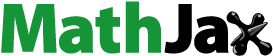
ABSTRACT
To study the effect of nanomedicine on lower extremity deep vein thrombosis (LEDVT) and its correlation with susceptibility genes (SGs), preliminary nanoparticle LSA was constructed using lauric acid and stearic acid, and CTAB-HAuCL4-TEOS (CHT) was obtained using cetyltrimethyl ammonium bromide (CTAB), chloroauric acid (HAuCL4), and tetraethoxysilane (TEOS) to prepare UK-LSA-CHT by combining with urokinase (UK). Twenty rabbits were grouped into UK-LSA-CHT, LSA, UK, and D0 groups. Human umbilical vein endothelial cells (HUVEC) were selected for the cytotoxicity test. The plasma endothelin (ET-1), interleukin 6 (IL-6), and nuclear factor κβ (NF-κβ) of the rabbits were detected with the double antibody sandwich method. Expression of platelet activating factor (PAF) was detected by immunohistochemical staining. Genotype distribution was detected with the second-generation sequencing technology (SGST). The results showed that expressions of ET-1, IL-6, and NF-κβ) in the UK-LSA-CHT group were lower than those in the LSA group and the UK group after 12 hours (P < 0.05). Frequencies of allele G and T, and GT and TT genotype ratios of SG NOS3 rs1799983 in the UK -LSA-CHT group were less than those in LSA and UK groups (P < 0.05). The UK-LSA-CHT group had an observably lower value in PAF positive expression than LSA and UK groups (P < 0.05). In short, UK-LSA-CHT had a good biocompatibility and safety and a good therapeutic effect on LEDVT. N0S3 rs1799983 alleles G and T may be the key risk factors for the occurrence and development of LEDVT.
1. Introduction
Lower extremity deep vein thrombosis (LEDVT) refers to that blood often coagulates and forms thrombus in the deep veins of the lower extremities. The newly formed iliac femoral vein fresh thrombus may fall off and flow into the heart and a pulmonary arteries with venous blood flow, causing pulmonary embolism, which is a common fatal complication after surgery [Citation1]. Clinical data shows that there are about 2–10 million cases worldwide each year, and the annual medical cost of venous thrombosis can reach more than 10 billion US dollars [Citation2–4]. LEDVT is mainly caused by venous blood flow stagnation, venous wall damage, and blood hypercoagulation. The clinical manifestations are sudden swelling of one limb, local pain, secondary varicose veins, dermatitis, pigmentation, stasis ulcers, and calf muscle tenderness, etc. [Citation5,Citation6]. Anticoagulation is the basic treatment for deep vein thrombosis. It can inhibit the spread of thrombosis, facilitate the autolysis of thrombus, recanalize the lumen, and reduce the incidence of post-thrombotic syndrome and mortality. However, anticoagulation alone cannot effectively eliminate thrombosis. Thrombolysis is generally divided into catheter contact thrombolysis and system thrombolysis. A catheter contact test is to place the test catheter into the venous thrombosis, and the thrombolytic drug directly acts on the thrombus. Systemic thrombolysis is the systemic application of thrombolytic drugs via peripheral veins. Among them, catheter contact thrombolysis has obvious advantages, which can greatly increase the dissolution rate of thrombus, reduce the incidence of post-thrombotic syndrome, short treatment time, and fewer complications, providing the first choice for clinical thrombolysis [Citation7]. The thrombolytic drugs currently applied in clinical practice have evolved from the first generation of urokinase (UK) and streptokinase to the third generation of retiprose and tenecteplase, which has greatly improved the half-life of the drug and the efficiency of thrombolysis [Citation8,Citation9]. But in fact, many thrombolytic drugs have obvious adverse reactions and are relatively expensive. It is necessary to further explore the delivery effectiveness and safety of thrombolytic drugs.
