ABSTRACT
Norovirus (NoV) has been recognized as a leading cause of gastroenteritis worldwide. This review estimates the prevalence and genotype distribution of NoV in China to provide a sound reference for vaccine development. Studies were searched up to October 2020 from CNKI database and inclusion criteria were study duration of at least one calendar year and population size of >100. The mean overall NoV prevalence in individuals with sporadic diarrhea/gastroenteritis was 16.68% (20796/124649, 95% CI 16.63–16.72), and the detection rate of NoV was the highest among children. Non-GII.4 strains have replaced GII.4 as the predominant caused multiple outbreaks since 2014. Especially the recombinant GII.P16-GII.2 increased sharply, and virologic data show that the polymerase GII.P16 rather than VP1 triggers pandemic. Due to genetic diversity and rapid evolution, predominant genotypes might change unexpectedly, which has become major obstacle for the development of effective NoV vaccines.
Introduction
The burden of NoV worldwide
With the gradual promotion of rotavirus vaccines, NoV has now become the key pathogen associated with gastroenteritis/diarrhea.Citation1,Citation2 It was estimated that more than 600 million people worldwide suffer from acute gastroenteritis (AGE) due to NoV infection, resulting in approximately 210,000 deaths annually.Citation3 Analysis of the burden of diarrhea across 195 countries by the 2016 Global Burden of Diseases, Injuries, and Risk Factors Study (GBD)Citation4 attributed an estimated 19496 deaths to NoV (95% CI: 8747–38421). Among these cases, a significant number were younger than 5 years (10,629; 5274–19582) or older than 70 years (3693; 810–8647). Bartsch in 2016 concluded that NoV caused direct health system costs 4.2 billion U.S. dollars and the socioeconomic burden amounted to more than 60 billion U.S. dollars per year globally, 39.8 billion U.S. dollars of which could be attributed to the disease in children under 5 years old.Citation5
NoV etiology
NoV is a non-enveloped icosahedral symmetrical structure with a diameter of 25–40 nm. NoV genome is 7.5–7.7 kb single-stranded, positive-stranded RNA, with 3 open reading regions (ORF-1, ORF-2 and ORF-3). ORF1 encodes the nonstructural proteins, including NTPase, 3 C-like protease, and RNA-dependent RNA polymerase (RdRp). ORF2 encodes the capsid protein VP1 and ORF3 encodes the minor structural protein VP2. VP2 is located inside the viral particle and is thought to participate in capsid aggregation. According to the position of the protein in the capsid, VP1 is divided into a shell domain (S region) and a protruding domain (P region). The P domain contains the main antigenic site and is further divided into the P1 and P2 domains. The P2 region forms a binding pocket composed of an RGD-like motif located at the bottom and around certain specific Amino acid (AA); this then becomes the binding site between the virus particles and human histo-blood group antigens (HBGAs).Citation6 Importantly, this is considered to be a key aspect of immune recognition and receptor binding. In addition, the eukaryotic cell can express VP1 and self-assemble to form virus-like particles (VLPs), whose morphology and antigenicity are similar to those of natural viral particles. Thus, VLPs can be used as candidate vaccines against NoV. Due to the frequent recombination of ORF1 and ORF2 sequences, NoV strains usually use the double genotype encoding RdRp and VP1 sequence.Citation7 According to the nucleotide sequence of VP1, NoV can be divided into 7 genogroups (GI-GVII) and more than 30 genotypes. GI, GII, and GIV.1 NoV are closely related to human disease, and GII genogroup includes 22 genotypes and GI genogroup includes 9 genotypes.Citation8
The diversity of NoV genotypes and the global pandemics caused by its novel strains have shown an evolutionary pattern similar to that of influenza viruses. The Centers for Disease Control and Prevention (CDC) in United States launched CaliciNet as early as 2009 to analyze the epidemiologic of NoV and concluded that NoV has become the leading pathogen of foodborne disease. The NoV Outbreak Network in China (CaliciNet China) was not established until 2016 and involving only 6 provinces up to 2018. The selection of antigen components based on epidemiological investigation is expected to crucially provide the direction for NoV vaccine research. However, the absence of NoV epidemic data, coupled with the characteristic genetic diversity and rapid evolution of NoV has currently restricted vaccine development. This review analyzed the molecular epidemiology of NoV in China over the past 20 years in order to provide a sound reference for vaccine development.
Methods
Methods for Epidemiology of NoV in China
Search Strategy: The China National Knowledge Infrastructure (CNKI) database was searched up to 31 October 2020 with the following search terms “norovirus,” “calicivirus” or “norwalk virus” in combination with gastroenteritis or diarrhea.
Inclusion Criteria: Chinese mainland-based investigations into sporadic gastroenteritis/diarrheal diseases, which included spatial distribution and age information. Inclusion criteria for the review were study duration of at least one calendar year, population size of >100 and diagnosis by RT-PCR or ELISA. Publications in both English and Chinese were considered.
Data Extraction: The following information was extracted from each included study: study district; study duration; age group; number of cases screened; number of cases positive for the NoV; Peak NoV detection; genotype information; number of NoV GI, GII, and GIV detections.
Statistical Analysis: The web based open source statistical calculator OpenEpi version 3 (https://www.openepi.com) was used to calculate the 95% confidence intervals for proportions.Citation9
NoV GII.2 VP1 sequence and phylogenetic tree construction.
To define why recombinant GII.P16-GII.2 caused pandemic outbreaks, the sequences from GenBank were downloaded and MEGA5.05 software employed to analyze the evolution of the VP1 region.
Results
Epidemiology of NoV in China
NoV prevalence and age distribution
NoV infection is very common, both in gastroenteritis patients and healthy individuals.Citation10,Citation11 The prevalence of NoV infection in symptomatic and asymptomatic individuals of 20% and 7% globally.Citation2 The NoV prevalence in asymptomatic patients was 9.7% in Africa.Citation12 Surprisingly, NoV was up to 29.8% detected in Mexican Children, with 49.2% having at least one positive stool from June to August 1998.Citation13 Asymptomatic individuals might be act as reservoirs, facilitating the transmission of the NoV. Considering whether NoV was shed from a recent symptomatic NoV gastroenteritis or whether the subjects were experiencing primary asymptomatic infections is difficult to determine, it’s plausible that the results of studies on asymptomatic infections are controversial. Given that relatively few past studies focused on NoV infections without symptomatic disease in China, asymptomatic infections were excluded in our research.
The mean overall NoV prevalence in individuals with sporadic diarrhea/gastroenteritis from 52 studies originating from 28 provinces () was 16.68% (20796/124649, 95% CI 16.63–16.72).Citation14–65 Data were stratified by age into three categories: children, hospitalized young children ≤5 years and mixed (studies that did not report age stratified). Prevalence was significant difference in patients with diarrhea/gastroenteritis children 24.00% (5889/24537, 95% CI 23.92–24.08), hospitalized young children ≤5 years 17.39% (3801/21852, 95% CI 17.32–17.47) and mixed 13.26% (8776/66177, 95% CI 13.21–13.31). Of note, A 6-year study showed that NoV detection among adult patients with infectious diarrhea reached 19.28% (2330/12083, 95% CI 19.23–19.33), this data far exceeds the mixed population.Citation65 Considering the samples were only taken from Shanghai might introduce bias assessment, the spatial distribution of NoV infection were summarized (). In general, the spatial distribution of infection favors southern coastal regions, such as Yellow Sea (Jiangsu), East China Sea (Shanghai and Fujian), North China Sea (Guangxi), which were severely hit by NoV infection. Interestingly, inland areas concentrated in Southwest China, notably Chongqing and Sichuan, were extremely eye-catching. Studies in Guizhou excluded from our research revealed NoV infection also especially serious.Citation66,Citation67 TaiwanCitation68,Citation69 and Hong KongCitation7,Citation70,Citation71 were reported to experience severe NoV burdens, but data related to detection rates in sporadic diarrhea was absent. In July 2017, the CDC in Jiangxi announced that NoV was found in food-borne diarrhea cases in Ji’an, which is the second discovery of NoV in Ji’an and the first reported in food-borne sporadic diarrhea cases. Possibly due to poor surveillance, no NoV infection cases have been reported in areas such as Macau and Tibet. Several limitations of our study need to be addressed. NoV infection emerges obvious geographical distribution, causing the data 19.28% drawn from Shanghai adult patients could thus for be higher than the national infection rates. Judging from the number of studies, coastal cities were included more than inland, which might introduce prevalence bias across all age groups. The prevalence was higher in children and young children ≤5 years were more likely to be hospitalized, which correlates with studies from Taiwan and Hong Kong.Citation68,Citation70 Moreover, NoV seasonality is obvious in China, where great majority NoV disease peaks from October to March of the following year.
