ABSTRACT
We aimed to evaluate the protective effects of ulinastatin (UTI) on rats with acute lung injury induced by lipopolysaccharide (LPS) via the Toll like receptor 4 (TLR4)/myeloid differentiation factor 88 (MyD88)/nuclear factor-kappa B (NF-κB) signaling pathway. Forty-eight male Wistar rats were randomly divided into model, control, dexamethasone (DXM) and UTI groups. The body weight loss ratio and wet-to-dry weight ratio (W/D) of lung tissue were calculated at 10 h. The permeability of pulmonary vascular endothelium was detected by Evans blue method. Tumor necrosis factor-α (TNF-α), interleukin-6 (IL-6) and IL-1β levels in bronchial lavage fluid were detected by enzyme-linked immunosorbent assay. Total cells and neutrophils were counted by microscopy. TLR4, MyD88 and NF-κB expressions were detected by Western blotting. Compared with model group, DXM and UTI groups had significantly higher body weights and lower W/D values (P < 0.05). In DXM and UTI groups, the lung tissue structure was close to normal, inflammatory cell infiltration was alleviated, and hematoxylin-eosin staining scores were significantly lower than that of model group (P < 0.05). Compared with model group, the concentrations of Evans blue, IL-1β, IL-6 and TNF-α levels, and protein expressions of TLR4, MyD88 and NF-κB in DXM and UTI groups decreased significantly (P < 0.05). UTI inhibits LPS-induced activation of the TLR4/MyD88/NF-κB signaling pathway, thereby alleviating inflammatory response and protecting against lung injury.
Introduction
With the aggravation of air pollution and changes in lifestyles in recent years, the incidence rate of acute respiratory distress syndrome (ARDS) is increasing annually. ARDS occurs mostly upon diseases such as severe trauma, shock and infection [Citation1,Citation2]. Due to injury of pulmonary capillary and alveolar epithelial cells, patients may undergo diffuse pulmonary interstitial and alveolar edema, with the clinical manifestations of hypoxemia and respiratory distress. It is well-established that inflammatory response is the root cause for ARDS [Citation3]. The onset and progression of acute lung injury (ALI) are also caused by uncontrollable inflammatory response, leading to mutual activation and interaction among a large number of inflammatory factors and effector cells [Citation4]. Regardless of many studies on ALI/ARDS treatment, the mortality rate remains high, and the molecular mechanism is still unclear. Lung injury has been associated with the activation of Toll like receptor 4 (TLR4) [Citation5], which then releases large amounts of inflammatory mediators via the downstream pathway myeloid differentiation factor 88 (MyD88)/nuclear factor-kappa B (NF-κB). Ulinastatin (UTI) is a broad-spectrum hydrolase inhibitor, and its anti-inflammatory effect has attracted widespread attention [Citation6]. It can inhibit proteolytic activity and prevent endotoxin from stimulating macrophages to release inflammatory factors, thereby relieving the damage to tissues [Citation7]. However, whether UTI can mitigate lung injury by suppressing lipopolysaccharide (LPS)-induced inflammatory cascade via the TLR4/MyD88/NF-κB signaling pathway remains elusive.
Therefore, we herein intended to explore whether UTI exerted protective effects on an ALI rat model established by LPS injection via the TLR4/MyD88/NF-κB signaling pathway. This study provided valuable experimental evidence for future clinical use.
Materials and methods
Animals and main reagents
Forty-eight SPF Wistar male rats aged 12–15 weeks and weighing 200 g-220 g were provided by the Animal Experimental Center of our hospital. All the experimental procedures involving animal study were performed in accordance with the guidelines for Animal Ethical Committee (Xuzhou Central Hospital, China).
LPS was purchased from Sigma (USA). UTI was bought from Merck & Co., Inc. (USA). Positive control drug dexamethasone (DXM) was obtained from the National Institute for Food and Drug Control (China). Evans blue was provided by Tianjin Baihaoxin Biotechnology Co., Ltd. (China). BCA protein concentration determination kit and DAB chemiluminescence kit were purchased from Beijing Huaxia Biotechnology Co., Ltd. (China). TLR4, MyD88 and NF-κB antibodies were bought from Santa Cruz Biotechnology (USA). Tumor necrosis factor-α (TNF-α), interleukin-6 (IL-6) and IL-1β enzyme-linked immunosorbent assay (ELISA) kits were obtained from Nanjing Jiancheng Bioengineering Institute (Zhongda Hospital, China).