Nowadays, the rapid development of nanotechnology provides a constructive method for the delivery of thrombolytic drugs in the human body. Compared with traditional drug delivery methods, nanomedicine can prolong the drug half-life and drug targeting and improve drug stability and effect, so it is superior highly [Citation10]. Moreover, the innovation cost of nanomedicine delivery technology is lower than that of traditional innovative drug research and development models, and the effect of improving drug action is very obvious, so it has been widely and extensively used in the medical field [Citation11,Citation12]. At present, there are dozens of nanomedicines in the market, including classic liposome drugs such as amphotericin B liposomes, daunorubicin liposomes, doxorubicin liposomes, and paclitaxel liposomes [Citation13]. As an important experimental technology, deoxyribonucleic acid (DNA) sequencing has a wide range of applications in biological research, but traditional Sanger sequencing can no longer fully meet the needs of research. Re-sequencing the genomes of model organisms and sequencing the genomes of some non-model organisms require sequencing technology with lower cost, higher throughput, and faster speed, so the SGST was born [Citation14–16]. The second-generation sequencing technology (SGST), also known as high-throughput sequencing, is a DNA sequencing technology developed based on polymerase chain reaction (PCR) and gene chips. Compared with the first-generation sequencing technology, SGST pioneered the introduction of reversible termination ends, thereby realizing sequencing while synthesizing, that is to determine the DNA sequence by capturing the tags of the newly synthesized ends. It not only shows fast sequencing speed and low sequencing cost, but also maintains high accuracy. Although the sequencing technology has developed from the first generation to the third and even the fourth generation, the second-generation short-read sequencing technology still occupies an absolute dominant position in the global sequencing market [Citation17]. Therefore, this article intends to analyze the changes of nanomedicine treatment of SG for LEDVT based on SGST.
In summary, the application of SGST and composite nanomedicine in the treatment of clinical diseases has always been a very hot topic. In this study, it was assumed that the composite nano-drug particles prepared with LSA as basic materials had better physical and chemical properties, showed better therapeutic effects on LEDVT than conventional drugs, and were related to the expression of susceptible genes. Therefore, the nano-precipitation method was used to prepare new nano-drug particles UK-LSA-CHT with LSA as basic materials. Twenty New Zealand white rabbits were prepared as a model of LEDVT, and treated with the new nano-drug particles UK-LSA-CHT. The index changes of the model after treatment were analyzed, the curative effect of composite nanomedicine on LEDVT and the expression of related SGs were analyzed, aiming to provide reference for the monitoring and treatment of the molecular level of LEDVT.
2. Materials and methods
2.1 Experimental animals
Twenty New Zealand white rabbits of 8–10 weeks (weighing 2–3 kg) were purchased from the Animal Research Centre of Shenzhen Longhua District Central Hospital were selected as the research objects. They were all fed with ordinary rabbit feed and raised in a normal temperature environment, and were allowed to obtain food and water freely. The rabbits were classified into UK-LSA-CHT, LSA, UK, and D0 groups (NS for control) treated with different medicines. The experiment was implemented in accordance with the experimental protocol, strictly regulated the operating procedures, and did not violate the ethical requirements.
2.2. Construction for lower extremity deep vein thrombosis of rabbit
2 mL/kg of 10% chloral hydrate (WeGene, Shanghai of China) was injected for anesthesia into the ear vein of the rabbit, and the changes in the rabbit’s heart rate were observed. The rabbit was put in a supine position and disinfected the inner side of both limbs, an oblique wound of about 5 cm was opened along the femoral vein at the proximal left lower limb, and then the subcutaneous and muscle tissue were separated carefully. Then, the proximal end was clamped with a blood vessel clamp, the distal end of the vessel wall was clamped with toothless temporal forceps, and 0.1 mL of thrombin was injected into the femoral vein with a 1 mL syringe. After 2 hours, the clamps were removed, and the muscle and skin were sutured well. Finally, it was injected with 40,000 U of gentamicin sulfate solution and put back in the cage to continue feeding.