Table 1. The detection of NoV in sporadic AGE/diarrhea cases. The detection of NoV in sporadic gastroenteritis/diarrhea cases
Figure 1. Heat map of unique datasets from each province. The study references are as follow:Chongqing,Citation17 Shaanxi,Citation32,Citation45 Sichuan,Citation36 Fujian,Citation21,Citation36 Shanghai-,Citation20,Citation26,Citation36,Citation39,Citation65 Jiangsu,Citation18,Citation19,Citation36,Citation61 Guangxi,Citation36,Citation37,Citation42 Qinghai,Citation29,Citation41 InnerMongolia,Citation36 Beijing,Citation15,Citation36,Citation55 Guangdong,Citation14,Citation23,Citation25,Citation36,Citation43,Citation48 Hubei,Citation40 Shandong,Citation36 Gansu,Citation33,Citation36 Tianjin,Citation16,Citation24,Citation60 Anhui,Citation36,Citation47 Jilin,Citation34–36 Ningxia,Citation22,Citation46 Xinjiang,Citation36,Citation44 Zhejiang,Citation27,Citation38,Citation49,Citation51,Citation54,Citation58 Shanxi,Citation36,Citation57 Hebei,Citation36,Citation62 Yunnan,Citation36 Heilongjiang,Citation36,Citation59,Citation63 Hainan,Citation50,Citation53 Hunan,Citation28,Citation36 Liaoning,Citation52,Citation56 Henan.Citation36
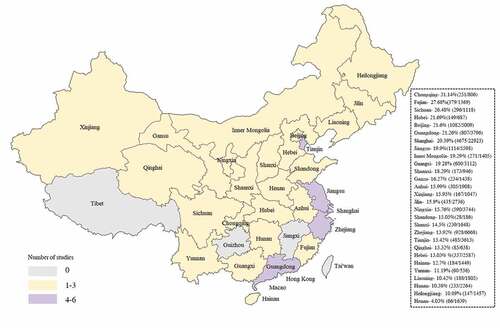
NoV genotype diversity
A total of 13 studies reporting the predominant infection-causing NoV genotypes in different time periods and locationsCitation20,Citation31,Citation36,Citation40,Citation60,Citation72–79 were analyzed to derive an epidemiologic summary of NoV strains in China (). Before 2014, more than half of NoV infections were caused by GII.4, and it was still the dominant strains in some regions until 2015, while decreased significantly after 2016. In fact, since the fall of 2014, non-GII.4 strains replaced GII.4 as the predominant cause in outbreaks of NoV gastroenteritis. In the winter of 2014–2015, GII.17 was involved in multiple epidemics.Citation80–83 Following this, NoV GII.2 became the circulation strain in many regions of China since 2016. Comprehensive epidemiological data confirms that GII.6 has shown sustained and widespread transmission in the last 20 years, although it did not yet cause large-scale outbreaks like GII.4, GII.17 and GII.2.
Figure 2. The predominant genotype of NoV in 1999–2019.Citation20,Citation31,Citation36,Citation40,Citation60,Citation72–79
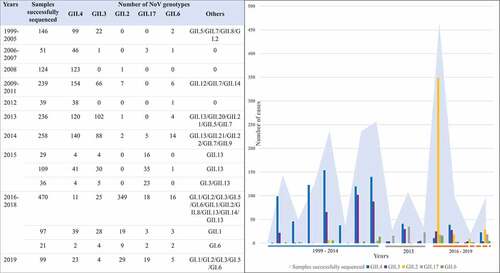
In order to accurately summarize the diversity of NoV genotypes, capsid-based genotypes detected from 28 studies were analyzed to determine the prevalent and widely distributed NoV genotypes in two time periods ().Citation14,Citation17,Citation19–21,Citation23,Citation25,Citation31,Citation32,Citation34,Citation35,Citation40,Citation46,Citation55,Citation58,Citation59,Citation61,Citation62,Citation77,Citation79,Citation84–91 It was verified that GII.4 was the most frequently detected, accounting for nearly 70%, subsequently preceded by NoV GII.3, GII.6 and GII.12 prior to 2014. However, GII.2 replaced GII.4 as the most predominant (accounting for more than 50%), and GII.17 was the next most widely distributed genotypes (accounting for 15.38%) from 2014 to 2019. Moreover, GI genogroup has shown an obvious upward trend after 2014, accounting for nearly 5%, of which GI.2, GI.3, especially GI.6 have demonstrated an absolute advantage. It is worth noting that GI.1 was not detected in the studies included between 2014 and 2019. Ongoing surveillance should be needed to determine the evolution of the epidemic strains across regions and time in China.
Figure 3. (a) The Proportion of NoV Genotypes from 1999 to 2014 (1250 cases from 15 studies);Citation14,Citation17,Citation19–21,Citation23,Citation25,Citation31,Citation32,Citation34,Citation35,Citation55,Citation84–86 (b)The Proportion of NoV Genotypes from 2014 to 2019 (1697 cases from 13 studies).Citation40,Citation46,Citation58,Citation59,Citation61,Citation62,Citation77,Citation79,Citation87–91
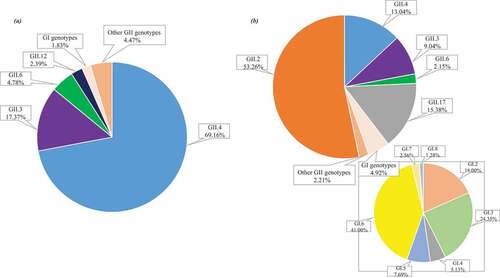
The National Public Health Emergency Management Information System (PHEMIS) reported 56 NoV outbreaks nationwide from 2006 to 2013.Citation92 However, 915 NoV outbreaks involved in 26 provinces from 2014 to 2018 were reported by PHEMIS, and CaliciNet China reported 556 NoV outbreaks from October 2016 to September 2018 in 6 provinces.Citation77,Citation93 NoV outbreaks increase when new strains circulate in the community, and the surge of NoV outbreaks after 2016 was mainly attributed to the recombinant strain GII.P16-GII.2. A logical follow-up question concerns why GII.P16-GII.2 caused such large-scale outbreaks?
Molecular evolution of reemerging NoV GII.P16-GII.2
Using NCBI database, we downloaded 304 GII.2 VP1 capsid sequences worldwide from 1971 to 2019. Overall, The VP1 sequences were 96.57% AA identical to each other and most of the sequences were from Japan and China. The key AAs Asn352/Arg353/Asp382 of NoV GII.2 HBGA binding sites in the P2 subdomain are highly conserved (), this result correlates with the statement reported by National institute for viral disease control and prevention, China CDC.Citation94 Of note, GII.2 experienced limited evolutions after 2016 and mutations only appeared in a few sequences.
Figure 4. Summary of mutations in the P2 subdomain of GII.2 strains from 1970–2019 (Strains without mutations were not involved). Red letters denote concentrated mutations that have appeared in multiple sequences. GenBank accession numbers: ASO96884, AGI17590, AFN06726, AFX71659, AGT62523.

Further, we screened out 62 sequences from 304 to construct evolutionary tree by minimum evolution method to analyze the full-length sequence evolution of GII.2 VP1 region (). The VP1 gene phylogenetic tree could be grouped into two major clusters: the GII.2 strains from 1970s (Group I) and 2004–2019 (Group II). Sequence analysis revealed significant time and geographical characteristics, e.g. sequences from 2008 to 2014 in the GroupII.1 formed a phylogenetic cluster concentrated in Japan, while the sequences (GroupII.2) from 2016 to 2019 were concentrated in China. Collectively this reveals that mutations in GII.2 VP1 sequence stably accumulate in human populations. Of note, one strain isolated from 2008 (Accession Number: BBB87008) recognized as GII.P2-GII.2 was closely related to the lineage of the GII.P16–GII.2 strain after 2016 in GroupII.2. Further research by Ao.et al revealed that the GII.P2–GII.2 strain emerged in 2016 harbors a capsid sequence identical to the GII.P16–GII.2 reemerged in 2016–2017 in Fengtai.Citation95 Phylogenetic and sequence analyses indicated that GII.P16-GII.2 had no remarkable change on capsid protein compared with GII.P2–GII.2, implying that factors other than capsid proteins contribute to the GII.P16–GII.2 virus epidemic.Citation96
Figure 5. Phylogenetic analysis of GII.2 full length VP1 sequences. Minimum evolution method phylogenetic inference of VP1 aa sequences was performed. Statistical evaluation was performed by 1,000 bootstrap replications and percentages of clustering (>50%) are shown at nodes. Scale bars indicate the number of substitutions per site. Country/region abbreviations: CN, China (circles); JP, Japan (squares); US, United States (triangles); MY, Malaysia (diamonds); BJ, Beijing; TW, Taiwan; HK, Hong Kong; TZ, Taizhou; ZS, Zhoushan.
