ABSTRACT
In the present era of global climate change, the scarcity of potable water is increasing both due to natural and anthropogenic causes. Water is the elixir of life, and its usage has risen significantly due to escalating economic activities, widespread urbanization, and industrialization. The increasing water scarcity and rising contamination have compelled, scientists and researchers, to adopt feasible and sustainable wastewater treatment methods in meeting the growing demand for freshwater. Presently, various waste treatment technologies are adopted across the globe, such as physical, chemical, and biological treatment processes. There is a need to replace these technologies with sustainable and green technology that encourages the use of microorganisms since they have proven to be more effective in water treatment processes. The present review article is focused on demonstrating how effectively various microbes can be used in wastewater treatment to achieve environmental sustainability and economic feasibility. The microbial consortium used for water treatment offers many advantages over pure culture. There is an urgent need to develop hybrid treatment technology for the effective remediation of various organic and inorganic pollutants from wastewater.
HIGHLIGHTS
Microbial engineering approaches for wastewater treatment.
Current and emerging sources of water pollution are discussed.
Various treatment technologies for wastewater treatment.
Biological methods and microbes are used for degradation.
Parameters responsible for the degradations processes of wastewater.
GRAPHICAL ABSTRACT
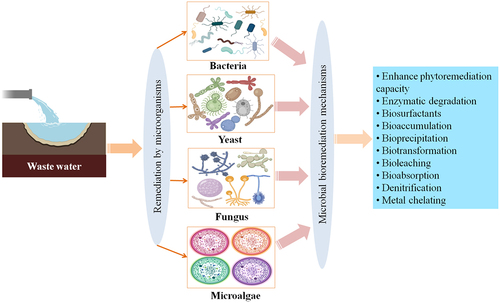
1 Introduction
World water consumption has almost doubled in a few decades [Citation1]. The growing concerns over water contamination have led to extensive research and development in water treatment techniques. They are expanding to promote the reuse of water and improve the quality of water for human consumption. Water pollution is a worldwide issue that presents a severe threat to the survival of all life forms. Aquatic pollution can be caused by organic and inorganic impurities and microbiological contaminants. Population growth, industrial and mining activities, sewage and wastewater, radioactive waste, chemical fertilizers, pesticides, urban development, and other anthropogenic sources are all responsible for rising levels of aquatic pollution. Water quality is determined by the concentrations of particles and chemicals in water, such as heavy metals, nutrients, microorganisms, polycyclic aromatic hydrocarbon (PAH), and other pollutants. Many organic contaminants are endocrine-disrupting chemicals associated with testicular, prostate, and breast cancers. They can also cause serious complications in human and animal reproductive health, such as sperm count reduction in males and the production of fragile eggs in females, among other things [Citation2].
Wastewater treatment has evolved as a feasible technique for tackling water scarcity and protecting the ecosystem from the harmful impacts of polluted/wastewater in the contemporary environment [Citation3]. Many countries have passed stricter laws for treating sewage water before dumping it into water bodies. From sustainability, improved water management and wastewater recycling have begun to get active attention [Citation4]. Many physical and chemical processes (flotation, precipitation, oxidation, adsorption, etc.) used for wastewater treatment are expensive, demand high maintenance, and require a complicated functional setup. As a result, there is an urgent necessity to move to green and sustainable technologies such as microbial wastewater treatment to serve as a long-term alternative to traditional wastewater treatment methods [Citation3]. Wastewater treatment using microbes such as fungi, bacteria, microalgae, and others has caught the researcher’s attention in recent years. The presence of a significant amount of nutrients such as nitrogen, phosphorus, and carbon in wastewater emanating from different sources can aid in the growth and survival of these microbes [Citation5].
Phytoremediation is frequently used to remediate wastewater. On the other hand, excessive salts in wastewater can induce plant toxicity. To solve the problem, Sarawaneeyaruk et al. [Citation6] isolated multifarious plant growth-promoting bacteria (PGPB) Bacillusspp from the municipal wastewater and by using this PGPB they successfully enhanced plant growth under municipal wastewater irrigation. Hence, such green technology would be sustainable and help maintain a balance between socio-economic and environmental perspectives [Citation3].
Food, water, and energy are interconnected and wastewater is crucial in this nexus. Although various wastewater treatment methods are available the aim of this review is to draw attention on the importance of microbes in wastewater. The goal of this review is to help in protecting water resources using effective treatment method. This review illustrates the wastewater treatment process by utilizing microorganisms (bacteria, fungi, microalgae, and yeast), highlighting the advantages and applications of microbes over other conventional approaches. This review further aims to provide useful information to researchers working in relevant fields worldwide to pique their interest in using microbes to improve and cost-effectively treat wastewater ().
Table 1. Categories of water pollutants and their probable effects are tabulated as.
2 Emerging sources of water pollution
A variety of organic contaminants can be found in water, including insecticides, herbicides and organohalides [Citation7]. Industrial effluents contain inorganic pollutants such as silt from stormwater runoff and heavy metals from acid mine drainage. Different sources of domestic waste enter water bodies. Pesticides used in gardens and lawns may also enter the water bodies [Citation8]. Cleaning products, detergents, and skincare products contain significant amounts of pollutants that can pollute water bodies and make them unfit for human consumption [Citation9]. Chemicals and acids from industries like steel and paper are discharged into rivers [Citation10]. Water bodies receive over 70% of industrial waste, containing many toxins [Citation8]. Major agricultural wastes are fertilizers, pesticides (herbicides, insecticides), and other agrochemicals. Fertilizer production continues to rise year after year to increase productivity, resulting in increased waste generation. Irrigation contributes significantly to surface water pollution in China and is also a cause of nitrogen groundwater pollution in the United States [Citation9]. Toxic chemicals can accumulate in the body and eventually reach toxic levels, causing food chain disruption. Another major source of water pollution is nutrient enrichment.
3 Emerging technologies for wastewater treatment
Physical treatment of wastewater entails removing contaminants from the water without affecting the biochemical properties of contaminants. Physical treatments usually follow chemical and biological treatments. Screening, Flotation, Flow equalization, Membrane-based technology, Thermal treatment, and other physical treatment techniques are commonly used. Some of the common chemical unit processes used in wastewater treatment technology include precipitation, adsorption, disinfection, chlorination, neutralization, chemical exchange, etc. [Citation14] to bring changes in the quality of water.
3.1 Biological treatment for the wastewater
Biological treatment involves removing contaminants from wastewater using biological organisms or processes. Microbes are critical to wastewater treatment and reclamation, making them a promising green technology tool. The biological treatment uses bacteria, fungi, microalgae, yeast, and other microbial groups. Biological treatments are less expensive than physical and chemical treatments [Citation15]. Among the most widely used biological wastewater treatment methods are:
Table 2. Showing advantages of biological methods in wastewater treatment [Citation17].
3.1.1 Activated sludge method
One of the most commonly used biological processes in wastewater treatment to date is the activated sludge. It has been effectively used to treat industrial and municipal wastewater. This process uses a biological floc that consists of bacteria and protozoa under aerobic conditions [Citation16]. The basic principle behind all activated sludge processes is that microbes grow within metabolizing organic material where they form clumps. The oxidizable matter is used as food for the microorganisms forming a suspended floc in the wastewater. The aeration/agitation provides the continued oxygen supply. The mixed liquor, which is the mix of wastewater and activated sludge, is allowed to settle down to segregate the activated sludge solids from treated wastewater while a part of the settled activated sludge is returned to the aeration site. The entire activated sludge process comprises interlinked elements such as an aeration tank, source of aeration, clarifier, and a collection system. The biological reaction occurs in an aeration tank fitted with a stirrer for mixing and source of oxygen, which is connected to a tank/clarifier where the settled solids are segregated from treated water along with a collection loop which either returns the activated sludge back to the aeration tank or is removed from the process. The process has high efficiency and can also be used for nutrient removal [Citation18].
The activated sludge process is used in the treatment of industrial wastewater as well as domestic. In spite of having advantages like low operational cost with added treatment efficiency, the major drawback is the generation of excess quantity of waste activated sludge. The organic matter generated by the process needs to be properly treated and managed to reduce the ecological and financial burden [Citation19].
3.1.2 Bioreactors and biofilters
Using physical retention and microbial biodegradation, membrane bioreactors eliminate pollutants from wastewater [Citation20]. Biofilters use biological processes to filter wastewater [Citation21]. It grows on top of the media, which is composed of gravel, sand, and ceramic. For example, a biofilm can contain a microbial (bacterial) community that helps to decompose organic content in water [Citation37]. This process has been extensively used to remove H2S from municipal wastewater, according to Zhang et al. [Citation38].
3.1.3 Biosorption
Certain biological molecules naturally can accumulate metals like copper, zinc, nickel, chromium, palladium from wastewater [Citation39]. The process of biosorption is complex and involves various interactive mechanisms like ion-exchange, absorption, precipitation, and complexation through the participation of functional groups like hydroxyl, carbonyl, etc. [Citation40]. It is a reversible process that involves interactions rather than oxidation to bind the biosorbent in an aqueous solution.[Citation41]
The biosorbent is suspended in a contaminant solution (e.g. metal ions). After a while, contaminant-rich biosorbent can be separated. Microbes immobilize on adsorbants to form a biosorbent that captures contaminants [Citation42].
Agricultural waste, microbial biomasses, industrial-by-products offer advantages over chemical methods in terms of efficiency, large abundance and low cost [Citation43]. Factors like pH, concentration of the metals, ionic strength, other pollutants present in wastewater, temperature, etc., effect the process of biosorption [Citation44]
4 Different microbial groups in wastewater degradation
Microbial treatment can be used instead of traditional wastewater treatment methods because it is cheaper, more efficient, and more competent [Citation45]. Bioremediation involves bacteria, fungi, microalgae, yeast, etc. [Citation46]. These microbes are responsible for degrading or converting contaminants into lesser harmful products [Citation47]. They have become ideal bioagents of remediation owing to their high surface area-to-volume ratio, small size, and substantial surface area [Citation48]. These microbes use biosorption and bioaccumulation to bioremediate. Adsorption occurs when pollutants (metals) interact with functional groups on the cell surface [Citation49]. Biosorption can use both live and dead biomass. Bioaccumulation involves intracellular and extracellular processes. Toxins bioaccumulate when they are absorbed from the environment. Bioaccumulation uses only living biomass, limiting its reuse, and costing more than biosorption [Citation50].
4.1 Bacterial removal of organic and inorganic pollutants
The treatment of wastewater effluents is based on the capability of bacterial cells to concentrate pollutants (metals). The microbial population and xenobiotic content determine the rate of biodegradation. Plants feed rhizosphere microbes’ organic carbon, which helps degrade pollutants. Aquatic plants’ biofilms can degrade organics like phenols, amines, and aliphatic aldehydes [Citation51]. Methanotrophs use methane to obtain carbon and energy and break down various harmful organic compounds [Citation52]. Eichhornia crassipes can help clean up eutrophic water by influencing nitrogen production [Citation53]. Tolypothrixceytonica and Anabaena oryzae have also been shown to be effective in treating industrial wastewater [Citation54]. Aphanocapsu sp. and Plectonema sp. have the ability to degrade crude oil [Citation55]. Anaerobic bacteria in sewage treatments include sulfate-reducing bacteria like Desulfovibrio, Desulfotomaculum, Desulfobacter, and Desulfococcus genera [Citation56].
The factors like abundance, size, growth under controlled conditions and resistance to environmental changes have marked bacteria as important biosorbents [Citation57]. Metal ion biosorption into the cell wall can be active or passive. Passive biosorption occurs in both living and dead/inactive bacterial cells [Citation58]. Active biosorption includes metal ion uptake within living bacterial cells. Metal ion binding involves ion exchange, chelation, complexation, and micro precipitation [Citation59].
