ABSTRACT
The uterus undergoes significant modifications throughout pregnancy to support embryo development and fetal growth. However, conditions like fibroids, adenomyosis, cysts, and C-section scarring can cause myometrial damage. The importance of the uterus and the challenges associated with myometrial damage, and the need for alternative approaches are discussed in this review. The review also explores the recent studies in tissue engineering, which involve principles of combining cells, scaffolds, and signaling molecules to create functional uterine tissues. It focuses on two key approaches in uterine tissue engineering: scaffold technique using decellularized, natural, and synthetic polymer and 3D bioprinting. These techniques create supportive structures for cell growth and tissue formation. Current treatment options for myometrial damage have limitations, leading to the exploration of regenerative medicine and integrative therapies. The review emphasizes the potential benefits of tissue engineering, including more effective and less invasive treatment options for myometrial damage. The challenges of developing biocompatible materials and optimizing cell growth and differentiation are discussed. In conclusion, uterine tissue engineering holds promise for myometrial regeneration and the treatment of related conditions. This review highlights the scientific advancements in the field and underscores the potential of tissue engineering as a viable approach. By addressing the limitations of current treatments, tissue engineering offers new possibilities for improving reproductive health and restoring uterine functionality. Future research shall focus on overcoming challenges and refining tissue engineering strategies to advance the field and provide effective solutions for myometrial damage and associated disorders.
GRAPHICAL ABSTRACT
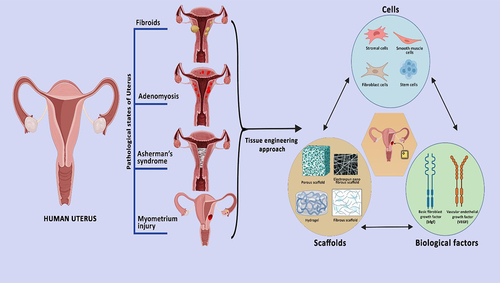
Introduction
1. Uterus
The female uterus is a hormone-responsive tissue that helps the fetus develop by providing it with a healthy environment and nourishment. It is hollow, thick, and pear-shaped situated in the female pelvic area that is angled toward the abdomen [Citation1]. The uterus formation begins between the fifth and sixth weeks of pregnancy by the fusion of the Mullerian or paramesonephric ducts laterally, with the top part remaining unfused and forming the fallopian tube and the lower half fuses and forms the uterus and upper section of the vagina [Citation2]. By 30 weeks Uterine cavity will be visible and the cervical canal will be differentiated. The lack of circulating testosterone and Anti Mullerian Hormone causes uterine development in female embryos [Citation3].
2. Anatomy and physiology
The uterus is divided into four main divisions from top to bottom. The term ‘fundus’ refers to the upper portion of the uterus. The corpus, a wide, curving region, is where the fallopian tubes join to the uterus (body), which begins about at the fallopian tube level and extends downward, the cervix and the isthmus, which is the uterus lower neck, it extends from the isthmus downstream and joins to Vagina. The uterus is situated behind the bladder and in front of the rectum [Citation4]. Uterus undergoes significant changes throughout pregnancy, including hypertrophy and hyperplasia, and it is responsible for fetal protection as well as fetal expulsion at term. Because the uterus is a moveable organ, ligaments are used to support and maintain it in place. Ligaments and folds are classified into two categories. fibromuscular ligaments and peritoneal folds. There are three types of peritoneal folds. Anteriorly, the Uterovesical fold of Peritoneum surrounds the uterus from the junction of the cervix and the uterine body to the top surface of the bladder. The posterior ligament is made up of a retro-vaginal fold that is wrapped to the posterior wall of the uterine body and then extends to the rectum [Citation5]. Another type is fibromuscular ligament divided into three distinct types. Broad ligament, transverse cervical ligament, and uterosacral ligament. The broad ligament connects the sides below and above the fallopian tube to the pelvis, followed by the transverse cervical ligament, which is attached to the cervix and the lateral wall of the pelvis and provides better support to the uterus. Finally, the round ligament keeps the uterus in an anteverted position [Citation6].
3. Layers of uterus
The uterus is a thick-walled muscular structure that has three distinct layers. The endometrium is the innermost layer of the uterus, composed of connective tissue stroma surrounding glands and surface epithelium. Throughout the menstrual cycle, the endometrium undergoes complex changes and serves as the location for blastocyte implantation. The stroma is made up of fibroblast-like stromal cells and extracellular matrix, and stromal cells undergo different morphological stages during each phase of the menstrual cycle. The perimetrium, on the other hand, is the outer lining of the uterus, consisting of a thin layer of loose connective tissue lined by squamous mesothelium. The myometrium is the middle muscular layer of the uterus, providing the bulk of the uterus and is composed of three distinct layers of smooth muscle fibers and nearby neuro-vascular tissues.
The inner and outer myometrial layers are mostly associated with the initial layers of the paramesonephric ducts, with fibers arranged in longitudinal, crisscross and circular directions, respectively as shown in . The middle intermediate layer contributes to the uterine thickness with many blood vessels providing nutritional and nervous supply [Citation7]. Actin and myosin proteins interact to help with uterine contraction by electrical coupling, with changes in extracellular and intracellular calcium transport aiding depolarization and action potential. Gap junctions transform the myometrium during labor into a functioning syncytium, making it easier for contractile excitement to spread across the uterine wall. Hormones, such as prostaglandins and oxytocin, affect intracellular calcium liberation and influence the size of the myometrium during pregnancy [Citation8].
Figure 1. Arrangement of smooth muscle fibers in myometrium - inner circular layer, middle ‘mesh-like’ layer and outer longitudinal layer.

This review article emphasizes the importance of myometrium tissue engineering for repairing damage caused to myometrium due to various conditions. The myometrium is a vital component of the uterus, responsible for contraction and providing structural support during pregnancy. The article highlights the challenges associated with myometrial invasion and the differentiation of its three layers, making it difficult to assess the depth of myometrial invasion. The review highlights the need for further research in myometrial tissue engineering to develop effective treatments for myometrial disorders.
4. Cellular components of myometrium
4.1. Uterine smooth muscle cells
The physiological unit of contraction in the uterine muscles are smooth muscles individually. These muscles are of irregular shape, the size ranges between 5-10 µm in diameter and 300–600 µm in length [Citation9], 1962; During the gestation the consecutive process of hypertrophy occur, which is followed by hyperplasia in smooth muscles of uterus [Citation10]. Till the end of the gestation period, 3–5 folds of hypertrophy is seen. The size of smooth muscles cells changes according to the changing species [Citation11].
4.1.a. Ultrastructure of uterine myocytes
The smooth muscles of the uterus () are elongated cells with a centrally placed nucleus and trilaminar plasma membrane, which acts as a barrier between the extracellular and intracellular environments. Two significant modifications found in the ultrastructure of the smooth muscles are the sarcoplasmic reticulum and the contractile apparatus. In uterine smooth muscles, contraction occurs due to the interaction of thick and thin myofilaments. Three different types of filaments have been identified () [Citation12–14]. The cytoplasm of smooth muscles contains myosin filaments, tropomyosin, filamin, caldesmon, and intermediate filaments composed of vimentin and desmin, which form the cytoskeleton [Citation15]. The myofilament arrangement is random, which opposes the rate of development of power in striated muscle cells. The dense body ctactinin binds with vinculin, and this vinculin further binds with the thin filaments. Thin filaments are found inserted in thick cytoplasmic patches which are present associated with dense bodies of the plasma membrane. The sarcoplasmic reticulum found in the uterus is not well-developed, but a dense network of sacs and tubules are present in this region, and calcium is found accumulated in the sarcoplasmic membrane [Citation16]. After estrogen therapy, the endoplasmic reticulum of uterine smooth muscles shows higher importance of rough endoplasmic reticulum and increased rate of protein synthesis. Other essential cell organelles such as mitochondria, nuclei, and Golgi bodies are also found in this region [Citation17,Citation18].
4.1.b. Coupling of uterine smooth muscles
During the binding of the uterine muscles, the neighboring cells of the muscle bundles come closer together. The smooth uterine muscles contain gap junctions, which are present in nearly all tissues. These gap junctions appear as two membranes bound together with a small gap of 2–3 nm when viewed under an electron microscope. They are composed of a large number of channels and hemichannels. The uterine muscles contain a 43kDa cx-43 protein, which is responsible for the formation of these channels. The channels are made up of small molecules, inorganic ions, amino acids, and connexions [Citation19,Citation20]. Gap junctions are abundant in uterine muscles and help the cells to work metabolically and electrically. Uterine smooth muscles exhibit spontaneous activity, which is characterized by repolarization and depolarization. During pregnancy, a single plateau type action potential is observed in the circular muscles, while variations in action potential, similar to that of longitudinal muscles, are seen during labor. The plasma membrane of the smooth muscles has a potential difference of around −70 mV during quiescence, which drops during depolarization due to increased Ca2+ and Na+ permeability [Citation21]. The myometrial cells have a fast stimulation rate, resulting in increased synchronous stimulation of the large area of the uterus, which leads to an increase in the contractile force achieved. During an action potential, a discharge rate of one cycle per second is seen, and a fused tetanic type contraction is produced due to the summation of twitch contraction and repetition of the generation of the action potential caused by increased intracellular calcium concentration. A twitch contraction is generated by a single action potential [Citation11,Citation22,Citation23].