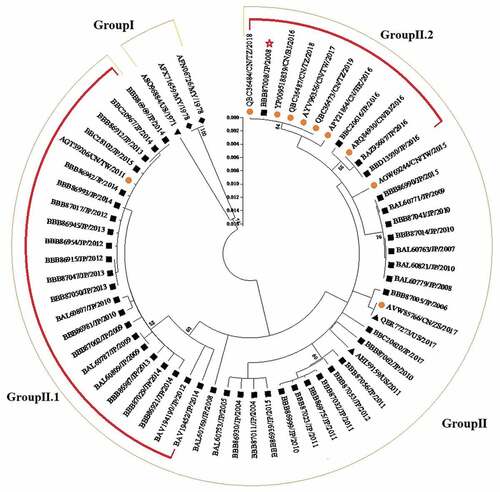
It was reported that GII.P2-GII.2 was only sporadically detected in Japan before 2009. However, NoV GII.2 associated outbreaks were observed in Japan during 2009–2010 and the frequency of GII.2 in individual cases and outbreaks was close to that of GII.4. Genetic analyses revealed the GII.2 epidemic resulted from GII.P2-GII.2 and GII.P16-GII.2. It is worth noting that this likely represents the first epidemic of GII.P16-GII.2 worldwide.Citation97 GII.P16-GII.4 increased in Australia and New Zealand in 2017, and it has been verified the polymerase of GII.P16-GII.4 was derived from the GII.P16-GII.2 virus which was dominant at the end of 2016.Citation98 The polymerase of GII.P16 is more adaptable than others, and it has been hypothesized that outbreaks caused by GII.P16-GII.2 and GII.P16-GII.4 are mainly due to the polymerase GII.P16 and slight change of capsid genotypes may also have contributed to immune escape and conferred higher virological fitness.
Typically, various genotypes of NoV circulated in human populations during the same period, providing opportunities for recombination between different strains. We assumed that multiple viruses are transcribed simultaneously in a single cell of an infected host and template switching occurs during the transcription process, and after optimized selection, the recombinant strain is finally formed. Most scholars endorse the sub-genomic RNA NoV gene recombination theory: the promoter site located in the overlapping region of RdRp/Capsid, and the sequence between the 5ʹ end of the NoV genome and the ORF1/ORF2 overlapping region is highly consistent.Citation99–102 According to the above theory, underlying theoretical recombination mechanism of GII.P16-GII.2 is demonstrated in .Citation103,Citation104
Figure 6. The hypothesis of recombination GII.P16-GII.2: GII.P16-GII.X positive-sense RNA as a template to synthesize negative-sense RNA. This negative-sense RNA and its internal subgenomic RNA are used as templates to synthesize positive-sense genomic RNA and subgenomic RNA respectively. These two positive-sense RNAs serve as template to synthetic negative-sense genomic RNA and subgenomic RNA. Recombination occurs during the process of synthesizing GII.P16-GII.X positive-sense RNA and subgenomic RNA. The RdRp region of the positive-sense RNA initiates synthesis at the promoter (blue triangle) located at the 3′end of negative-sense RNA. However, the process stalls at the ORF1/ORF2 overlap region subgenomic promoter (red triangle) and then switches template to GII.PX-GII.2 negative-sense subgenomic RNA to continue transcription and finally the recombinant strain GII.P16-GII.2 formed.
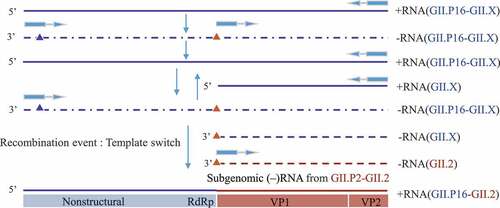
Progress toward NoV vaccines
Simulation studies have shown that 100–6125 NoV AGE cases could been prevented per 10 thousand individuals vaccinated.Citation105 Unfortunately, NoV vaccination has not yet been given permission globally. Live attenuated and inactivated NoV particle vaccines cannot been created due to the prior lack of an in vitro culture system for human NoV. Candidate vaccines in the research pipeline predominately target P particle (PP) and virus-like particle (VLP).
The PP protein occurs as several oligomers, including 2-mer, 12-mer and 24-mer. The subunit vaccines based on PP have been presented the characteristics of high immunogenicity, easily expressed and low cost. The surface of PP protein has three ring structures which are suitable for inserting and displaying foreign epitopes on the surface of the particle. Using this approach, NoV PP have been used to develop vaccines with enhanced antigen immunogenicity using epitopes from rotavirus, HIV, influenza, and EV71. For example, Tan et al.Citation106 chimerized PP with rotavirus VP8 antigen, which not only blocked the binding of NoV VLPs to HBGAs receptors but also produced higher levels of neutralizing antibodies against Rv infection compared with free VP8 antigen. Furthermore, the trivalent subunit vaccine (AstV P-HEV P-NoV P) induced higher levels of antibody response after intranasal immunization of mice compared to the three mixed vaccines.Citation107 PP can be easily produced in Escherichia coli and preclinical data based on GII.4 PP have been sprung up in recent yearsCitation108–114 (). Although PP vaccine ease of particle production at scale offers a potential cost advantage, it has not yet progressed to trials in humans until now.
Table 2. Human NoV vaccine candidates in preclinical trials
Notwithstanding these results, the extensive antigenic diversity between different NoV genotypes affords only weak cross-protection, leading many researchers to focus on the development of multivalent VLP vaccines containing major epidemic genotypes. The antigenicity and receptor binding characteristics of VLPs are similar to those of PPs. However, VLPs using VP1 as antigen are more advantageous than PPs in inducing the production of high-level, high-affinity NoV-specific IgG antibodies, balancing Th1 and Th2 immune responses, and cross-immune protection of different NoV genotypes.Citation123 The technical platforms for preparing NoV VLP vaccines vary widely and include expression systems involving adenovirus, E. coli (bacteria), baculovirus (insect), Pichia/Hansenula (yeast) as well as expression in transgenic plants, and some of which have entered the clinical research phase (). The bivalent GI.1/GII.4 VLP vaccine designed by Takeda Pharmaceutical Company Limited has now entered phase II clinical stage, and Clinical studies have shown that this vaccine have good safety and immunogenicity, and it may induce the production of broadly neutralizing antibodies against new genotypes and heterologous strains. The recombinant NoV bivalent (GI.1/GII.4) vaccine (Hansenula) developed by the domestic Lanzhou Institute of Biological Products Co., Ltd. and the tetravalent (GI.1+ GII.3+ GII.4+ GII.17) recombinant NoV vaccine (Pichia pastoris) jointly developed by Shanghai Pasteur and Zhifei Biopharmaceutical Co., Ltd. have been approved by the National Medical Products Administration (NMPA) Clinical Trial License. Notably, the tetravalent recombinant NoV vaccine has applicability to the most NoV genotypes and has obtained clinical approval worldwide. With the emergence of non-GII.4 epidemic strains, more multivalent VLP vaccines are being assessed in preclinical studiesCitation115–119(). In addition, the incorporation of rotavirus VP6 nanotubes as a delivery vehicle for NoV VLPs represents a unique feature, and this multivalent vaccine can induce protective immune responses to the vast majority of circulating NoV and RV genotypesCitation120–122 ().
Table 3. Human NoV vaccine candidates in clinical trials
Discussion
Economic development has accelerated population movement and provided opportunities for NoV infections to spread nationwide, e.g., the widespread distribution of contaminated frozen seafood. Fruit or vegetables irrigated with contaminated water can also act as a source of NoV infections. People of all ages can be infected with NoV, children are especially at risk and those under 5 year of age experience high hospitalization rates. In addition to coastal areas, NoV infections have been quite serious in Southwest China, which may be due to dietary habits and a lack of awareness of NoV prevention and control. In view of the increasingly serious NoV infection in China, epidemic surveillance needs to be strengthened urgently. In 2016, CaliciNet China started with 9 laboratories in 3 provinces and by 2018 their efforts increased to 17 laboratories in 6 provinces to monitor the epidemiology of NoV.Citation77 While the monitoring area of CaliciNet China continues to expand, we will have a comprehensive understanding of China’s NoV outbreaks in the future to better respond to infectious diarrhea outbreaks and targeting control and prevention efforts for risk reduction.