Modeling and grouping
All rats were fed for 7 days to adapt to the environment, numbered, and then randomly divided into 4 groups (n = 12), i.e. control group, model group, DXM intervention group and UTI intervention group. For the model group, 0.5 mL of LPS (5 mg/kg) was injected into the tail vein [Citation8], and the control group was given an equal volume of normal saline. The DXM intervention group and UTI intervention group were intraperitoneally injected with 1 mL of DXM solution (2 mg/kg) and 1 mL of UTI solution (5 mg/kg) respectively 24 h and 10 min before LPS injection for pretreatment. The control and model groups were given equal volumes of normal saline. The rats were intraperitoneally injected with 10% chloral hydrate 10 h after modeling. Under anesthesia, they were intubated after tracheotomy, and sacrificed by opening the abdomen and bled through the abdominal aorta. Three rats from each group were used to measure the wet-to-dry weight ratio (W/D) of lung tissue and pulmonary vascular endothelial permeability, three were utilized for the collection of bronchial lavage fluid (BALF) sample and ELISA, three were used for the pathological observation of lung tissue, and the remaining three were employed for Western blotting.
Body weight measurement
Body weights were recorded before and 10 h after modeling to calculate the weight loss ratio: (Weight10 h after modeling – weightbefore modeling)/weightbefore modeling
Measurement of W/D of lung tissue
After the rats were sacrificed, the lower lobe of the left lung was taken after thoracotomy, and the blood on the surface of lung tissues was gently sucked dry. The tissues were weighed (wet weight W), dried in a 60°C oven for 48 h, and weighed (dry weight D) to obtain W/D [Citation9].
Detection of pulmonary vascular endothelial permeability
The Evans blue method was used for detection [Citation10]. Briefly, dried lung tissue was added formamide according to the ratio of 1 mL/100 mg wet lung weight, homogenized, and centrifuged at 4,000 rpm for 0.5 h after reaction in a 60°C water bath for 24 h. The resulting supernatant was added to a 96-well plate and measured at 620 nm. The Evans blue concentration was calculated according to a standard curve and converted to μg/g dry lung tissue.
Collection of BALF sample
After anesthesia, the rats were sacrificed by right ventricular blood collection. Tracheal separation was performed under aseptic conditions. The trachea cannula was inserted into the left main bronchus, and then ligated by silk thread. The left lung was washed with PBS three times, 5 mL each time. BALF was collected with the recovery of above 80%, and thereafter centrifuged at 2,000 rpm for 10 min to obtain the supernatant which was stored in a − 80°C refrigerator prior to use. The precipitate was resuspended, evenly spread on a glass slide, and stained with Wright Giemsa solution. The slide was observed under a microscope to count total cells and neutrophils [Citation11].
Pathological observation of lung tissue
Tissue samples were fixed in 10% formaldehyde for 24 h, sectioned and observed after hematoxylin-eosin (HE) staining for pathological changes under a light microscope. Evaluation was conducted in terms of infiltration of alveolar cells, alveolar hemorrhage, alveolar septal edema and alveolar fibrin deposition. Four sections were taken for each rat and six different optical fields were selected for each section. The scores were finally averaged. Pathological findings were scored from 0 to 4 points: 0, without injury; 1 point, mild injury (with an area of about 25% of optical field); 2 points, moderate injury (with an area of about 50% of optical field); 3 points, severe injury (with an area of about 75% of optical field); 4 points, injury covers almost the entire optical field. The sections were observed and paragraphed by experienced pathologists in a blinded manner under a light microscope [Citation12].
Detection of TLR4, MyD88 and NF-κB protein expressions by Western blotting
Lung tissue was homogenized by grinding, lysed on ice for 30 min and centrifuged to collect the supernatant. Protein samples were resolved by 5% SDS-PAGE, and the products were electronically transferred onto a PVDF membrane. Subsequently, the membrane was blocked overnight at 4°C, incubated with primary antibodies against TLR4, MyD88, NF-κB and β-actin (1:1500 diluted) for 1 h, washed, and incubated with HRP-labeled goat anti-rabbit IgG antibody (1:10,000 diluted). After being treated with ECL reagent for 30 min, the membrane was exposed to X-ray film, developed and air-dried at room temperature. Relative protein expression levels were represented by using β-actin expression as the internal reference [Citation13].
