ABSTRACT
Algae-based biofuel developed over the past decade has become a viable substitute for petroleum-based energy sources. Due to their high lipid accumulation rates and low carbon dioxide emissions, microalgal species are considered highly valuable feedstock for biofuel generation. This review article presented the importance of biofuel and the flaws that need to be overcome to ensure algae-based biofuels are effective for future-ready bioenergy sources. Besides, several issues related to the optimization and engineering strategies to be implemented for microalgae-based biofuel derivatives and their production were evaluated. In addition, the fundamental studies on the microalgae technology, experimental cultivation, and engineering processes involved in the development are all measures that are commendably used in the pre-treatment processes. The review article also provides a comprehensive overview of the latest findings about various algae species cultivation and biomass production. It concludes with the most recent data on environmental consequences, their relevance to global efforts to create microalgae-based biomass as effective biofuels, and the most significant threats and future possibilities.
GRAPHICAL ABSTRACT
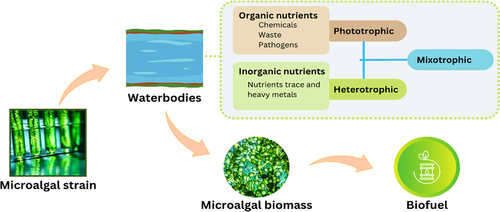
1. Introduction
Biomass materials are organic substances converted into biofuel from different sources, such as plant matter, algae, or animal by-products and their processed forms. Unlike nonrenewable energy sources like petroleum, natural gas, and coal are the commercial feedstock material used to produce synthetic fuels [Citation1]. The rising petroleum prices and concerns about fossil fuels role contribution to global warming have triggered alternative resolutions in the search for biofuel due to their environmentally friendly benefits toward the ecological aspects [Citation2]. Based on both financial cost and environmental perspective, the process involving petroleum-based products provides expansion of wider opportunities, such as the production of high-dense fuel, over alternative biofuels. Which requires large tracts of arable land for the production of food crops and other biomass.
Industrialization and human population expansion increased the global demand for energy on a global scale by around 4 to 5% [Citation3]. Biofuels are renewable resources that produce fewer harmful emission when burned compared to fossil fuels and do not emit carbon dioxide (CO2) into the atmosphere, moreover the released carbon are absorbed by plant during their growth. Somehow most of the released compounds, such as carbon, hydrogen, and oxygen atoms, are absorbed by the organisms producing the biomass. The processes that produce items based on microalgae are said to result in the emission of considerable volumes of carbon dioxide, as determined by evaluations of ecological footprints. The plan for the zero-carbon effort that was made public includes reductions based on the construction of a climate action project that has three trends that can earn carbon credits. These trends are carbon capture, mitigation of wastewater treatment, and renewable or clean electricity. To satisfy the requirements for energy and triumph over these obstacles, eco-friendly approaches are necessary. Much interest has focused on researching and developing sustainable, clean, and renewable energy sources, including biofuels [Citation4]. One such example is the continued consumption of fossil fuels, causing increasingly severe problems despite their widespread use. Anthropogenic activities have resulted in an excessive buildup of a wide variety of hazardous elements and pollutants, which are believed to be destructive to life on Earth and the many ecosystems found worldwide. It appears that in the not-too-distant future, the application of microalgal biotechnology will be vital to the process of lowering the number of dangerous compounds in the environment. Microalgae have a smaller footprint than first-generation energy crops, where microalgae biomass can produce the similar amount of oil and biomass due to their faster growth rate, higher lipid content, and higher biomass yield. Microalgae can thrive in harsh environments, metabolize various nutrients, and survive on water that would normally be unusable by humans. It is anticipated that microalgae will play an essential role in this mitigation, and it will be explored how the production of renewable energy from microalgae can help to the reduction of a variety of environmental challenges in an integrated manner. The use of biodiesel as a biofuel has recently gained significant interest. There has been discussion about using microalgae as the feedstock for the third and fourth generations of biodiesel production. Several optimistic predictions have made that microalgae have exponential potential as a source of bioenergy [Citation5]. Microalgae not only serve as a natural carbon sink that contributes to the reduction of global warming but also create a wide range of commercially significant and valuable products. Due to the fact that microalgae can flourish in nutrient-rich wastewater, microalgae can be utilized to treat effluent in a manner that is both non-harmful to the environment and sustainable. Since it does not compete with food crops for arable land, it can grow in various environments, including seawater, and it removes CO2 and phosphorous while growing, making it useful for treating wastewater. Therefore, algal oil production does not harm traditional agriculture [Citation6]. Commercializing microalgae-based biofuels involves choosing the optimum species for outdoor growing, optimizing photosynthetic efficiency, and lowering production costs. Increased research on biomass residues, gas, liquid fractions, and extraction of lipids extraction would also enhance the productivity of biofuel [Citation7]. Heated power plant generators with waste heat and used to create electricity. Some biomass-burning power plants have been running for some time [Citation8]. Similarly, wastewater contamination can be reduced by the process of bioremediation, which makes use of microalgae [Citation9]. Numerous types of research have provided evidence for this strategic approach. Making biofuels from microalgae while cleaning wastewater has significant economic and environmental benefits [Citation10]. Microalgae with a short life cycle, a rapid growth rate, and a high CO2 utilization efficiency could be one strategy to produce biomass from waste water nutrients while also using renewable resources. Microalgae are not only more productive, but they may also thrive in murky water on unarable ground. Therefore, much effort has been put toward providing sustainable microalgae-based biofuels [Citation11]. Bioenergy can also be generated by various methods such as thermochemical processes, acid/base transesterification, supercritical solvents, and microorganism-derived fuel cells. Although the techniques mentioned above are simple to execute, they are not yet widely employed in business due to their high price tag and the requirement for technological advancements [Citation12]. This necessitates using the most efficient downstream process available to maintain competitively low production costs. A novel integration approach is currently being applied into the circular bioeconomy concept of zero-carbon or low-carbon emission [Citation13]. This strategy places primary emphasis on the utilization of reducing, recycling, and reusing solutions, which ultimately results in a small carbon footprint. This initiative seeks to reduce humans reliance on fossil fuels by shifting toward alternative sources of energy and value-based products as part of its implementation.
This review paper covers the basics and preliminary extraction methods from both traditional and cutting-edge modern techniques on microalgal fuel production that uses extraction and pre-treatment process. A few alternative methods were also investigated elaborate the basic engineering concepts involved and utilized for the biofuel production. Recent breakthroughs in microalgal biofuel generation from a different source will be critically addressed, along with several microalgae growing and cultivation methods and the numerous species of microalgae that are utilized for harvesting biomass. Further procedures use acid and base-mediated transesterification to convert biomass into fuel from algae. This study also examined ethanol, biobutanol, and biodiesel, as well as prospective energy sources of the future, such as hydrogen and microbial fuel cells, which are investigated
1.1. Significance of different biofuels from biological sources
Liquid biofuels have garnered much attention recently due to the extensive infrastructure to use them, particularly in the transportation sector [Citation14]. Bioethanol, which can be produced by fermenting either starch or sugar into ethyl alcohol, has become the most widely produced liquid biofuel. Second-generation biofuel, or cellulosic ethanol, is made from low-value biomass composed of cellulose instead of edible food crops [Citation15]. Some examples of cellulose-based biomass include lignocellulosic crop residues, wood chips, and municipal waste. The production of cellulosic ethanol typically involves using a wide variety of grasses that can be grown on low-quality land such as sugarcane bagasse, a by-product of the sugar processing industry [Citation16]. Since cellulose biomass does not convert as quickly as biofuels from the first generation, cellulosic ethanol is typically utilized as a gasoline component rather than a standalone fuel [Citation17]. shows the different generations of biofuel production mechanisms, their respective by-product conversation in each stage, and elaborates on the sequential process between the recent advancement in biofuel production from biological sources.
2. Importance of algae as a biofuel
Third-generation biomass uses microalgae biomass as raw material to create these biofuels are compatible with a wide variety of fuels, the most common of which are gasoline, diesel, and aviation gasoline. Microalgae biomass is a renewable feedstock that can be used as a sustainable energy source [Citation18]. Microalgae biomass is more preferable for the production of biodiesel as their biomass composition has higher lipid content than other biomass [Citation19]. Studies indicate that microalgae have the potential to produce more energy per hectare of land than traditional crops do [Citation20,Citation21]. Microalgae cultivation throughout the year usually takes 7 to 14 days, depending on the cultivation condition. Once it reaches a stationary growth, these microalgae biomass may have an oil content of 20–50% biomass dry weight, generating more oil per acre than any other crop [Citation22]. Microalgae cultivation requires a steady supply of several inorganic nutrients to maintain high algae yields. Many factors, including light intensity, hydrogen ion concentration, available nutrient concentrations, salinity, etc influence their growth parameter.