The structure of NoV RNA is unstable, this instability lead to the formation of new strains through the recombination between different NoV strains and antigenic drifts, triggering outbreaks, and escaping immunity. High viral load and faster reproduction speed promoted the outbreak of GII.P16-GII.2 and molecular evolution analysis has demonstrated that RdRp rather than VP1 sequence triggers pandemic.Citation71 The widespread prevalence of GII.4 generates new variant strains through point mutations in the P2 region of the VP1 and accumulation of homologous recombination between different genotypes, showing genetic diversification and triggering pandemic! Since 1995, there have been at least 6 epidemic strains of GII.4, namely US 1995/96, Farmington Hills 2002, Hunter 2004, Den Haag 2006b/Yerseke_2006a, New Orleans 2009 and Sydney 2012. The study found GII.4 Sydney_2012 strain tends to undergo stable mutations during the asymptomatic shedding phase and may generate new variants in chronic patients via rapid evolution with stable mutations, so the evolution of GII.4 strain is more inclined to accumulated over time and co-evolution at the genome level.Citation124,Citation125 Evolution analysis shows that GII.17 evolves faster than GII.4 viral protein (VP1) and is more likely to result in immune evasion. The rate of nucleotide substitution in the P2 region of the VP1 capsid protein of the GII.17 strain has averaged 1.2 × 10−2 since 1978, but its evolution rate has accelerated to 3 × 10−2 after 2014.Citation126 Why has the evolution rate of GII.17 accelerated after 2014? It may be that recombination has resulted in obvious nucleotide substitution in the VP1 region of GII.17 and accelerated evolution, which in turn led to changes in its receptor recognition site and epitope, giving GII.17 rapid spread and strong infectivity. Whether GII.2 and GII.17 will be sustained pandemic like GII.4, still needs monitoring and further confirmation.
Either from China or global perspective, GII.6 deserves attention. According to the Centers for Disease Control and Prevention (CDC) in United States, 7% outbreaks were caused by GII.P7-GII.6 from September 1, 2019 to August 31, 2020. It was reported the proportion of GII.6 strains in non-GII.4 strains was second only to GII.3 in developing countries from 1997 to 2018.Citation9 In China, GII.6 followed by GII.3, was the second prevalent strain among non-GII.4 genotypes, accounting for 6% before 2014. Although data showed the proportion of GII.6 had declined in recent years, it still played a prominent role in gastroenteritis outbreak, e.g., CaliciNet China epidemiological surveillance showed 13 of the 16 outbreaks were caused by GII.6 in the winter between 2017 and 2018.Citation77,Citation127 GI genotypes should be strengthened in routine surveillance. Although the main epidemic strains causing NoV infection were mainly GII genotypes, GI genotypes detected have increased significantly in recent years, e.g., GI strains accounted for 12.5% and more than 16.67% of patients with AGE in Heilongjiang Province and Fushun City Liaoning Province from 2017 to 2019.Citation59,Citation128
Immunoassay research based on VLP and PP vaccine candidates helps to understand the immune response of NoV to the host. The findings from in clinical and preclinical studies have suggested that VLP and PP are promising vaccines and multivalent vaccination might be an effective strategy for inducing broadly neutralizing antibodies protective against challenge with novel and heterologous NoV strains. The uncertainty of NoV evolution brings great risks to long-term vaccine development. Due to the lack of simple and standardized animal models and effective tissue culture systems in vitro that can be applied to vaccine evaluation, HBGA in vitro blocking test is usually evaluated for the protective effect of NoV vaccine by laboratories. The HBGA receptor binding domain of VP1 was relatively conservative, and the antibodies produced by infected persons with the same genotype can cross-protect different mutant strains. Dai et al.Citation129 in 2013 conducted genetic analysis on GII.4 strains from 1974 to 2012, and found that the HBGA receptor blocking experiment produced antibodies from GII.4 in infected volunteers which can cross-protect 14 different GII.4 strains. Swanstrom et al. in 2014 analyzed the GII.2 sequence from 1976 to 2010 and found that serum infected with GII.2.1976 can block GII.2.2002, GII.2.2008 and GII.2.2010.Citation130 Most researchers agree that cross-immunity exists only possible within the same genogroup.Citation115–118 However, evidence confirms that cross-immunity can exist between genogroups (GI and GII). Czakó et al. in 2015 demonstrated that following GI.1 infection, cross-blocking antibodies against GII.4 were also produced.Citation117 Although results were not consistent, molecular evolution mechanism have shown that the key residues bind to HBGAs between GI and GII genogroups are highly conserved,Citation131 which provides a theoretical basis for the development of a vaccine that induces broad-spectrum cross-protective antibody immunity against multiple NoV genotypes. Whether multivalent vaccines under development can meet the needs of preventing strains not included in the vaccine and whether the breadth and stability of the vaccine response are sufficient to cope with the diversity and cyclical changes of NoV genotypes remains to be verified. Currently, NoV vaccine development aims to expand the protective efficacy by increasing exposure to genotypes. Candidate vaccines under research prioritize GII.4 and GI.1 strains. Although GII.4 has been supplanted by the GII.2 and GII.17 genotypes for some time in China, it remained prevalent strain globally. In contrast, GI.1 rarely caused an epidemic and GII.6 has a continuous and extensive epidemic capacity on a global scale based on comprehensive global epidemiologic data. Of note, prior work has showed that recombinant strain carrying GII.P16 RdRp genotype like GII.2 and GII.4 have caused pandemics, whether GII.P16 combination with other capsid genotypes would cause pandemic remains to be monitored continual. Molecular epidemiologic surveillance for identifying promptly the emergence of NoV variants is highly significant to the development of multivalent NoV vaccines with broad protective efficacy. During the process of vaccine development, it is necessary to make adjustments according to the change of the predominant strains.
Conclusions
In China, there is very little data on NoV infections in asymptomatic individuals. The frequency and severity of NoV infections was surprisingly serious in gastroenteritis patients, notably children. Because of the rapid evolution, non-GII.4 strains have become the predominant strains and caused multiple outbreaks since 2014. Immunization is one of the most effective strategies to prevent infectious disease, but genetic variability remains the major obstacle for the development of effective vaccines against NoV. Successfully developing a vaccine that can broadly induce neutralizing antibodies protective against novel and heterologous strains has being an international problem and also the key problem to be addressed in future work. Presently, however, the selection of antigen components remains an important factor in evaluating vaccine efficacy. Nonetheless, much uncertainty exists when predicting future NoV predominant strains, and ongoing vaccine development efforts must be ready to face this obstacle. Thankfully, NoV epidemic surveillance can be a tool to guide vaccine development. Disease outbreaks can be effectively prevented and controlled by cutting off the route of transmission at the earliest possible stage, and the best way is to strengthen the public’s awareness of self-protection before vaccination available.
Author contributions
Formal analysis, Na Wei; Investigation, Na Wei; Original draft preparation, Na Wei; Manuscript critical revisions, Jun Ge, Changyao Tan, Yunlong Song, Mengru Bao and Jianqiang Li. All authors have read and agreed to the published version of the manuscript.
Disclosure of potential conflicts of interest
The authors declare no conflict of interest for this paper.
Additional information
Funding
References
- Hemming M, Räsänen S, Huhti L, Paloniemi M, Salminen M, Vesikari T. Major reduction of rotavirus, but not norovirus, gastroenteritis in children seen in hospital after the introduction of RotaTeq vaccine into the National Immunization Programme in Finland. Eur J Pediatr. 2013;172:739–46. doi:10.1007/s00431-013-1945-3.
- Ahmed SM, Hall AJ, Robinson AE, Verhoef L, Premkumar P, Parashar UD, Koopmans M, Lopman BA. Global prevalence of norovirus in cases of gastroenteritis: a systematic review and meta-analysis. Lancet Infect Dis. 2014;14:725–30. doi:10.1016/S473-3099(14)70767-4.
- Pires SM, Fischer-Walker CL, Lanata CF, Brecht D, Hall AJ, Kirk MD, Duarte ASR, Black RE, Angulo FJ. Aetiology-specific estimates of the global and regional incidence and mortality of diarrhoeal diseases commonly transmitted through food. Plos One. 2015;10:e0142927. doi:10.1371/journal.pone.0142927.
- GBD 2016 Diarrhoeal Disease Collaborators. Estimates of the global, regional, and national morbidity, mortality, and aetiologies of diarrhoea in 195 countries: a systematic analysis for the Global Burden of Disease Study 2016. Lancet Infect Dis. 2018;18:1211–28. doi:10.1016/S473-3099(18)30362-1.
- Bartsch SM, Lopman BA, Sachiko O, Hall AJ, Lee BY. Global economic burden of Norovirus gastroenteritis. Plos One. 2016;11:e0151219. doi:10.1371/journal.pone.0151219.
- Todd K, Tripp R. Human Norovirus: experimental models of infection. Viruses. 2019;11:151. doi:10.3390/v11020151.
- Chi-Wai CM, Kirsty K, Lin-Yao Z, Mohammad KN, Nelson L, Lui GCY, Nelson EAS, Lai RWM, Leung TF, Chan PKS, et al. Bimodal seasonality and alternating predominance of Norovirus GII.4 and Non-GII.4, Hong Kong, China, 2014–2017. Emerg Infect Dis. 2018;24:767–69. doi:10.3201/eid2404.171791.
