tetraglycine linker, which has been previously tested in both mice and rats.Citation20,Citation21
Immunization
Mice were immunized intramuscularly (i.m.) with 60 µg of F-sKLH or unconjugated sKLH carrier protein as a control. Conjugates were adsorbed on 300 µg of aluminum adjuvant (Alhydrogel85, Brenntag) and delivered in a final volume of 30 µl in each hind leg for a total of 60 µl. Mice were immunized on days 0, 14, and 28. Rats were immunized i.m. with 60 µg of either OXY-sKLH or sKLH. Conjugates were adsorbed on 90 µg of aluminum to a final volume of 150 µl and delivered into one hind leg. Rats were immunized on days 0, 21, and 42.
Antibody analysis
Serum antibody analysis was performed via indirect ELISA on blood sampled from facial veins in mice (days 14 and 34) and from tail veins in rats (day 49). Ninety-six-well plates were coated with 5 ng/well of F-BSA conjugate or OXY-OVA conjugate (for mice and rats, respectively) or unconjugated BSA or OVA as a control. Conjugates were diluted in 50 mM Na2CO3, pH 9.6 (Sigma) and blocked with 1% porcine gelatin. Serum was incubated on the plate and then washed and incubated with an HRP-conjugated goat anti-mouse IgG (1:30,000, Jackson ImmunoResearch Laboratories), IgG1 (1:35,000, Alpha Diagnostic International), IgG2a (1:7500, Alpha Diagnostic International), or goat anti-rat IgG (1:50,000, Jackson ImmunoResearch Laboratories) to assess hapten-specific serum IgG antibody levels using statistical analysis previously described.Citation23,Citation37
Effect of vaccine on opioid-induced antinociception
To determine the efficacy of the vaccine to block opioid-induced analgesia, a hot plate test was performed to establish whether vaccination protects against centrally-mediated effects of opioids. Rodents were acclimated to the testing environment for 1 h prior to measuring baseline. Maximum latency to respond was either 30 s (rats) or 60 s (mice) to avoid tissue damage due to thermal injury. Rodents were placed on a hot plate (Columbus Instruments, OH) set to 54°C and removed after displaying a lift or flick of the hind paw or jumping. In mice, baselines were measured on day 35 (7 days after last immunization), followed by administration of 0.05 mg/kg fentanyl, subcutaneously (s.c.). The hot plate response was measured again 30 min after injection. In rats, baselines were measured on day 56 (14 days after last immunization), and rats were given 2.25 mg/kg of oxycodone, delivered s.c. at time 0 and again at 17 min (cumulative dose of 4.5 mg/kg oxycodone). The hot plate response was measured at 15 and 30 min. Data are displayed as percent MPE calculated as: (postdrug latency − baseline latency)/(maximal cutoff − baseline latency) × 100.
Effect of vaccine on opioid-induced respiratory depression and bradycardia
Oxygen saturation, heart rate (beats per minute, BPM), and breath rate (breaths per minute, BRPM) were measured by pulse oximetry before and after drug administration in rats. Oximetry was measured using a MouseOx Plus (Starr Life Sciences, Oakmont, PA). Rats were acclimated to the testing environment for 1 h prior to measuring baseline. After collection of baseline responses, rats were given two s.c. doses of 2.25 mg/kg oxycodone, delivered at time 0 and 17 min. Oximetry measurements were collected at baseline and post-challenge at 15 and 30 min.
Analysis of oxycodone and fentanyl concentrations in serum and brain
After the final oximetry and hot plate measurements, animals were anesthetized, decapitated, and trunk blood and brain were collected for analysis. For fentanyl samples, samples were processed using liquid chromatography coupled to mass spectrometry (LC-MS) as previously described.Citation20 Oxycodone samples were processed using gas chromatography coupled to mass spectrometry (GC-MS) as previously described.Citation6
Analysis of GI microbiome in vaccinated mice
Murine fecal samples were collected pre-challenge on day 35 and flash frozen in liquid nitrogen. The fecal content was processed using DNeasy PowerSoil® kits (Qiagen, Germantown, Maryland) modified to include a bead-beating step where fecal pellets were lysed using glass beads by a MagnaLyser tissue disruptor.Citation31 Sequencing and bioinformatics were performed by the University of Minnesota Genomic Center, MN, United States. At the University of Minnesota, after DNA isolation, 16S ribosomal DNA hypervariable regions V5 and V6 were amplified via PCR using primers with the V5F RGGATTAGATACCC and V6R CGACRRCCATGCANCACCT gene-specific sequences, Illumina adaptors, and molecular barcodes as described to produce 427 base pair (bp) amplicons. Samples were sequenced on an Illumina MiSeq (Illumina, San Diego, California) using MiSeq 600 cycle v3 kit.Citation39
16S rRNA sequencing data analysis
Primer sequences were removed from raw sequencing reads and low-quality bases (Phred score <20) were trimmed from 3ʹ end using Cutadapt.Citation40 Microbial taxonomy assignment abundance quantification was analyzed with Greengenes database (ver.08/13) using dada2 pipeline.Citation41 Microbial diversity was quantified by Shannon’s index (α-diversity), and Bray–Curtis dissimilarity (β-diversity) using R package “vegan.”Citation42 The dissimilarity between pairs of treatment groups was assessed using permutation multivariate analysis of ANOVA (PERMANOVA), and the significance of pair-wise comparison was adjusted for multiple comparison using Bonferroni correction. Individual differential taxa were identified using DESeq2 with significance determined based on a false discovery rate (FDR) of 0.05.Citation43 Relative abundance of functional content of microbial communities was obtained using PICRUSt.Citation44
Results
Housing conditions do not affect vaccine efficacy against fentanyl in mice
To determine the effects of housing conditions on vaccine efficacy, mice housed in either conventional or SPF conditions were immunized on days 0, 14, and 28 with either F-sKLH or unconjugated sKLH (control). After a primary immunization, F-sKLH elicited significantly lower antibody titers in SPF mice compared to conventionally housed mice ()). However, on day 34 no significant differences in antibody response were detected between conventionally and SPF housed mice ()). To further assess whether housing conditions would affect the quality of the humoral response, IgG1 and IgG2a subclasses were measured on day 34. Vaccinated groups in both SPF and conventional housing showed equivalent levels and distribution of IgG1 ()) and IgG2a titers ()).