4.1.c. Gestational regulation of calcium transport
In earlier reported articles, it has been seen that many articles are gestational regulated [Citation24]. In rat uterine muscles cells high density of calcium channels are seen during pregnancy [Citation25]. Through RT PCR demonstration of Ca2+ gated ion channels, increased activity was found during the preterm labor in the myometrium of rats [Citation26]. This increased activity was seen due to withdrawal of the progesterone hormone, it also increases muscular contraction during labor due to increased activity of Ca2+ ions. Prostaglandin and carbachol are uterine muscle stimulants. During advanced gestation, the G protein mediate sensitivity was found increased in the contractile elements for calcium ions [Citation27]. The hormone level affect sarcolemma activity due to Mg2+, Ca2+ and ATPase efficiency in uterine smooth muscles [Citation28]. At the end of the pregnancy Na+ level found to be increased and during the advance pregnancy secretion of oxytocin show an inhibitory action on the Ca2+ pump in sarcoplasmic reticulum and sarcolemma [Citation29]. All these channels play an important role in the regulation of Ca2+ gated ion channels during advance pregnancy. All the above-mentioned factors are important in regulation of Ca influx across the uterine membrane, it also promotes myometrial contraction and labor.
4.2. Stem cells in myometrium
The presence of myometrial and endometrial stem cells can be indicated mainly from how the uterus enlarges, remodels and regenerates during pregnancy and during menstrual cycle, as shown in . It can be noted that such uterine remodeling occurs cyclically and repeatedly throughout a woman’s reproductive life, and these properties have paved ways to studies to prove the existence of endometrial and myometrial stem cell systems. Tissue-specific adult somatic stem cells from the myometrium, help in the remodeling of tissues, though they do not have specific markers, they cannot be defined and identified easily due to its scarcity, distinct characteristics studied that Oct-4/CD44/Nanog can serve as cell surface markers for identifying a subset of murine myometrial cells. These cells demonstrate characteristics of stem/progenitor cells and their abundance increases with reproductive maturity and the elevation of serum estrogen and progesterone levels, indicating the presence of myometrial stem cells, which are also hormone dependent [Citation30,Citation31]
Figure 4. Proposed model for the possible role of putative myometrial stem cells in pregnancy-induced uterine enlargement and pathogenesis of leiomyoma [Citation30].
![Figure 4. Proposed model for the possible role of putative myometrial stem cells in pregnancy-induced uterine enlargement and pathogenesis of leiomyoma [Citation30].](/cms/asset/96ab7454-4a5f-4d9e-a73d-19f148ea3e90/kbie_a_2251847_f0004_b.gif)
Ona et al (2015) tried to establish a surface marker definition for myometrial stem/progenitor cells, through side-population and main population studies and identified the expression of CD49f and CD34 on the side population myometrial cells. Since these two surface markers, have significance in the identification of hematopoietic stem cells, along with mesenchymal stem cells (MSCs), embryonic fibroblasts, interstitial dendritic cells, vascular endothelial progenitors, and epithelial progenitor, it can be indicated that the likelihood of CD49f and CD34 could potentially be surface markers for stem/progenitor cells of myometrium.
The presence of myometrial stem cells was possible using various surface markers that were possibly identified from isolation of human endometrial stromal colony-forming cells (putative endometrial stromal/progenitor cells). Though these proved as an evidence of the existence of the myometrial stem cells, but defining and identifying a definitive set of surface markers is necessary to identify and isolate both myometrial and endometrial stem cells in order to understand uterine tissue regeneration in a better manner. The complete characterization of uterine stem/progenitor cells will help in understanding the mechanisms involved in physiological regeneration of the female reproductive tract [Citation30,Citation32].
4.3. Telocytes in myometrium
Telocytes are a kind of interstitial cell that coexists with myometrial cells. These telocytes are small, oval, or triangular-shaped structures, which act as a scaffold that helps during tissue repair/renewal. They have specific long prolongations called telopodes, which have connections with neighboring cells. These cells are also found in the stroma of several other organs like heart, skeletal muscles, certain blood vessels, uterus, placenta, GIT, small intestine, and lungs [Citation33]. However, the functional role(s) of TCs is not yet understood; but studies based on photomicrographs as illustrated in did show the entire length of these telopodes, along with highlighting the podoms (dilations) and podomers (thin segments) that help in branching and establishing homo- and heterocellular contacts [Citation34].
Figure 5. Representative ultrathin section of human pregnant myometrium. SMCs are shown in cross-section and were digitally colored brown. In their vicinity, numerous Telocytes are observed (blue) [Citation35].
![Figure 5. Representative ultrathin section of human pregnant myometrium. SMCs are shown in cross-section and were digitally colored brown. In their vicinity, numerous Telocytes are observed (blue) [Citation35].](/cms/asset/9962e4e6-6611-4c0c-9dcf-6c02d2c81629/kbie_a_2251847_f0005_oc.jpg)
Telocytes, being the recent study of interest, also do play a role in the understanding normal physiology and pathology of Uterus. Especially, in myometrial contractility, and immune responses. In the development and secretions of the placenta, they do play a role toward angiogenesis and tissue development and help in controlling of the myometrial muscle tone and motor activity. Telocytes can be regarded as the ‘hormonal sensors’ in human uterus, as they do help in endometrial growth and differentiation in a hormone-dependent manner, causing hyperplasia that helps toward cell migration and proliferation of myometrial tissue.
Studies to contribute insights from genetic and immunologic profiling of TCs do provide a window of opportunity to explore its paracrine effects and activities along with immune cells. Especially, the Telocytes applications in regenerative medicine can serve an area of interest toward uterine tissue regeneration and in tissue engineering applications [Citation36].
5. Muscle fiber orientation and mechanical and elastic property of myometrium
Myometrial tissue contractility and its mechanical properties are being studied to monitor the uterine activity during labor/pregnancy and in general as well. In the uterine smooth muscle cells, similar to the skeletal, cardiac and other visceral smooth muscles, Calcium (Ca) is required for contractions. where there is spontaneous depolarization, either due to calcium influx/efflux or through membrane activity that helps in contraction and relaxation of uterine muscles.
Izumi (1985) illustrated in his paper, which the resting membrane potential of longitudinal muscle cells in the rat myometrium elevated until the final stage of gestation and then declined. The action potential generated has an impact on the shape of the myometrium’s longitudinal and circular muscles during gestation, and these changes differ in the final stage and during parturition, and can modify the Ca-influx for the uterine smooth muscle; contractile proteins like myosin and calmodulin help in increasing the cell volume and are essential factors for the production of smooth muscle contraction, where the contractile force, i.e., the Ca-sensitivities [Citation26].
Mariatou et al hypothesized and studied that the structural and functional variations in the inner and outer layers of the myometrium can be connected with a variance in elastin distribution in women of reproductive age, according to magnetic resonance imaging and transvaginal ultrasonography. They used immunocytochemistry, orcein staining, and image analysis to examine 51 hysterectomy specimens that represented all phases of the normal menstrual cycle. They discovered that elastin was more prevalent in the outer myometrial smooth muscle and less prevalent in the inner smooth muscle. Elastin was found in the endometrial and myometrial arteries, arterioles, and surrounding bigger vessels, according to immunoreactivity and staining. This study suggests that Elastin is also a contributing factor along with Ca-sensitivity that helps in the contraction and in understanding the elastic property of myometrial muscles [Citation37].
6. Myometrium diseases
Adenomyosis, fibroid, and malignancy are among the different myometrial diseases. Adenomyosis is known as a benign disorder of the uterus. In this disorder, the stroma and endometrial glands are verified pathologically in the myometrium [Citation38]. Adenomyosis is a disorder in which most of the affected woman are asymptomatic, a few have symptoms such as dysmenorrhea, abnormal bleeding through the uterus, infertility. and dyspareunia [Citation39]. The patients with adenomyosis suffer from heavy pelvic and menstrual pain for years, it has been diagnosed in histopathological studies after hysterectomy of the perimenopausal women [Citation40]. Adenomyosis is also identified in young women [Citation41]. In a few patients, fibroid and endometriosis is also present with adenomyosis. There are currently no international guidelines available for the treatment of adenomyosis. While the pathogenesis of this disease has been studied for several decades, the prognosis of the disease is largely dependent on factors such as inflammatory receptors, neuroangiogenic factors, and growth factors [Citation42]. Due to the invagination of the basalis endometrium in the myometrium via an interrupted junction zone adenomyosis appear, this special junction zone is the hormone responsive structure found in the inner region of myometrium [Citation39].
For survival of the myometrial interface eutopic endometrium contribute due to several molecular changes [Citation43]. In both eutopic endometrium and adenomyosis lesions extracellular pathway through the matrix, responsiveness toward the hormones and altered apoptosis process play major role. To explain this, role of the hypothesis in support of tissue repair and injury of the myometrial tissue is most accepted [Citation44]. In chronic peristaltic myometrium the contraction of the wall of myometrium induce the microtrauma of the junctional zones, which further cause inflammation and it promote increased secretion of the estrogen, which results in induced vicious cycles. This autotraumatization in chronic hyperperistalsis in the junctional zones is repeatedly promoted by positive feedback mechanism. The transuterine infiltration and repeated microtrauma (TIAR) theory promote that adenomyosis is related to the history of C section, multiparity and prior uterine surgery [Citation40]. However, to better understand the disease its onset time, prognosis and associated symptoms are required to understand it.
6.1. Effect of adenomyosis on infertility
Infertility appears as a result of adenomyosis in multiparous women, it can also be termed as reproductive failure [Citation40]. A cross section study published on adenomyosis reported appearance of infertility in 24.4% woman at 40 years of age and 38.2% women below 40 years of age [Citation45]. One cause of infertility seen is abnormal uterotubal transport, which leads to infertility caused due to the distortion of the uterine cavity cause disturbed sperm transfer and peristalsis [Citation46]. Hyperperistalsis dysfunction in the junctional zone causes an increase in pressure inside the uterus cause disturbed myocyte contraction. which further results in the loss of normal contractions in uterus [Citation47].