Detection of TNF-α, IL-6 and IL-1β levels in BALF by ELISA
The TNF-α, IL-6 and IL-1β levels in BALF were measured strictly according to the instructions of ELISA kits using a multifunctional microplate reader [Citation14].
Statistical analysis
All data were analyzed by SPSS16.0 software. The categorical data conforming to normal distribution were expressed as mean ± standard deviation. Multigroup comparisons were performed by one-way analysis of variance, and pairwise comparisons were conducted with the independent samples t test. P < 0.05 was considered statistically significant.
Results
Effects of UTI on body weight and lung tissue W/D of LPS-induced rats
Lung tissue W/D reflects the abnormality of vascular permeability and the severity of pulmonary edema [Citation15]. Compared with the control group, the body weights of model, DXM and UTI groups decreased significantly (P < 0.05). Compared with the model group, the body weights of DXM and UTI groups increased significantly (P < 0.05). Compared with the control group, W/D of the model group significantly increased after LPS stimulation (P < 0.05). Compared with the model group, the W/D values of DXM and UTI groups decreased significantly (P < 0.05) ().
Effects of UTI on numbers of neutrophils and total cells in BALF of LPS-induced rats
The numbers of neutrophils and total cells in BALF indirectly reflect the infiltration of neutrophils in lung tissue and the severity of inflammation [Citation16]. Compared with the control group, the numbers of neutrophils and total cells in BALF of the model group increased significantly (P < 0.05). Compared with the model group, the numbers of DXM and UTI groups significantly decreased (P < 0.05) (). Therefore, UTI significantly inhibited LPS-induced inflammatory cell infiltration.
Effects of UTI on pathological injury of lung tissue in LPS-induced rats
The lung tissue of the control group was intact, without congestion, and the alveolar space was clear. No edema or inflammatory cell infiltration was found in the septum. Compared with the control group, the alveolar space of the model group was significantly smaller. The septum was thickened, the alveolar wall showed congestion and edema, a large amount of inflammatory cell infiltration appeared, and the HE staining score was significantly higher (P < 0.05). In DXM and UTI groups, the lung tissue structure was close to normal, inflammatory cell infiltration was alleviated, and the HE staining scores were significantly lower than those of the model group (P < 0.05) ( and ).
Figure 3. Effects of UTI on pathological injury of lung tissue in LPS-induced rats. Magnification: 100 × . A: Control group, normal alveoli (asterisk) and alveolar septa (black arrow) with few neutrophils are shown; B: model group, with thickened septum (asterisk), alveolar bleeding (black arrow) as well as considerable cell infiltration and alveolar fibrin deposition (yellow arrow); C: DXM group, with significantly alleviated cell infiltration and alveolar fibrin deposition; D: UTI group, with significantly alleviated cell infiltration and alveolar fibrin deposition.
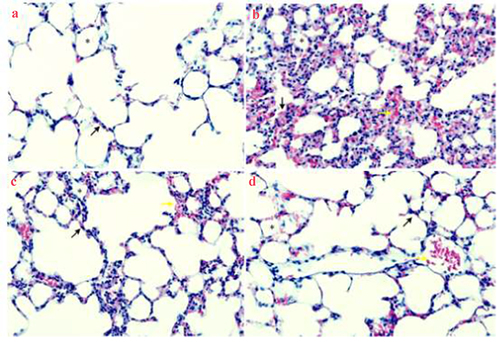
Table 1. HE staining scores.
Effects of UTI on pulmonary vascular endothelial permeability of LPS-induced rats
After being stimulated by inflammatory factors, multiple signaling pathways in endothelial cells are activated, eventually leading to cell contraction, morphological changes and increase in vascular permeability [Citation17]. Evans blue concentration was used to reflect the pulmonary vascular endothelial permeability. Compared with the control group, the concentration of Evans blue in the model group was significantly elevated (P < 0.05). Compared with the model group, the concentrations of DXM and UTI groups decreased significantly (P < 0.05) ().