Figure 1. In vivo comparison of the effect of conventional and SPF housing conditions on vaccine responses against fentanyl in mice . The F-sKLH vaccine and the unconjugate sKLH control were tested in male BALB/c mice (n = 12/group) in either conventional or SPF housing. Conjugates were adsorbed on alum adjuvant and injected in mice i.m. on days 0, 14, and 28. Blood was collected via facial vein at days 14 and 34 to test for fentanyl-specific serum antibody titers via ELISA. IgG titers are shown for (a) 14 days post-immunization and (b) 34 post-immunization. Serum from 34 days post-immunization was tested for (c) IgG1 subclass titers and (d) IgG2a subclass titers. On day 35, all mice were challenged s.c. with 0.05 mg/kg fentanyl. (e) Effect of F-sKLH in blocking fentanyl-induced antinociception in the hot plate test at 30-min post-challenge. After being tested on the hot plate, mice were euthanized and brains were collected. (f) Brain fentanyl concentrations in mice measured via LC-MS. Statistical analysis performed via Welch’s t-test between control and immunized mice in different housing conditions, after establishing no statistical difference between control groups under each housing condition via t-test. * p ≤ 0.05, ** p ≤ 0.01, *** p ≤ 0.001, **** p ≤ 0.0001.
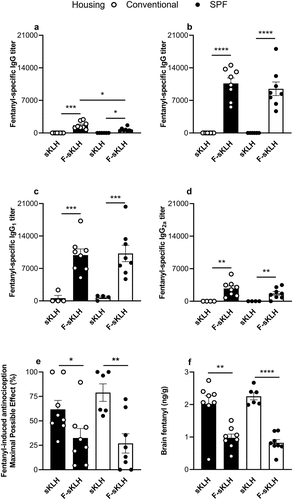
After immunization, the ability of F-sKLH to reduce drug distribution to the brain was assessed by a hot plate test of centrally mediated analgesia. Animals were challenged with 0.05 mg/kg s.c. fentanyl and the hot plate response was measured 30 min post-challenge and compared to baseline as a percent of maximum possible effect (MPE). Both conventionally and SPF housed vaccinated mice showed a significant reduction in fentanyl-induced analgesia (p < 0.05) at 30 min post-challenge, with no significant difference seen between housing conditions in vaccinated groups ()). Fentanyl distribution to the brain was also directly analyzed via LC-MS post-mortem ()). Across both conditions, the F-sKLH vaccine was able to effectively block fentanyl distribution to the brain as compared to control animals. All together, these data indicate that housing conditions do not have a significant effect on fentanyl vaccine efficacy in mice.
Housing conditions do not affect vaccine efficacy against oxycodone in rats
An anti-oxycodone conjugate vaccine was used to assess vaccine efficacy in rats housed in either conventional or SPF housing conditions (). Rats were immunized on days 0, 21, and 42 with either an oxycodone conjugate (OXY-sKLH) vaccine or the unconjugated carrier protein as a control. Blood was collected on day 49 to assess oxycodone-specific antibodies in serum via ELISA ()). While the vaccine produced high anti-oxycodone serum IgG titers, there was no significant differences between housing conditions.
Figure 2. In vivo comparison of conventional and SPF housing conditions on vaccine responses against oxycodone in rats. The OXY-sKLH vaccine was tested in male Sprague–Dawley rats (n = 12/group) in either conventional or SPF housing. Conjugates were adsorbed on alum adjuvant and injected i.m. on days 0, 21 and 42. Blood was collected via tail vein on day 49 to measure (a) oxycodone-specific IgG tiers via ELISA. On day 56, all rats were challenged with two s.c. doses of 2.25 mg/kg oxycodone at 0 min and 17 min (to provide a cumulative dose of 4.5 mg/kg). Hotplate responses were measured at baseline, 15 mins and 30 mins, and maximum possible effect (MPE%) was calculated for (b) 2.25 mg/kg and (c) 4.5 mg/kg cumulative dose. Efficacy of OXY-sKLH was further assessed by measuring (d) oxygen saturation, (e) heart rate and (f) breath rate at baseline, 15 min, and 30 min post-drug challenge. After 30 min, rats were euthanized for blood and brain collection to measure oxycodone distribution to the (g) serum and (h) brain, via GC-MS. Statistical analysis was performed via Welch’s t-test between control and immunized rats in different housing conditions. Symbols: * represents significance between conventionally housed animals or as indicated by lines between groups, # represents significance between SPF-housed animals. * or # p ≤ 0.05, ** p ≤ 0.01, *** p ≤ 0.001, **** p ≤ 0.0001.