4.1.1 Dye degradations
Synthetic dyes have many advantages over natural dyes in terms of color variety, speed of coloration, absorption and water solubility [Citation60], which explains the global dye production of 800,000 tons per year [Citation61]. The impact of textile effluents on the overall health of the aquatic ecosystem is growing in concern as dye demand and production rise. Textile wastewater contains inorganic and organic additives and chemicals [Citation62] as well as dyes [Citation63] in concentrations ranging from 10 to 200 mg/L. In textile industries, the azo dyes (70%) are commonly used because of their low cost and ease of use. Since all dyes do not fix to fabrics during dyeing, unfixed dyes are washed out and found in high concentrations in effluents [Citation64]. Bacterial-assisted dye degradation is nontoxic and can decolorize colored complex dyes. lists some studies on bacterial dye degradation.
Table 3. Shows the bacterial degradation of dyes and the mechanism involved.
Aeromonas hydrophila, Bacillus subtilis, Bacillus cereus have been studied effectively and have the potential for bioremediation of azo dyes [Citation65]. Under anoxic conditions, Pseudomonas sp., Pseudomonas luteola, Proteus mirabilis have the ability to degrade azo dyes [Citation66]. These bacteria utilize oxidoreductive enzymes for dye degradation. Aerobic bacteria use oxygen-catalyzed azoreductase to break the azo bonds [Citation67]. Some bacterial strains degrade dyes in aerobic conditions and use mono and dioxygenase for oxidizing the aromatic ring of organic compounds [Citation68]. Anaerobic bacteria use the enzyme azoreductase to degrade azo dyes. And generally, anaerobic conditions favor decolorization (Chang et al., 2001b). Mostly first-order kinetics is followed with respect to the concentration of the dye in the decolorization reaction and in some; zero-order kinetics is also seen [Citation69]. The oxidoreductive enzymes also are involved in hydroxylation, desulfonation, and deamination. Pseudomonas aeruginosa could decolorize various azo dyes [Citation70] and Navitan Fast Blue S5R under aerobic conditions.
4.1.2 Petrochemicals degradation
Petroleum hydrocarbons are divided into resins, asphaltenes, aromatics, and saturates [Citation79]. Their degradation by microbes () is complex, dependent on the nature and number of hydrocarbons available. The biodegradation of hydrocarbons is determined by agents such as temperature and concentration of inorganic nutrients such as phosphorus, nitrogen, and iron in some instances [Citation80]. The vulnerability of hydrocarbons to attack by microbes is different, with linear alkanes being most susceptible and cyclic alkanes the least [Citation81,Citation82]. Polycyclic hydrocarbons having higher molecular weight might not be degraded [Citation83]. Acinetobacter sp. degrades n-alkane having chain length C10-C40, utilizing carbon as the sole source [Citation84]. Mycobacterium, Burkholderia, Gordonia, Brevibacterium, Dietzia, Aeromicrobium, Pseudomonas, Aeromonas, Flavobacteria, Nocardia, Modococci, Chrobacteria, Moraxella, Cyanobacteria, Streptomyces, Bacilli, Arthrobacter, and other bacteria can degrade petroleum products [Citation85]. The poly-aromatic hydrocarbons could be degraded by Sphingomonas [Citation86]. The biodegradation efficiency of soil bacteria [Citation87] and marine bacteria [Citation88] are not the same. The microbes utilize specific enzymes systems (oxygenase, peroxidase, and hydroxylase) in degrading the petroleum hydrocarbons in aerobic conditions, and it starts with the attachment of microbial cells onto the substrates and is followed by the production of biosurfactants [Citation89,Citation90]. Biosurfactants are synthesized by various microorganisms () and are heterogeneous surface-active compounds. Biosurfactants are involved in enhancing the solubility and, finally, the removal of the contaminant [Citation91]. They augment the surface area and the amount of oil available for the bacteria to utilize [Citation92] and decrease surface tension to help form micelles.
Table 4. Biosurfactants produced by the various microbes for degradation of petroleum hydrocarbons.
4.1.3 Pharmaceutical and personal care products
Pharmaceuticals and personal care products (PPCPs) are emerging persistent pollutants [Citation93]. Pharmaceuticals have increased steadily globally [Citation94], especially since the COVID-19 pandemic. Individuals contribute PPCPs to the aquatic ecosystem () by using sanitizers, shampoos, household cleaners, detergents, and medicines. PPCPs are complex and persistent molecules that reenter the hydrologic cycle, increasing antibacterial resistance, reproductive abnormalities, and tumor growth. These unregulated pollutants persist in water bodies, and many metabolites are converted back to their parent form [Citation95]. They precipitate specific pollutants into complex and toxic forms that easily spread in aquatic phases. shows the circulation of PPCP in the surrounding [Citation96]. The breakdown of PPCPs by microorganisms is difficult because pharmaceuticals were designed to be toxic to bacteria [Citation97]. Nonetheless, some native bacterial species can help degrade pharmaceutical pollutants [Citation98]. Microbes reduce or degrade the complex structure to a nontoxic or less toxic form.
Figure 2. Representative diagram of pharmaceuticals and personal care products circulating in the environment adopted from [Citation73].
![Figure 2. Representative diagram of pharmaceuticals and personal care products circulating in the environment adopted from [Citation73].](/cms/asset/9d48491c-ce00-46fe-b13f-b32de0f1644d/kbie_a_2184518_f0002_oc.jpg)
4.1.4 Pesticides
Pesticides are chemicals utilized to kill pests and are classified based on their functions (herbicides, algicides, fungicides, bactericides, nematicides, rodenticides, and insecticides) [Citation99]. Chemical classes of organic pesticides include organophosphorus, organochlorine, carbamates, acetamides, neonicotinoids, pyrethroids, triazoles, and triazines. Inorganic pesticides include lead arsenate, and boric acid complexes, etc. Organochlorine compounds like chlordane, DDT, toxaphene, and heptachlor have been included in the list of persistent organic pollutants [Citation100,Citation101].
It has been reported by many researchers that effectively, less than 5% of total used pesticides are involved in targeting the pests while the rest of them are precipitated in the surrounding water and soil [Citation102]. The pesticides left in the ecosystem have a detrimental effect on the ecosystem [Citation103] and need to be removed. The chemical and physical methods of pesticide removal are unsustainable [Citation104], hence bacteria could function as bio-weapon to fight toxic agricultural chemicals [Citation105]. Various studies have elaborated on the role of bacteria in bioremediating pesticides, viz. Endosulfan removal by Bacillus and Staphylococcus [Citation106]; Malathion removal by Arthrobacter sp., Pseudomonas putida [Citation107]; Ridomil and Fitoraz removal by Pseudomonas putida and Acinetobacter sp. [Citation108]; Napthelene removal by Cyanobacteria [Citation109]; Endosulfan removal by Staphylococcus aureus, Achromobacter sp., Rhodococcus sp. [Citation110]; Malathion, Ridomil and Fitoraz removal by Pseudomonas putida, Rhodococcus and Arhtrobacter sp. [Citation111].
4.1.5 Heavy metals degradation
Heavy metals such as lead (Pb), cadmium (Cd), chromium (Cr), arsenic (As), and mercury (Hg) are ubiquitous environmental pollutants, having high toxicity and density. Natural and anthropogenic sources of heavy metal pollution cause detrimental effects on all living beings [Citation112]. The microbial cells require cations for numerous cellular activities, but increasing concentration may retard growth by forming internal complexes [Citation113]. Bacteria have the ability to immobilize and also mobilize, transform and uptake heavy metals [Citation114]. Many studies have been published on the role of endophytic bacteria in heavy metal bioaccumulation and detoxification [Citation115,Citation116]. These studies show that bacteria secrete organic acids to help with the bioremediation process. Bacteria also produce biosurfactants released as root exudates and increase metal bioavailability in aquatic environments [Citation117]. It was found that glutathione was involved in the intracellular sequestration of cadmium ions in the cells of Rhizobium leguminosarum [Citation118]. Heavy metals can be reduced to less or nontoxic metals by iron-reducing bacteria like Geobacter sp. and sulfur-reducing bacteria like Desufuromonas sp. Sulfate-reducing bacteria and metal-reducing bacteria, for example, can convert chromium from the highly toxic Cr (VI) to the less toxic Cr (III) [Citation119]. Sulfate-reducing bacteria produce a lot of hydrogen sulfide, which causes metal cations to precipitate [Citation120]. Vibrio harveyi strain could precipitate the divalent lead as a salt of lead phosphate [Citation121]. Many ionizable cell wall groups can help bacteria absorb metal ions (amino, carboxyl, phosphate, and hydroxyl gp). In metal remediation, microbial methylation is also important. For example, Bacillus sp., Clostridium sp., Pseudomonas sp., and Escherichia sp., can biomethylate Hg (II) [Citation122]. Various heavy metals respond to the microorganism differently depending on the conditions. Some bacterial cells produce siderophores, and they form metal complexes, limiting their bioavailability and removing their toxicity [Citation123]. Some of the bacterial species involved in the bioremediation of heavy metals () have been tabulated.
Table 5. Some Bacterial species used for the removal of heavy metals.
4.2 Fungi and yeast
Fungi can help in the removal of pollutants (heavy metals) by increasing their bioavailability and converting them to lesser toxic forms [Citation124]. Fungi are simple to grow and produce a significant amount of biomass. Several fungal strains have shown the ability to digest a variety of environmental contaminants, including dyes, pharmaceutical drugs, aromatic hydrocarbons, and heavy metals [Citation125,Citation126]. The two important characteristics of fungi that make them an ideal candidate for wastewater treatment are the secretion of many extracellular enzymes [Citation127] and the hyphal mesh of fungi that protects the internal sensitive organelles from the ill effects of contaminants. Fungi are drawn to the rhizosphere by root exudates. Many factors influence plant-fungi interactions in the rhizosphere, including soil characteristics, plant species, water type, climate, and other microorganisms [Citation128]. Plant-fungi interactions perform a variety of important functions, including metal-chelating siderophores emission, denitrification, and detoxification (). The organic wastes are transformed into industrially important biochemicals and other valuable compounds by fungi, which is an advantage of using fungal culture in wastewater treatment over bacterial culture (proteins, organic acids). Animal feed can also be made from fungal biomass [Citation129]. Pleurotus pulmonarius, Stachybotrys sp., Cephalosporium aphidicola, Aspergillus parasitica, Verticillum terrestre, Candida sp., Acremonium sp., Glomus sp., Minimedusa sp., Talaromyces, Hydnobolites, Peziza, and other fungal species can be used in wastewater treatment [Citation130]. shows the effective fungal degradation of wastewater.
Figure 3. A schematic diagram of wastewater treatment by microorganisms with their different bioremediation mechanisms.

Table 6. Some common fungal species used in pollutant remediation in wastewater.
Over the last years, many research studies have suggested the effective role of ligninolytic fungi in degrading synthetic dyes [Citation131]. Interestingly, fungi possess ligninolytic enzymes that degrade complex dyes, including laccase, manganese peroxidase, and lignin peroxidase. Some research studies reflecting fungi’s role in degrading dyes have been elucidated in .
Table 7. Shows the degradation of some dyes by fungi along with the mechanism involved.
Many studies have shown that yeast can be used to eliminate pollutants (heavy metals) from the environment [Citation132]. Yeast can also help reduce COD levels and remove mono and polyphenols [Citation133] because yeast can absorb, accumulate, and degrade toxic compounds into nontoxic forms. It can be used to treat textile wastewater. Saccharomyces cerevisiae, Galactomyces geotrichum, Trichosporon beigelii, and Candida krusei can degrade dyes in wastewater [Citation134].