Effects of UTI on levels of IL-1β, IL-6 and TNF-α in BALF of LPS-induced rats
The lung injury model established by administration of bacterial endotoxin LPS is in line with the clinical course of ALI. LPS leads to the massive production and release of pro-inflammatory mediators such as TNF-α, IL-6 and IL-1β. Meanwhile, it induces oxidation/antioxidation imbalance, lipid peroxidation, and production of considerable oxygen free radicals causing damage to tissues and cells [Citation18]. Compared with the control group, the levels of IL-1β, IL-6 and TNF-α in the model group were significantly raised (P < 0.05). Compared with the model group, the levels of DXM and UTI groups significantly dropped (P < 0.05) ().
Effects of UTI on protein expressions of TLR4, MyD88 and NF-κB in lung tissue of LPS-induced rats
HMGB1, TLR4 and NF-κB play key roles in the onset and progression of sepsis. TLR-4 is a downstream factor of the HMGB1/TLR-4/NF-κB signaling pathway [Citation19]. When HMGB1 binds TLR-4, it activates the NF-κB pathway, thereby promoting the release of inflammatory factors [Citation20]. Compared with the control group, the protein expressions of TLR4, MyD88 and NF-κB in the model group significantly increased (P < 0.05). Compared with the model group, the expressions of DXM and UTI groups significantly reduced (P < 0.05) (). Thus, the protective effects of UTI on rat lung injury were associated with proteins of the TLR4/MyD88/NF-κB signaling pathway.
Discussion
ALI, as a common clinical respiratory critical disease, is pulmonary parenchyma injury mainly caused by the interaction of inflammatory mediators and effector cells [Citation21]. At present, effective therapies are still lacking in clinical practice, so it is imperative to seek prevention and treatment measures. UTI can inhibit fibrin decomposition and stabilize lysosomal membranes [Citation22], which is often used to treat shock and acute pancreatitis. However, the influence of UTI on ALI has rarely been reported. Therefore, this study used LPS to construct an ALI model to explore the mechanism for protecting lung injury.
The pathological characteristics of ALI mainly include lung epithelial cell damage, osmotic pulmonary edema, as well as migration and infiltration of a large number of inflammatory cells [Citation23]. Alveolar epithelial or endothelial cells are damaged mainly because the regulatory network composed of inflammatory mediators is uncontrollable. Neutrophils are recruited when stimulated by the external environment. After being activated, they can release a large amount of superoxide oxidase or free radicals which in turn damage lung epithelial and endothelial cells, and indirectly causing pulmonary edema [Citation24]. UTI can inhibit the activity of proteolytic enzymes, thereby attenuating further activation of macrophages by inflammatory stimuli produced by tissue cell destruction, and the release of inflammatory factors by endotoxin-stimulated macrophages [Citation25]. In this study, LPS significantly reduced body weight and lung tissue W/D, which was relieved by UTI. HE staining results showed that UTI protected lung tissue structure, as well as alleviated lung hemorrhage, edema and inflammatory infiltration. The concentration of Evans blue was measured to reflect the permeability of rat pulmonary vascular endothelium. The results showed that the concentration of Evans blue in the model group was significantly higher than that in the control group. Compared with the model group, the concentration of Evans blue decreased significantly in DXM and UTI groups, indicating that UTI not only protected against LPS-induced ALI, but also decreased the pulmonary vascular permeability.
Upon ALI, a large number of inflammatory cells are infiltrated to produce cytokines and inflammatory mediators, and inflammatory signals are amplified step by step, which result in microcirculatory disorders, enhanced permeability of alveolar epithelial cells and injury of pulmonary capillary endothelium, eventually causing alveolar hemorrhage and pulmonary edema [Citation26]. As key factors involved in the inflammatory cascade, IL-1β, IL-6 and TNF-α play crucial roles in inducing cell infiltration and accelerating the progression of respiratory system diseases. To investigate the role of UTI in pulmonary gas exchange, this study examined the changes of these factors in BALF, and showed that UTI significantly reduced IL-1β, IL-6 and TNF-α levels. When TLR4 is released outside cells, it not only promotes inflammatory reaction, but also interacts with the inflammatory mediators to form an immune-stimulating complex [Citation27]. Besides, TLR4 is a key receptor for endotoxin transmembrane signaling, which can activate MyD88-dependent pathway, promote TκB phosphorylation, and then activate NF-κB [Citation28]. This pathway is associated with cellular oxidation, apoptosis and inflammatory response [Citation29–31]. Herein, the protein levels of TLR4, MyD88 and NF-κB significantly increased after modeling, while those of the UTI group decreased, suggesting that the protective effects of UTI on LPS-induced ALI in rats were associated with the TLR4-mediated MyD88/NF-κB pathway.