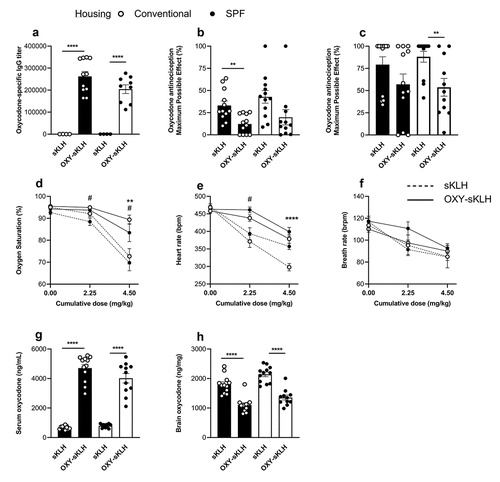
On day 56 (14 days after final immunization), rats were challenged with 2.25 mg/kg dose of s.c. oxycodone at time 0 and a second dose 17 min later. Vaccine efficacy was assessed by hot plate antinociception () and (c)) measured prior to drug delivery and at 15- and 30-min post-challenge. After each dose, rats in both housing conditions showed trends toward significantly lowered MPE%, but these trends were only statistically significant for conventional housing after the first dose, and for SPF after the second dose.
As another measure of vaccine efficacy, a pulse oximeter was used to assess oxycodone-induced respiratory depression and bradycardia, which are both commonly associated with opioid use.Citation45 At a dose of 2.25 mg/kg oxycodone, only SPF-housed control animals showed a significant decrease in oxygen saturation compared to control ()). However, at a cumulative dose of 4.5 mg/kg oxycodone, both conventional and SPF housed animals showed significant differences in oxygen saturation compared to their respective controls. This suggests that the oxycodone conjugate vaccine was able to effectively protect animals against this opioid-induced respiratory depression under both housing conditions.
The heart rate of control animals decreased in both SPF and conventionally housed rats after oxycodone administration ()). At a dose of 2.25 mg/kg oxycodone, this drop was statistically significant only between vaccine and control groups housed under SPF conditions. At a cumulative dose of 4.5 mg/kg oxycodone, conventionally housed animals showed a significant change in heart rate between vaccine and control groups, which was not seen in the SPF animals. Breath rates of animals in all housing conditions and vaccine groups trended downwards as the dose of oxycodone increased, but none were statistically significant ()). After 30 min post-drug challenge, blood and brain were collected and tested to determine the distribution of oxycodone in each compartment. In both SPF and conventionally housed animals, vaccination with OXY-sKLH led to a significant increase in serum oxycodone concentrations and a decrease in brain oxycodone concentrations ()) and (h).
Magnitude of change in metrics of vaccine efficacy do not differ between SPF and conventionally housed animals
While there was no significant difference between vaccine response in SPF and conventionally housed rodents, the percent change in response from control was calculated in each group to ensure the magnitude of response was similar between groups. Mean of the control was calculated and compared between SPF and conventional housing. Next, the percent change in efficacy between the immunized animals and the mean of the control was determined and compared between housing conditions (). There was no change in magnitude of the response in any of the metrics tested, with the exception of the rat serum oxycodone concentrations. Of note, the mean control values were significantly different between both rat serum and brain oxycodone concentrations when comparing housing conditions.
Table 1. Statistical analysis of metrics of vaccine efficacy between housing conditions in control groups and change from percent change from mean control value in immunized groups
Effect of housing conditions and vaccination on microbiome in mice
To evaluate whether housing conditions or vaccination group are responsible for microbiota changes, gut microbial composition was evaluated by 16S rRNA gene sequencing of fecal pellets from each group. The alpha diversity was quantified by the Shannon diversity index, which accounts for both operational taxonomic unit (OTU) richness and evenness. The Shannon diversity was significantly decreased in SPF groups compared with conventionally raised groups, regardless of vaccination status ()).
Figure 3. The impact of housing environment and vaccination on mouse GI microbiome. After immunization, mouse fecal samples were collected for analysis of microbiome via 16s rRNA sequencing. (a) Box plots show that conventionally housed mice exhibited a significant increase in α-diversity (calculated by Shannon’s index) compared to SPF mice. (b) Non-metric multidimensional scaling (NMDS) plot was used to visualize the unweighted UniFrac distances of each group (calculated by Bray-Curtis distance). (c) Changes in abundance of bacteria by environment or vaccination at family and genus levels were displayed in the heatmaps. Bacteria were selected from the comparison of SPF and conventionally housed sKLH controls with FDR<0.1. (d) In control mice housed in either SPF or conventional conditions, bacterial gene functions were predicted from 16S rRNA gene-based microbial compositions using the PICRUSt.