2.3. Preparation and evaluation of nanomedicine
The nanoprecipitation method was adopted to prepare the nanomedicines, and the specific steps were given as follows. 0.5 g of cetyltrimethyl ammonium bromide (CTAB) (WeGene, Shanghai of China) was dissolved in 245 mL of distilled water and 5 mL of 0.5 mol/L sodium hydroxide (NaOH) solution, and then allowed to react at 74°C for 20 minutes; 10 mL of 37% acetaldehyde and 3.5 mL of 0.03 mol/L HAuCL4 (Hubei Hengjingrui Chemical Co., Ltd., China) were added, and then added with 2 g of tetraethoxysilane (TEOS) (ROCAN, Wuhan of China) and 5 g of ethanol (ROCAN, Wuhan of China) 10 minutes later to incubate for another 2 hours; after the mixture was centrifuged for half an hour, the precipitate was collected to obtain the product CTAB-HAuCL4-TEOS (CHT); 230 mg of lauric acid and 75 mg of stearic acid (Guangzhou Yixin Chemical Technology Co., Ltd., China) were dissolved in methanol, and stirred at 70°C for half an hour with a magnetic device to obtain LSA; 2 mL of UK (Kangfayuan Co., Ltd. Shandong of China) was dissolved in 13 mL of methanol solution and then added with 5 mL LSA; 20 mg of prepared CHT was put into 35 mL of dimethyl formamide (DMF) (ROCAN, Wuhan of China) solution and ultrasonically dispersed for 20 minutes, added the above solution, and then stirred with a magnetic stirrer for another 2 hours; the mixed solution was centrifuged for about 20 minutes at 10,000 rpm to obtain the final UK-LSA-CHT nanoparticles. Enzyme-linked immunosorbent assay (ELISA) was to determine the content of loaded UK in UK-LSA-CHT and the content of UK protein. Then, the encapsulation rate (ER) and drug loading (DL) of UK-LSA-CHT could be calculated with below equations: ER = (total amount of UK – amount of free UK)/total amount of UK, and DL = (total amount of UK – amount of free UK)/weight of medicine system. Dynamic light scattering was to measure the particle size, specific surface area, and heating curve of nanoparticles.
Cytotoxicity: this article selected human umbilical vein endothelial cells (HUVEC) (Yaji Biological Co., Ltd., Shanghai of China) for the cytotoxicity test. The cells were seeded in a 96-well plate at a density of 2,000 cells/well, and cultured in Roswell Park Memorial Institute (RPMI) 1640 medium containing (BIOROC, Tianjin of China) 10% fetal bovine serum (FBS), which was replaced with 0, 10, 50, and 100 μg/mL fresh UK-LSA-CHT medium every 2 days. When the cells grew to about 80%, the cell viability was detected with cell counting kit-8 (CCK-8) at the 0th, 12th, 24th, 36th, 48th, 60th, and 72nd hour. Then, HUVEC and presto blue (PB) were mixed and incubated, and the optical density (OD) at 450 nm was recorded 2 hours later. The release of lactate dehydrogenase (LDH), PGI2, and nitric oxide (NO) were measured with a microplate reader (Science Instrument Co., Ltd. Suzhou of China) at the 0th, 6th, 12th, 18th, and 24th hour. And the initial release was set to 1 as the standard.
2.4. Evaluation of thrombolysis in vivo
The main organs of rabbits were stained with hematoxylin-eosin (HE), and the specific steps were described as below. The rabbit was executed to remove the heart, lung, kidney, and liver tissues, which were prepared into wax-coated sections; the sections were hydrated with gradient alcohol and xylene, stained with hematoxylin for about 10 minutes, differentiated in 70% hydrochloric acid with 10 seconds, and then rinsed with distilled water; the sections were added with eosin staining solution to stain for around 5 minutes, cleared with gradient alcohol and xylene, and then mounted with neutral gum.