- Vinjé J, Doern GV. Advances in laboratory methods for detection and typing of Norovirus. J Clin Microbiol. 2015;53:373–81. doi:10.1128/JCM.01535-14.
- Mans J. Norovirus infections and disease in Lower-MiddleandLow-Income Countries, 1997-2018. Viruses. 2019;11:341. doi:10.3390/v11040341.
- Phillips G, Tam CC, Rodrigues LC, Lopman B. Risk factors for symptomatic and asymptomatic norovirus infection in the community. Epidemiol Infect. 2011;139:1676–86. doi:10.1017/S0950268810002839.
- Phillips G, Tam CC, Conti S, Rodrigues LC, Brown D, Iturriza-Gomara M, Gray J, Lopman B. Community incidence of norovirus-associated infectious intestinal disease in England: improved estimates using viral load for norovirus diagnosis. Am J Epidemiol. 2010;171:1014–22. doi:10.93/aje/kwq021.
- Mans J, Armah GE, Steele AD, Taylor MB. Norovirus Epidemiology in Africa: a Review. PLoS One. 2016;11:e0146280. doi:10.1371/journal.pone.0146280.
- Garcı´a C, DuPont HL, Long KZ, Santos JI, Ko G. Asymptomatic norovirus infection in Mexican children. J Clin Microbiol. 2006;44:2997–3000. doi:10.1128/JCM.00065-06.
- Lin YX, Wei QD, Feng R, Zang LR. Genotypes of norovirus in Zhuhai region, 2011-2013. Pract Prev Med. 2017;24:364–68. (in Chinese). doi:10.3969/j.1006-3110.2017.03.032.
- Cao YN, Zhang XJ, Chen N. Epidemiological surveillance of norovirus and rotavirus in children with diarrhea. J Pub Health Prev Med. 2020;31:42–44. (in Chinese).
- Fang YL, Wang W, Wang P, Zhao Y, Zhang TQ, Li X, et al. Molecular epidemiology of norovirus in children with acute gastroenteritis in Tianjin, China. Chin J Contemp Pediatr. 2019;21:139–43. (in Chinese). doi:10.7499/j.1008-8830.2019.02.006.
- Liao Y, Chen JH, Liu ZY, Peng Z, et al. The characterization of norovirus genotypes in children in Chongqing. J Chongqing Med Univ. 2011;36:242–45. (in Chinese). doi:10.13406/j.cnki.cyxb.2011.02.001.
- Fu JG, Ai J, Zhang J, Wu QB, Qi X. Molecular epidemiology of genogroup II norovirus infection among hospitalized children with acute gastroenteritis in Suzhou (Jiangsu, China) from 2010 to 2013. J Med Virol. 2015;88:954–60. doi:10.1002/jmv.24429.
- Fu JG, Ai J, Qi X, Zhang J, Tang FY, Zhu YF. Emergence of two novel norovirus genotype II.4 variants associated with viral gastroenteritis in China. J Med Virol. 2014;86:1226–34. doi:10.002/jmv.23799.
- Yao WL, Cai JH, Wang XS, Ge YL, Zhu QR, Zeng M. Surveillance of norovirus-associated diarrhea in children in Shanghai, 2009-2011. Chin J Pediatr. 2014;52:339–44. doi:10.3760/cma.j.0578-1310.2014.05.005.
- Chen ZH, Huang GL, Chen XL. Molecular epidemiological study on norovirus in infants with diarrhea in Xiamen. Maternal Child Health Care China. 2012;27:5312–14. (in Chinese).
- Zhang Y, Chen Y, Liu Z, Zhang Y. Acute diarchea in children in Yinchuan: etiology and related factors. World Chin J Digestol. 2015;23:2975–80. (in Chinese). doi:10.11569/wcjd.v23.i18.2975.
- Shen JC, Liu YF, Xie HP. Genotyping study of Novovirus infection in clinic children under 5 years in Guangzhou. J Med Pest Control. 2010;26:393–95. (in Chinese). doi:10.3969/j.1003-6245.2010.05.001.
- Chen J, Li JM, Zhang Y, Liu H, Gao L, Xu WT, et al. The analysis of disease burden of viral diarrhea cases in Tianjin during 2008-2009. J Trop Med. 2012;12:87–90. (in Chinese).
- Tang YL, Dai YC, Nie J, Li JD, Huang BM. Epidemiology and genotype analysis of norovirus infection in children with acute diarrhea in Jiangmen. J Pract Med. 2011;27:2841–43. (in Chinese). doi:10.3969/j.1006-5725.2011.15.061.
- Lu L, Zhong H, Xu M, Su L, Xu J. Genetic diversity and epidemiology of Genogroup II noroviruses in children with acute sporadic gastroenteritis in Shanghai, China, 2012–2017. BMC Infect Dis. 2019;19:736. doi:10.1186/s12879-019-4360-1.
- Jin SY, Lin J, Xu XJ, Zhong DM. Genotypes and molecular characteristics of Norovirus from children with acute diarrhea in Hangzhou area from 2010 to 2011. Chin J Clin Lab Sci. 2012;30:924–26. (in Chinese). doi:10.13602/j.cnki.jcls.2012.11.028.
- Chen Y, Zhou SF, Sun QL, Zhang F, Liang C, Zhang H, et al. Infection and genotypes of norovirus among children with acute diarrhea in sentinel hospitals in Hunan Province, 2012-2014. Pract Prev Med. 2016;23:773–76. (in Chinese). doi:10.3969/j.1006-3110.2016.07.002.
- Yuan L, Pan XP, Liu GX, Yang WC. Analysis of viral diarrhea in children under 5 years old in Xining from 2015 to 2016. J Med Pest Control. 2018;34:147–50. (in Chinese). doi:10.7629/yxdwfz201802013.
- Zeng M, Chen J, Gong S, Xu X, Zhu C, Zhu Q. Epidemiological surveillance of norovirus and rotavirus diarrhea among outpatient children in five metropolitan cities. Chin J Pediatr. 2010;48:564–70. (in Chinese). doi:10.3760/cma.J.0578-1310.2010.08.002.
- Fang ZY, Xie HP, Lv HX, Zhang Q, Jiang X. Investigation of human calicivirus (HuCV) diarrhea among infantile and young children in China, 1999-2005. Chin J Virol. 2007;23:9–15. doi:10.1631/jzus.2007.B0900.
- Liu P, Wang X, Lee JC, Teunis P, Hu S. Genetic susceptibility to norovirus GII.3 and GII.4 infections in Chinese pediatric diarrheal disease. Pediatr Infect Dis J. 2014;33:305–09. doi:10.1097/INF.0000000000000443.
- Yan YX, Zhou YK, Zhang HF, Wang F, Jin M, Zhang Q, et al. Molecular epidemiology of norovirus infection among hospitalized children under 5 years with diarrhea in Lanzhou, 2015-2018. Int J Virol. 2020;027:53–56. (in Chinese). doi:10.3760/cma.j.1673-4092.2020.01.013.
- Li X, Yang XD, Xu S, Wu DL, Sun LW, Shen B, et al. Detection of Norovirus genotype GII.4 Sydney 2012 variant from children under 5 years of age in Jilin Province, 2012-2013. Chin J Vaccines Immunization. 2015;21:283–88. (in Chinese).
- Li X, Yang XD, Xu S, Wu DL, Sun LW, Lu X, et al. Epidemiological characteristics and genotype of Norovirus in Infants under 5 years old in Jilin Province in 2011. Chin J Vaccines Immunization. 2013;19:431–34. (in Chinese).
- Kong XY. Molecular epidemiology of norovirus in some areas of China from 2011 to 2014. Chinese Center for Disease Control and Prevention; 2015. (in chinese).
- Tan DM, Liu W, Deng LL. Molecular epidemiology of norovirus infection among sporadic cases of acute gastroenteritis in Nanning city. Chin J Public Health. 2011;27:1372–75. (in Chinese). doi:10.111/j.600-0714.2011.01024.x.
- Chen LP, Ji L, Shen YH, Xu DS. Epidemic characteristics of norovirus in Huzhou during 2015-2017. Chin J Health Lab Tec. 2020;30:666–8+72. (in Chinese).
- Xue CY, Pan LF, Zhu WP, Wang YP, Fu HQ, Cui CC, Lu L, Qiao S, Xu B. Molecular epidemiology of genogroup II norovirus infections in acute gastroenteritis patients during 2014-2016 in Pudong New Area, Shanghai, China. Gut Pathog. 2018;10:7. doi:10.1186/s13099-018-0233-1.