Conclusion
In summary, UTI can inhibit LPS-induced activation of the TLR4/MyD88/NF-κB signaling pathway, thereby alleviating inflammatory response and protecting against lung injury. Nevertheless, further studies are in need to clarify whether UTI can affect LPS-induced lung injury by regulating other pathways.
Highlights
Lipopolysaccharide induces activation of the TLR4/MyD88/NF-κB signaling pathway.
Ulinastatin inhibits the pathway activation.
Ulinastatin relieves inflammatory response and protects against lung injury.
Disclosure statement
No potential conflict of interest was reported by the author(s).
Additional information
Funding
References
- Bellani G, Laffey JG, Pham T, et al. Epidemiology, patterns of care, and mortality for patients with acute respiratory distress syndrome in intensive care units in 50 countries. JAMA. 2016;315(8):788–800.
- Mekontso Dessap A, Boissier F, Charron C, et al. Acute cor pulmonale during protective ventilation for acute respiratory distress syndrome. prevalence, predictors, and clinical impact. Intensive Care Med. 2016;42(5):862–870.
- Kacmarek RM, Villar J, Sulemanji D, et al. Open lung approach for the acute respiratory distress syndrome: a pilot, randomized controlled trial. Crit Care Med. 2016;44(1):32–42.
- Ionescu L, Byrne RN, Van HT, et al. Stem cell conditioned medium improves acute lung injury in mice: in vivo evidence for stem cell paracrine action. Am J Physiol Lung Cell Mol Physiol. 2012;303(11):L967–977.
- Cong L, Yang S, Zhang Y, et al. DFMG attenuates the activation of macrophages induced by co culture with LPC injured HUVE 12 cells via the TLR4/MyD88/NF κB signaling pathway. Int J Mol Med. 2018;41:2619–2628.
- Wei F, Liu S, Luo L, et al. Anti-inflammatory mechanism of ulinastatin: inhibiting the hyperpermeability of vascular endothelial cells induced by TNF-α via the RhoA/ROCK signal pathway. Int Immunopharmacol. 2017;46:220–227.
- Li X, Su L, Zhang X, et al. Ulinastatin downregulates TLR4 and NF-kB expression and protects mouse brains against ischemia/reperfusion injury. Neurol Res. 2017;39(4):367–373.
- Wang Y, Huang W, Zhang J, et al. The therapeutic effect of Bletilla striata extracts on LPS-induced acute lung injury by regulation of inflammation and oxidation. RSC Adv. 2016;6(92):89338–89346.
- Yin JT, Wan B, Liu DD, et al. Emodin alleviates lung injury in rats with sepsis. J Surg Res. 2016;202(2):308–314.
- Kaya M, Ahishali B. Assessment of permeability in barrier type of endothelium in brain using tracers: evans blue, sodium fluorescein, and horseradish peroxidase. Methods Mol Biol. 2011;763:369–382.
- Sun Q, Fang L, Roth M, et al. Bronchial thermoplasty decreases airway remodelling by blocking epithelium-derived heat shock protein-60 secretion and protein arginine methyltransferase-1 in fibroblasts. Eur Respir J. 2019;54(6):1900300.
- Andersen MB, Bodtger U, Andersen IR, et al. Metastases or benign adrenal lesions in patients with histopathological verification of lung cancer: can CT texture analysis distinguish? Eur J Radiol. 2021;138:109664.
- Jin YH, Li ZT, Chen H, et al. Effect of dexmedetomidine on kidney injury in sepsis rats through TLR4/MyD88/NF-kappaB/iNOS signaling pathway. Eur Rev Med Pharmacol Sci. 2019;23:5020–5025.