2.5. Serological test
The expression of endothelin (ET-1), interleukin 6 (IL-6), and nuclear factor κβ (NF-κβ) of the rabbits in each other were detected with the double antibody sandwich method. And the specific steps were defined as follows. After the standard product was diluted, a blank well, a standard well, and three sample wells to be tested (ET-1, IL-6, and NF-κβ) were set; 50 μL of diluted solution of standard product was added into the standard well of enzyme label package plate (Science Instrument Co., Ltd. Suzhou of China), the three sample wells were added with 50 μL of ET-1, IL-6, and NF-κβ, respectively, to the bottom of the plate without touching the wall, and then the plate was shaken so that the solution could be mixed fully; the plate was sealed with a sealing film and incubated at 37°C lasting for 30 minutes, filled up with washing solution after the liquid was discarded, and then added with 50 μL enzyme label reagent to each well to continue incubation and washing; it was added with 50 μL diaminobenzidine color reagent (BIOROC, Tianjin of China) to each well, incubated at 37°C for about 20 minutes in the dark, and added with stop solution to stop the reaction. The blank was set to zero, the optical density (OD) of each well at 450 nm was recorded, and the relative concentration of each sample to be tested was obtained from the standard curve.
2.6. Immunohistochemical staining
The positive expression of platelet activating factor (PAF) was detected with immunohistochemical staining. The specific steps in detail are described as follows. The prepared lower limb slice was soaked in phosphate buffer solution (PBS), added with 10% goat serum after 25 minutes, and incubated at 37°C for 30 minutes. It was added with corresponding rabbit antibody working solution and kept at 4°C for overnight, and then add with biotin-labeled goat anti-rabbit secondary antibody and incubated for half an hour. Then, it was added with diaminobenzidine (BIOROC, Tianjin of China) to develop color for 45 seconds, flushed with distilled water, and mounted with neutral gum. Ten high-definition visual fields under the microscope were selected to observe and record the positive cells, and the integral OD was obtained with Image-pro Plus6.0 image analysis software to indicate the protein expression, which could be calculated with integral OD = positive surface area × average OD.
2.7. Expression profile analysis of susceptibility gene
The real-time quantitative PCR was adopted for miRNA genotype analysis. The total volume of the reaction system was 15 μL, including 5 μL of buffer, 0.5 μL of probe buffer, 0.5 μL of primer solution, and 3 μL of genomic DNA. The amplification conditions were set to pre-denaturation at 95°C for 10 minutes, operation for 5 seconds at 90°C, operation at 60°C for 30 seconds, extension at 75°C for 10 seconds, and it should be repeated for 30 cycles. After completion, the second-generation high-throughput sequencing platform was applied for miRNA genotype analysis.
2.8. Statistical methods
The data processing was analyzed by SPSS19.0 version statistical software, the measurement data was expressed by the mean ± standard deviation ( ± s), and the count data was displayed with the percentage (%). The physical and chemical properties of UK-LSA-CHT and LSA nanoparticles were compared by t-test. The data of UK-LSA-CHT, LSA, UK, and D0 groups were compared by analysis of variance. The result P < 0.05 indicated statistical significance, and Origin 8.5 was adopted for drawing.
3. Results
The theme of this study was to use the SGST to explore the application effects of nano-drug particles in the treatment of LEDVT and the relationship with related SGs. A rabbit model of deep vein thrombosis was undertaken as a research sample, medical nano-drug particles based on LSA materials were applied to the model treatment. The SGST was used to detect serum factors, SG expression profiles, and other indicators after model treatment, and the application value of nano-drug-loading technology in the treatment of LEDVT was analyzed from the molecular level.