- Li J, Zhang T, Xu JQ, Zhan JB, Guan XH, Xing XS, et al. Epidemic characteristics and genotypes of norovirus in Hubei Province, 2015. Chin J Dis Control Prev. 2017;349–52+61. (in Chinese). doi:10.16462/j.cnki.zhjbkz.2017.04.007.
- Tian D, Liu GX, Qiong XU, Zhao SC, Zhang HY, Feng YL, et al. Analysis of the prevalence of norovirus genotypes in Qinghai Province from 2016 to 2017. J Pathogen Biol. 2018;13:1258–60. (in Chinese). doi:10.13350/j.cjpb.181117.
- Nong H, Liu HH, Yang C, Yin LJ, Zhan XJ, Qin JQ. Results of norovirus detection of some diarrhea specimens in Nanning City, Guangxi Province. Mod Preventive Med. 2020;47:488–91. (in Chinese).
- Zeng J, Wang Y, Bai P, Li R. Molecular epidemiology of Norovirus infection in Conghua, Guangzhou in 2011. Guang Dong Med J. 2013;34:549–51. (in Chinese). doi:10.3969/j.1001-9448.2013.04.017.
- Shabiremu T, Xiaokelaiti N, Zhou HJ, Jin M, Kong XY, Zhou YK, et al. Molecular epidemiology of norovirus infection in hospitalized children with acute gastroenteritis in Urumqi, Xinjiang,2012-2014. Dis Surveillance. 2018;33:24–28. (in Chinese). doi:10.3784/j.1003-9961.2018.10.006.
- Han YF, Gao J, Wei JJ, Deng T, Zhang JD, Tang WJ, et al. Surveillance results of rotavirus and norovirus diarrhea in Hanzhong, Shaanxi. China Trop Med. 2020;20:731–42. (in Chinese). doi:10.13604/j.cnki.46-1064/r.2020.08.08.
- Dang W. Study on the pathogenic spectrum of viral diarrhea and the molecular epidemiology of norovirus in Ningxia from 2016 to 2018. Ningxia Medical University; 2019. (in Chinese).
- Ying H, Shi YL, Kui Z, Jiang LL, Rong W, Li W, et al. Molecular epidemiological analysis of Norovirus in patients with foodborne diseases in sentinel hospital, Ma’anshan City, Anhui Province. Chin J Prev Med. 2019;53:581–85. (in Chinese). doi:10.3760/cma.j.0253-9624.2019.06.008.
- Ren Y, Xu SJ, Zhang HL, Quan DF, Lai XL, Man YX, et al. Laboratory analysis of viral diarrhea pathogens in Longhua district, Shenzhen city from 2015 to 2017. J Trop Med. 2018;18:1346–50. (in Chinese).
- Sun MH, Zhou XH, Xu PH, Xu JY. Monitoring and genotype analysis of the norovirus epidemic strains in Haiyan county in 2013. Chin J Health Lab Tec. 2015;025:2777–79. (in Chinese).
- Shan BY, Li M, Pan JP, Chen N. Analysis of the etiology and epidemiological characteristics of viral diarrhea. J Pathogen Biol. 2018;9:1010–13. (in Chinese). doi:10.13350/j.cjpb.180918.
- Ji L, Zhu YY, Chen LP, Shen YH, Wu XF, Xu DS. Infection status and genotype characteristics of GI norovirus in AGE/diarrhea cases in Huzhou, Zhejiang, 2017-2018. Dis Surveillance. 2019;34:536–40. (in Chinese). doi:10.3784/j.1003-9961.2019.06.014.
- Li JY, Liu YB, Zhao YQ, Liu HS, Dong X. Monitoring results analysis of norovirus infected diarrhea in Shenyang from 2015 to 2017. Chin J Health Lab Tec. 2019;29:1884–5+9. (in Chinese).
- Wang JH, Lin XP. Epidemiological study on viral diarrhea in Haikou City. J Prev Med Chin PLA. 2016;34:320. (in Chinese). doi:10.13704/j.cnki.jyyx.2016.s2.291.
- Chen WP, Cui DW, Yang XZ, Zheng SF, Xie GL, Chen Y. Molecular epidemiological analysis of norovirus in patients with acute gastroenteritis in Zhejiang province during 2016. Chin J Clin Lab Sci. 2018;36:304–08. (in Chinese). doi:10.13602/j.cnki.jcls.2018.04.17.
- Sang S, Zhao Z, Suo J, Xing Y, Jia N, Gao Y, et al. Study on the molecular epidemiological characteristics of norovirus in acute gastroenteritis of Beijing. Chin J Epidemiol. 2013;34:263. doi:10.3760/cma.j.0254-6450.2013.03.014.
- Cai T, Yue LX, Zhang KY. Analysis of Norovirus detection in patients with Diarrhea in Dalian in 2013. Strait J Prev Med. 2015;21:38–39. (in Chinese).
- Xin XY, Li XL, Zhang GH, Guo HF, Li YY. Analysis on epidemiological characteristics of norovirus infectious diarrhea, Taiyuan city,2015-2017. Preventive Med Tribune. 2019;361–64. (in Chinese). doi:10.16406/j.pmt.1672-9153.2019.05.015.
- Chen C. Epidemiology and spatiotemporal dynamic of norovirus associated with sporadic acute gastroenteritis during 2013-2018, Zhoushan Islands, China. Nanchang University; 2019. (in Chinese).
- Shi X, Shu C, Xu J, Leng Y. Analysis on the detection of norovirus and its genetic characteristics among patients with diarrhea in Heilongjiang Province from 2017 to 2019. Chin J PHM. 2020;36:457–9+98. (in Chinese). doi:10.19568/j.cnki.23-1318.2020.04.005.
- Lei Y, Zhuang ZC, Tian H, Li XY. Molecular characteristics of norovirus in acute gastrointestinal illness, Tianjin, 2019. Dis Surveillance. 2020;35:913–19. (in Chinese). doi:10.3784/j.1003-9961.2020.10.011.
- Xu JF, Xu H, Song YS, Xue Y, Shao SH, Shen HX. Molecular epidemiological characteristics and genotype analysis of norovirus in Zhenjiang region. Chin J Clin Lab Sci. 2017;35:857–60. (in Chinese). doi:10.1360/j.cnki.jcls.017.11.14.
- Bai X, Zhang SH, Shen ZX. Analysis of Norovirus genotype in sporadic diarrhea cases in Hebei Province. Int J Virol. 2020;01:44–47. (in Chinese). doi:10.3760/cma.j.1673-4092.2020.01.011.
- Xu J, Shi X, Shu C, Leng Y, Cao B, Wang KL. A study on molecular epidemiology of Norovirus in Heilongjiang province from 2012 to 2015. Chin J PHM. 2016;32:569–72+79. (in Chinese). doi:10.19568/j.cnki.23-1318.2016.05.001.
- Yu J, Lai S, Wang X, Liao Q, Feng L, Ran L, et al. Analysis of epidemiology characteristics of norovirus among diarrheal outpatients in 27 provinces in China, 2009 – 2013. Chin J Epidemiol (Zhonghua Liu Xing Bing Xue Za Zhi). 2015;36:199–204. doi:10.3760/cma.j.0254-6450.2015.03.003.
- Lin S, Pan H, Xiao W, Gong X, Kuang X, Teng Z, et al. Epidemiologic characteristics of Norovirus among adult patients with infectious diarrhea, in Shanghai, 2013-2018. Chin J Epidemiol. 2019;40:883–88. (in Chinese). doi:10.3760/cma.j.0254-6450.2019.08.003.
- Yan Y, Wu Y, Guo J, Tian K, Wang D. Genotype features of Norovirus Associated With Acute Gastroenteritis Through Sentinel Surveillance in Guizhou Province, 2011. Chin J Virol. 2013;29:51–55. doi:10.13242/j.cnki.bingduxuebao.002351.
- Yan Y, Guo J, Jiang WJ, Tian KC, Li SJ, Hu J, et al. A preliminary study on Norovirus variant for GII.4 in Guiyang regions. Chin J Microbiol Immunol. 2012;32:606–09. (in Chinese). doi:10.3760/cma.j.0254-5101.2012.07.008.
- Burke RM, Shu-Man S, Catherine Y, Huang YC, Parashar UD, Lopman BA, et al. Burden of severe Norovirus disease in Taiwan, 2003-2013. Clin Infect Dis. 2018;9. doi:10.1093/cid/ciy298.
- Liu TC, Kuo TY, Wu CY, Liao WT, Hall AJ, Wu FT. Recombinant GII.P16-GII.2 Norovirus, Taiwan, 2016. Emerg Infect Dis. 2017;23:1180. doi:10.3201/eid2307.170212.
- Chan MCW, Leung TF, Chung TWS, Kwok AK, Nelson EAS, Lee N, et al. Virus Genotype distribution and virus burden in children and adults hospitalized for Norovirus gastroenteritis, 2012–2014, Hong Kong. Rep. 2015;5:11507. doi:10.1038/srep11507.