A wide variety of molecular contraction is also seen in infertile women. In women suffering from adenomyosis infertility is caused because of increased inflammatory markers, lead expression of the adhesive molecules, changed gene function, increased oxidative stress, increased inflammatory markers and transformed pathway of sex hormones [Citation48]. Through a study published in IVF Cases, it has been found that the probability of clinical pregnancy decrease upto 42.7% in women with no adenomyosis, it decreases upto 13% in women gone for ultrasound for the seventh time [Citation49]. Thus, it attributes toward the morphological features of ultrasound worsen the reproductive outcome, i.e., higher is the frequency of ultrasounds less will be the probability of conception.
6.2. Benign and malignant pathology of the uterus
‘Fibroids’ is a disease that affects myometrium tissues and are estimated to appear in 20–40% of women in their reproductive age. Fibroids can vary greatly in size and volume. They can be evaluated based on 4 categories of baseline sizes. 1) Very small (0.5 to 0.9 cm), 2) Small (1 to 1.9 cm), 3) Medium (2 to 2.9 cm), and 4) Large (>4 cm) [Citation50]. The cause of fibroids is not known, but improved scanning technology has made diagnosis easier, and these techniques can also provide information about changes in endometrial function and myometrial contractility. Abnormalities are present in 50% of fibroid cases on cytogenetic evaluations, were resultant of single clone of the smooth muscles. It includes trisomy of 10q, monosomy of 12q and deletion of the 3q with the intramural subtype showing the highest percentage [Citation51–53].
Fibroids are more likely to appear in women with a higher BMI, but multiparous women are less susceptible [Citation54,Citation55]. Symptoms of fibroids can include abdominal and pelvic pain, abnormal bleeding, dysmenorrhea, miscarriages, bloating, and discomfort. Abnormal uterine bleeding is common in fibroid patients, and menorrhagia is more complex in fibroids due to several factors. Hyaline degeneration is a common degenerative process seen in fibroids [Citation50,Citation55,Citation56]. Different types of fibroids require different methods of treatment. Pedunculated fibroids are easily removed through ** [Citation57]*** myomectomy, while subserous fibroids are removed through an incision above the bulging portion [Citation58]. Intramural fibroids larger than 5 cm in diameter require a different type of myomectomy due to their risk of complications. The location of these fibroids can be determined using 4D ultrasound, and pre-operative administration of GnRHa can reduce their volume. A vasoconstrictor injection can also decrease complication rates, but intramural fibroids with adenomyosis and an overdose of GnRHa can worsen the condition.
7. Wound healing in myometrium
Myometrium tissue damage can be in the form of scars and wounds. The scars are the resultant of cesarean. It is difficult to find the samples for hysterotomy scars to understand the sequence of modifying post-partum in the uterine wounds after cesarean delivery. For the maintenance of organ integrity remodeling of mammalian tissue is very important. After cesarean delivery and myomectomy of fibroids a series of events occur to heal the wound occurred in the myometrium and remodeling of ECM takes place. There are various proteins and peptides involved in the process of wound healing in myometrium. There are mainly three complex steps involved in the healing process [Citation59].
1. Phase 1 –inflammatory phase
This process starts and continues up to 72 h, it will have symptoms such as pain, redness swelling. When exudates and edema accumulate, a blood clot develops and closes the region. In the initial 2 h following a muscular injury, there is a myofilament response accompanied by contraction of peripheral muscle fibers. Within 24 h, edema and anoxia lead to cell damage and death. Protein breakdown products released from injured cells further contribute to edema, tissue hypoxia, and cell death. Subsequently, phagocytosis initiates the removal of cell debris and edema from the affected area
2. Phase 2 - tissue formation
Muscular regeneration and repair take place during the fibro-elastic/collagen-forming phase, which can last anywhere from 48 h to 6 weeks. Structures are rebuilt and regeneration happens throughout this time. At 4 days, fibroblast cells start producing Type III collagen, and fiber structure is random and immature. Capillary budding takes place, delivering nutrients to the area, and collagen cross-linking commences. The number of fibroblasts reduces as the process of collagen remodeling progresses. This phase concludes with the onset of wound contracture and the narrowing of the wound margins.
3. Phase 3- tissue remodeling
The remodeling phase, which can span anywhere from 3 weeks to 12 months, is the final stage of recovery. The development of a tight, durable scar is aided by the cross-linking and shortening of the collagen strands over time. This stage sees the final aggregation, orientation, and organization of collagen fibers. Because fibrous scar tissue inhibits muscle recovery, regeneration of the damaged muscle does not completely return the muscle to its earlier state. Complete regeneration is hindered because the two processes of fibrosis and healing compete with one another [Citation60]. Growth factors such as transforming growth factor-beta 1 (TGF-b1), a common molecule that starts a chain of events that activates both myogenesis and fibrosis, and decorin, a proteoglycan that prevents fibrosis by attaching to TGF-b1, are responsible for catalyzing many of these processes [Citation61,Citation62]. These growth factors are under active investigation to determine their role in muscle healing [Citation63]. Incomplete wound healing form large scar defects, which further complicates the future pregnancies and increases the risk of uterine rupture, placenta accreta and placental abruption [Citation64].
8. Current methods of treating myometrium damage
Generally patching is used despite of regeneration and repair of the tissue. In the cases where wound healing is maximum, the fibroproliferative response of the wound repair is dominant. It has been analyzed that women given vaginal birth after cesarean are more prompt to rupture of the uterine walls. A study published in C57/BL6 mice proposed significant remodeling of the scars on 15th day after Cesarean Delivery (CD), this duration was similar to 7.5 months of humans. The biochemical endpoints were found in the uterus of mice even after 60 days, equivalent to 2.5 years in human. For remodeling of such CD scars a newly developed noninvasive technique is required [Citation65].
Another report published on the healing of the uterine walls in comparing with other tissues. A total of seven female subjects were recruited for the study, all of whom presented with injured myometrial tissue. They were suffering from heavy bleeding and pain followed by iatrogenic myometrium injury. On histopathologic study appearance of fibroids, keloid-like structure and scars were seen on the site of wounds. The elastosis and myofiber disarray were seen as marker of aberrancy in the existing wounds after iatrogenic trauma of myometrium [Citation66]. Lavaging is frequently used to treat uterine wounds with saline solution. It has been seen that after lavaging the wounds for three times, healing of the dehiscence muscular occurs successfully. For healing the myometrial tissue, lavaging, and layering were done continuously for eight months [Citation67].
9. Tissue engineering of uterus
To address the challenges associated with dissociated cell injection, the field of tissue engineering has emerged as a promising approach for the next generation of cell therapy. Tissue engineering aims to create functional tissues or organs by combining cells, biomaterials, and biochemical cues in a controlled environment [Citation68]. Tissue engineering and regenerative medicine (TE & RM) have been developed as a new field of biomedical engineering with a modern concept of tissue healing and regeneration. TE & RM works on various approaches, i.e., 1) it can be implemented directly by using cells isolated from the patients; 2) Another method that is being studied extensively is the ‘scaffolding biomaterial’ which is a cell-free approach, where the cell-laden scaffolding structure mimics the extracellular matrix of the tissue, 3) through the growth factors and bioactive molecules targeting tissue specificity [Citation69]. Scaffolds are designed from various materials which include the use of decellularized matrix, natural as well as synthetic polymers, which is being discussed in this review.
9.1. Cell-based therapy
Cell-based therapy, also known as regenerative medicine, utilizes living cells to restore or enhance the function of damaged tissues or organs. It shows promise for treating conditions such as Asherman’s syndrome, thin endometrium and uterine scars [Citation70–72]. The therapy relies on the regenerative capabilities of cells to repair tissues. Stem cells, immune cells, and specialized cells can be employed in these treatments. One of the studies investigated the therapeutic effects of Bone marrow mesenchymal stem cells (BMSCs) and TGF-β3 on regenerative injuries in rat myometrium. BMSCs showed potential for differentiation into uterine smooth muscle cells and promoted healing of uterine scar tissue. The combination of BMSCs and TGF-β3 resulted in improved repair and enhanced function of the myometrium compared to other groups. TGF-β3 also reduced collagen fiber production [Citation73].
In another study, Bone marrow mesenchymal stem cells (BMSCs) were utilized to enhance a thin endometrium. The BMSCs were directly administered into the uterine cavity. The experimental group of rats displayed a significantly thicker lining in the endometrium compared to the control group. Moreover, the experimental group exhibited higher levels of cytokeratin, vimentin, integrin αγβ3, and leukemia inhibitor factor (LIF) expression. Following BMSC transplantation, the presence of Bromodeoxyuridine-positive cells was observed in the endometrium. In summary, the migration and immunomodulatory properties of BMSCs likely contributed to the improvement in endometrium thickness [Citation74].
9.2. Scaffold based approach
Scaffolds are fabricated using diverse techniques such as electrospinning and 3D bioprinting. Electrospinning involves the use of an electric field to create ultrafine micro to nanofibers from a polymer solution or melt. This technique can produce scaffolds with a high surface area and controlled pore size, mimicking the natural extracellular matrix. Electrospun scaffolds offer a favorable microenvironment for cell attachment, proliferation, and differentiation [Citation75,Citation76]. Few studies have utilized 3D bioprinting technique for uterine tissue engineering. Use of 3D bio-printing has been increasing from a decade and its often used in various industries. They are used for their ease of fabrication, and has added to considerable advancement for healthcare in regenerative medicine [Citation77]. 3D bioprinting technique is based on the fundamental concept of biomimicry, self-assemble and use of mini tissues as building blocks. Biomimetic components show dynamic effects on the construction of bioprints for its migration, attachment, function and proliferation of both endo and exogenous cells [Citation78–80]. Some of the different types of biomaterials used for scaffold fabrication are discussed in detail.