4.3 Microalgae
These include the use of eukaryotic algae and cyanobacteria for biological wastewater treatment [Citation135]. The term ‘phycoremediation’ refers to the use of algal species for bioremediation. Chlorella sp., Picochlorum sp., Tetraselmis sp., Scenedesmus sp., and other algal and cyanobacterial strains like Anabaena sp., Oscillatoria sp., Spirulina sp., Chroococcus sp., Pseudospongiococcus sp., Scytonema sp., Dolichospermum [Citation136] are used in wastewater treatment. Microalgae have the following characteristics that make them an ideal candidate for wastewater treatment:
Capability to utilize both inorganic and organic carbon, nitrogen, and phosphorus present in wastewater for growth [Citation137].
The life cycle is short and requires less nutrients [Citation164].
Scope of re-using algal biomass through adsorption/desorption mechanism [Citation165].
The growth of algal biomass is independent of environmental conditions, hence can be produced throughout the year [Citation166].
The efficiency of algal biomass is better than membranes to remove heavy metals [Citation167].
Source of Oxygen and helps in degradation process by heterotrophic bacteria [Citation168].
Useful in both anaerobic and aerobic effluent treatment plants [Citation169].
Depending on the nutrient source, capital investment, and culture conditions (biofuels, CO2 capture), microalgae culture-based wastewater treatments can be open or closed.
4.3.1 Open type
Algae are grown in open systems in places like ponds, lagoons, and deep channels. Natural (ponds, lagoons) or artificial (man-made ponds, tanks, containers) sites can be used. For domestic and industrial wastewater treatment, stabilization ponds containing bacteria and microalgae culture are most commonly used in temperate and tropical climates. Many studies have demonstrated the effective use of open microalgal cultured treatment plants in treating wastewater [Citation170].
4.3.2 Closed systems
Microalgae is grown in closed environments in such systems. Photobioreactors are one example of such a system. Reduced water evaporation, higher biomass yield, and contamination elimination are all advantages of closed-type treatment over open type [Citation171]. A pilot-scale tubular bioreactors are used to grow a diverse range of microalgae, including Arthrospira sp., Chlorella sp., Haematococcus sp., Spirulina and Phaeodactylumsp [Citation172].
Algal biosorbents have a high sorption capacity [Citation173]. Using algae-based biosorption, heavy metal ion extraction from wastewater could be an environmentally friendly, cost-effective, and efficient method [Citation174]. Textile wastewater contains algae cultivation nutrients (phosphates, nitrates, micronutrients, etc.) as well as organic dyes [Citation175]. Many studies () have shown that microalgae can remove pollutants from wastewater; for example, C. vulgaris and S. quadricaudacan remove nitrate [Citation190]; Chlorella sp., Scenedesmus sp., Cosmariumsp. for wastewater treatment [Citation191]; Chlorella sp., Scenedesmus sp., Cosmarium sp. for treatment of wastewater (aquaculture wastewater and textile wastewater [Citation192]. According to Ojha et al. [Citation207], C. vulgaris and S. quadricauda cultures can be used for wastewater remediation.
Table 8. Some microalgae species used in wastewater treatment.
Organic dyes are major pollutants in water. They can be found in many manufacturing industries, including textiles, plastics, and medicines. These dyes, when accumulated in aquatic systems, results into eutrophication and limited reoxygenation capacity. The production of poisonous amines during the decomposition of dyes is one of the most serious concerns [Citation208]. Microalgae and Cyanobacteria represent a possible option for the bioremediation of wastewater. Microalgae decolorize dyes by adsorption or degradation. Microalgae can make use of wastewater dyes and nutrients. During the bioconversion process, microalgae can consume the dyes as a source of carbon and convert them to metabolites. The degradation of dyes by microalgae has been elucidated through some research studies in ().
Table 9. Shows the degradation of some dyes by microalgae along with the mechanism involved.
5 Factors affecting microbial biodegradation
Microbes can degrade various physical and chemical wastes through removal, alteration, immobilization, or detoxification. Microbes play a role because of their enzymatic pathways. Many factors influence bioremediation efficiency, including soil type, temperature, pH, oxygen, and other electron acceptors, nutrients, biological factors, and so on.
5.1 Environmental determinants
Temperature is the most important of all the physical factors that influence microorganism survival [Citation209]. Microbial enzymes involved in biodegradation require the right temperature to metabolize substances. The rate of microbial activity increases as the temperature rises and peaks at the optimum temperature. The temperature of water influences various processes such as mineralization, diffusion, and chemical reactions [Citation210]). Temperature extremes can kill bacteria and other microbes, affecting their growth [Citation211]. Increases in temperature within the optimum range raise the reaction temperature, thereby increasing the solubility of contaminants, improving diffusion, and so on. The bacterial consortium of Bacillus pumilus HKG212 and Zobellella taiwanensisAT was used by [Citation212] to degrade reactive green 19, and their findings revealed that the highest degradation occurs at 32.04°C.
The measurement of pH indicates microbial growth potential [Citation224]. The pH range determines the survival of bacterial species, and thus bioremediation. Acidophilic, neutrophilic, and alkaliphilic biodegrading bacteria require acidic, neutral, and basic media for optimal activity [Citation225]. According to [Citation226], the pH of the affected site can be changed to achieve the desired biodegradation results. At pH 4.5, they were able to degrade malachite green by 98% using RuO2-TiO2,and Pt coated Ti mesh electrodes.
Moisture has an impact on the rate of biodegradation because moisture affects the content and concentration of soluble materials available, as well as the osmotic pressure and pH of aquatic systems.
Different microbes require different oxygen levels, such as aerobic, anaerobic, and semi-anaerobic conditions. In most cases, the presence of oxygen can help with hydrocarbon metabolism. Some contaminants, such as petroleum hydrocarbons in wastewater, inhibit bacterial growth by reducing compressed oxygen and electron acceptors [Citation227]. Although shaking can improve oxygenation, delivering enough oxygen for the biodegradation of organic pollutants is a part of an operational issue and is costly [Citation228].
An optimum quantity of nutrients and other chemicals is important for microbial metabolism [Citation229]). Additional input of nutrients changes the nutrient balance for microbial growth, affecting the rate and effectiveness of biodegradation [Citation230]. Microbes require various nutrients, including carbon, nitrogen, and phosphorus, to survive and continue their metabolic activities [Citation231]. Varjani et al. [Citation232] identified phosphorus as a critical factor in microbe growth.
5.1.1 Contaminant concentration
The type and number of contaminants can have an impact on biodegradation. A high biodegradation rate can be achieved by increasing the contaminant concentration [Citation233]. Heavy contaminants, such as oil petroleum-containing wastewater, have been fatal to the microbial community and negatively impact their biocatalytic activity. Low molecular weight contaminants with simple structures can achieve a high bioremediation rate [Citation234]. Kerosene, for example, can be completely biodegraded at optimal concentrations due to its simple structure and low molecular weight [Citation235].
5.1.2 Salinity
According to [Citation236] organic pollutants present in wastewater which contain alkaline chemicals biodegrade very slowly due to their ability to persist in waste. Contaminants with a high salt content may reduce biodegradation activity by inhibiting biological movement [Citation237].
5.2 Biological factors
Biological factors influence the breakdown of organic pollutants as microorganisms compete for limited carbon sources, and antagonistic interactions between microbes exist. Major biological factors that affect the bioremediation activity of microbes include enzyme activity, interactions (competition, succession, and predation), population size and composition, mutation, etc. [Citation238]. The rate of biodegradation is dependent on the substrate as well as the biocatalyst [Citation239] and the specificity of the enzyme. Inhibition of enzymatic activities due to several factors like competition for carbon and nutrient sources can affect the biodegradative activity of microbes [Citation240].
6 Microbial consortium: emerging technology
The microbial consortium is the emerging biotechnology-based green approach. Using a single microbe strain to treat wastewater may not give effective results, and efficiency can be compromised. Thus, many research findings have proposed applying microbial consortia [Citation241,Citation242]. Consortia comprising different groups of environmental microbes capable of degrading pollutants in wastewater can be an effective choice. Such consortiums have many advantages over the application of a single strain like fast removal, assistance in secondary application of treated wastewater, along with promoting ecological sustainability.
In the natural habitat, biofilm is formed by aggregating different groups of microbes attached through exopolymeric substances. The whole system is synergistic with microbial partners’ contributing toward forming a strong community [Citation243].
The development of consortia is an emerging approach for wastewater treatment. The algal-bacterial consortium has many advantages owing to its biomass refiniability and reduced power consumption [Citation244]. The fundamental principle in the microbial community is utilizing beneficial relationships which are promoting in pollutant removal from wastewater. The synergy is observed in the relationship wherein bacteria are involved in BOD removal, and algae remove nitrogen and phosphorus by absorption [Citation245]. The relationship established between algae and bacteria provides a suitable ground for bioremediation [Citation246]. Photosynthesis is undertaken by cyanobacteria bacteria and converts inorganic carbon present in wastewater to organic carbon [Citation247]. The CO2 produced by bacterial oxidation serves as the carbon source for photosynthetic algae. Decomposers like Acinetobacter can remove BOD and oxidize organic carbon sources into CO2, which serves in algae growth [Citation248].
Extensive research findings have supported microbial consortium as a potential candidate for wastewater treatment [Citation249]. Recent experimental studies conducted by [Citation250] revealed the application of Ecobacter bacterial consortium facilitated the bioaugmentation for the biological removal of nitrogen compounds; showing ammonium was transformed by the microorganism reduction reaction; thus, presented decrease in the concentration of ammonium at the end of the treatment period. In their studies, Qi et al. [Citation251] proved effectively that a well-established microbial (algal-bacterial) consortium in the phycosphere can be optimized and used in advanced wastewater treatment. The results of the research conducted by [Citation252] showed that treatment of paper pulp wastewater by microbial consortium between microalgae and bacteria allowed good efficiency in removal of organic matter and nutrients. Rehman et al. [Citation253] studied microbial consortium with Klebsiella sp. LCR187, Bacillus subtilis LOR166, Acinetobacter sp. BRS156 and Acinetobacter junii TYRH47 and Typhadomingensis and Leptochloafuscato treat oil field wastewater. Tara et al. [Citation254] reported greater than 90% removal efficiency of pollutants from textile wastewater using microbial consortium. Leong et al. [Citation255] reported 94% pollutant removal efficiency from municipal wastewater using microalgae consortium with bacteria. Microbes carry out the degradation through the secretion of various enzymes and organic acids [Citation256]. Monica et al. [Citation257] used Effective Microorganism (EM), which comprises Lactobacillus, Aspergillus, Pseudomonas, Streptomyces, and Saccharomyces, for biodegradation of sewage load in the water. Lactobacillus does the breakdown of lignin and cellulose in this consortium. Pseudomonas releases bioactive compounds which act on sewage and detoxifies or precipitate the metal. Aspergillus decomposes organic matter rapidly, producing alcohol and esters. shows the effective utilization of consortia between microbes for treating wastewater from various sources.
Table 10. Microbial consortium for treatment of wastewater.