- Cheung SKC, Kwok K, Zhang LY, Mohammad KN, Lui GCY, Lee N, Nelson EAS, Lai RWM, Leung TF, Chan PKS. Higher viral load of emerging Norovirus GII.P16-GII.2 than pandemic GII.4 and epidemic GII.17, Hong Kong, China. Emerg Infect Dis. 2018;25:119–22. doi:10.3201/eid2501.180395.
- Jin M, Sun JL, Chang ZR, Li HY, Duan ZJ. Outbreaks of noroviral gastroenteritis and their molecular characteristics in China, 2006-2007. Chin J Epidemiol. 2010;31:549–53. doi:10.3760/cma.j.0254-6450.2010.05.017.
- Shen JC, Liu YF, Li QJ, Xie HP, Jiang LY, Wu XW, et al. Norovirus infection and genotype in young children in Guangzhou, 2008 South China. J Med Pest Control. 2010;36:1–10. (in Chinese). doi:3969/j.1003-6245.2010.05.00.
- Shen Z, Qian F, Li Y, Hu Y, Yuan Z, Zhang J. Novel Norovirus GII.4 Variant, Shanghai, China, 2012. Emerg Infect Dis. 2013;19:1337–39. doi:10.3201/eid1908.130026.
- Zhang P, Chen LP, Fu Y, Ji L, Wu XF, Xu DS, Han J. Clinical and molecular analyses of norovirus-associated sporadic acute gastroenteritis: the emergence of GII.17 over GII.4, Huzhou, China, 2015. BMC Infect Dis. 2016;16:1–8. doi:10.1186/s12879-016-2033-x.
- Hao SX, Xu H, Xue Y, Xu JF. Analysis on Norovirus infection and evolutionary recombination in 832 diarrhea samples of Zhenjiang area in 2015. Mod Preventive Med. 2017;44:4181–85. (in Chinese).
- Jin M, Wu SY, Kong XY, Xie HP, Fu JG, He YQ, Feng W, Liu N, Li J, Rainey JJ. Norovirus outbreak surveillance, China, 2016–2018. Emerg Infect Dis. 2020;26(3):437–45. doi:10.3201/eid2603.191183.
- Fu JG, Bao CJ, Huo X, Hu JL, Shi C, Lin Q, Zhang J, Ai J, Xing Z. Increasing recombinant strains emerged in Norovirus outbreaks in Jiangsu, China: 2015–2018. Sci Rep. 2019;9:20012. doi:10.1038/s41598-019-56544-2.
- Gao ZY, Liu BW, Yan HQ, Li WH, Jia L, Tian Y, Chen Y, Wang Q, Pang X. Norovirus outbreaks in Beijing, China, from 2014 to 2017. J Infect. 2019;79:159–66. doi:10.1016/j.jinf.2019.05.019.
- Chen HL, Qian FX, Xu J, Chan M, Shen Z, Zai SB, Shan M, Cai J, Zhang W, He J. A novel norovirus GII.17 lineage contributed to adult gastroenteritis in Shanghai, China, during the winter of 2014–2015. Emerging Microbes Infect. 2015;4(1):e67. doi:10.1038/emi.2015.67.
- Chan MCW, Lee N, Hung TN, Kwok K, Cheung K, Tin EKY, Lai RWM, Nelson EAS, Leung TF, Chan PKS, et al. Rapid emergence and predominance of a broadly recognizing and fast-evolving norovirus GII.17 variant in late 2014. Nat Commun. 2015;6:10061. doi:10.1038/ncomms10061.
- Lu J, Lm S, Fang L, Yang F, Mo YL, Lao JQ, Zheng H, Tan X, Lin H, Rutherford S, et al. Gastroenteritis outbreaks caused by Norovirus GII.17, Guangdong Province, China, 2014-2015. Emerg Infect Dis. 2015;21:1240–42. doi:10.3201/eid2107.150226.
- Fu J, Ai J, Jin M, Jiang C, Zhu Y. Emergence of a new GII.17 norovirus variant in patients with acute gastroenteritis in Jiangsu, China, September 2014 to March 2015. Eur Communicable Dis Bull. 2015;20:21157. doi:10.2807/1560-7917.ES2015.20.24.
- Wu W, Zhang HB, Zhang HL, Yang H, He YQ. The genotype of norovirus in Shenzhen, 2010. Chin J Virol. 2012;28:219–23. (in Chinese). doi:10.13242/j.cnki.bingduxuebao.002269.
- Tu LT, Liu FP, Huang YC, Huang CG, Yang S, Tsao KC, Lai M-W, Chen C-J. Genetic susceptibility to Norovirus GII.4 Sydney Strain infections in Taiwanese children. Pediatr Infect Dis J. 2017;36:353–57. doi:10.1097/INF.0000000000001446.
- Song SL, Wei T, Zhang XH. Molecular characteristics of norovirus responsible for diarrhea outbreaks in Yuhang district of Hangzhou,2006-2007. Chin J Prev Med. 2010;11:225–27. (in Chinese). doi:10.16506/j.1009-6639.2010.03.006.
- Luan MC, Liu YL, Lang XY, Yu L, Bo ZJ. Genetic characteristics of noroviruses from foodborne diarrhea in Dalian. Chin J Microecol. 2018;30:1313–16. (in Chinese). doi:10.13381/j.cnki.cjm.201811018.
- Yao X, Jiang XH, Chen LL, Zhang CL, Xie NH. Genotype analysis of acute gastroenteritis outbreaks by norovirus in Fuzhou city of China. Chin J Viral Dis. 2020;10:1–5. (in Chinese). doi:10.16505/j.2095-0136.2019.0072.
- Han JK, Wu XF, Chen LP, Fu Y, Xu DS, Zhang P, Ji L. Emergence of norovirus GII.P16-GII.2 strains in patients with acute gastroenteritis in Huzhou, China, 2016–2017. BMC Infect Dis. 2018;18:342. doi:10.1186/s12879-018-3259-6.
- Wang X, Yong W, Du XF, Zhang Z, He M, Qiao M, et al. Molecular epidemiology of Norovirus outbreaks in Nanjing,China,2014-2017. Chin J Virol. 2018;04:483–90. (in Chinese). doi:10.13242/j.cnki.bingduxuebao.003393.
- Cheng Y, Meng JT, Zhang XC, Huang WW, Chen ZH, Li GY, et al. Prevalence of Norovirus genotypes in Chengdu. J Preventive Med Inform. 2018;34:1294–97. (in Chinese).
- Zhang J, Chang ZR, Sun JL, Liao QH, Xing WJ, Liu FF. Infectious diarrhea epidemics caused by norovirus and its control strategy in China. Dis Surveillance. 2014;29:516–21. (in Chinese). doi:10.3784/j.1003-9961.2014.07.004.
- Lian YY. The epidemic characteristics and transmission risk factors of norovirus outbreaks reprorted to the National Public Health Emergency Management Information System in China from 2014-2018. Chinese Center for Disease Control and Prevention; 2019. (in Chinese).
- Ao YY, Wang JJ, Hua L, He YQ, Dong XG, Xuan W, Peng J, Zhang H, Jin M, Duan Z. Norovirus GII.P16/GII.2–associated gastroenteritis, China, 2016. Emerg Infect Dis. 2017;23:1172–75. doi:10.3201/eid2307.170034.
- Ao YY, Xie XL, Dong XG, Jin M, Duan ZJ. Genetic analysis of an emerging GII.P2–GII.2 Norovirus associated with a 2016 outbreak of acute gastroenteritis in China. Virol Sin. 2019;34:111–14. doi:10.1007/s12250-019-00084-6.
- Tohma K, Lepore CJ, Ford-Siltz LA, Parra GI, Duprex WP. Phylogenetic analyses suggest that factors other than the capsid protein play a role in the epidemic potential of GII.2 Norovirus. Msphere. 2017;2:e00187–17. doi:10.1128/mSphereDirect.-17.
- Iritani N, Kaida A, Abe N, Sekiguchi J, Kubo H, Takakura KI, Goto K, Ogura H, Seto Y. Increase of GII.2 norovirus infections during the 2009–2010 season in Osaka City, Japan. J Med Virol. 2012;84:517–25. doi:10.1002/jmv.23211.
- Lun J, Hewitt J, Yan G, Enosi Tuipulotu D, William R, White P. Recombinant GII.P16/GII.4 Sydney 2012 was the dominant Norovirus identified in Australia and New Zealand in 2017. Viruses. 2018;10:548. doi:10.3390/v10100548.
- Waters A, Coughlan S, Hall WW. Characterisation of a novel recombination event in the norovirus polymerase gene. VIROLOGY. 2007;363:11–14. doi:10.1016/j.virol.2007.03.012.