9.2.1. Decellularized extracellular matrix (dECM) scaffolds
ECM mainly consists of collagen, elastin, fibronectin, laminin, and matricellular proteins where each protein has specific advantages such as collagen provides mechanical strength, elastin provides elasticity, fibronectin provides regulation to cell behavior and laminin acts as binding site. Some studies [Citation81] explored the possibility of applying this decellularized extracellular matrix to uterine regeneration; it is a method used to create a structural ECM framework by removing cellular components from organs, tissues, and cells in order and maintain a nanostructured ECM microenvironment. dECMs are classified based on the source such as organ/tissue derived and cell-derived matrix.
Myocytes derived from rat and humans were used in allo- & xenogeneic approaches, where a neo-myometrial patch was derived that can be explored as a treatment option for uterine defects. In this study, isolated human and rat myocytes were cultured alternatively on decellularized rat and human scaffolds in allogenic and xenogeneic manner where the xeno-neo-myometrial patch showed better myocyte contractions, that can be used to treat uterine defects [Citation82].
Hellstorm [Citation83], and Santoso et al [Citation84], studied how a decellularized uterus can be transplanted and used to treat absolute uterine factor infertility; where these scaffolds were recellularized and transplanted into rats, and they noted that there was no significant changes in collagen and elastin compositions, and there were no rejection of ovarian hormones and these studied yielded positive results contributed toward treatment options through decellularization-recellularization of scaffolds for uterine transplantation options. In 2016, Hellstrom et al [Citation85], used their optimized decellularization method and recellularized it with primary uterine cells and also fluorescently tagged mesenchymal stem cells to develop a patch to repair partially defected uterus in rats as well as to check the support to pregnancy. The results showed that though there was fetal development in over the patches, the study needs to be further explored to gain a determined result on the possibility of creating a complete bioengineered uterus. Similar experiments were conducted by Tiemann et al, using sheep uterus to study the recellularization of cells on these patches. In this study, three different types of decellularization protocols using 0.5% SDS, 2% SDS, and sequential perfusion of 2% SDS and 1% Triton X-100 are used. The scaffolds are then characterized for integrity, and it is identified that protocol 2 (2%SDS) is the most favorable treatment for tissue integrity out of all protocols. But all three treatment groups promote cell viability and proliferation [Citation86].
Qing Yao. et.al, developed a decellularized rabbit uterus (dUECM) for xenotransplantation through crosslinking groups for rat uterus regeneration and reconstruction; the study showed promising results in-vivo and in-vitro with good cytocompatibility and reduced host immune reactions, good mechanical strength, good enzymatic degradation rate, excellent biocompatibility, yielding toward ideal applications for uterus regeneration and construction [Citation77].
In 2021, Arezoo et al, studied how stem cells derived from menstrual blood cells when recellularized on mouse decellularized uterus can help toward achieving the different potential cell phenotypes on these decellularization scaffolds [Citation80]. Thus, these studies proved an evolving tissue engineering method that can contribute toward whole human uterus bioengineering.
9.2.2. Polymer-based scaffolds
9.2.2.a. Natural Polymers
Existing macromolecules that are present in ECM constitute natural biomaterials. Collagen, gelatin, hydrogels made of hyaluronic acid, fibrin, glycosaminoglycans, alginate, Matrigel, silk, hydroxyapatite, and other substances are among them. These materials have particular benefits, such as mechanical and adhesion qualities similar to those of natural ECM. illustrates the utilization of natural biomaterials possessing strong biocompatibility in several studies to develop scaffolds for uterine tissue engineering [Citation98].
Table 1. The use of collagen-based scaffolds in uterine tissue engineering.
Collagen is used extensively in uterine tissue engineering studies because of its advantage in maintaining the biological and structural integrity of the extracellular matrix, and its high abundance, low antigenicity, and mostly on the fact that myometrium consists of collagen in high amounts. Shuong Hong Lü et al (2009) conducted a study with the help of bionics and studied how engineered uterine tissues (EUTs) can produce the same physiological environment as that of in-vivo endometrial layer. This study paved way to study uterine diseases and embryonic development though EUTs. In this study, smooth muscle cells were mixed with Collagen and Matrigel and seeded to give a stromal layer, which was studied further by seeding epithelial cells on it. Similarly, the smooth muscle cells were also mixed with Matrigel to form a layer, and then both stromal & epithelial cells were seeded on this layer. In the first technique, embryos were cultured and studied, which showed blastocysts development, but further studies must be done to investigate embryonic implantation in the EUTs [Citation99].
Recently, Mesenchymal Stem cells (MSCs) are being used as cell therapy and for tissue regeneration for the treatment of uterine diseases. MSCs can be categorized as coming from bone marrow, umbilical cord, adipose tissue, or human menstrual blood, depending on their cellular source. Because of their ease of collection, minimal immunogenicity, and high proliferative capacity, umbilical cord derived MSCs (UC-MSCs) have been viewed as a prospective source for cell-based therapy. Lijun Ding et al, transplanted bone marrow mesenchymal stem cells (BM-MSCs) on collagen scaffolds, to replace damaged endometrium in rat’s uterus. The collagen scaffolds were degradable, provided a 3D structure for proper adherence, proliferation, and migration without impairing the stem cell genes. This acts as a potential method to explore further to study embryonic development [Citation100].
Song et al experimented with the regeneration of uterine endometrium for cell replacement therapy by generating endometrium-like cells from human embryonic stem cells (hESCs) and seeded these on a collagen scaffold to replace damaged uterine tissue. The differentiation of the hESCs was induced by coculturing them with endometrial stromal cells, to which other cytokines such as epidermal growth factor, were added. The hESC-derived endometrial cells were then shifted to a collagen scaffold which was transplanted to an animal model (rat). It was observed that the hESC-derived cells survived and recovered the replacement of uterine horns in a rat with severe uterine damage [Citation96].
Wanqing Ji. et. al have used human induced pluripotent stem cells derived from mesenchymal stem cells (hiMSC) and loaded it to a sodium alginate hydrogel and obtained scaffolds using 3D bioprinter. The hydrogel scaffolds obtained indicated permissive in-vitro living environment and high survival duration for hiMSCs when compared to in vivo conditions. They concluded that the hiMSC hydrogel scaffolds played an important role in repair and histological structure of a damaged endometrium and restoring the abilities to support embryos. Development of this multidisciplinary technology can effectively solve many problems in near future and may be administered to patients with endometrial injuries in the future [Citation101,Citation102].
9.2.2.b. Synthetic polymers
Synthetic Polymers can be tailored with customizable design to exhibit varied capabilities and be standardized for mass manufacturing, synthetic polymer scaffolds have shown significant promise in tissue engineering. illustrates the utilization of synthetic biomaterials in several studies to develop scaffolds for uterine tissue engineering.
Table 2. The use of synthetic scaffolds in uterine tissue engineering.
Xiao et al [Citation103] studied Intrauterine adhesion using Poly (glycerol sebacate) (PGS), as it has robust elasticity that can provide insights for uterine tissue engineering. In this study, PGS scaffolds were fabricated with BMSCs to prevent intrauterine adhesion, the study was designed to prove that PGS/BMSCs scaffold showed better results to restore the morphology and function of the injured rat uterus compared with other methods. The intrauterine adhesion was also studied using other polymers like collagen, poly (lactic-co-glycolic acid) (PLGA), and with phosphate buffered saline (PBS) as well. The results showed that PGS/BMSCs but not PLGA/BMSCs and collagen/BMSCs could prompt the restoration of endometrium by directly differentiating into endometrial stromal cells. Collectively, PGS/BMSCs and collagen/BMSCs exhibited comparable ability to increase the pregnancy rates of uterus following injury, but significantly stronger than PLGA/BMSCs.
In 2020, Magalhaes et al [Citation75] studied that PLGA/PGA scaffolds combined supports live birth in rabbits and described that the in vitro fabrication of engineered uterine tissue by using electrospinning technique scaffolds and seeding primary uterine cells and implanting in the rabbit model. Over the time, the engineered uterine tissue formed patent cavities, organized cellular and anatomical structures inside the endometrium and myometrium compartments. Reproductive studies showed that autologous tissue-engineered constructs supported late-stage pregnancies and viable live births.
In a study conducted by Hanuman et al [Citation104], () the potential of Polycaprolactone (PCL) in the tissue engineering of myometrium was investigated. The study was conducted in two parts. In the first part, the research group explored the potential of aminolysis modification of PCL. Electrospun PCL, being an elastic polymer, exhibits promise for uterine tissue engineering, but its hydrophobic nature requires further modification. To address this issue, the study employed aminolysis surface modification techniques and in vitro characterization using human uterine fibroblast cells. The results of this part showed that the aminolysed PCL nanofiber demonstrated increased uterine cell adhesion and proliferation when compared to non-modified PCL nanofiber.
Figure 6. A) Electrospun Polycaprolactone nanofibers were surface modified and seeded with human uterine fibroblast for cytocompatibility studies. a) MTT assay for checking proliferation of cells on scaffolds and b) live/dead assay of human uterine fibroblast cells on modified nanofibrous surface a) TCPS, (b) PCL, (c) APCL and (d) MPCL scaffolds [Citation76].
![Figure 6. A) Electrospun Polycaprolactone nanofibers were surface modified and seeded with human uterine fibroblast for cytocompatibility studies. a) MTT assay for checking proliferation of cells on scaffolds and b) live/dead assay of human uterine fibroblast cells on modified nanofibrous surface a) TCPS, (b) PCL, (c) APCL and (d) MPCL scaffolds [Citation76].](/cms/asset/332cca24-c99a-4cd4-a55a-5a6deff6f8ca/kbie_a_2251847_f0006_oc.jpg)
In the second part of the study, Hanuman et al [Citation76] conducted two step wet chemistry surface modification by conjugating maltose sugar on aminolysed scaffold to enhance cell adhesion and extracellular matrix remodeling. The results of this part showed that the maltose-conjugated PCL nanofiber demonstrated greater uterine cell adhesion and proliferation when compared to aminolysed PCL nanofiber. Based on these results, the study concluded that aminolysed and maltose-conjugated PCL could be utilized for in vivo uterine tissue regeneration.