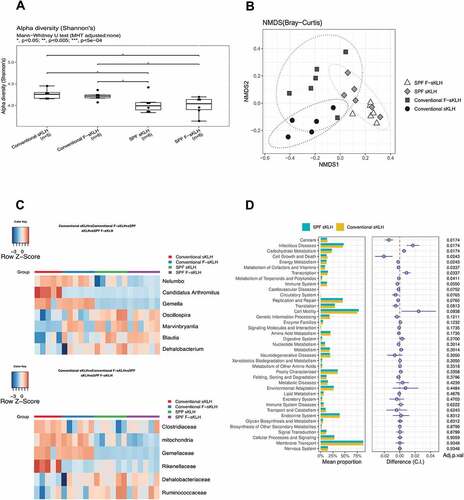
Nonmetric multidimensional scaling (NMDS) analysis of the Bray–Curtis distance plot was also performed among the fecal samples from different groups. There was a clear separation between conventional and SPF sKLH-immunized controls (PERMANOVA test, FDR q = 0.007; )) and conventional and SPF F-sKLH (PERMANOVA test, FDR q = 0.007; ), Table S1). This suggests the changes in microbiota are influenced more by environmental conditions than vaccination status.
SPF mice receiving sKLH displayed a decreased relative abundance of Proteobacteria at the phylum level, Rikenellaceae, Gemellaceae, and Clostridiaceae at the family level and Candidatus Arthromitus, Gemella, and Nelumbo at the genus level compared to conventionally-housed sKLH controls. The relative abundance of Dehalobacteriacea and Ruminococcaceae at the family level and Marvinbryantia and Oscillospira at the genus levels were increased (), Table S2).
Additionally, the overlap between F-sKLH and control groups in the SPF condition (PERMANOVA test, FDR q = 0.114) represents resilience to modulation by vaccination ()). Interestingly, there was significant and distinct clustering of the F-sKLH group compared to control animals in conventional housing (PERMANOVA test, FDR q = 0.046) ()). There was a reduction of Rikenellaceae at the family level and Anaerostipes and Candidatus Arthromitus at the genus level, and expansion of Marvinbryantia at the genus level in conventionally housed F-sKLH treatment group ()).
The functional profiles of the microbial communities were analyzed in each group using PICRUSt. Of 42 KEGG pathways tested, 8 non-human-gene pathways differed in abundance between SPF and conventional raised controls (FDR q < 0.05). These include pathways related to cell growth and death, transcription, cancer, infectious diseases, carbohydrate metabolism, energy metabolism, metabolism of cofactors and vitamins, and metabolism of terpenoids and polyketides. There were no significant changes in functional composition related to vaccination in either conventional or SPF groups (), Table S3). However, when comparing SPF and conventional sKLH controls, KEGG pathways related with cell growth and death, cancer, energy metabolism, metabolism of cofactors and vitamins, and metabolism of terpenoids and polyketides were found to be down-regulated while KEGG pathways related with carbohydrate metabolism, transcription, and infectious disease were up-regulated in SPF controls. This suggests that environmental factors have a more important impact on microbial changes compared to vaccination.
Discussion and conclusion
The effect of the microbiome on health and disease has been increasingly investigated in recent years. Changes in the microbiome have been associated with cardiovascular disease, autoimmune conditions, cancer, obesity, diabetes, and potentially even mental health disorders.Citation46,Citation47 Studies have suggested that the microbiome plays a role in the immune response to vaccination,Citation27–29 making it critical that vaccine efficacy is tested in conditions that closely mimic the human microbiome. Since opioid use is also correlated to gut dysbiosis, it is even more imperative that vaccines for OUD are tested in a variety of contextsCitation31,Citation32,Citation34,Citation35 to ensure vaccines will translate into a clinical setting. In this study, we sought to determine if changes in animal housing conditions affected the efficacy of vaccines against fentanyl and oxycodone in mice and rats. We found that while there were some minor differences in microbiota diversity between the two housing conditions, there were no major differences in the efficacy of either vaccine.
Differences in housing conditions and subsequent effects on behavior and disease have been noted in the literature in both mice and rats. Published studies show that housing conditions can affect the development of experimental autoimmune encephalitis in mice,Citation48 and rats housed in conventional vs. SPF conditions showed varying responses to surgical trauma and anesthesia.Citation49 Studies exploring the differences between wild and laboratory mice also show that immune composition varies greatly between environmental conditions. Wild mice have more robust numbers and heterogeneity of circulating IgG, as well as significantly greater numbers of T cells, with less NK cells and dendritic cells.Citation50 In this study, we found that there was a significant increase in alpha diversity in the GI microbiota when comparing mice in conventional vs. SPF conditions, regardless of vaccination status. Since alterations in GI microbiota have been closely tied to many pathologies and alterations in metabolism, this study supports the need for careful consideration of mouse models when testing new therapies, and the requirement for comparisons between housing conditions to ensure microbiota differences do not affect treatment outcomes.