3.1. Physical and chemical properties of nanoparticles
As shown in ) below, the average particle size (APS), width, ER, and DL of UK-LSA-CHT nanoparticles were obviously higher than those of LSA nanoparticles, and the differences were statistically significant (P < 0.05). ) showed the temperature that the UK-LSA-CHT nanoparticles of different concentrations could reach under 2 W/cm2 near-infrared light irradiation. It revealed that the D0 group can reach a maximum of 37.71°C within 15 minutes, and the 0.1, 0.5, and 1 mg/mL UK-LSA-CHT could reach a maximum temperature of 39.64°C, 42.31°C, and 46.85°C within 15 minutes. It suggested that as the concentration of UK-LSA-CHT nanoparticles increased, the temperature under infrared irradiation also increased greatly. showed the electron micrographs of UK-LSA-CHT nanoparticles under the field of view of 100 nm and 150 nm, which suggested directly that there were fatty acid coatings and regular textures on the periphery.
3.2. Cytotoxicity test
showed the changes in cell viability under different concentrations of UK-LSA-CHT nanoparticles. It suggested that the cell viability of the four groups all decreased to varying degrees over time, and the 100 mg/mL UK-LSA-CHT nanoparticles had the most obvious decline speed, but all were above 85%. gave the release of LDH, PGI2, and NO of different concentrations of UK-LSA-CHT nanoparticles. It indicated that the release of LDH and NO of the four groups of cells all showed a downward trend over time, of which the cells treated with 50 and 100 mg/mL UK-LSA-CHT had obvious lower LDH and NO release than 0 mg/mL UK-LSA-CHT nanoparticles after 12 hours (P < 0.05); and the PGI2 release of cells treated with 50 and 100 mL UK-LSA-CHT nanoparticles was higher greatly than that with 0 mg/mL UK-LSA-CHT nanoparticles 12 hours later (P < 0.05).
3.3. HE staining results of main organs for rabbits in four groups
The HE staining results of the heart, liver, spleen, lung, and kidney tissues of rabbits in each group given in clearly suggested that there were no inflammation damage and other pathological abnormalities in each organ. The HE staining results of the lung clearly showed many foam-like structures with different staining depths and various sizes; the bronchial epithelium was intact, and there was no obvious inflammatory cell infiltration around the bronchial tube wall and blood vessels. HE staining results of the liver revealed that the liver lobules were clearly demarcated, and the liver cells were in complete shape and arranged radially around the central vein, with no obvious damage except for more red blood cells. In the heart, the nucleus was blue, round or oval, and the cardiomyocytes were tightly connected and arranged in a neat manner. In the kidney, structures of renal tubules and glomeruli were visible, the renal tubules were slightly expanded, the intercellular substance was congested obviously, and there was no inflammatory cell infiltration. HE staining of spleen disclosed that the splenic corpuscles were normal, the capsule was thick and composed of dense connective tissue, smooth muscle fibers could be seen, and trabecula of different sizes could be observed in the splenic parenchyma.
3.4. Serological test results for rabbits of four groups
The serological test results were given in , which suggested that the expressions of ET-1, IL-6, and NF-κβ in the UK-LSA-CHT, LSA, and UK groups all showed a downward trend over time; the expressions of ET-1, IL-6, and NF-κβ of the rabbits in D0 group were always notably greater than those of the other three groups (P < 0.05); the expression of ET-1, IL-6, and NF-κβ in UK-LSA-CHT group was lower remarkably than that of LSA and UK groups 12 hours later (P < 0.05).
3.5. Immunohistochemical staining results in platelet activating factor of four groups of rabbits
As shown in below, the PAF expression in rabbit of UK-LSA-CHT group was lower notably than that of the LSA and UK groups (P< 0.05); and the PAF expression in D0 group was higher observably than that of the other three groups (P < 0.05). illustrated the immunohistochemical staining results in PAF in rabbits of every group. ) showed a darker coloration in yellow or brown, so it was deemed as positive expression of PAF, while , b, and c showed lighter colorations, most of which were blue, so they were determined as weakly positive or negative.
3.6. Analysis on SGrs1799983 expression profile in four groups
As shown in below, the allele frequencies of G, and GT and TT genotype ratios of SGN0S3 rs1799983 in the UK-LSA-CHT group were remarkably decreased in contrast to the LSA and UK groups (P < 0.05); and the allele frequencies of G, and GT and TT genotype ratios of SGN0S3 rs1799983 in D0 group were observably increased compared with the other groups (P < 0.05).