9.3. Biological factors for myometrial tissue engineering
Li et al (2011), conducted a study using the importance of scaffolds through delivery of biological factors like Basic fibroblast growth factor (bFGF), a potent mitogen that promotes the growth of many cell types, including fibroblasts, vascular endothelial cells, and smooth muscle cells, crucial for tissue regeneration and wound healing. However, studies have shown that bFGF is also crucial for endometrial proliferation, remodeling, and angiogenesis during the menstrual cycle in the female reproductive system.
To stimulate angiogenesis and wound repair, however, the amount of endogenous bFGF generated during wound healing is insufficient. In this study, collagen-based delivery of bFGF was explored, and was noted that it showed great effectiveness and efficiencies in the regeneration of uterine horns in rats and could have a potential clinical application in uterine regeneration [Citation105]. Similarly, Vascular endothelial growth factor (VEGF) which is an angiogenic growth factor, is critical for the migration and proliferation of endothelial cells and formation of new vessels. VEGF is conjugated to collagen and studied. The Collagen–VEGF combination promoted remodeling of the scarred uterus and improved uterine function in the full-thickness rat uterus injury model. Collagen-VEGF injection at scar sites can be a pragmatic solution for the treatment of severe uterine damages [Citation93].
10. Challenges of myometrium tissue engineering
Myometrium is a highly vascularized tissue; the muscles of myometrium must contract rhythmically in sync acting as a stop valve by default to stop any excessive bleeding occurring due to injury or childbirth. Myometrium is the middle layer of the uterus with muscles arranged in 3 different criss-cross directions (oblique, transverse, and longitudinal), thus it is difficult to differentiate each of these 3 layers microscopically or through imaging.
Myometrial invasion is difficult, and the irregularity of the normal endomyometrial junction may make it difficult to determine the exact superficial reference point for measuring and assessing the depth of myometrial invasion. A specific issue is the formation of abnormal endometrial stroma, which may be made of spindly eosinophilic cells and mimics myometrium. Failure to detect this form of stroma may result in the incorrect diagnosis of myometrial invasion. High-powered examination often reveals smooth transitions from typical-appearing endometrial stroma and a contrast between the fibrillary look of altered stroma and the well-organized bundles of myometrium. During adenomyosis, the layer separating endometrium and myometrium lacks a submucosal layer allowing endometrium to penetrate myometrium during pregnancy, hence making in difficult to differentiate the layers.
In vivo models are, in general, costly, low-throughput, and time-consuming. Also, ECM (extracellular matrix) composition is misinterpreted at times. Usually, ultimate cure for any disorders of the uterus is hysterectomy to avoid recurrence of the problem and control post-partum hemorrhage; as a result, this could also be a reason as to why tissue patches for myometrium have not yet come into the market as hysterectomy can be efficiently performed.
Future scope
Uterus is a hollow organ with thick walls, capable of contraction. These are designed especially for adaptation and implantation of the embryo to develop it in the fetus. Myometrial tissue engineering is an emerging and challenging field, which can work as a boon for treatment of several infertility problems. Two methods of myometrium engineering are used, they are scaffold tissue engineering using biomaterials and 3D bioprinting. 3D bioprinting has emerged as a new important tool in the field of myometrial tissue engineering. The future of myometrial tissue engineering is now based on TE-RM. One possible solution to address the issue of myometrial disorders in women of fertile age who cannot undergo hysterectomy is the development of tissue-engineered myometrial patches. These patches could potentially be used to repair or replace damaged or diseased myometrial tissue, allowing for the restoration of normal uterine function and avoiding the need for hysterectomy.
Although the development of tissue-engineered myometrial patches is currently in its nascent stages, ongoing research in this field is demonstrating promising outcomes and holds the potential to provide a minimally invasive therapeutic intervention for women with myometrial disorders who desire to retain their fertility.
An ideal scaffold for uterine tissue engineering must have a number of characteristics, depicted in . During menstruation, the uterus expands and contracts, and during pregnancy, its size changes. Therefore, it is essential to design an adaptable elastic and fatigue resistance scaffold that can tolerate contraction and relaxation without losing structural integrity [Citation106]. Furthermore, given that the uterus is a hormone-responsive organ with a vascular structure and an abundance of glands, the scaffold should have a sufficient amount of porosity. This porosity allows for the correct flow of nutrients, hormones and oxygen, which is necessary for effective tissue regeneration [Citation107]. The uterus comprises three distinct layers, so a novel approach to design scaffolds that either replicate these layers or mimic the extracellular matrix, to enable more efficient cell recellularization could be attempted. Subsequently, the ideal scaffold must have good biocompatibility to ensure that it is well-tolerated by the host tissue and promotes effective integration and recellularization. In summary, an optimal uterine tissue engineering scaffold must possess appropriate elasticity, porosity, and a biomimetic structure, combined biocompatibility to provide a comprehensive solution for effective uterine myometrium tissue regeneration.
The major challenges include optimizing the conditions for cell growth and differentiation, ensuring vascularization, and promoting seamless integration with the native tissue. However, continued advancements in tissue engineering techniques and biomaterials may enable the realization of tissue-engineered myometrial patches as a viable solution for the management of myometrial disorders in women of reproductive age.
Conclusion
In summary, this review has discussed the challenges associated with myometrial tissue engineering, including the complex microstructure and functionality of the myometrium, difficulties in imaging and diagnosis, and the lack of suitable animal models. The review has also highlighted the potential of tissue-engineered myometrial patches as a therapeutic option for women with myometrial disorders who desire to preserve their fertility. Various approaches have been explored in the development of tissue-engineered myometrial patches, including the use of natural and synthetic biomaterials, scaffold design, and surface modification techniques.
While the current research shows promise in addressing the challenges of designing and fabricating functional myometrial tissue that can be implanted within the uterus. Ongoing advancements in tissue engineering techniques and biomaterials may facilitate the realization of tissue-engineered myometrial patches as a viable solution for the management of myometrial disorders in women of reproductive age. However, further research and development are required to optimize the conditions for cell growth and differentiation, ensure vascularization, and promote seamless integration with native tissue.
Author contribution statement
M.N. and G.P. conception and design, S.H. analysis and interpretation of the data; S.H. drafting of the paper, M.N. and G.P. revising it critically for intellectual content; and M.N. final approval of the version to be published; and that all authors agree to be accountable for all aspects of the work
Acknowledgments
M.N. acknowledges the Department of Science & Technology, India for the SERB start-up research grant (SRG/2019/002130) and SERB-POWER research grant (SPG/2021/003703) for financial support. S.H. acknowledges Intramural grant (MAHE/DREG/PHD/IMF/2019) and Dr. T.M.A. Pai Ph.D. Scholarship from the Manipal Academy of Higher Education (MAHE). The authors also thank the Manipal Institute of Regenerative Medicine, MAHE for the infrastructural support.
Disclosure statement
No potential conflict of interest was reported by the author(s).
Additional information
Funding
References
- Ameer MA, Fagan SE, Sosa-Stanley JN, et al. StatPearls [Internet]. 2022 Feb 23 [cited 2022 Aug 30]: https://www.ncbi.nlm.nih.gov/books/NBK470297/
- Mann GS, Sellars MEK, Sidhu PS. The paediatric uterus, ovaries and testes. Clin Ultrasound. 2011 Jan 1;2:1468–21.
- Habiba M, Heyn R, Bianchi P, et al. The development of the human uterus: Morphogenesis to menarche. Hum Reprod Update. 2021;27(1):1–26. doi: 10.1093/humupd/dmaa036
- Gasner A, AP A. Physiology, uterus. StatPearls [Internet]. 2022 May 19 [cited 2022 Aug 30]. Available from: https://www.ncbi.nlm.nih.gov/books/NBK557575/
- Puntambekar S, Nanda SM, Parikh K Ligaments and supports of the uterus. Laparosc Pelvic Anat Females [Internet]. 2019;25–50. cited 2022 Sep 1. Available from: https://link.springer.com/chapter/10.1007/978-981-13-8653-4_3
- Fiander A, Thilaganathan B. Royal college of obstetricians and gynaecologists (Great Britain). Your essential revision guide: MRCOG part one: the official companion to the Royal College of Obstetricians and Gynaecologists revision course. p. 538.
- Pino JH. Arrangement of muscle fibers in the myometrium of the human uterus: A mesoscopic study. MOJ Anat Physiol. 2017;4(2):280–283. doi: 10.15406/mojap.2017.04.00131
- Zara F, Dupuis O. Uterus. Biomech Living Organs Hyperelastic Const Laws Finite Elem Model. 2017 Jan 1;325–346.
- Finn CA, Porter DG. Handbooks in reproductive biology. In The uterus [Internet]. Vol. 1, London: Elek Science; 1975. [cited 2022 Sep 15]. Available from: https://www.ncbi.nlm.nih.gov/nlmcatalog/7509853
- Carsten M. Regulation of myometrial composition, growth and activity. The Maternal [Internet]. 1968 [cited 2022 Sep 15]. Available from: https://books.google.com/books?hl=en&lr=&id=mSzLBAAAQBAJ&oi=fnd&pg=PA355&ots=MMrE3PTpHU&sig=82pRRpuWHMtdTDoQxl8cwvuKO8I
- Kao CY. Electrophysiological properties of uterine smooth muscle. Biol Uterus. 1989;403–454.