When analyzing the microbiome via sequencing, a distinct change in bacterial species was observed between conventionally housed control and immunized mice. Interestingly, these changes in microflora mirrored the changes observed in SPF mice when compared to conventionally housed mice. While no change in diversity between control and immunized mice was found in the SPF group, this finding may be due to a lack of microbial diversity due to the stringent microbial environment in these housing conditions. This finding suggests that the vaccine composition may have influenced the GI microbiome. While the fentanyl-based F hapten has been shown to be inactive at the mu-opioid receptor,Citation21 formal GLP toxicology studies of conjugate vaccines containing fentanyl-based haptens are warranted. Histopathology and blood chemistry analysis in mice injected with OXY-sKLH,Citation24 as well as GLP toxicology studies of OXY-sKLH in rats (Pravetoni, personal communication), did not show any sign of toxicity. There have been reports that opioids modulate toll-like receptor (TLR) 4,Citation51 a TLR involved in regulation of the GI microbiome, and that OXY-KLH responses were altered in TLR4KO mice.Citation17 Follow-up studies will be needed to explore any potential interaction between anti-opioid vaccination, immunity, and microbiome.
Despite these minor differences in GI microbiota, we found no major significant differences in the efficacy of vaccines targeting fentanyl or oxycodone when examining opioid-specific antibody titers, pharmacokinetic parameters, and physiological and behavior responses. This outcome was unexpected, as there have been several studies noting the effects of the microbiome on immune response after vaccination.Citation27–30 These results help validate previous data of preclinical studies of anti-opioid vaccines conducted in both SPF and conventional housing conditions. This also suggests that efficacy of anti-opioid vaccines are not significantly affected by environmental factors associated with housing conditions in laboratory animals, making interpretation of data from different laboratories more consistent. Additionally, these data suggest that OUD patients with opioid-induced dysbiosis may equally benefit from anti-opioid vaccination.
One point of interest is that in control rats, serum, and brain oxycodone levels were significantly increased in SPF housing conditions compared to conventional conditions (). This would suggest that the metabolism of oxycodone is decreased in SPF conditions, which may be linked to differences in the microbiota. Published literature has established a link between diversity of GI microbiota and the dysregulation of metabolism of morphine.Citation32 While few studies have addressed the potential of the microbiome to affect the metabolism of other opioid molecules, such as oxycodone or fentanyl, it is possible that decreased microbial diversity in SPF rats would lead to decreased oxycodone metabolism, resulting in increased oxycodone concentrations in the serum and brain. This would be consistent with the findings that the functional differences in microbiome between SPF and conventional mice ()) resulted in differences in metabolism of carbohydrates, vitamins, cofactors, terpenoids, and polyketides. These potential differences in metabolism and microbiome conditions will need to be further evaluated, although they may be of note for future experimental design and when comparing studies of laboratory animals housed under different conditions.
Future follow-up experiments will address some of the limitations of the current study to further explore the interplay between the microbiome and vaccination against OUD. One limitation is that current studies were only performed in male mice and rats. Significant differences in titers between male and female mice after anti-opioid vaccination were previously reported,Citation15,Citation25 and there have been noted sex differences in microbiome composition.Citation52 Future studies will compare male and female animals to determine whether the GI microbiome affects the efficacy of anti-opioid vaccines in a sex-dependent fashion. Another limitation of this study was that the SPF condition likely does not fully capture the changes in microbiome that are found in chronic opioid users. For example, previous literature indicates that active opioid users have an increase in abundance in Bifidobacterium and decreases in Baceterodacea, Clostridiales XI, and Ruminococcaceae, which were not recapitulated in the current study.Citation34 However, there was a decrease in overall alpha diversity and in Rikenellaceae, which are consistent with previous literature using mice chronically treated with morphine.Citation32 While it has been shown that rats chronically treated with opioid agonists and antagonists still mount an anti-opioid antibody response after vaccination,Citation20,Citation21,Citation38 the relationship between chronic opioid treatment and microbiome composition has not yet been explored in the context of vaccines for OUD. This will be critical in future studies to determine whether opioid users will respond to anti-opioid vaccination.
As testing of OUD vaccines in clinical settings is underway (e.g., OXY-sKLH, NCT 04458545), the impact of vaccination on GI microbiota and vice versa will help to inform clinicians on therapeutic management of OUD patients. Pre-clinical studies are often conducted in microbially controlled environments, which may be masking or changing pathologies and/or outcomes related to disease treatment. In the case of OUD, looking at the impact of the GI microbiome on potential therapeutic efficacy becomes even more crucial when the target population may have a higher incidence of abnormal microbiome function. We found that while SPF and conventionally housed animals displayed differences in GI microbiota composition, these differences did not affect the efficacy of either an anti-oxycodone or anti-fentanyl vaccine. These studies validate previous and future data testing opioid vaccines preclinically in different housing conditions, and support future testing of OUD vaccines as a treatment strategy for individuals that may have deficits or abnormal microbiomes, as well as otherwise healthy individuals.