4. Discussion
LEDVT is a common but fatal complication of surgery. Because the thrombus in the blood is very unstable in the acute phase, it is easy to fall off and block the blood vessels at the distal end, causing the damage of a series of organ. The traditional antithrombotic drugs such as UK can treat the deep vein thrombosis, but they have certain side effects such as bleeding [Citation18,Citation19]. Therefore, the nanoprecipitation method was adopted to prepare the nanomedicine UK-LSA-CHT, and the physical and chemical properties of UK-LSA-CHT were analyzed. The results revealed that the APS, width, ER, and DL were higher observably than that of LSA nanoparticles (P < 0.05), which was similar to the results of Abouhussein et al. (2019) [Citation20]. Abouhussein et al. proposed a self-nano-emulsifying drug delivery system, and used the ternary phase diagram to optimize the thermodynamic stability, emulsification, dispersion, dilution robustness, in vitro dissolution, particle size, and zeta potential of the system. Finally, the experiment revealed that the nano drug delivery system showed strong anticoagulant effect in the prevention and treatment of thrombosis in the rat model. Such results indicated that UK-LSA-CHT nanoparticles had better DL performance. In addition, under infrared light irradiation, UK-LSA-CHT nanoparticles at 0.1, 0.5, and 1 mg/mL can reach a maximum of 39.64°C, 42.31°C, and 46.85°C within 15 minutes, respectively, which suggesting that UK-LSA-CHT nanoparticles could react responsively under the infrared light irradiation [Citation21]. From the point of view of cytotoxicity, the cell activity of the four groups all showed different degrees of decline over time, but they were all above 85%, which indicated that different concentrations of UK-LSA-CHT had low or no toxicity, so it could be applied in practical treatment [Citation22]. In addition, the LDH and NO release of cells treated with 50 and 100 mg/mL UK-LSA-CHT were greatly decreased in comparison with the cells treated with 0 mg/mL UK-LSA-CHT 12 hours later, but the release of PGI2 was increased highly (P< 0.05). Such results were different from the results of Wang et al. (2020) [Citation23]. LDH can stabilize the cell lysis process, PGI2 plays a role in vasomotor, and NO can have anti-platelet and anti-inflammatory effects. Thus, UK-LSA-CHT has good biocompatibility and safety.
HE staining results of heart, liver, spleen, lung, and kidney tissues revealed clearly that there were no inflammation damage and other pathological abnormalities in each organ, which further showed that the constructed nanomedicine system was safe and feasible [Citation24]. The expressions of ET-1, IL-6, and NF-κβ of the rabbits in the D0 group were always remarkably greater than those of the other groups, and the expressions in UK-LSA-CHT group was reduced in contrast to the LSA and UK groups after 12 hours (P< 0.05), which was in contrast to Molloy et al. [Citation25], who applied nanocapsules with high doses of eptifibatide to the treatment of thrombosis and tail hemorrhage in mouse models. It was found that eptifibatide nanocapsule delivery can selectively inhibit thrombosis in vitro is similar, which indicated that UK-LSA-CHT, LSA, and UK all had certain therapeutic effects on deep vein thrombosis, and the effect of UK-LSA-CHT nanomedicine was the best [Citation26]. The PAF expression of the rabbits in the UK-LSA-CHT group was improved greatly compared to the LSA and UK groups, and the PAF expression in the D0 group was the best among the four groups (P < 0.05). It was similar to the results of Galvano et al. [Citation27], who had proved that PAF could play a critical role in thrombosis, acute inflammation, anaphylactic shock, and other symptoms, indicating that the UK-LSA-CHT group was more effective in the treatment of deep vein thrombosis than other groups. The second-generation sequencing technology disclosed that the G allele frequency and GT and TT genotype ratios of SGN0S3 rs1799983 in the UK-LSA-CHT group were lower remarkably than LSA and UK groups, while those in the D0 group were the highest among all groups (P < 0.05), which indicated that allele T and G of NOS3 rs1799983 may be the key factor for occurrence of LEDVT.