- Singer SJ, Nicolson GL. The fluid mosaic model of the structure of cell membranes. Science [Internet]. 1972;175(4023):720–731. [cited 2022 Sep 15]. Available from: https://pubmed.ncbi.nlm.nih.gov/4333397/
- Somlyo AP, Somlyo AV. Ultrastructure of smooth muscle. Smooth Muscle [Internet]. 1975;3–45. [cited 2022 Sep 15]. Available from: https://link.springer.com/chapter/10.1007/978-1-4684-2751-6_1
- Verhoeff A, Garfield R, … JR-AP, 1986 undefined. Myometrial activity related to gap junction area in periparturient and in ovariectomized oestrogen treated sheep. europepmc.org [Internet]. [cited 2022 Sep 15]. Available from: https://europepmc.org/article/med/3705974
- Bergman RA. Uterine smooth muscle fibers in castrate and estrogen-treated rats. J Cell Bio [Internet]. 1968;36(3):639–648. [cited 2022 Sep 15]. Available from: https://pubmed.ncbi.nlm.nih.gov/5645552/
- Broderick R, Broderick KA. Ultrastructure and calcium stores in the myometrium. undefined. 1990;1–33.
- Ross R, Klebanoff SJ. Fine structural changes in uterine smooth muscle and fibroblasts in response to estrogen. J Cell Bio [Internet]. 1967;32(1):155–167. [cited 2022 Sep 15]. Available from: https://pubmed.ncbi.nlm.nih.gov/10976207/
- Garfield RE, Somlyo AP. Structure of smooth muscle. Calcium Contract [Internet]. 1985;1–36. [cited 2022 Sep 15]]. Available from: https://link.springer.com/chapter/10.1007/978-1-4612-5172-9_1
- Peracchia C. Structural correlates of gap junction permeation. Int Rev Cytol. 1980 Jan 1;66(C):81–146.
- Sáez JC, Martinez AD, Branes MC, et al. Regulation of gap junctions by protein phosphorylation. Braz J Med Biol Res [Internet]. 1998;31(5):593–600. [cited 2022 Sep 15]]. Available from: http://www.scielo.br/j/bjmbr/a/ktDJgyvmVKThZP9qM8JYHCt/?lang=en
- Supplement JM-PR, 1962 undefined. Regulation of activity in uterine smooth muscle. pubmed.ncbi.nlm.nih.gov [Internet]. [cited 2022 Sep 15]. Available from: https://pubmed.ncbi.nlm.nih.gov/14470252/
- Hille B. Ionic channels in excitable membranes. Current problems and biophysical approaches. Biophys Elsevier [Internet]. 1978 [cited 2022 Sep 15]. Available from: https://www.sciencedirect.com/science/article/pii/S0006349578854897
- Rubányi G, Balogh I, … AK-AM, 1980 undefined. Ultrastructure and localization of calcium in uterine smooth muscle. europepmc.org [Internet]. [cited 2022 Sep 15]. Available from: https://europepmc.org/article/med/7446226
- Mironneau J. Ion channels and the control of uterine contractility. In: Garfield RE Tabb TN, editors. Control of uterine contractility. 1st ed. London: CRC Press; 2019. pp. 1–22. doi: 10.1201/9780429261756-1
- Tezuka N, Ali M, Chwalisz K, et al. Changes in transcripts encoding calcium channel subunits of rat myometrium during pregnancy. American Journal Of Physiology-Cell Physiology [Internet]. 1995;269(4):C1008–C1017. [cited 2022 Sep 15]. Available from: https://pubmed.ncbi.nlm.nih.gov/7485440/
- Izumi H, Bian K, Bukoski RD, et al. Agonists increase the sensitivity of contractile elements for Ca++ in pregnant rat myometrium. Am J Obstet Gynecol [Internet]. 1996;175(1):199–206. [cited 2022 Sep 15]]. Available from: https://pubmed.ncbi.nlm.nih.gov/8694052/
- Batra S. Effect of estrogen and progesterone treatment on calcium uptake by the myometrium and smooth muscle of the lower urinary tract. Eur J Pharmacol [Internet]. 1986;127(1–2):37–42. [cited 2022 Sep 15]. Available from: https://pubmed.ncbi.nlm.nih.gov/3758176/
- Magocsi M, Penniston JT. Oxytocin pretreatment of pregnant rat uterus inhibits Ca2+ uptake in plasma membrane and sarcoplasmic reticulum. Biochim Biophys Acta - Biomembr. 1991 Mar 18;1063(1):7–14.
- Nelson MT, Patlak JB, Worley JF, et al. Calcium channels, potassium channels, and voltage dependence of arterial smooth muscle tone. American Journal Of Physiology-Cell Physiology [Internet]. 1990;259(1):C3–C18. [cited 2022 Sep 15]. Available from: https://pubmed.ncbi.nlm.nih.gov/2164782/
- Ono M, Bulun SE, Maruyama T. M i n i r e v i e w tissue-specific stem cells in the myometrium and tumor-initiating cells in leiomyoma 1. Biol Reprod [Internet]. 2014;91(6):149–150. [cited 2022 Sep 11]. Available from: http://www.biolreprod.org
- Brakta S, Mas A, Al-Hendy A. The ontogeny of myometrial stem cells in OCT4-GFP transgenic mouse model. Stem Cell Res Ther [Internet]. 2018 Nov 29;9(1):1–8. [cited 2022 Sep 11]. Available from: doi: 10.1186/s13287-018-1079-7
- Maruyama T, Masuda H, Ono M, et al. Human uterine stem/progenitor cells: Their possible role in uterine physiology and pathology. Reproduction. 2010;140(1):11–22. doi: 10.1530/REP-09-0438
- Othman ER, Elgamal DA, Refaiy AM, et al. Identification and potential role of telocytes in human uterine leiomyoma. Contracept Reprod Med [Internet]. 2016 Dec;1(1): [cited 2023 Jun 12]. Available from: https://pubmed.ncbi.nlm.nih.gov/29201401/
- Cretoiu D, Cretoiu SM, Simionescu AA, et al. Telocytes, a distinct type of cell among the stromal cells present in the lamina propria of jejunum. Histol Histopathol [Internet]. 2012 Aug;27(8):1067–1078. [cited 2023 Jun 12]. Available from: https://pubmed.ncbi.nlm.nih.gov/22763879/
- Cretoiu SM, Cretoiu D, Marin A, et al. Telocytes: Ultrastructural, immunohistochemical and electrophysiological characteristics in human myometrium. Reproduction. 2013;145(4):357–370. doi: 10.1530/REP-12-0369
- Aleksandrovych V, Walocha JA, Gil K. Telocytes in female reproductive system (human and animal). J Cell Mol Med [Internet]. 2016 Jun 1;20(6):994–1000. [cited 2023 Jun 12]. Available from: https://pubmed.ncbi.nlm.nih.gov/27060783/
- Metaxa-Mariatou V, McGavigan CJ, Robertson K, et al. Elastin distribution in the myometrial and vascular smooth muscle of the human uterus. Mol Hum Reprod [Internet]. 2002;8(6):559–565. [cited 2023 Jun 12]. Available from: https://pubmed.ncbi.nlm.nih.gov/12029074/
- McCluggage WG, Robboy SJ. Mesenchymal uterine tumors, other then pure smooth muscle neoplasms, and adenomyosis. In: Stanley J Robboy R editors. Robboy’s pathology of the female reproductive tract [Internet]. Elsevier Health Sciences; 2009. pp. 450–453. [cited 2022 Sep 15]. Available from: https://books.google.com/books/about/Robboy_s_Pathology_of_the_Female_Reprodu.html?id=ab545XL-MBEC
- Pinzauti S, Lazzeri L, Tosti C, et al. Transvaginal sonographic features of diffuse adenomyosis in 18-30-year-old nulligravid women without endometriosis: association with symptoms. Ultrasound Obstet Gynecol [Internet]. 2015 Dec 1;46(6):730–736. [cited 2022 Sep 15]. Available from: https://pubmed.ncbi.nlm.nih.gov/25728241/
- Vannuccini S, Tosti C, Carmona F, et al. Pathogenesis of adenomyosis: an update on molecular mechanisms. Reprod Biomed Online [Internet]. 2017 Nov 1;35(5):592–601. [cited 2022 Sep 15]. doi: 10.1016/j.rbmo.2017.06.016
- Peric H, Fraser IS. The symptomatology of adenomyosis. Best Pract Res Clin Obstet Gynaecol [Internet]. 2006 Aug;20(4):547–555. [cited 2022 Sep 15]. Available from: https://pubmed.ncbi.nlm.nih.gov/16515888/
- Andrei Taran F, Wallwiener M, Kabashi D, et al. Clinical characteristics indicating adenomyosis at the time of hysterectomy: a retrospective study in 291 patients. Arch Gynecol Obstet [Internet]. 2012 Jun;285(6):1571–1576. [cited 2022 Sep 15]. Available from: https://pubmed.ncbi.nlm.nih.gov/22193824/
- Van den Bosch T, Van Schoubroeck D. Ultrasound diagnosis of endometriosis and adenomyosis: State of the art. Best Pract Res Clin Obstet Gynaecol [Internet]. 2018 Aug 1;51:16–24. [cited 2022 Sep 15]. Available from: https://pubmed.ncbi.nlm.nih.gov/29506961/
- Leyendecker G, Bilgicyildirim A, Inacker M, et al. Adenomyosis and endometriosis. Re-visiting their association and further insights into the mechanisms of auto-traumatisation. An MRI study. Arch Gynecol Obstet [Internet]. 2015 Apr 1;291(4):917–932. [cited 2022 Sep 15]. Available from: https://pubmed.ncbi.nlm.nih.gov/25241270/