Abbreviations
Opioid use disorder (OUD), medication-assisted therapy (MAT), gastrointestinal (GI), specific-pathogen free (SPF), contract research organization (CRO), good laboratory practices (GLP), investigational new drug (IND), intramuscular (i.m), subcutaneous (s. c), OXY (oxycodone hapten), F (fentanyl hapten), subunit keyhole limpet hemocyanin (sKLH), bovine serum albumin (BSA), chicken ovalbumin (OVA), maximum possible effect (MPE), beats per minute (BPM), breaths per minute (BRPM), polymerase-chain reaction (PCR), Nonmetric multidimenstional scaling (NMDS)
Disclosure of potential conflicts of interest
Pravetoni is the inventor of “Fentanyl hapten-conjugates and methods for making and using same,” Application No. 62/932,757 and “Fentanyl haptens, fentanyl hapten conjugates, and methods for making and using the same,” Application No. 62/989,417. All other authors declare no competing interests.
Acknowledgments
This work was supported by U01DA038876 (MP), T32DA007097 (BC), R01DA047089 (SR), and R01DA044582 (SR).
Data availability
16s rRNA sequencing data are available under NCBI accession number PRJNA738384.
Additional information
Funding
References
- NSDUH. 2018 (accessed 2021 Jan 15). https://www.samhsa.gov/data/release/2018-national-survey-drug-use-and-health-nsduh-releases
- CDC. 2020 (accessed 2021 Jan 15). http://wonder.cdc.gov
- Network CHA Increase in fatal drug overdoses across the United States driven by synthetic opioids before and during the COVID-19 pandemic. (2020).
- Pravetoni M. Biologics to treat substance use disorders: current status and new directions. Hum Vaccin Immunother. 2016;12(12):3005–19. doi:10.1080/21645515.2016.1212785.
- Stowe GN, Vendruscolo LF, Edwards S, Schlosburg JE, Misra KK, Schulteis G, Mayorov AV, Zakhari JS, Koob GF, Janda KD, et al. A vaccine strategy that induces protective immunity against heroin. J Med Chem. 2011;54(14):5195–204. doi:10.1021/jm200461m.
- Pravetoni M, Le Naour M, Tucker AM, Harmon TM, Hawley TM, Portoghese PS, Pentel PR. Reduced antinociception of opioids in rats and mice by vaccination with immunogens containing oxycodone and hydrocodone haptens. J Med Chem. 2013;56(3):915–23. doi:10.1021/jm3013745.
- Anton B, Leff P. A novel bivalent morphine/heroin vaccine that prevents relapse to heroin addiction in rodents. Vaccine. 2006;24(16):3232–40. doi:10.1016/j.vaccine.2006.01.047.
- Pravetoni M, Comer SD. Development of vaccines to treat opioid use disorders and reduce incidence of overdose. Neuropharmacology. 2019;158:107662. doi:10.1016/j.neuropharm.2019.06.001.
- Bonese KF, Wainer BH, Fitch FW, Rothberg RM, Schuster CR. Changes in heroin self-administration by a rhesus monkey after morphine immunisation. Nature. 1974;252(5485):708–10. doi:10.1038/252708a0.
- Tenney RD, Blake S, Bremer PT, Zhou B, Hwang CS, Poklis JL, Janda KD, Banks ML. Vaccine blunts fentanyl potency in male rhesus monkeys. Neuropharmacology. 2019;158:107730. doi:10.1016/j.neuropharm.2019.107730.
- Townsend EA, Bremer PT, Faunce KE, Negus SS, Jaster AM, Robinson HL, Janda KD, Banks ML. Evaluation of a dual fentanyl/heroin vaccine on the antinociceptive and reinforcing effects of a fentanyl/heroin mixture in male and female rats. ACS Chem Neurosci. 2020;11(9):1300–10. doi:10.1021/acschemneuro.0c00064.
- Sulima A, Jalah R, Antoline JFG, Torres OB, Imler GH, Deschamps JR, Beck Z, Alving CR, Jacobson AE, Rice KC, et al. A stable heroin analogue that can serve as a vaccine hapten to induce antibodies that block the effects of heroin and its metabolites in rodents and that cross-react immunologically with related drugs of abuse. J Med Chem. 2018;61(1):329–43. doi:10.1021/acs.jmedchem.7b01427.
- Baruffaldi F, Kelcher AH, Laudenbach M, Gradinati V, Limkar A, Roslawski M, Birnbaum A, Lees A, Hassler C, Runyon S, et al. Preclinical efficacy and characterization of candidate vaccines for treatment of opioid use disorders using clinically viable carrier proteins. Mol Pharm. 2018;15(11):4947–62. doi:10.1021/acs.molpharmaceut.8b00592.
- Bremer PT, Schlosburg JE, Lively JM, Janda KD. Injection route and TLR9 agonist addition significantly impact heroin vaccine efficacy. Mol Pharm. 2014;11(3):1075–80. doi:10.1021/mp400631w.
- Crouse B, Robinson C, Huseby Kelcher A, Laudenbach M, Abrahante JE, Pravetoni M. Mechanisms of interleukin 4 mediated increase in efficacy of vaccines against opioid use disorders. NPJ Vaccines. 2020;5(1):99. doi:10.1038/s41541-020-00247-7.