7 Conclusion
Water contamination from various sources has become a serious problem around the world. The use of microbes as a treatment for water pollution is a viable alternative. Microbial remediation is an evolutionary and revolutionary technique for wastewater treatment that is currently in use. Microbes (bacteria, fungi, algae, and yeast) are naturally occurring and thus offer a long-term solution to the problem of water pollution. Lack of appropriate information about microbes’ metabolic capacity to degrade contaminants and a lack of controlled conditions such as temperature, pH, the appropriate number of contaminants, nutrients, and more time consumption are all possible limitations with the use of microbes in the treatment process. If the process is not controlled, contaminants may not be completely degraded, resulting in toxic byproducts. As a result, appropriate inside characterization can be an effective way to overcome the drawbacks of microbial-assisted wastewater remediation. Success in microbial wastewater treatment can also be attributed to advances in genetic engineering. Engineered microbial strains with high metabolic potential and well-understood detoxification pathways will undoubtedly aid in combating the wastewater threat to the greatest extent possible. Although several studies have been performed on the use of microbial consortium like microalgae-bacterial systems for the treatment of waste water, but still there is a need for further research in optimizing parameters for large-scale units. Maintaining the stability of the consortium is the main challenging task. More emphasis should be placed on some parameters viz. on selection of capable microbial strains, modeling the system in the long run and optimizing operational parameters, techno economic feasibility, etc. The present era demands to develop environmentally friendly technology that is also commercially viable. Engineered microbes must be integrated from the scientific stage to the practical and pilot stage in order to make significant advances in the use of microbes in the wastewater treatment process. Effective coordination across various disciplines and updated technologies are required to develop better environmental management in the near future.
Acknowledgments
Authors acknowledge to Department of chemical engineering IIT (BHU) Varanasi and PGDAV Delhi University for providing facilities.
Disclosure statement
No potential conflict of interest was reported by the authors.
Additional information
Funding
References
- Van Vliet MT, Flörke M, Wada Y. Quality matters for water scarcity. Nat Geosci. 2017;10(11):800–1290 802.
- Yadav D, Rangabhashiyam S, Verma P, et al. Environmental and health impacts of contaminants of emerging concerns: recent treatment challenges and approaches. Chemosphere. 2021;272:129492.
- Singh D, Goswami RK, Agrawal K, et al. Bioinspired remediation of wastewater: a contemporary approach for environmental clean up. Curr Res Green Sustain Chem. 2022;5:1–165.
- Morin-Crini N, Crini G, editors. Eau xindustriellescontaminées. France: press universitaires de Franche-Comte; 2017. p. 513.
- Dutta P, Bhakta S, Sahu E. et al. Analysis of growth and biochemical contents of microalgae grown with wastewater effluent of Emami paper mill Balasore, in: Role Microalgae Wastewater Treat. Singapore: Springer; 2019. pp. 153–168.
- Sarawaneeyaruk S, Lorliam W, Krajangsang S, et al. Enhancing plant growth under municipal wastewater irrigation by plant growth promoting rhizospheric Bacillus spp. J King Saud Univ Sci. 2019;31(3):384–389.
- Khanal SK, Giri B, Nitayavardhana S, et al. Anaerobic bioreactors/digesters: design and development. In: Venkataramana G, editor. Current developments in biotechnology and bioengineering. Elsevier; 2017. pp. 261–279.
- Qadri H, Bhat RA, Mehmood MA, et al. Fresh-water pollution dynamics and remediation. Fresh Water Pollut Dyn Rem. Sringer singapore; 2020. p. 1–13.
- Sanda BY, Ibrahim I. Causes, categories and control of water pollution. Int J Sci Eng Sci. 2020;4(9):84–90.
- Jabeen A, Huang X, Aamir M. The Challenges of Water Pollution, Threat to Public Health, Flaws of Water Laws and Policies in Pakistan. J Water Resource Prot. 2015;7(December):1516–1526.
- Singh SK. Water pollution in developing countries. Nat Con Inn Res CPMSED-2015. 2015;1:1–5.
- Javier MS, Sara MZ, Hugh T. Water pollution from agriculture: a global review ||. The Food and Agriculture Organization of the United NationsRome, 2017 and the International Water Management Institute. FAO and IWMI; 2017.
- Dwivedi AK. Researches in Water Pollution: a Review. Int Res J Nat Sci. 2017;4(1):34–42.
- Collivignarelli MC, Abbà A, Benigna I, et al. Overview of the main disinfection 890 processes for wastewater and drinking water treatment plants. Sustainability. 2018;10(1):86.
- Samer M., editor. Wastewater Treatment Engineering; 2015.
- Sikosana ML, Sikhwivhilu K, Moutloali R, Madriya DM(2019). Municipal wastewater treatment technologies:Areview. Procedia Manufacturing. 35;1018–1024.
- Chang JS, Chou C, Lin Y, et al. Kinetic characteristics of bacterial azo dye decolorization by Pseudomonas luteola. Water Res. 2001;35:2041.
- Rajesh Banu J, Kaliappan S. Treatment of tannery wastewater using hybrid upflow anaerobic sludge blanket reactor. J Environ Eng Sci. 2007;6:415–421.
- Cheah WY, Show PL, Yap YJ, et al. Enhancing microalga Chlorella sorokiniana CY-1 biomass and lipid production in palm oil mill effluent (POME) using novel-designed photobioreactor. Bioengineered. 2020;11(1):61–69.
- Pachaiappan R, Cornejo-Ponce L, Rajendran R, et al. A review on biofiltration techniques: recent advancements in the removal of volatile organic compounds and heavy metals in the treatment of polluted water. Bioengineered. 2022;13(4):8432–8477.
- Chattopadhyay I, Usman TM, Varjani S. Exploring the role of microbial biofilm for industrial effluents treatment. Bioengineered. 2022;13(3):6420–6440.
- Zhang B, Yu Q, Yan G, et al. Seasonal bacterial community succession in four typical wastewater treatment plants: correlations between core microbes and process performance. Sci Rep. 2018;8:1–11.
- Guibaud G, van Hullebusch E, Bordas F. Lead and cadmium biosorption by extracellular polymeric substances (EPS) extracted from activated sludges: pH-sorption edge tests and mathematical equilibrium modelling. Chemosphere. 2006;64(11):1955–1962.
- Zhuang WQ, Fitts JP, Ajo-Franklin CM, et al. Recovery of critical metals using biometallurgy. Curr Opin Biotechnol. 2015;33:327–335.
- Šoštarić TD, Petrović MS, Pastor FT, et al. Study of heavy metals biosorption on native and alkali-treated apricot shells and its application in wastewater treatment. J Mol Liq. 2018;259:340–349.
- Gadd GM. Biosorption: critical review of scientific rationale, environmental impor‐ tance and significance for pollution treatment. J Chem Technol Biot. 2009;84(1):13–28.
- Fomina M, Gadd GM. Biosorption: current perspectives on concept, definition and application. Biores Technol. 2014;160:3–14.
- Aktar, K., Zerin, T., & Banik, A. (2019). Biodegradation of textile dyes by bacteria isolated from textile industry effluents. Stamford Journal of Microbiology, 9(1), 5–8.
- Dixit S and Garg S(2019). Development of an efficient recombinant bacterium and its application in the degradation of environmentally hazardous azo dyes. International Journal of Science and Technology. 16; 7137–7146.
- Srinivasan, S., Sadasivam, S. K., Gunalan, S., Shanmugam, G., & Kothandan, G. (2019). Application of docking and active site analysis for enzyme linked biodegradation of textile dyes. Environmental Pollution, 248, 599–608.
- Shindhal T, Rakholiya P, Varjani S, et al. A critical review on advances in the practices and perspectives for the treatment of dye industry wastewater. Bioengineered. 2021;12(1):70–87.
- Kumar M, Borah P, Devi P. Priority and emerging pollutants in water. In: Devi P, Singh P, Kumar KK, editors. Inorganic pollutants in water. UK: Elsevier; 2020. pp. 33–49.
- Ojha N, Kara R, Abbas S, et al. Bioremediation of industrial waste water: a review. IOP Conf Ser: Earth Environ Sci. 2021;796:1.
- Azubuike CC, Chikere CB, Okpokwasili GC. Bioremediation techniques– classification based on site of application: principles, advantages, limitations, and prospects. World J Microbiol Biotechnol. 2016;32(11):180.
- Simpson DR. Biofilm processes in biologically active carbon water purification. Water Res. 2008;42:2839–2848.
- Pandey VC, Singh J, Singh D, et al. Methanotrophs: promising bacteria for environmental remediation. Int J Environ Sci Technol. 2014;11:241–250.
- Han B, Zhang S, Zhang L, et al. Characterization of microbes and denitrifiers attached to two species of floating plants in the wetlands of Lake Taihu. PLoS ONE. 2018;13(11):e0207443.
- Idi A, Nor MHM, Wahab MFA, et al. Photosynthetic bacteria: an eco-friendly and cheap tool for bioremediation. Rev Environ Sci Bio/Technol. 2015;14(2):271–285.
- Liu W-L, Guan M, Liu S-Y, et al. Fungal denitrification potential in vertical flow microcosm wetlands as impacted by depth stratification and plant species. Ecol Eng. 2015;77:163–171.
- Srivastava P, Yadav AK, Mishra BK. The effects of microbial fuel cell integration into constructed wetland on the performance of constructed wetland. Bioresour Technol. 2015;195:223–230.
- Un, U. T., & Ocal, S. E. (2015). Removal of heavy metals (Cd, Cu, Ni) by electrocoagulation. International Journal of Environmental Science and Development, 6(6), 425.
- Shahid MJ, AL-Surhanee AA, Kauadri F, et al. Role of microorganisms in the remediation of wasteland in floating treatment wetlands: a review. Sustainibility. 2020;12:5559.
- Volesky B, Holan ZR. Biosorption of heavy metals. Biotechnol Prog. 1995;11:235–250.
- Pandey AK, Sarada DV, Kumar A Microbial decolorization and degradation of reactive red 198. Proceedings of the National Academy of Sciences; Washington, DC; 2016. p. 805–815.
- Singh S, Kumar V, Upadhyay N, Singh J, Singla S, Datta S(2017). Efficient biodegradation of acephate by Pseudomonas pseudocaligenes PS-5 in the presence and absense of heavy metal ions[Cu(II) and Fe(III) and humic acid. Biotech 7(4):262.
- Elliott A, Hanby W, Malcolm B. The near infra-red absorption spectra of natural and synthetic fibres. Br J Appl Phys. 1954;5:377.
- Doble M, Kumar A. Biotreatment of industrial effluents. Resour Conserv recycl. 2007;51(2):507–508.
- Hassaan MA, El Nemr A. Advanced OxidationProcesses for textile wastewater treatment. Int J Photochem Photobiol. 2017;511:507–508.
- Thakur JK, Paul S, Dureja P, et al. Degradation of sulphonated azo dye red HE7B by Bacillus sp. and elucidation of degradative pathways. Curr Microbiol. 2014;69:183–191.
- Du L-N, Li G, Zhao Y-H, et al. Efficient metabolism of the azo dye methyl orange by Aeromonas sp. strain DH-6: characteristics and partial mechanism. Int Biodeterior Biodegrad. 2015;105:66–72.
- Xu F, Mou Z, Geng J, et al. Azo dye decolorization by a halotolerant exoelectrogenic decolorizer isolated from marine sediment. Chemosphere. 2016;158:30–36.
- Neifar M, Chouchane H, Mahjoubi M, et al. Pseudomonas extremorientalis BU118: a new salt-tolerant laccase-secreting bacterium with biotechnological potential in textile azo dye decolourization. Biotech. 2016;3(6):107.
- Srinivasan S, Shanmugam G, Surwase SV, et al. In silico analysis of bacterial systems for textile azo dye decolorization and affirmation with wetlab studies. Clean (Weinh). 2017;45(9):1600734.
- Srinivasan S, Sadasivam SK. Exploring docking and aerobic-microaerophilic biodegradation of textile azo dye by bacterial systems. J Water Process Eng. 2018;22:180–191.
- Basutkar MR, Fatima N, Chakra P, et al. Decolorisation of reactive red 120 azo dye by micrococcus sp. Int J Adv Res Biol Sci. 2019;6(5):118–125.