- Campo S, Campo V, Benagiano G. Adenomyosis and infertility. Reprod Biomed Online [Internet]. 2012 Jan;24(1):35–46. [cited 2022 Sep 15]. Available from: https://pubmed.ncbi.nlm.nih.gov/22116070/
- Younes G, Tulandi T. Effects of adenomyosis on in vitro fertilization treatment outcomes: a meta-analysis. Fertil Steril [Internet]. 2017 Sep 1;108(3):483–490.e3. [cited 2022 Sep 15]. Available from: https://pubmed.ncbi.nlm.nih.gov/28865548/
- Harada T, Khine YM, Kaponis A, et al. The impact of adenomyosis on women’s fertility. Obstet Gynecol Surv [Internet]. 2016 Sep 1;71(9):557 [[cited 2022 Sep 15]]. Available from. https://pmc/articles/PMC5049976/
- Mehasseb MK, Bell SC, Pringle JH, et al. Uterine adenomyosis is associated with ultrastructural features of altered contractility in the inner myometrium. Fertil Steril [Internet]. 2010 May 1;93(7):2130–2136. [cited 2022 Sep 15]. Available from: https://pubmed.ncbi.nlm.nih.gov/19268938/
- Mavrelos D, Holland TK, O’Donovan O, et al. The impact of adenomyosis on the outcome of IVF-embryo transfer. Reprod Biomed Online [Internet]. 2017 Nov 1;35(5):549–554 [cited 2022 Sep 15]. Available from: https://pubmed.ncbi.nlm.nih.gov/28802706/
- Moshesh M, Peddada SD, Cooper T, et al. Intraobserver variability in fibroid size measurements: Estimated effects on assessing fibroid growth. J Ultrasound Med. 2014;33(7):1217–1224. doi: 10.7863/ultra.33.7.1217
- Cramer SF, Patel A. The frequency of uterine leiomyomas. Am J Clin Pathol [Internet]. 1990;94(4):435–438. [[cited 2022 Sep 15]]. Available from: https://pubmed.ncbi.nlm.nih.gov/2220671/
- Brosens I, Deprest J, Cin PD, et al. Clinical significance of cytogenetic abnormalities in uterine myomas. Fertil Steril [Internet]. 1998;69(2):232–235. [[cited 2022 Sep 15]]. Available from: https://pubmed.ncbi.nlm.nih.gov/9496334/
- Wallach EE, Vlahos NF. Uterine myomas: an overview of development, clinical features, and management. Obstet Gynecol [Internet]. 2004 Aug;104(2):393–406. [[cited 2022 Sep 15]]. Available from: https://pubmed.ncbi.nlm.nih.gov/15292018/
- Ryan GL, Syrop CH, Van Voorhis BJ. Role, epidemiology, and natural history of benign uterine mass lesions. Clin Obstet Gynecol [Internet]. 2005 Jun;48(2):312–324. [[cited 2022 Sep 15]]. Available from: https://pubmed.ncbi.nlm.nih.gov/15805789/
- HIROSI K. Recent studies on the electrophysiology of the uterus. CFSG 9 (1961): 51-70. Kuriyama: Recent Studies On The electrophysiology… - Google Scholar [Internet]. [cited 2022 Sep 15]. Available from: https://scholar.google.com/scholar_lookup?&title=Recentstudiesoftheelectrophysiologyoftheuterus&pages=51-70&publication_year=1961&author=Kuriyama%2CH
- MacKenzie LW, Garfield RE. Hormonal control of gap junctions in the myometrium. American Journal Of Physiology-Cell Physiology [Internet]. 1985;248(3):C296–C308. [[cited 2022 Sep 15]]. Available from: https://pubmed.ncbi.nlm.nih.gov/3976879/
- Simms-Stewart D, Fletcher H. Counselling patients with uterine fibroids: A review of the management and complications. Obstet Gynecol Int. 2012;2012:1–6. doi: 10.1155/2012/539365
- Tanos V, Berry KE. Benign and malignant pathology of the uterus. Best Pract Res Clin Obstet Gynaecol [Internet]. 2018 Jan 1;46:12–30. [cited 2022 Sep 15]. Available from: https://pubmed.ncbi.nlm.nih.gov/29126743/
- Van Der Voet LF, De Vaate AM B, Veersema S, et al. Long-term complications of caesarean section. The niche in the scar: a prospective cohort study on niche prevalence and its relation to abnormal uterine bleeding. BJOG An Int J Obstet Gynaecol [Internet]. 2014 Jan 1;121(2):236–244 [[cited 2022 Sep 12]]. Available from. https://onlinelibrary.wiley.com/doi/full/10.1111/1471-0528.12542
- Tidball JG, Villalta SA. Regulatory interactions between muscle and the immune system during muscle regeneration. Am J Physiol Regul Integr Comp Physiol [Internet]. 2010 May;298(5):R1173–R1187. [[cited 2023 Jun 12]]. Available from: https://pubmed.ncbi.nlm.nih.gov/20219869/
- Li Y, Foster W, Deasy BM, et al. Transforming growth factor-beta1 induces the differentiation of myogenic cells into fibrotic cells in injured skeletal muscle: a key event in muscle fibrogenesis. Am J Pathol [Internet]. 2004;164(3):1007–1019. [cited 2023 Jun 12]. Available from: https://pubmed.ncbi.nlm.nih.gov/14982854/
- Jahanyar J, Joyce DL, Southard RE, et al. Decorin-mediated transforming growth factor-beta inhibition ameliorates adverse cardiac remodeling. J Heart Lung Transplant [Internet]. 2007 Jan;26(1):34–40. [[cited 2023 Jun 12]]. Available from: https://pubmed.ncbi.nlm.nih.gov/17234515/.
- Tinelli A, Hurst BS, Mettler L, et al. Ultrasound evaluation of uterine healing after laparoscopic intracapsular myomectomy: An observational study. Hum Reprod. 2012;27(9):2664–2670. doi: 10.1093/humrep/des212
- Vikhareva Osser O, Valentin L. Risk factors for incomplete healing of the uterine incision after caesarean section. BJOG An Int J Obstet Gynaecol [Internet]. 117(9):1119–1126. [2010 Aug 1[cited 2022 Sep 12]]. Available from: https://onlinelibrary.wiley.com/doi/full/10.1111/j.1471-0528.2010.02631.x
- Buhimschi CS, Zhao G, Sora N, et al. Myometrial wound healing post-cesarean delivery in the MRL/MPJ mouse model of uterine scarring. Am J Pathol. 2010;177(1):197–207. doi: 10.2353/ajpath.2010.091209
- Roeder HA, Cramer SF, Leppert PC. A look at uterine wound healing through a histopathological study of uterine scars. Reprod Sci [Internet]. 2012 May;19(5):463–473. [cited 2022 Sep 15]. Available from: https://pubmed.ncbi.nlm.nih.gov/22344737/
- Ida A, Kubota Y, Nosaka M, et al. Successful management of a cesarean scar defect with dehiscence of the uterine incision by using wound lavage. Case Rep Obstet Gynecol [Internet]. 2014;2014:1–4. [[cited 2022 Sep 15]]. Available from: https://pubmed.ncbi.nlm.nih.gov/25431714/
- Haraguchi Y, Shimizu T, Yamato M, et al. Concise review: Cell therapy and tissue engineering for Cardiovascular disease. Stem Cells Transl Med [Internet]. 2012 Feb 1;1(2):136. [cited 2023 May 25]]. Available from: https://pmc/articles/PMC3659688/
- Hellström M, Bandstein S, Brännström M. Uterine tissue engineering and the future of uterus transplantation. Ann Biomed Eng [Internet]. 2017 Jul 1;45(7):1718 [[cited 2023 Jun 8]]. Available from. https://pmc/articles/PMC5489617/
- Gharibeh N, Aghebati-Maleki L, Madani J, et al. Cell-based therapy in thin endometrium and Asherman syndrome. Stem Cell Res Ther [Internet]. 2022 Dec 1;13(1):1–13. [cited 2023 May 25]. Available from: doi: 10.1186/s13287-021-02698-8
- Song YT, Liu PC, Tan J, et al. Stem cell-based therapy for ameliorating intrauterine adhesion and endometrium injury. Stem Cell Res Ther [Internet]. 2021 Oct 30;12(1):1–14. [cited 2023 May 25]. Available from: https://stemcellres.biomedcentral.com/articles/10.1186/s13287-021-02620-2
- Liu F, Hu S, Wang S, et al. Cell and biomaterial-based approaches to uterus regeneration. Regen Biomater [Internet]. 2019 Jun 1;6(3):141. [cited 2023 May 25]. Available from: https://pmc/articles/PMC6547309/
- Yang J, Chen Z, Li S, et al. Role of TGF-β3 and bone marrow mesenchymal stem cells on regeneration of myometrial injury in rats. J Radiat Res Appl Sci 2022 Dec 1;15(4):100476. doi: 10.1016/j.jrras.2022.100476
- Zhao J, Zhang Q, Wang Y, et al. Uterine infusion with bone marrow mesenchymal stem cells Improves endometrium thickness in a rat model of thin endometrium. Reprod Sci [Internet]. 2015 Feb 13;22(2):181 [cited 2023 May 25]. Available from. https://pmc/articles/PMC4287599/
- Magalhaes RS, Williams JK, Yoo KW, et al. A tissue-engineered uterus supports live births in rabbits. Nat Biotechnol [Internet]. 2020 Nov 1;38(11):1280–1287 [[cited 2022 Sep 14]]. Available from: https://pubmed.ncbi.nlm.nih.gov/32601434/
- Hanuman S, Nune M. Design and characterization of maltose-conjugated Polycaprolactone nanofibrous scaffolds for uterine tissue engineering. Regen Eng Transl Med. 2022 Jun 1;8(2):334–344. doi: 10.1007/s40883-021-00231-0