5. Conclusion
In this article, the nanomedicine UK-LSA-CHT was prepared by using the nanoprecipitation method, and the rabbits were divided into a UK-LSA-CHT group, a LSA group, a UK group, and a D0 group according to different drug treatment methods. Cytotoxicity test, in vivo dissolution, serological test, and SG analysis showed that UK-LSA-CHT had good biocompatibility and safety, and had good therapeutic effects on LEDVT. Alleles G and T of N0S3 rs1799983 may be the key risk factors for the occurrence and development of LEDVT. However, this study had only conducted corresponding efficacy evaluations at the animal and cellular levels, and had not yet implemented any human thrombosis experiments. It was considered to select patient samples for further clinical observations. In short, the results of this article provided a theoretical basis for the substitution of clinical thrombolytic drugs.
Disclosure statement
No potential conflict of interest was reported by the author(s).
Data availability statement
All data, models, and code generated or used during the study appear in the submission.
Additional information
Funding
References
- Tang KC, Yang ZP, Zeng Q, et al. Effect of miR-495 on lower extremity deep vein thrombosis through the TLR4 signaling pathway by regulation of IL1R1. Biosci Rep. 2018 Dec 21;38(6):BSR20180598.
- Akin‑Bali DF, Eroglu T, Ilk S, et al. Evaluation of the role of Nrf2/Keap1 pathway‑associated novel mutations and gene expression on antioxidant status in patients with deep vein thrombosis. Exp Ther Med. 2020;20(2):868–881.
- Lu J, Fang Q, Ge X. Role and mechanism of mir-5189-3p in deep vein thrombosis of lower extremities. Ann Vasc Surg. 2021 Aug;17:S0890–5096(21)00492–1.
- Jiang YR, Niu LL, Feng N, et al. Correlation between the polymorphism of coagulation-related genes and lower extremity deep venous thrombosis. Fa Yi Xue Za Zhi. 2021 Apr;37(2):145–150.
- Ju S, Gao Y, Cao X, et al. Association between the lower extremity deep venous thrombosis, the warfarin maintenance dose, and CYP2C9*3, CYP2D6*10, and CYP3A5*3 genetic polymorphisms: a case-control study. Genet Test Mol Biomarkers. 2017 Sep;21(9):539–546.
- Zhang CL, Li ZM, Song ZH, et al. Coagulation factor V gene 1691G>A polymorphism as an indicator for risk and prognosis of lower extremity deep venous thrombosis in Chinese Han population. Medicine (Baltimore). 2018 Jun;97(22):e10885.
- Zhang L, Feng X, Zhang D, et al. Deep vein thrombosis in hospitalized patients with COVID-19 in Wuhan, China: prevalence, risk factors, and outcome. Circulation. 2020 Jul 14;142(2):114–128.
- Needleman L, Cronan JJ, Lilly MP, et al. Ultrasound for lower extremity deep venous thrombosis: multidisciplinary recommendations from the society of radiologists in ultrasound consensus conference. Circulation. 2018 Apr 3;137(14):1505–1515.
- Ren B, Yan F, Deng Z, et al. Extremely high incidence of lower extremity deep venous thrombosis in 48 patients with severe COVID-19 in Wuhan. Circulation. 2020 Jul 14;142(2):181–183.
- Houghton DE, Lekah A, Macedo TA, et al. Resolution of acute lower extremity deep vein thrombosis with rivaroxaban compared to warfarin. J Thromb Thrombolysis. 2020 Feb;49(2):199–205.
- Marturano A, Hendrickx ML, Falcinelli E, et al. Development of anti-matrix metalloproteinase-2 (MMP-2) nanobodies as potential therapeutic and diagnostic tools. Nanomedicine. 2020;24:102–103.