- Franca RDG, Vieira A, Carvalho G, et al. Oerskovia'paurometabola can efficiently decolorize azo dye acid red 14 and remove its recalcitrant metabolite. Ecotoxicol Environ Saf. 2020;191:110007.
- Masarbo RS, Karegoudar TB. Decolourisation of toxic azo dye fast red E by three bacterial strains: process optimisation and toxicity assessment. Int J Environ Anal Chem. 2020;51(2): 507–508 .
- Joshi AU, Hinsu AT, Kotadiya RJ, et al. Decolorization and biodegradation of textile di-azo dye acid blue 113 by Pseudomonas stutzeri AK6. 2020; 3 Biotech.
- Srinivasan S, Sadasivam SK. Biodegradation of textile azo dyes by textile effluent non-adapted and adapted Aeromonas hydrophila. Environ Res. 2021;194:110643.
- Chen G, An X, Li H, et al. Detoxification of azo dye direct black G by thermophilicAnoxybacillus sp. PDR2 and its application potential in bioremediation. Ecotoxicol Environ Saf. 2021;214:112084.
- Hassaan, M. A., & El Nemr, A. (2017). Advanced oxidation processes for textile wastewater treatment. International Journal of Photochemistry and Photobiology, 2(3), 85–93.
- Wuhrmann K, Mechsner K, Kappeler T. Investigations on rate determining factors in the microbial reduction of azo dyes. Eur J Appl Microbiol Biotechnol. 1980;9:325–338.
- Lin J, Zhang X, Li Z, et al. Biodegradation of reactive blue 13 in a two-stage anaerobic/aerobic fluidized beds system with a Pseudomonas sp. isolate. Bioresour Technol. 2010;101(1):34–40.
- Sarayu K, Sandhya S. Aerobic biodegradation pathway for remazol orange by Pseudomonas aeruginosa. Appl Biochem Biotechnol. 2010;160(4):1241–1253.
- Khandare RV, Govindwar SP. Microbial degradation mechanism of textile dye and its metabolic pathway for environmental safety. In: Chandra R, editor. Environmental waste management. UK: CRC Press; 2015.
- Chang, J. S., Chou, C., Lin, Y. C., Lin, P. J., Ho, J. Y., & Hu, T. L. (2001). Kinetic characteristics of bacterial azo-dye decolorization by Pseudomonas luteola. Water research, 35(12), 2841–2850.
- Nachiyar CV, Rajkumar GS. ‘Degradation of tannery and textile dye, navian fast blue S5R by Pseudomonas aerugenosa. World J Microbiol Biotechnol. 2003;19:609.
- Colwell RR, Walker JD, Cooney JJ. Ecological aspects of microbial degradation of petroleum in the marine environment. Crit Rev Microbiol. 1977;5(4):423–445.
- Cooney JJ, Silver SA, Beck EA. Factors influencing hydrocarbon degradation in three freshwater lakes. Microb Ecol. 1985;11(2):127–137.
- Perry JJ. Microbial metabolism of cyclic alkanes. In: Atlas R, editor. Petroleummicrobiology. New York, NY, USA: Macmillan; 1984. pp. 61–98.
- HJ UWR, Reed G, editors. Contaminant soil areas, different countries and contaminant monitoring of contaminants. environmental process II. Soil decontamination biotechnology. WILEY‐VCH Verlag GmbH; 2000; Vol. 11: pp. 5–42.
- Atlas R, Bragg J. Bioremediation of marine oil spills: when and when not—the Exxon Valdez experience. Microb Biotechnol. 2009;2(2):213–221.
- Throne-Holst M, Wentzel A, Ellingsen TE, et al. Identification of novel genes involved in long-chain n-alkane degradation by Acinetobacter sp. strain DSM 17874. Appl environ microbiol. 2007;73(10):3327–3332.
- Daugulis AJ, McCracken CM. Microbial degradation of high and low molecular weight polyaromatic hydrocarbons in a two-phase partitioning bioreactor by two strains of Sphingomonas sp. Biotechnol Lett. 2003;25(17):1441–1444.
- Pinholt Y, Struwe S, Kjoller A. Microbial changes during oil decomposition in soil. Holarctic Ecol. 1979;2:195–200.
- Mulkins Phillips GJ, Stewart JE. Distribution of hydrocarbon utilizing bacteria in Northwestern Atlantic waters and coastal sediments. Can J Microbiol. 1974;20(7): 955–962. PubMed] [Google Scholar.
- Ilori MO, Amobi CJ, Odocha AC. Factors affecting biosurfactant production by oil degrading Aeromonas spp. isolated from a tropical environment. Chemosphere. 2005;61(7):985–992.
- Obayori OS, Ilori MO, Adebusoye SA, et al. Degradation of hydrocarbons and biosurfactant production by Pseudomonas sp. strain LP1. World J Microbiol Biotechnol. 2009;25(9): 1615–1623. Google Scholar.
- Brusseau ML, Miller RM, Zhang Y, et al. Biosurfactant and cosolvent enhanced remediation of contaminated media. ACS Symp Ser. 1995;594:82–94. Google Scholar.
- Nikolopoulou M, Kalogerakis N. Biostimulation strategies for fresh and chronically polluted marine environments with petroleum hydrocarbons. J Chem Technol Biot. 2009;84(6):802–807.
- Sathishkumar P, Meena RAA, Palanisami T, et al. Occurrence, interactive effects and ecological risk of diclofenac in environmental compartments and biota-a review. Sci Total Environ. 2020;698:134057.
- Narayanan M, El-Sheekh M, Ma Y, et al. Current status of microbes involved in the degradation of pharmaceutical and personal care products (PPCPs) pollutants in the aquatic ecosystem. Environ Pollut. 2022;300:118922.
- Dhodapkar RS, Gandhi KN. Pharmaceuticals and personal care products in aquatic environment: chemicals of emerging concern? Pharm Pers Care Prod: Waste Manage Treat Technol. Chennai, India: Elsevier-Butterworth-Heinemann; 2019; pp. 63–85.
- Chojnacka K, Skrzypczak D, Izydorczyk G, et al. Biodegradation of pharmaceuticals in photobioreactors–a systematic literature review. Bioengineered. 2022;13(2):4537–4556.
- Amran RH, Jamal MTPugazhendi A, AL-Harbi M, Gandhourah M, Al-Otaibi A and Haque MF(2021). Biodegradation and Bioremediation of Petroleum hydrocarbons in marine Ecosystems by microorganisms. A review. Nature Environment and Pollution Technology, 21(3):1149–1157.
- Castelo-Grande T, Augusto PA, Monteiro P, et al. Remediation of soils contaminated with pesticides: a review. Int J Environ Analyt Chem. 2010;90:438–467.
- UNEP. Riding the world of POPs: a guide on the Stockholm convention on persistent organic pollutants. 2005. Available online: (accessed on 20 September 2016). http://www.pops.int/documents/guidance/beg_guide.pdf.
- UNEP. The hazardous chemicals and waste conventions; WHO: Rome, Italy. UNEPFAO Nairobi, KenyaGeneva, Switzerland. 2013. Available online accessed on 5 March 2013. http://www.pops.int/documents/background/hcwc.pdf
- Nawaz K, Hussain K, Choudary N, et al. Lashari MI. Eco-friendly role of biodegradation against agricultural pesticides hazards. Afr J Microbiol Res. 2011;5(3):177–183.
- Bilal M, Iqbal HM, Barceló D. Persistence of pesticides-based contaminants in the environment and their effective degradation using laccase-assisted biocatalytic systems. Sci Total Environ. 2019;695:133896.
- Sun Y, Kumar M, Wang L, et al. Biotechnology for soil decontamination: opportunity, challenges, and prospects for pesticide biodegradation. In:Pacheco-Torgal F, Ivanov V, Tsang DC, editors. Bio-based materials and biotechnologies for eco-efficient construction. United Kingdom: Woodhead Publishing; 2020. pp. 261–283.
- Tarla DN, Erickson LE, Hettiarachchi GM, et al. Phytoremediation and bioremediation of pesticide-contaminated soil. Appl Sci. 2020;10(4):1217.
- Mohamed AT, El Hussein AA, El Siddig MA, et al. Degradation of oxyfluorfen herbicide by soil microorganisms: biodegradation of herbicides. Biotechnol. 2011;10:274–279.
- Gadipelly C, Pérez-González A, Yadav GD, Ortiz I, Ibanez R, Rathod VK, Marathe KV (2014) Pharmaceutical industry wastewater: review of the technologies for water treatment and reuse. Ind Eng Chem Res 53(29):11571–11592. https://doi.org/10.1021/ie501210j.
- Hussaini S, Shaker M, Asef M. Isolation of bacterial for degradation of selected pesticides. Bull Environ Pharmacol Life Sci. 2013;2:50–53.
- Singh M. Green remediation. Tool for safe and sustainable environment: a review. Appl Water Sci. 2017a;7:2629–2635.
- da Silva Rodrigues, D. A., da Cunha, C. C. R. F., Freitas, M. G., de Barros, A. L. C., Neves, P. B., Pereira, A. R., … & Afonso, R. J. D. C. F. (2020). Biodegradation of sulfamethoxazole by microalgae-bacteria consortium in wastewater treatment plant effluents. Science of The Total Environment, 749, 141441.
- Alvarez LH. Influence of redox mediators and salinity level on the (bio)transformation of direct blue 71: kinetics aspects. J Environ Manag. 2016;183:84–89.
- Muthusaravanan S, Sivarajasekar N, Vivek JS, et al. Phytoremediation of heavy metals: mechanisms, methods and enhancements. Environ Chem Lett. 2018;16:1339–1359.
- Ahemad M, Malik A. Bioaccumulation of heavy metals by zinc resistant bacteria isolated from agricultural soils irrigated with wastewater. Bacteriol J. 2011;2:12–21.
- Gaur VK, Sharma P, Gaur P, et al. Sustainable mitigation of heavy metals from effluents: toxicity and fate with recent technological advancements. Bioengineered. 2021;12(1):7297–7313.
- Guo W, Ngo H-H, Dharmawan F, et al. Roles of polyurethane foam in aerobic moving and fixed bed bioreactors. Bioresour Technol. 2010;101:1435–1439.
- Shin CJ, Nam JM, Kim JG. Floating mat as a habitat of Cicuta virosa, a vulnerable hydrophyte. Landsc Ecol Eng. 2015;11:111–117.
- Rajkumar M, Ae N, Freitas H. Endophytic bacteria and their potential to enhance heavy metal phytoextraction. Chemosphere. 2009;77:153–160.
- Zhao F, Yang W, Zeng Z, et al. Nutrient removal efficiency and biomass production of different bioenergy plants in hypereutrophic water. Biomass Bioenergy. 2012;42:212–218.
- Wu H, Zhang J, Li P, et al. Nutrient removal in constructed microcosm wetlands for treating polluted river water in northern China. Ecol Eng. 2011;37:560–568.
- Wang C-Y, Sample DJ. Assessment of the nutrient removal effectiveness of floating treatment wetlands applied to urban retention ponds. J Environ Manag. 2014;137:23–35.
- Mónica, P., Darwin, R. O., Manjunatha, B., Zúñiga, J. J., Diego, R., Bryan, R. B., … & Maddela, N. R. (2016). Evaluation of various pesticides-degrading pure bacterial cultures isolated from pesticide-contaminated soils in Ecuador. African Journal of Biotechnology, 15(40), 2224–2233.
- Igiri BE, Okuduwa SIR, Idoko GO, et al. Toxicity and bioremediation of heavy metals contaminated ecosystems from tannery wastewater: a review. Hindawi J Toxicol. 2018;2018:2568038.
- Rajkumar M, Ae N, Prasad MNV, et al. Potential of siderophore-producing bacteria for improving heavy metal phytoextraction. Trends Biotechnol. 2010;28:142–149.