- Yao Q, Zheng YW, Lin HL, et al. Exploiting crosslinked decellularized matrix to achieve uterus regeneration and construction. Artificial Cells, Nanomed, Biotechnol [Internet]. 2020 Jan 1;48(1):218–229 [cited 2022 Sep 14]. Available from: https://www.tandfonline.com/doi/abs/10.1080/21691401.2019.1699828
- Nikolova MP, Chavali MS. Recent advances in biomaterials for 3D scaffolds: A review. Bioact Mater. 2019;4(October):271–292. doi: 10.1016/j.bioactmat.2019.10.005
- Lee JM, Yeong WY. Design and printing strategies in 3D bioprinting of cell-hydrogels: A review. Adv Healthc Mater [Internet]. 2016 Nov 23;5(22):2856–2865. [[cited 2022 Sep 15]]. Available from: https://pubmed.ncbi.nlm.nih.gov/27767258/
- Arezoo N, Mohammad H, Malihezaman M. Tissue engineering of mouse uterus using menstrual blood stem cells (MenScs) and decellularized uterine scaffold. Stem Cell Res Ther [Internet]. 2021;12(1):1–12. doi: 10.1186/s13287-021-02543-y
- Zhang X, Chen X, Hong H, et al. Decellularized extracellular matrix scaffolds: Recent trends and emerging strategies in tissue engineering. Bioact Mater. 2022 Apr 1;10:15–31. doi: 10.1016/j.bioactmat.2021.09.014
- Young RC, Goloman G. Allo- and xeno-reassembly of human and rat myometrium from cells and scaffolds. Tissue Eng - Part A. 2013;19(19–20):2112–2119. doi: 10.1089/ten.tea.2012.0549
- Hellström M, El-Akouri RR, Sihlbom C, et al. Towards the development of a bioengineered uterus: Comparison of different protocols for rat uterus decellularization. Acta Biomater. 2014 Dec 1;10(12):5034–5042. doi: 10.1016/j.actbio.2014.08.018
- Santoso EG, Yoshida K, Hirota Y, et al. Application of detergents or high hydrostatic pressure as decellularization processes in uterine tissues and their subsequent effects on in vivo uterine regeneration in murine models. PLoS One. 2014;9(7):e103201. doi: 10.1371/journal.pone.0103201
- Hellström M, Moreno-Moya JM, Bandstein S, et al. Bioengineered uterine tissue supports pregnancy in a rat model. Fertil Steril. 2016;106(2):487–496.e1. doi: 10.1016/j.fertnstert.2016.03.048
- Tiemann TT, Padma AM, Sehic E, et al. Towards uterus tissue engineering: a comparative study of sheep uterus decellularisation. Mol Hum Reprod [Internet]. 2020 Mar 26;26(3):167–178. [cited 2020 Apr 13]. Available from. http://www.ncbi.nlm.nih.gov/pubmed/31980817
- Park DW, Choi DS, Ryu HS, et al. A well-defined in vitro three-dimensional culture of human endometrium and its applicability to endometrial cancer invasion. Cancer Lett [Internet]. 2003 Jun 10;195(2):185–192. [cited 2023 Jun 12]. Available from: https://pubmed.ncbi.nlm.nih.gov/12767527/
- Lalitkumar PGL, Lalitkumar S, Meng CX, et al. Mifepristone, but not levonorgestrel, inhibits human blastocyst attachment to an in vitro endometrial three-dimensional cell culture model. Hum Reprod [Internet]. 2007 Nov 1;22(11):3031–3037. [cited 2023 Jun 12]. Available from: https://dx.doi.org/10.1093/humrep/dem297
- Ghosh D, Sengupta J. Physiology: Morphological characteristics of human endometrial epithelial cells cultured on rat-tail collagen matrix. Hum Reprod [Internet]. 1995;10(4):785–790. [cited 2023 Jun 12]. Available from: https://pubmed.ncbi.nlm.nih.gov/7650121/
- Lü SH, Bin WH, Liu H, et al. Reconstruction of engineered uterine tissues containing smooth muscle layer in collagen/matrigel scaffold in vitro. Tissue Eng - Part A [Internet]. 2009 Jul 1;15(7):1611–1618 [cited 2020 Apr 13]. Available from: https://www.liebertpub.com/doi/10.1089/ten.tea.2008.0187
- Meng CX, Andersson KL, Bentin-Ley U, et al. Effect of levonorgestrel and mifepristone on endometrial receptivity markers in a three-dimensional human endometrial cell culture model. Fertil Steril [Internet]. 2009 Jan;91(1):256–264. [cited 2023 Jun 12]. Available from: https://pubmed.ncbi.nlm.nih.gov/18206148/
- Bin WH, SH L, Lin QX, et al. Reconstruction of endometrium in vitro via rabbit uterine endometrial cells expanded by sex steroid. Fertil Steril. 2010 May 1;93(7):2385–2395. doi: 10.1016/j.fertnstert.2009.01.091
- Lin N, Li X, Song T, et al. The effect of collagen-binding vascular endothelial growth factor on the remodeling of scarred rat uterus following full-thickness injury. Biomaterials. 2012 Feb 1;33(6):1801–1807. doi: 10.1016/j.biomaterials.2011.11.038
- Pence JC, Clancy KBH, Harley BAC. The induction of pro-angiogenic processes within a collagen scaffold via exogenous estradiol and endometrial epithelial cells. Biotechnol Bioeng [Internet]. 2015 Oct 1;112(10):2185–2194. [[cited 2023 Jun 12]]. Available from: https://pubmed.ncbi.nlm.nih.gov/25944769/
- Schutte SC, James CO, Sidell N, et al. Tissue-engineered endometrial model for the study of cell-cell interactions. Reprod Sci [Internet]. 2015 Mar 16;22(3):308–315 [cited 2023 Jun 12]. Available from: https://pubmed.ncbi.nlm.nih.gov/25031317/
- Song T, Zhao X, Sun H, et al. Regeneration of uterine horns in rats using collagen scaffolds loaded with human embryonic stem cell-derived endometrium-like cells. Tissue Eng - Part A. 2015;21(1–2):353–361. doi: 10.1089/ten.tea.2014.0052
- Xu L, Ding L, Wang L, et al. Umbilical cord-derived mesenchymal stem cells on scaffolds facilitate collagen degradation via upregulation of MMP-9 in rat uterine scars. Stem Cell Res Ther. 2017;8(1):1–13. doi: 10.1186/s13287-017-0535-0
- Yoshimasa Y, Maruyama T. Bioengineering of the uterus. Reprod Sci [Internet]. 2021 Jun 1;28(6):1596–1611 [cited 2022 Sep 14]. Available from. https://link.springer.com/article/10.1007/s43032-021-00503-8
- Lu S, Ph D, Liu H, et al. Reconstruction of engineered uterine tissues containing smooth muscle layer in collagen/Matrigel scaffold in vitro. Tissue Eng Part A. 2009;15(7):1611–1618. doi: 10.1089/ten.tea.2008.0187
- Ding L, Li X, Sun H, et al. Transplantation of bone marrow mesenchymal stem cells on collagen scaffolds for the functional regeneration of injured rat uterus. Biomaterials 2014 Jun 1;35(18):4888–4900. doi: 10.1016/j.biomaterials.2014.02.046
- Ji W, Hou B, Lin W, et al. 3D bioprinting a human iPSC-derived MSC-loaded scaffold for repair of the uterine endometrium. Acta Biomater. 2020 Oct 15;116:268–284. doi: 10.1016/j.actbio.2020.09.012
- Heidari Kani M, Chan EC, Young RC, et al. 3D cell culturing and possibilities for myometrial tissue engineering. Ann Biomed Eng [Internet]. 2017 Jul 1;45(7):1746–1757 [cited 2023 Aug 4]. Available from: https://pubmed.ncbi.nlm.nih.gov/27770218/
- Xiao B, Yang W, Lei D, et al. PGS scaffolds promote the in vivo survival and directional differentiation of bone marrow mesenchymal stem cells restoring the morphology and function of wounded rat uterus. Adv Healthcare Mater [Internet]. 2019 Mar 1;8(5):1801455 [[cited 2022 Sep 14]]. Available from: https://onlinelibrary.wiley.com/doi/full/10.1002/adhm.201801455
- Hanuman S, Pande G, Nune M. Aminolysis of Polycaprolactone nanofibers for applications in uterine tissue engineering. Trends Biomater Artif Organs [Internet]. 2021 Oct 1;35(4):347–354. [[cited 2023 Aug 4]]. Available from: https://go.gale.com/ps/i.do?p=HRCA&sw=w&issn=09711198&v=2.1&it=r&id=GALE%7CA680295058&sid=googleScholar&linkaccess=fulltext
- Li X, Sun H, Lin N, et al. Regeneration of uterine horns in rats by collagen scaffolds loaded with collagen-binding human basic fibroblast growth factor. Biomaterials [Internet]. 2011;32(32):8172–8181. http://dx.doi.org/10.1016/j.biomaterials.2011.07.050
- Uribe-Gomez J, Posada-Murcia A, Shukla A, et al. Soft elastic fibrous scaffolds for muscle tissue engineering by touch spinning. ACS Appl Bio Mater [Internet]. 2021 Jul 19;4(7):5585–5597. [cited 2023 Aug 2]. Available from: doi: 10.1021/acsabm.1c00403
- Loh QL, Choong C. Three-dimensional scaffolds for tissue engineering applications: role of porosity and pore size. Tissue Eng Part B Rev [Internet]. 2013 Dec 1;19(6):485–502. [cited 2022 Sep 15]. Available from: https://pubmed.ncbi.nlm.nih.gov/23672709/