- Worobey M, Holmes EC. Evolutionary aspects of recombination in RNA viruses. J Gen Virol. 1999;80:2535–43. doi:10.1099/0022-1317-80-10-2535.
- Bull RA, Hansman GS, Clancy LE, Tanaka MM, White PA. Norovirus recombination in ORF1/ORF2 overlap. Emerg Infect Dis. 2005;11:1079–85. doi:10.3201/eid1107.041273.
- Bull RA, Tanaka MM, White PA. Norovirus recombination. J Gen Virol. 2008;88:3347–59. doi:10.1099/vir.0.83321-0.
- Miller WA, Dreher TW, Hall TC. Synthesis of brome mosaic virus subgenomic RNA in vitro by internal initiation on (−)-sense genomic RNA. Nature. 1985;313:68–70. doi:10.1038/313068a0.
- Graaf MD, Beek JV, Koopmans MPG. Human norovirus transmission and evolution in a changing world. Nat Rev Microbiol. 2016;14:421–33. doi:10.1038/nrmicro.2016.48.
- Bartsch SM, Lopman BA, Hall AJ, Parashar UD, Lee BY. The potential economic value of a human norovirus vaccine for the United States. Vaccine. 2012;30:7097–104. doi:10.1016/j.vaccine.2012.09.040.
- Tan M, Huang P, Xia M, Fang PA, Zhong W, Mcneal M, Wei C, Jiang W, Jiang X. Norovirus P particle, a novel platform for vaccine development and antibody production. J Virol. 2011;85:753–64. doi:10.1128/JVI.01835-10.
- Xia M, Wei C, Wang L, Cao D, Meng XJ, Jiang X, Tan M. A trivalent vaccine candidate against hepatitis E virus, norovirus, and astrovirus. Vaccine. 2016;34:905–13. doi:10.1016/j.vaccine.2015.12.068.
- Tan M, Fang P, Chachiyo T, Xia M, Huang P, Fang Z, Jiang W, Jiang X. Noroviral P particle: structure, function and applications in virus-host interaction. Virology. 2008;382:115–23. doi:10.1016/j.virol.2008.08.047.
- Verma V, Tan W, Puth S, Cho K, Lee E. Norovirus (NoV) specific protective immune responses induced by recombinant P dimer vaccine are enhanced by the mucosal adjuvant FlaB. Transl Med. 2016;14:135. doi:10.1186/s967-016-0899-4.
- Xia M, Tan M, Wei C, Zhong W, Wang L, Mcneal M, Jiang X. A candidate dual vaccine against influenza and noroviruses. Vaccine. 2011;29:7670–77. doi:10.1016/j.vaccine.2011.07.139.
- Wang L, Cao D, Wei C, Meng X, Jiang X, Tan M. A dual vaccine candidate against norovirus and hepatitis E virus. Vaccine. 2014;32(4):445–52. doi:10.1016/j.vaccine.2013.11.064.
- Jiang L, Fan R, Sun S, Fan P, Su W, Gao F, Gao F, Xu F, Kong W, Jiang C. A new EV71 VP3 epitope in norovirus P particle vector displays neutralizing activity and protection in vivo in mice. Vaccine. 2015;33:6596–603. doi:10.1016/j.vaccine.2015.10.104.
- Yu Y, Fu L, Shi Y, Guan S, Yang L, Gong X, Yin H, He X, Liu D, Kuai Z, et al. Elicitation of HIV-1 neutralizing antibodies by presentation of 4E10 and 10E8 epitopes on Norovirus P particles. Immunol Lett. 2015;168(2):271–78. doi:10.1016/j.imlet.2015.10.003.
- Dai Y, Xia M, Huang Q, Tan M, Lin Q, Zhuang Y, Long Y, Li J-D, Jiang X, Zhang X-F, et al. Characterization of antigenic relatedness between GII.4 and GII.17 Noroviruses by use of serum samples from Norovirus-infected patients. J Clin Microbiol. 2017;55:3366–73. doi:10.1128/JCM.00865-17.
- Malm M, Tamminen K, Lappalainen S, Uusi-Kerttula H, Vesikari T, Blazevic V. Genotype considerations for virus-like particle-based bivalent norovirus vaccine composition. Clin Vaccine Immunol. 2015;22(6):656–63. doi:10.1128/CVI.00015-15.
- Malm M, Tamminen K, Timo V, Blazevic V. Norovirus GII.17 Virus-Like Particles Bind to Different Histo-Blood Group Anti-gens and Cross-React with Genogroup II-Specific Mouse Sera. Viral Immunol. 2018;31:649–57. doi:10.1089/vim.2018.0115.
- Czakó R, Atmar R, Opekun AR, Gilger M, Graham D, Estes M, Hodinka RL. Experimental human infection with Norwalk virus elicits a surrogate neutralizing anti-body response with cross-genogroup activity. Clin Vaccine Immunol. 2015;22:221–28. doi:10.1128/CVI.00516-14.
- Han Z, Zhang X, Liu Z, Zhang H, Tang F, Li Q. Cross-blockade antibody spectrum of predominant genotypes of norovirus. Chin J Biologi-cals. 2019;32:1316–21. (in Chinse). doi:10.13200/j.cnki.cjb.002907.
- Son H, Jeong H, Cho M, Lee J, Cheon D, Yoon K, Jeong A-Y, Jung S, Kim K, Cheon D-S. Seroepidemiology of predominant Norovirus strains circulating in Korea by using recombinant virus-like particle antigens. Foodborne Pathog Dis. 2013;10:461–66. doi:10.1089/fpd.2012.1300.
- Tamminen K, Lappalainen S, Huhti L, Vesikari T, Blazevic V. Trivalent combination vaccine induces broad heterologous im-mune responses to norovirus and rotavirus in mice. PLoS ONE. 2013;08:e70409. doi:10.1371/journal.pone.0070409.
- Blazevic V, Lappalainen S, Nurminen K, Huhti L, Vesikari T. Norovirus VLPs and rotavirus VP6 protein as combined vaccine for childhood gastroenteritis. Vaccine. 2011;29:8126–33. doi:10.1016/j.vaccine.2011.08.026.
- Malm M, Diessner A, Tamminen K, Liebscher M, Vesikari T, Blazevic V. Rotavirus VP6 as an adjuvant for bivalent Norovirus vaccine produced in Nicotiana benthamiana. Pharmaceutics. 2019;11:229. doi:10.3390/pharmaceutics11050229.
- Tamminen K, Huhti L, Koho T, Lappalainen S, Blazevic V, Vesikari T, Blazevic V. A comparison of immunogenicity of norovirus GII-4 virus-like particles and P-particles. Immunology. 2011;135:89–99. doi:10.1111/j.365-2567.011.03516.x.
- Mai H, Gao Y, Cong X, Wang H, Liu N, Huang XJ, Xu L, Chen Y, Wei L. GII.4 Sydney_2012 norovirus infection in immunocompromised patients in Beijing and its rapid evolution in vivo. J Med Virol. 2016;88(2):224–33. doi:10.1002/jmv.24332.
- Lindesmith LC, Donaldson EF, Baric RS. Norovirus GII.4 Strain Antigenic Variation. J Virol. 2011;85:231–42. doi:10.1128/JVI.01364-10.
- Cao MC, Li XX, He J. Researches on prevalence and molecular evolution of norovirus GII.17: an overview. Chin J Public Health. 2018;34:134–36. (in Chinese). doi:10.11847/zgggws1118316.
- Dong XG, Qin M, Wang ZE, Yang XX, Wu QR, Feng HR, Wei X, Yu H, Li J, Li J. Should we pay attention to recombinant norovirus strain GII.P7/GII.6? J Infect Public Heal. 2019;12:403–09. doi:10.1016/j.jiph.2018.12.007.
- Zhu L, Tong JX, Yao XL, Deng XQ, Lu Y. Analysis of norovirus detection of patients with acute gastroenteritis in Fushun from 2017 to 2019. Chin Front Health Quarantine. 2020;43:337–39. (in Chinese). doi:10.16408/j.1004-9770.2020.05.011.
- Dai YC. Evolution mechanism of major epidemic norovirus GII.4 strain and passive immunization based on P Particle. Southern Medical University; 2013. (in Chinese).
- Swanstrom J, Lindesmith LC, Donaldson EF, Yount B, Baric RS. Characterization of blockade antibody responses in GII.2.1976 Snow Mountain virus-infected subjects. J Virol. 2014;88(2):829–37. doi:10.1128/JVI.02793-13.
- Tan M, Jiang X, Madhani HD. Norovirus gastroenteritis, carbohydrate receptors, and animal models. PLoS Pathog. 2010;6:e1000983. doi:10.1371/journal.ppat.1000983.