- Zhao S, Li Z, Huang F, et al. Nano-scaled MTCA-KKV: for targeting thrombus, releasing pharmacophores, inhibiting thrombosis and dissolving blood clots in vivo. Int J Nanomed. 2019;14:4817.
- Deng RH, Zou MZ, Zheng D, et al. Nanoparticles from cuttlefish ink inhibit tumor growth by synergizing immunotherapy and photothermal therapy. ACS Nano. 2019;13(8):8618–8629.
- Wu J, Zhu H, Zhao M, et al. IQCA-TASS: a nano-scaled P-selectin inhibitor capable of targeting thrombus and releasing IQCA/TARGD(S)S in vivo. J Mater Chem B. 2017 Feb 7;5(5):917–927.
- Zhao Y, Xie R, Yodsanit N, et al. Biomimetic fibrin-targeted and H2O2-responsive nanocarriers for thrombus therapy. Nano Today. 2020;35:100986.
- Huang M, Ji Y, Yan J, et al. A nano polymer conjugate for dual drugs sequential release and combined treatment of colon cancer and thrombotic complications. Mater Sci Eng C Mater Biol Appl. 2020;110:110697.
- Su M, Dai Q, Chen C, et al. Nano-medicine for thrombosis: a precise diagnosis and treatment strategy. Nanomicro Lett. 2020 Apr 20;12(1):96.
- Bonnard T, Jayapadman A, Putri JA, et al. Low-fouling and biodegradable protein-based particles for thrombus imaging. ACS Nano. 2018 Jul 24;12(7):6988–6996.
- Sun Q, Shi P, Lin C, et al. Effects of astragalus polysaccharides nanoparticles on cerebral thrombosis in SD rats. Front Bioeng Biotechnol. 2020 Dec;23(8):616759.
- Abouhussein DMN, Bahaa El Din Mahmoud D, Mohammad FE. Design of a liquid nano-sized drug delivery system with enhanced solubility of rivaroxaban for venous thromboembolism management in paediatric patients and emergency cases. J Liposome Res. 2019 Dec;29(4):399–412.
- Wang Y, Chen H, Zhang X, et al. Dimethyl 2, 2-[2, 2-(ethane-1,1-diyl)bis(1H-indole-3,2-diyl)]-diacetate: a small molecule capable of nano-scale assembly, inhibiting venous thrombosis and inducing no bleeding side effect. Int J Nanomedicine. 2018 Nov 22;13:7835–7844.
- Ma Y, Jiang L, Hu J, et al. Multifunctional 3D micro-nanostructures fabricated through temporally shaped femtosecond laser processing for preventing thrombosis and bacterial infection. ACS Appl Mater Interfaces. 2020 Apr 15;12(15):17155–17166.
- Wang D, Xu Y, Wang L, et al. Expanded poly(tetrafluoroethylene) blood vessel grafts with embedded reactive oxygen species (ROS)-responsive antithrombogenic drug for elimination of thrombosis. ACS Appl Mater Interfaces. 2020 Jul 1;12(26):29844–29853.
- Ellinghaus E, Ellinghaus D, Krusche P, et al. Genome-wide association analysis for chronic venous disease identifies EFEMP1 and KCNH8 as susceptibility loci. Sci Rep. 2017;7:45652.
- Molloy CP, Yao Y, Kammoun H, et al. Shear-sensitive nanocapsule drug release for site-specific inhibition of occlusive thrombus formation. J Thromb Haemost. 2017 May;15(5):972–982.
- Deng Q, Zhang L, Lv W, et al. Biological mediator-propelled nanosweeper for nonpharmaceutical thrombus therapy. ACS Nano. 2021 Apr 27;15(4):6604–6613.
- Ma H, Jiang Z, Xu J, et al. Targeted nano-delivery strategies for facilitating thrombolysis treatment in ischemic stroke. Drug Deliv. 2021 Dec;28(1):357–371.