- Huang C, Pan L, Lin F, et al. Monoclonal antibody against Toll-like receptor 4 attenuates ventilator-induced lung injury in rats by inhibiting MyD88-and NF-κB-dependent signaling. Int J Mol Med. 2017;39(3):693–700.
- Zhu T, Wei Z, Wang DX. Insulin up-regulates epithelial sodium channel in LPS-induced acute lung injury model in rats by SGK1 activation. Injury. 2012;43(8):1277–1283.
- Tao H, Li N, Zhang Z, et al. Erlotinib protects LPS-induced acute lung injury in mice by inhibiting EGFR/TLR4 signaling pathway. Shock. 2019;51(1):131–138.
- Yuan SY. Protein kinase signaling in the modulation of microvascular permeability. Vasc Pharmacol. 2002;39(4–5):213–223.
- Li X, Shan C, Wu Z, et al. Emodin alleviated pulmonary inflammation in rats with LPS-induced acute lung injury through inhibiting the mTOR/HIF-1α/VEGF signaling pathway. Inflamm Res. 2020;69(4):365–373.
- Zhou H, Deng M, Liu Y, et al. Platelet HMGB1 is required for efficient bacterial clearance in intra-abdominal bacterial sepsis in mice. Blood Adv. 2018;2(6):638–648.
- Zhou S, Wang G, Zhang W. Effect of TLR4/MyD88 signaling pathway on sepsis-associated acute respiratory distress syndrome in rats, via regulation of macrophage activation and inflammatory response. Exp Ther Med. 2018;15:3376–3384.
- Basios N, Lampropoulos P, Papalois A, et al. Apigenin attenuates inflammation in experimentally induced acute pancreatitis-associated lung injury. J Invest Surg. 2016;29(3):121–127.
- Leng YX, Yang SG, Song YH, et al. Ulinastatin for acute lung injury and acute respiratory distress syndrome: a systematic review and meta-analysis. World J Crit Care Med. 2014;3(1):34–41.
- Lin X, Barravecchia M, Kothari P, et al. β1-Na(+,)K(+)-ATPase gene therapy upregulates tight junctions to rescue lipopolysaccharide-induced acute lung injury. Gene Ther. 2016;23(6):489–499.
- Koma T, Yoshimatsu K, Nagata N, et al. Neutrophil depletion suppresses pulmonary vascular hyperpermeability and occurrence of pulmonary edema caused by hantavirus infection in C.B-17 SCID mice. J Virol. 2014;88(13):7178–7188.
- Atal SS, Atal S. Ulinastatin - a newer potential therapeutic option for multiple organ dysfunction syndrome. J Basic Clin Physiol Pharmacol. 2016;27:91–99.
- Pinheiro NM, Santana FP, Almeida RR, et al. Acute lung injury is reduced by the α7nAChR agonist PNU-282987 through changes in the macrophage profile. FASEB J. 2017;31(1):320–332.
- Li K, Yang J, Han X. Ketamine attenuates sepsis-induced acute lung injury via regulation of HMGB1-RAGE pathways. Int Immunopharmacol. 2016;34:114–128.
- Yao RB, Zhao ZM, Zhao LJ, et al. Sinomenine inhibits the inflammatory responses of human fibroblast-like synoviocytes via the TLR4/MyD88/NF-κB signaling pathway in rheumatoid arthritis. Pharmazie. 2017;72:355–360.
- Cong L, Yang S, Zhang Y, et al. DFMG attenuates the activation of macrophages induced by co culture with LPC injured HUVE 12 cells via the TLR4/MyD88/NF-κB signaling pathway. Int J Mol Med. 2018;41:2619–2628.
- Wu DM, Wang YJ, Han XR, et al. Tanshinone IIA prevents left ventricular remodelling via the TLR4/MyD88/NF-κB signalling pathway in rats with myocardial infarction. J Cell Mol Med. 2018;22:3058–3072.
- Su Q, Li L, Sun Y, et al. Effects of the TLR4/Myd88/NF-κB signaling pathway on NLRP3 Inflammasome in coronary microembolization-induced myocardial injury. Cell Physiol Biochem. 2018;47(4):1497–1508.