- Raleigh MD, Laudenbach M, Baruffaldi F, Peterson SJ, Roslawski MJ, Birnbaum AK, Carroll FI, Runyon SP, Winston S, Pentel PR, et al. Opioid dose- and route-dependent efficacy of oxycodone and heroin vaccines in rats. J Pharmacol Exp Ther. 2018;365(2):346–53. doi:10.1124/jpet.117.247049.
- Pravetoni M, Vervacke JS, Distefano MD, Tucker AM, Laudenbach M, Pentel PR. Effect of currently approved carriers and adjuvants on the pre-clinical efficacy of a conjugate vaccine against oxycodone in mice and rats. Plos One. 2014;9(5):e96547. doi:10.1371/journal.pone.0096547.
- Robinson C, Baehr C, Schmiel SE, Accetturo C, Mueller DL, Pravetoni M. Alum adjuvant is more effective than MF59 at prompting early germinal center formation in response to peptide-protein conjugates and enhancing efficacy of a vaccine against opioid use disorders. Hum Vaccin Immunother. 2019;15(4):909–17. doi:10.1080/21645515.2018.1558697.
- Nguyen JD, Hwang CS, Grant Y, Janda KD, Taffe MA. Prophylactic vaccination protects against the development of oxycodone self-administration. Neuropharmacology. 2018;138:292–303. doi:10.1016/j.neuropharm.2018.06.026.
- Raleigh MD, Baruffaldi F, Peterson SJ, Le Naour M, Harmon TM, Vigliaturo JR, Pentel PR, Pravetoni M. A fentanyl vaccine alters fentanyl distribution and protects against fentanyl-induced effects in mice and rats. J Pharmacol Exp Ther. 2019;368(2):282–91. doi:10.1124/jpet.118.253674.
- Robinson C, Gradinati V, Hamid F, Baehr C, Crouse B, Averick S, Kovaliov M, Harris D, Runyon S, Baruffaldi F, et al. Therapeutic and prophylactic vaccines to counteract fentanyl use disorders and toxicity. J Med Chem. 2020;63(23):14647–67. doi:10.1021/acs.jmedchem.0c01042.
- Hwang CS, Bremer PT, Wenthur CJ, Ho SO, Chiang S, Ellis B, Zhou B, Fujii G, Janda KD. Enhancing efficacy and stability of an antiheroin vaccine: examination of antinociception, opioid binding profile, and lethality. Mol Pharm. 2018;15(3):1062–72. doi:10.1021/acs.molpharmaceut.7b00933.
- Laudenbach M, Baruffaldi F, Vervacke JS, Distefano MD, Titcombe PJ, Mueller DL, Tubo NJ, Griffith TS, Pravetoni M. The frequency of naive and early-activated hapten-specific B cell subsets dictates the efficacy of a therapeutic vaccine against prescription opioid abuse. J Immunol. 2015;194(12):5926–36. doi:10.4049/jimmunol.1500385.
- Laudenbach M, Baruffaldi F, Robinson C, Carter P, Seelig D, Baehr C, Pravetoni M. Blocking interleukin-4 enhances efficacy of vaccines for treatment of opioid abuse and prevention of opioid overdose. Sci Rep. 2018;8(1):5508. doi:10.1038/s41598-018-23777-6.
- Hwang CS, Smith LC, Wenthur CJ, Ellis B, Zhou B, Janda KD. Heroin vaccine: using titer, affinity, and antinociception as metrics when examining sex and strain differences. Vaccine. 2019;37(30):4155–63. doi:10.1016/j.vaccine.2019.05.061.
- Kosten TA, Shen XY, Kinsey BM, Kosten TR, Orson FM. Attenuation of cocaine-induced locomotor activity in male and female mice by active immunization. Am J Addict. 2014;23(6):604–07. doi:10.1111/j.1521-0391.2014.12152.x.
- Pulendran B. Immunology taught by vaccines. Science. 2019;366(6469):1074–75. doi:10.1126/science.aau6975.
- Collins N, Belkaid Y. Do the microbiota influence vaccines and protective immunity to pathogens? Engaging our endogenous adjuvants. Cold Spring Harb Perspect Biol. 2018;10(2):a028860. doi:10.1101/cshperspect.a028860.
- Praharaj I, John SM, Bandyopadhyay R, Kang G. Probiotics, antibiotics and the immune responses to vaccines. Philos Trans R Soc Lond B Biol Sci. 2015;370(1671):20140144. doi:10.1098/rstb.2014.0144.
- de Jong SE, Olin A, Pulendran B. The impact of the microbiome on immunity to vaccination in humans. Cell Host Microbe. 2020;28:169–79. doi:10.1016/j.chom.2020.06.014.
- Banerjee S, Sindberg G, Wang F, Meng J, Sharma U, Zhang L, Dauer P, Chen C, Dalluge J, Johnson T, Roy S. Opioid-induced gut microbial disruption and bile dysregulation leads to gut barrier compromise and sustained systemic inflammation. Mucosal Immunol. 2016;9:1418–28. doi:10.1038/mi.2016.9.
- Wang F, Meng J, Zhang L, Johnson T, Chen C, Roy S. Morphine induces changes in the gut microbiome and metabolome in a morphine dependence model. Sci Rep. 2018;8(1):3596. doi:10.1038/s41598-018-21915-8.