- Kumar A, Bisht BS, Joshi VD, et al. Review on bioremediation of polluted environment: a management tool. International Journal of Environmental Sciences. 2011;1:1079–1093.
- Muneer B, Iqbal MJ, Shakoori FR, et al. Tolerance and biosorption of mercury by microbial consortia: potential use in bioremediation of wastewater. Pak J Zool. 2013;45(1):247–254.
- Oshima T, Kondo K, Ohto K, et al. Preparation of phosphorylated bacterial cellulose as an adsorbent for metal ions. React Funct Polym. 2008;68:376–383.
- Marchal M, Briandet R, Koechler S, et al. Effect of arsenite on swimming motility delays surface colonization in Herminiimonasarsenicoxydans. Microbiology. 2010;156:2336–2342.
- Sinha SN, Biswas M, Paul D, et al. Biodegradation potential of bacterial isolates from tannery effluent with special reference to hexavalent chromium. Biotechnol bioinforma bioeng. 2011;1:381–386.
- Priyalaxmi R, Murugan A, Raja P, et al. Bioremediation of cadmium by Bacillus safensis (JX126862), a marine bacterium isolated from mangrove sediments. Int J Curr Microbiol App Sci. 2014;3:326–335.
- Sinha SN, Biswas K. Bioremediation of lead from river water through lead-resistant purple-nonsulfur bacteria. Global J Microbiol Biotechnol. 2014;2:11–18.
- Boonsong K and Chansiri M(2008). Domestic wastewater treatment using vetiver grass cultivated with floating platform technique. AU J. Technology;12:73–80.
- Wu YH, Zhou P, Cheng H, et al. Draft genome sequence of Microbacteriumprofundi Shh49T, an Actinobacterium isolated from deep-sea sediment of a polymetallic nodule environment. Genome Announce. 2015;3:1–2.
- Sofu A, Sayilgan E, Guney G. Experimental design for removal of Fe (II) and Zn (II) ions by different lactic acid bacteria biomasses. Int J Environ Res. 2015;9:93–100. 201.
- Guo Y, Huang T, Wen G, et al. The simultaneous removal of ammonium and manganese from groundwater by iron-manganese co-oxide filter film: the role of chemical catalytic oxidation for ammonium removal. Chem Eng J. 2017;308:322–329.
- Fauziah SH. Assessing the bioaugmentation potentials of individual isolates from landfill on metal-polluted soil. Environ Earth Sci. 2017;76:401.
- Pugazhendhi A, Boovaragamoorthy GM, Ranganathan K, et al. New insight into effective biosorption of lead from aqueous solution using Ralstonia solanacearum: characterization and mechanism studies. J Clean Prod. 2018;174:1234–1239.
- Pramanik K, Mitra S, Sarkar A, et al. Alleviation of phytotoxic effects of cadmium on rice seedlings by cadmium resistant PGPR strain Enterobacter aerogenes MCC 3092. J Hazard Mater. 2018;351:317–329.
- Lakshmi S, Suvedha K, Sruthi R, et al. Hexavalent chromium sequestration from electronic waste by biomass of Aspergillus carbonarius. Bioengineered. 2020;11(1):708–717.
- Agrawal K, Verma P. Myco-valorization approach using entrapped Myrothecium verrucaria ITCC-8447 on synthetic and natural support via column bioreactor for the detoxification and degradation of anthraquinone dyes. Int Biodeterior Biodegrad. 2020;153:105052.
- Espinosa EJ, Rene ER, Pakshirajan K, et al. Fungal pelleted reactors in wastewater treatment: applications and perspectives. Chem Eng J. 2016;283:553–571.
- Gayathiri E, Prakash P, Selvam K, et al. Plant microbe-based remediation approaches in dye removal: a review. Bioengineered. 2022;13(3):7798–7828.
- Singh AK, Bilal M, Iqbal HMN, et al. Bioremediation of lignin derivatives and phenolics in wastewater with lignin modifying enzymes: status, opportunities and challenges. Sci Total Environ. 2021;777:145988.
- Assress HA, Selvarajan R, Nyoni H, et al. Diversity, co-occurrence and implications of fungal communities in wastewater treatment plants. Sci Rep. 2019;9:1–15.
- Borne KE, Fassman-Beck EA, Tanner CC. Floating treatment wetland influences on the fate of metals in road runoff retention ponds. Water Res. 2014;48:430–442.
- Park D, Yun Y-S, Jo JH, et al. Mechanism of hexavalent chromium removal by dead fungal biomass of Aspergillusniger. Water Res. 2005;39(4):533–540.
- Luna JM, Rufno RD, Sarubbo LA. Biosurfactant from Candida sphaerica UCP0995 exhibiting heavy metal remediation properties. Process SafEnviron Prot. 2016;102:558–566.
- Donmez G, Aksu Z. Bioaccumulation of copper(ii) and nickel(ii) by the non-adapted and adapted growing Candida SP. Water Res. 2001;35(6):1425–1434.
- Ashokkumar P, Loashini VM, Bhavya V. Effect of pH, Temperature and biomass on biosorption of heavy metals by Sphaerotilusnatans. Int J Microbiol Mycol. 2017;6(1):32–38.
- Achal V, Kumari D, Pan X. Bioremediation of chromium contaminated soil by a brown-rot fungus, Gloeophyllumsepiarium. Res J Microbiol. 2011;6(2):166–171.
- Bhattacharya A, Gupta A, Kaur A, Malik D (2014) Efficacy of Acinetobacter sp. B9 for simultaneous removal of phenol and hexavalent chromium from co-contaminated system. Appl Microbiol Biotechnol 98:9829–9841.
- Magyarosy A, Laidlaw R, Kilaas R, et al. Nickel accumulation and nickel oxalate precipitation by Aspergillusniger. Appl Microbiol Biotechnol. 2002;59(2–3):382–388.
- Benazir, J. F., Suganthi, R., Rajvel, D., Pooja, M. P., & Mathithumilan, B. (2010). Bioremediation of chromium in tannery effluent by microbial consortia. African journal of biotechnology, 9(21), 3140–3143.
- Bhargava RN and Mishra S(2018). Hexavalent Chromium reduction potential of Cellulosimicrobium sp. isolated from common effluent treatment plant of tannery industries. Ecotox. Environ Saf. 147:102–109.
- Salehizadeh H, Shojaosadati SA. Removal of metal ions from aqueous solution by polysaccharide produced from Bacillus firmus. Water Res. 2003 Oct;37(17):4231–5. doi: 10.1016/S0043-1354(03)00418-4. PMID: 12946905.
- Gros M, Cruz-Morato C, Marco-Urrea E, et al. Biodegradation of the X-ray contrast agent iopromide and the fluoroquinolone antibiotic ofloxacin by the white rot fungus Trametes versicolor in hospital wastewaters and identification of degradation products. Water Res. 2014;60:228–241.
- Congeevaram, S., Dhanarani, S., Park, J., Dexilin, M., & Thamaraiselvi, K. (2007). Biosorption of chromium and nickel by heavy metal resistant fungal and bacterial isolates. Journal of Hazardous Materials, 146(1–2), 270–277. https://doi.org/10.1016/j.jhazmat.2006.12.017.
- Congeevaram, S., Dhanarani, S., Park, J., Dexilin, M., & Thamaraiselvi, K. (2007). Biosorption of chromium and nickel by heavy metal resistant fungal and bacterial isolates. Journal of Hazardous Materials, 146(1–2), 270–277. https://doi.org/10.1016/j.jhazmat.2006.12.017.
- Jafari, S. A., Cheraghi, S., Mirbakhsh, M., Mirza, R., & Maryamabadi, A. (2015). Employing response surface methodology for optimization of mercury bioremediation by Vibrio parahaemolyticus PG02 in coastal sediments of Bushehr, Iran. CLEAN–Soil, Air, Water, 43(1), 118–126. https://doi.org/10.1002/clen.201300616.
- Al-Garni, S. M., Ghanem, K. M., & Ibrahim, A. S. (2010). Biosorption of mercury by capsulated and slime layerforming Gram-ve bacilli from an aqueous solution. African Journal of Biotechnology, 9(38), 6413–6421.
- Jafari, S. A., Cheraghi, S., Mirbakhsh, M., Mirza, R., & Maryamabadi, A. (2015). Employing response surface methodology for optimization of mercury bioremediation by Vibrio parahaemolyticus PG02 in coastal sediments of Bushehr, Iran. CLEAN–Soil, Air, Water, 43(1), 118–126. https://doi.org/10.1002/clen.201300616.
- Salehizadeh H, Shojaosadati SA. Removal of metal ions from aqueous solution by polysaccharide produced from Bacillus firmus. Water Res. 2003 Oct;37(17):4231–5. doi: 10.1016/S0043-1354(03)00418-4. PMID: 12946905.
- Badia-Fabregat M, Lucas D, Pereira MA, et al. Continuous fungal treatment of non-sterile veterinary hospital effluent: pharmaceuticals removal and microbial community assessment. Appl Microbiol Biotechnol. 2016;100:2401–2415.
- Cruz-Morató C, Ferrando-Climent L, Rodriguez-Mozaz S, et al. Degradation of pharmaceuticals in non-sterile urban wastewater by Trametes versicolor in a fluidized bed bioreactor. Water Res. 2013;47:5200–5210.
- Å S, Hedeland M, Arvidsson T, et al. Identification of leachables from Trametes versicolor in biodegradation experiments. Trends Green Chem. 2018;4:1–4.
- Palli L, Castellet-Rovira F, Péerez-Trujillo M, et al. Preliminary evaluation of pleurotus ostreatus for the removal of selected pharmaceuticals from hospital wastewater. Biotechnol Prog. 2017;33:1529–1537.
- Molla AH, Fakhru’l-Razi A, Hanafi MM, et al. Potential nonphytopathogenic filamentous fungi for bioconversion of domestic wastewater sludge. J Environ Sci Heal Part A. 2002;37(8):1495–1507.
- Kang Y, Xu X, Pan H, et al. Decolorization of Mordant yellow 1 using Aspergillus sp. TS-A CGMMC 12964 by Biosorption and Biodegradation. 2018;9(1):222–232.
- Andleeb S, Atiq N, Robson GD, et al. An investigation of anthraquinone dye biodegradation by immobilized Aspergillus flavus in fluidized bed bioreactor. Environ Sci Pollut Res. 2012;19:1728–1737.
- Ibrahim NN, Talib SA, Ismail HN, et al. Decolorization of reactive red-120 by using macrofungus and microfungus Research article special issue. J Fundam Appl Sci. 2018;9(6S):954–964.
- Khan R, Fulekar MH. Mineralization of a sulfonated textile dye reactive red 31 from simulated wastewater using pellets of Aspergillusbombycis. Bioresour Bioprocess. 2017;4:1–11.
- Asses N, Ayed L, Hkiri N, et al. Congo red DecolorizationandDetoxification by Aspergillus niger: removal mechanisms and dye degradation pathway Nedra asses. BioMed Res Int. 2018. DOI:10.1155/2018/3049686
- Dayi B, Onac C, Kaya A, et al. New type biomembrane: transport and biodegradation of reactive textile dye. J Phys Chem Lett. 2020;5(7):9813–9819.
- Sheam MM, Biswas SK, Ahmed K, et al. Mycoremediation of reactive red H37B dye by Aspergillussalinarus isolated from textile effluents. Curr res Microbial Sci. 2021;2:100056.
- Gao T, Qin D, Zuo S, et al. Decolorisation and detoxification of triphenylmethane dyes by isolated endophytic fungus, Bjerkandertaadusta SWUSI4 under non nutritive conditions. Bioresources Bioprocess. 2020;7(53). DOI:10.1186/s40643-020-00340-8
- Machado MD, Soares EV, Soares HM. Removal of heavy metals using a brewer’s yeast strain of Saccharomyces cerevisiae: chemical speciation as a tool in the prediction and improving of treatment efficiency of real electroplating effluents. Journal of Haz‐ Ardous Materials. 2010;180(1–3):347–353.
- Namara CJ, Anastasiou CC, O’flaherty V, et al. Bioremediation of olive mill wastewater. Int Biodeterior Biodegrad. 2008;61(2):127–134.
- Bhakta JN, Munekage Y, Ohnishi K, et al. Isolation and charac‐ terization of cadmium and arsenic-absorbing bacteria for bioremediation. Water Air Soil Pollut. 2014;225 2150–2159. DOI:10.1007/s11270-014-2151-2
- Goswami RK, Agrawal K, Verma P. Microalgae-based biofuel-integrated biorefinery approach as sustainable feedstock for resolving energy crisis. In: Srivastava V, editor. Bioenergy research: commercial opportunities and challenges, Clean Energy Production Technologies. Singapore: Springer; 2021. pp. 267–293.
- Salama ES, Kurade MB, Abou-Shanab RAI, et al. Recent progress in microalgal biomass production coupled with wastewater treatment for biofuel generation, Renew. Sustain Energy Rev. 2017;79:1189–1211.
- Darda S, Papalas T, Zabaniotou A. Biofuels journey in Europe: currently the way to low carbon economy sustainability is still a challenge. J Clean Prod. 2019;208:575–588.
- Ajayan KV, Selvaraju M, Thirugnanamoorthy K. Growth and heavy metals accumulation potential of microalgae grown in sewage wastewater and petrochemical effluents. Pakistan J Biol Sci. 2011;14:805.
- Manzoor M, Ma R, Shakir HA, et al. Microalgal-bacterial consortium: a cost-effective approach of wastewater treatment in Pakistan, Punjab Univ. J Zool. 2016;31:307–320.
- Mehariya S, Goswami RK, Verma P, et al. Integrated approach for wastewater treatment and biofuel production in microalgae biorefineries. Energies. 2021;14:101747.
- Goher ME, El-Monem AMA, Abdel-Satar AM, et al. Biosorption of some toxic metals from aqueous solution using nonliving algal cells of Chlorella vulgaris. J Elem. 2016;21(3):703–714.
- Khan MJ, Rai A, Ahirwar A, et al. Diatom microalgae as smart nanocontainers for biosensing wastewater pollutants: recent trends and innovations. Bioengineered. 2021;12(2):9531–9549.
- Shi J, Podola B, Melkonian M. Removal of nitrogen and phosphorus from wastewater using microalgae immobilized on twin layers: an experimental study. J Appl Phyco. 2007;19:417–423.
- De Godos I, Muñoz R, Guieysse B. Tetracycline removal during wastewater treatment in high-rate algal ponds. J Hazard Mater. 2012;229–230:446–449.
- Maes HM, Maletz SX, Ratte HT, et al. Uptake, elimination, and biotransformation of 17α-ethinylestradiol by the freshwater alga Desmodesmussubspicatus. Environ Sci Technol. 2014;48:12354–12361.
- Zhou GJ, Ying GG, Liu S, et al. Simultaneous removal of inorganic and organic compounds in wastewater by freshwater green microalgae. Environ Sci. 2014;16:2018–2027.
- Hom-Diaz A, Llorca M, Rodríguez-Mozaz S, et al. Microalgae cultivation on wastewater digestate: β-estradiol and 17α-ethynylestradiol degradation and transformation products identification. J Environ Manag. 2015;155:106–113.
- Salgueiro JL, Pérez L, Maceiras R, et al. Bioremediation of wastewater using chlorella vulgaris microalgae: phosphorus and organic matter. Int J Environment Res. 2016;10:465–470.
- Fazal T, Mushtaq A, Rehman F, et al. Xu J. Bioremediation of textile wastewater and successive biodiesel production using microalgae. Renew Sust Energ Rev. 2018;82:3107–3126.
- Touliabah HE-S, El-Sheekh MM, Ismail MM, et al. A review of microalgae- and cyanobacteria-based biodegradation of organic pollutants. Molecules. 2022;27:1141.
- Das N, Chandran P. Microbial degradation of petroleum hydrocarbon contaminants: an overview. Biotechnol Res Int. 2011;2011:1–13.
- Varjani S, Joshi R, Srivastava VK, et al. Treatment of wastewater from petroleum industry: current practices and perspectives. Environ Sci Pollut Res. 2020;80:1–9.
- Das A, Mishra S. Removal of textile dye reactive green-19 using bacterial consortium: process optimization using response surface methodology and kinetics study. J Environ Chem Eng. 2017;5(1):612–627.
- Asira E. Factors that determine bioremediation of organic compounds in the soil. Acad J Interdiscip Stud. 2013;2:125–128.
- Lund P, Tramonti A, De Biase D. Coping with low pH: molecular strategies in neutralophilic bacteria. FEMS Microbiol Rev. 2014;38:1091–1125.
- Singh D, Goswami RK, Agrawal K, et al. BIoinspired remediation of wastewater: A contemporary approach for environmental cleanup. Current Research in Green and Sustainable Chemistry. 2022;5:100261.
- Aragaw TA. Functions of various bacteria for specific pollutant degradation and their application in waste water treatment: a review. Int J Environ Sci Technol. 2020. DOI:10.1007/s13762-020-03022-2
- Couto N, Fritt-Rasmussen J, Jensen PE, et al. Suitability of oil bioremediation in an Artic soil using surplus heating from an incineration facility. Environ Sci Pollut Res. 2014;21:6221–6227.
- Abatenah E, Gizaw B, Tsegaye Z, et al. The role of microorganisms in Bioremediation- a review. J Environ Biol. 2017;2(1):038–046.
- Varjani S, Rakholiya P, Yong H, et al. Microbial degradation of dyes: an overview. Bioresour Technol. 2020;314:123728.
- Pacwa-Płociniczak M, Płociniczak T, Iwan J, et al. Isolation of hydrocarbon-degrading and biosurfactant-producing bacteria and assessment their plant growth-promoting traits. J Environ Manag. 2016;168:175–184.
- Nzila A. Biodegradation of high-molecular-weight polycyclic aromatic hydrocarbons under anaerobic conditions: overview of studies, proposed pathways and future perspectives. Environ Pollut. 2018;239:788–802.
- Ibrahim, N. N., Talib, S. A., Ismail, H. N., & Tay, C. C. (2017). Decolorization of reactive red-120 by using macrofungus and microfungus. Journal of Fundamental and Applied Sciences, 9(6S), 954–964.
- Castillo-Carvajal LC, Sanz-Martín JL, Barragán-Huerta BE. Biodegradation of organic pollutants in saline wastewater by halophilic microorganisms: a review. Environ Sci Pollut Res. 2014;21:9578–9588.
- Basutkar MR, Shivannavar CT. Decolorization study of reactive red-11 by using dye degrading bacterial strain Lysinibacillusboronitolerans CMGS-2. Int J Curr Microbiol App Sci. 2019;8(6):1135–1143.
- Madhavi GN, Mohini DD. Review paper on – Parameters affecting bioremediation. Int J Life Sci Pharma Res. 2012;2:77–80.
- Varjani SJ. Microbial degradation of petroleum hydrocarbons. Bioresour Technol. 2017;223:277–286.
- Oruganti RK, Katam K, Show PL, et al. A comprehensive review on the use of algal-bacterial systems for wastewater treatment with emphasis on nutrient and micropollutant removal. Bioengineered. 2022;13(4):10412–10453.
- Syed T, Batool U, Aslam M, et al. Bioremediation and decontamination potential of flagellate Poteriospumellasp, Ann. Finance. 2019;23:142–153.
- Mamta BS, Rana MS, Raychaudhari S, et al. Algae and bacteria driven technologies for pharmaceutical remediation in wastewater. Removal of toxic pollutants through microbiological and tertiary treatment. Amsterdam, Netherlands: Elsevier; 2020.
- Qi F, Jia Y, Mu R, et al. Convergent community structure of algal bacterial consortia and its effect on advanced wastewater treatment and biomass production. Sci Rep. 2021;11:21118.
- Su Y, Mennerich A, Urban B. Synergistic cooperation between wastewater-born algae and activated sludge for wastewater treatment: influence of algae and sludge inoculation ratios. Bioresour Technol. 2012;105:67–73.
- Du H. Water depletion of climax forests over humid karst terrain: patterns, controlling factors and implications. Agric Water Manag. 2021; DOI:10.1016/j.agwat.2020.106541.
- Fontalvo NPM, Gamero WBM, ARdila HAM, et al. Removal of Nitrogeneous compounds from municipal waste water using a bacterial consortium: an opportunity for more sustainable water treatments. Water Air Soil Pollut. 2022;233:339.
- Maqbool M, Bhatti HN, Sadaf S, Zahid M and Shahid M(2019). A robust approach towards green synthesis of Polyaniline -Scenedesmus biocomposite for waste water treatment applications. Material Research Express. 6(5).
- Satiro J, Cunha A, Gomes AP, et al. Optimization of microalgae- bacteria consortium in the treatment of paper pulp waste water. Appl Sci. 2022;12:5799.
- Rehman K, Imran A, Amin I, et al. Enhancement of oil field-produced wastewater remediation by bacterially-augmented floating treatment wetlands. Chemosphere. 2018;217:576–583.
- Tara N, Arslan M, Hussain Z, et al. On-site performance of floating treatment wetland macrocosms augmented with dye-degrading bacteria for the remediation of textile industry wastewater. J Clean Prod. 2019;217:541–548.
- Varjani, S., Joshi, R., Srivastava, V. K., Ngo, H. H., & Guo, W. (2020). Treatment of wastewater from petroleum industry: current practices and perspectives. Environmental Science and Pollution Research, 27, 27172–27180.
- Varjani, S., & Upasani, V. N. (2019). Influence of abiotic factors, natural attenuation, bioaugmentation and nutrient supplementation on bioremediation of petroleum crude contaminated agricultural soil. Journal of environmental management, 245, 358–366.
- Leong WH, AzellaZaine SN, Ho YC, et al. Impact of various microalgal bacterial populations on municipal wastewater bioremediation and its energy feasibility for lipid-based biofuel production. J Environ Manag. 2019;249:109384.
- Freitag DG. The use of effective microorganisms (EM) in organic waste management. 2000. DOI:10.1097/00007691-200002000-00006
- Monica S, Karthik L, Mythill S, et al. Formulation of effective microbial consortia and; its application for sewage treatment. Microb Biochem Technol. 2011;3(3):51–55.
- Solé A, Matamoros V. Removal of endocrine disrupting compounds from wastewater by microalgae co-immobilized in alginate beads. Chemosphere. 2016;164:516–523.
- Vítˇezová M, Kohoutová A, Vítˇez T, et al. Methanogenic microorganisms in industrial wastewater anaerobic treatment. Processes. 2020;8:1546.
- Vassalle L, García-Galán MJ, Aquino SF, et al. Can high-rate algal ponds be used as post-treatment of UASB reactors to remove micropollutants? Chemosphere. 2020;248:125969.
- Xu Z, Wu Y, Jiang Y, et al. Arbuscular mycorrhizal fungi in two vertical-flow wetlands constructed for heavy metal-contaminated wastewater bioremediation. Environ. Sci. Pollut. Res. 2018;25:12830–12840.
- Martínez-Gallardo MR, Lopez MJ, Jurado MM, et al. Bioremediation of olive mill wastewater sediments in evaporation ponds through in situ composting assisted by bioaugmentation. Sci Total Environ. 2019;703:135537.