- Kiraly DD, Walker DM, Calipari ES, Labonte B, Issler O, Pena CJ, Ribeiro EA, Russo SJ, Nestler EJ. Alterations of the Host microbiome affect behavioral responses to cocaine. Sci Rep. 2016;6:35455. doi:10.1038/srep35455.
- Meckel KR, Kiraly DD. A potential role for the gut microbiome in substance use disorders. Psychopharmacology (Berl). 2019;236(5):1513–30. doi:10.1007/s00213-019-05232-0.
- Lee K, Vuong HE, Nusbaum DJ, Hsiao EY, Evans CJ, Taylor AMW. The gut microbiota mediates reward and sensory responses associated with regimen-selective morphine dependence. Neuropsychopharmacology. 2018;43(13):2606–14. doi:10.1038/s41386-018-0211-9.
- Beura LK, Hamilton SE, Bi K, Schenkel JM, Odumade OA, Casey KA, Thompson EA, Fraser KA, Rosato PC, Filali-Mouhim A, et al. Normalizing the environment recapitulates adult human immune traits in laboratory mice. Nature. 2016;532(7600):512–16. doi:10.1038/nature17655.
- Pravetoni M, Le Naour M, Harmon TM, Tucker AM, Portoghese PS, Pentel PR. An oxycodone conjugate vaccine elicits drug-specific antibodies that reduce oxycodone distribution to brain and hot-plate analgesia. J Pharmacol Exp Ther. 2012;341(1):225–32. doi:10.1124/jpet.111.189506.
- Raleigh MD, Peterson SJ, Laudenbach M, Baruffaldi F, Carroll FI, Comer SD, Navarro HA, Langston TL, Runyon SP, Winston S, et al. Safety and efficacy of an oxycodone vaccine: addressing some of the unique considerations posed by opioid abuse. PLoS One. 2017;12(12):e0184876. doi:10.1371/journal.pone.0184876.
- Gohl DM, Vangay P, Garbe J, MacLean A, Hauge A, Becker A, Gould TJ, Clayton JB, Johnson TJ, Hunter R, et al. Systematic improvement of amplicon marker gene methods for increased accuracy in microbiome studies. Nat Biotechnol. 2016;34(9):942–49. doi:10.1038/nbt.3601.
- Martin M. Cutadapt removes adapter sequences from high-throughput sequencing reads. EMBnet J. 2011;17(1):3. doi:10.14806/ej.17.1.200.
- Callahan BJ, McMurdie PJ, Rosen MJ, Han AW, Johnson AJA, Holmes SP. DADA2: high-resolution sample inference from illumina amplicon data. Nat Methods. 2016;13(7):581–83. doi:10.1038/nmeth.3869.
- vegan: Community Ecology Package (2017).
- Love MI, Huber W, Anders S. Moderated estimation of fold change and dispersion for RNA-seq data with DESeq2. Genome Biol. 2014;15(12):550. doi:10.1186/s13059-014-0550-8.
- Langille MG, Zaneveld J, Caporaso JG, McDonald D, Knights D, Reyes JA, Clemente JC, Burkepile DE, Vega Thurber RL, Knight R, et al. Predictive functional profiling of microbial communities using 16S rRNA marker gene sequences. Nat Biotechnol. 2013;31(9):814–21. doi:10.1038/nbt.2676.
- Boyer EW. Management of opioid analgesic overdose. N Engl J Med. 2012;367(2):146–55. doi:10.1056/NEJMra1202561.
- Clapp M, Aurora N, Herrera L, Bhatia M, Wilen E, Wakefield S. Gut microbiota’s effect on mental health: the gut-brain axis. Clin Pract. 2017;7:987. doi:10.4081/cp.2017.987.
- Shreiner AB, Kao JY, Young VB. The gut microbiome in health and in disease. Curr Opin Gastroenterol. 2015;31(1):69–75. doi:10.1097/MOG.0000000000000139.
- Arndt A, Hoffacker P, Zellmer K, Goecer O, Recks MS, Kuerten S. Conventional housing conditions attenuate the development of experimental autoimmune encephalomyelitis. Plos One. 2014;9(6):e99794. doi:10.1371/journal.pone.0099794.
- Letson HL, Morris J, Biros E, Dobson GP. Conventional and specific-pathogen free rats respond differently to anesthesia and surgical trauma. Sci Rep. 2019;9(1):9399. doi:10.1038/s41598-019-45871-z.
- Abolins S, King EC, Lazarou L, Weldon L, Hughes L, Drescher P, Raynes JG, Hafalla JCR, Viney ME, Riley EM, et al. The comparative immunology of wild and laboratory mice, mus musculus domesticus. Nat Commun. 2017;8(1):14811. doi:10.1038/ncomms14811.
- Zhang P, Yang M, Chen C, Liu L, Wei X, Zeng S. Toll-like receptor 4 (TLR4)/opioid receptor pathway crosstalk and impact on opioid analgesia, immune function, and gastrointestinal motility. Front Immunol. 2020;11:1455. doi:10.3389/fimmu.2020.01455.
- Kim YS, Unno T, Kim BY, Park MS. Sex differences in gut microbiota. World J Mens Health. 2020;38:48–60. doi:10.5534/wjmh.190009.