- Shrivastava JN, Verma S, Kumar V. Bioremediation of Yamuna water by mono and dual bacterial isolates. Indian J Sci Res Technol. 2013;1(1):56–60.
- Gaikwad GL, Wate SR, Ramteke DS, et al. Development of microbial consortia for the effective treatment of complex wastewater. J Bioremed Biodegrad. 2014;5(4):1.
- Biswas T, Banerjee S, Saha A, et al. Bacterial consortium based petrochemical waste water treatment: from strain isolation to industrial effluent treatment. Environ Adv. 2022;7. DOI:10.1016/j.envadv.2021.100132
- Jelic A, Cruz-Morato C, Marco-Urrea E, et al. Degradation of carbamazepine by Trametes versicolor in an air pulsed fluidized bed bioreactor and identification of intermediates. Water Res. 2012;46:955–964.
- Rodarte-Moralez AI, Feijoo G, Moreira MT, et al. Degradation of selected pharmaceutical and personal care products (PPCPs) by white-rot fungi. World J Microbial Biotechnol. 2011;27:1839–1846.
- Bodin H, Daneshvar A, Gros M, et al. Effects of biopellets composed of microalgae and fungi on pharmaceuticals present at environmentally relevant levels in water. Ecol Eng J Ecotechnol. 2016;91:169–172.
- Singh et al., 2016 Singh S, Lo S, Srivastava VC and Hiwarkar AD(2016). Comparative study of electrochemical oxidation for dye degradation : Parametric optimization and mechanism identification. Journal of Environmental Chemical Engineering . 4(3).
- Meckenstock, R. U., Boll, M., Mouttaki, H., Koelschbach, J. S., Tarouco, P. C., Weyrauch, P., … & Himmelberg, A. M. (2016). Anaerobic degradation of benzene and polycyclic aromatic hydrocarbons. Microbial Physiology, 26(1–3), 92–118.
- Xiong JQ, Kurade MB, Abou-Shanab RAI, et al. Biodegradation of carbamazepine using freshwater microalgae Chlamydomonas mexicana and Scenedesmus obliquus and the determination of its metabolic fate. Bioresour Technol. 2016;205:183–190.
- Singh M, Pant G, Hossain K and Bhatia A(2017). Green remediation:Tool for safe and sustainable environment:a review. Applied Water Sciences; 7(6):2629–2635.
- Eriksen NT. The technology of microalgal culturing. Biotechnol Lett. 2008;30:1525–1536.
- Shi W, Wang L, Rousseau DPL, et al. Removal of Estrone, 17α-Ethinylestradiol, and 17βEstradiol in algae and duckweed-based wastewater treatment systems. Environ Sci Pollut Res. 2010;17:824–833.
- Dzomba P, Kugara J, Mukunyaidze VV, et al. Biodegradation of tetracycline antibacterial using green algal species collected from municipal and hospital effluents. Der Chem Sin. 2015;6:27–33.
- Kumar M, León V, De SistoMaterano A, et al. Biosurfactant production and hydrocarbon-degradation by halotolerant and thermotolerant Pseudomonas sp. World J Microbiol Biotechnol. 2008;24(7):1047–1057.
- Mahmound A, Aziza Y, Abdeltif A, et al. Biosurfactant production by Bacillus strain injected in the petroleum reservoirs. J Ind Microbiol Biotechnol. 2008;35:1303–1306.
- Muthusamy K, Gopalakrishnan S, Ravi TK, et al. Biosurfactants: properties, commercial production and application. Curr Sci. 2008;94(6):736–747.
- Tabatabaee A, Assadi MM, Noohi AA, et al. Isolation of biosurfactant producing bacteria from oil reservoirs. Iran J Environ Health Sci Eng. 2005;2(1):6–12.
- Youssef N, Simpson DR, Duncan KE, et al. In situ biosurfactant production by Bacillus strains injected into a limestone petroleum reservoir. Appl environ microbiol. 2007;73(4):1239–1247.
- Daverey A, Pakshirajan K. Production of sophorolipids by the yeast Candida bombicola using simple and low-cost fermentative media. Food Res Int. 2009;42(4):499–504.
- Banerjee S, Tiwade PB, Sambhav K, et al. Effect of alginate concentration in wastewater nutrient removal using alginate-immobilized microalgae beads: uptake kinetics and adsorption studies. Biochem Eng J. 2019;149:107241.
- Eichlerová I, Homolka L, Benada O, et al. Decolorization of orange G and remazol brilliant blue R by the white rot fungus Dichomitussqualens: toxicological evaluation and morphological study. Chemosphere. 2007;69(5):795–802. DOI:10.1016/j.chemosphere.2007.04.083
- Goswami RK, Agrawal K, Mehariya S, et al. Microalgae-based biorefinery for utilization of carbon dioxide for production of valuable bioproducts, in: chemo-biological systems for CO2 utilization. Boca Raton: CRC Press; 2020. pp. 203–228.
- Hosseinzadeh A, Baziar M, Alidadi H, et al. Application 971 of artificial neural network and multiple linear regression in modeling nutrient recovery in vermicompost 972 under different conditions. Biores Technol. 2020;303:122926.
- Ji J, Kakade A, Yu Z, et al. Anaerobic membrane bioreactors for treatment of 991 emerging contaminants: a review. J Environ Manage. 2020;270:110913.
- Kadri T, Rouissi T, Kaur BS, et al. Biodegradation of polycyclic aromatic hydrocarbons (PAHs) by fungal enzymes: a review. J Environ Sci. 2017;51:52–74.
- Kang Y, Xu X, Pan H, et al. Decolorization of mordant yellow 1 using Aspergillus sp. TS-A CGMCC 12964 by biosorption and biodegradation. Bioengineered. 2017;9(1):222–232.
- Kujan P, Prell A, Safár H, et al. Use of the industrial yeast Candida utilis for cadmium sorption. Folia Microbiol (Praha). 2006;51(4):257–260.
- Lee Li C, Wang S, Du X, et al. Immobilization of iron-and manganese-oxidizing bacteria with a biofilm-forming bacterium for the effective removal of iron and manganese from groundwater. Bioresour Technol. 2016;220:76–84.
- Li M, Wu Y-J, Yu Z-L, et al. Nitrogen removal from eutrophic water by floating-bed-grown water spinach (Ipomoea aquaticaForsk.) with ion implantation. Water Res. 2007;41:3152–3158.
- Lim SL, Chu WL, andPhang SM. Use of Chlorella vulgaris for bioremediation of textile wastewater. Biores Technol. 2010;101(19):7314–7322.
- Liu J, Chen F. Biology and industrial applications of chlorella: advances and prospects, microalgae biotechnol. In: Posten C, Chen SF, editors. Microalgae biotechnology. advances in biochemical engineering/biotechnology. Cham: Springer; 2014. pp. 1–35.
- Malik ZA, Ahmed S. Degradation of petroleum hydrocarbons by oil field isolated bacterial consortium. Afr J Biotechnol. 2012;11:650–658.
- Meckenstock RU. Anaerobic degradation of benzene and polycyclic aromatic hydrocarbons. J Mol Microbiol Biotechnol. 2016;26:92–118.
- Mónica P, Darwin RO, Manjunatha B, et al. Maddela. evaluation of various pesticides-degrading pure bacterial cultures isolated from pesticide-contaminated soils in ecuador. Afr J Biotechnol. 2016;15:2224–2233.
- Newman LA, Reynolds CM. Phytodegradation of organic compounds. Curr Opin Biotechnol. 2004;15(3):225–230.
- Poo KM, Son E-B, Chang J-S, et al. Biochars derived from wasted marine macro-algae (Saccharina japonica and Sargassum fusiforme) and their potential for heavy metal removal in aqueous solution. J Environ Manag. 2018;206:364–372.
- Qiu S, Yu Z, Hu Y, et al. An evolved native microalgal consortium-snow system for the bioremediation of biogas and centrate wastewater: start-up, optimization and stabilization. Water Res. 2021;196:117038.
- Kumar RK, Agrawal K, Mehariya S, et al. Current perspective on wastewater treatment using photobioreactor for Tetraselmissp.: an emerging and foreseeable sustainable approach. Environ Sci Pollut Res. 2021;67:1–33.
- Rathoure AK, Dhatwalia VK. Toxicity and waste management using bioremediation. Harshey, PA: IGI Global; 2016. p. 421.
- Yao S, Lyu S, An Y, et al. Microalgae–bacteria symbiosis in microalgal growth and biofuel production: a review. J Appl Microbiol. 2019;126:359–368.
- Vassalle, L., García-Galán, M. J., Aquino, S. F., Afonso, R. J. D. C. F., Ferrer, I., Passos, F., & Mota, C. R. (2020). Can high rate algal ponds be used as post-treatment of UASB reactors to remove micropollutants?. Chemosphere, 248, 125969.
- Zhao X, Chen Z, Wang X, et al. Ppcps removal by aerobic granular sludge membrane bioreactor, Appl. Microbiol Biotechnol. 2014;98:9843–9848.
- Boduroglu G, Kılıç NK, Onmez GD. Bioremoval of ¨ reactive blue 220 by gonium sp. biomass. Environ Technol. 2014;35(19):2410–2415.
- Gupta VK, Bhushan R, Nayak A, et al. Biosorption and reuse potential of a blue green alga for the removal of hazardous reactive dyes from aqueous solutions. Bioremediat J. 2014;18(3):179–191.
- Santos GC, Corso CR. Comparative analysis of azo dye biodegradation by Aspergillus oryzae and Phanerochaetechrysosporium. Water Air Soil Pollut. 2014;225:2026.
- Adnan LA, MohdYusof AR, Hadibarata T, et al. Biodegradation of bis-azo dye reactive black 5 by white-rot fungus Trametes gibbosa sp. WRF 3 and its metabolite characterization. Water Air Soil Pollut. 2014;225:2119.
- Hamadi AA, G¨uven URAZ, Katircioglu H, et al. Adsorption of azo dyes from textile wastewater by Spirulina Platensis. Eurasian J Environ Res. 2017;1(1):19–27.
- Marzbali MH, Mir AA, Pazoki M, et al. Removal of direct yellow 12 from aqueous solution by adsorption onto spirulina algae as a high-efficiency adsorbent. J Environ Chem Eng. 2017;5(2):1946–1956.
- Sheekh MM, Abou-El-Souod GW, AsragHa E. Biodegradation of some dyes by green algae Chlorella vulgaris and the cyanobacterium Aphanocapsaelachista (2018). Egypt J Bot. 2018;58(3):311–320.
- Hussein MH, Abou El-Wafa GS, Shaaban-Dessuki SA, et al. Bioremediation of methyl orange onto Nostoccarneum biomass by adsorption, kinetics and isotherm studies. Global Adv Res J Microbiol. 2018;7(1):6–22.
- Naji NS, Salman JM. Effect of temperature variation on the efficacy of Chlorella vulgaris in decolorization of Congo red from aqueous solutions. Biochem Cell Arch. 2019;19(2):4169–4174.
- Lebron YAR, Moreira VR, Santos LVS. Studies on dye biosorption enhancement by chemically modified journal of chemistry 13 Fucus vesiculosus, Spirulina maxima and Chlorella pyrenoidosa algae. J Clean Prod. 2019;240:118197.
- Tan L, Shao Y, Mu G, et al. Enhanced azo dye biodegradation performance and halotolerance of Candida tropicalis SYF-1 by static magnetic field (SMF). BioresourTechnol. 2020;295:122283.
- Das, C., Ramaiah, N., Pereira, E. & Naseera, K. Efficient bioremediation of tannery wastewater by monostrains and consortium of marine Chlorella sp. and Phormidium sp. Int. J. Phytoremediation 20, 284–292 (2018).