Additionally, various pesticides, herbicides, and nitrogen and phosphorus concentrations on cultivable land are highly influential factors for cultivation. Transesterification is the most commonly used process to convert crude oils into methyl ester with the presence of a catalyst, a sequence of chemical reactions involves alcohol and vegetable oils as the reactants of choice. As a consequence of the procedures, triglycerides are converted into monoglycerides [Citation23].
2.1. Economic importance of biofuel
The determinantal effects on biofuels’ economic benefits are essential to fulfil the required energy demand. For instance, the manufacturing of ethanol from corn requires the use of fossil fuels for the deposition of fertilizer, the transportation of grain, and the process of distillation to form ethanol. This is because fossil fuels are the most efficient way to accomplish these tasks. Ethanol from maize has a lower energy gain process than other feedstock materials (e.g. sugarcane, cellulosic ethanol, and algal biodiesel), all of which have the potential to have an even more significant source of energy generation [Citation19]. Depending on the methods utilized in their production, biofuels may come with several substantial environmental drawbacks in addition to their positive effects on the environment [Citation24]. Biofuels derived from plants are a form of renewable energy that, in principle, have a negligible impact on climate change and global warming. This is because before the biofuel is burned, the photosynthesis process, which takes place when plants are growing, assimilates carbon dioxide from the atmosphere, a substantial greenhouse gas [Citation24]. Since there are numerous types of biofuels that are economically viable and significant for the commercial aspects, and they have been derived from a variety of sources, such as ethanol, which is thought to be a relatively inexpensive fuel with an average price range around 1.80 USD per gallon as of April 2023, and biodiesel, which is used in diesel engines with little to no modifications and emits fewer greenhouse gases than conventional diesel that is, there are many different types of biofuels that are significant for the commercial aspects. The production of biogas can be economically viable in addition to reducing the amount of methane that is released by organic waste. The price of biogas can fluctuate widely because to the many factors involved in its production, including the type of feedstock used, the manufacturing process, and the transportation costs. Another important kind of biofuel is made from cellulosic material.They do not pose a threat to the food supply, which makes them a more environmentally friendly alternative to the manufacture of ethanol and biodiesel than the former. The usage of biofuels is susceptible to being influenced by a number of factors, some of which include government laws, the demand of consumers, and the price of oil on the worldwide market. In addition, the cost of generating various types of biofuels, as well as the location and availability of feedstocks, can all have a substantial impact on the price of biofuels.
2.2. Environmental concern of biofuel
Common feedstocks like corn and soybeans generated the concept of food versus fuel because of their prevalence in producing the first generation of biofuels. Since it diverts arable land and feedstock away from the human food chain, the development of biofuels can potentially affect the economics of food pricing and availability [Citation25]. Energy crops grown to produce ethanol can also compete with the earth’s natural ecosystems [Citation26]. For instance, the emphasis on biodiesel destroys ancient tropical forests to make room for oil palm plantations. Additionally, the emphasis placed on corn-based ethanol leads to the conversion of grasslands and brushlands into monocultures of maize [Citation27].
The hydrology, erosion rate and overall biodiversity of wildlife habitats can all be negatively impacted when natural habitat is destroyed. When the vegetation in the cleared area is set on fire or decays, it has the potential to produce a large amount of carbon dioxide simultaneously [Citation28]. These high-diversity biofuel sources could be converted from degraded agricultural land that is no longer used for farming. This can enhance wildlife habitats, lessen erosion, purify waterways, sequester atmospheric carbon dioxide as carbon compounds in the soil, and revive arid or barren places. In the future, these biofuels may be refined into liquid fuels or burned to produce usable heat and electricity [Citation29].
2.3. Methods of microalgal cultivation
Microalgae are an excellent option for use as a raw material in the industry because of their high photosynthetic efficiency and ability to store a large quantity of the by-products that give birth to the development within their cells. This makes them an excellent choice for use as a raw material [Citation22]. Furthermore, compared to the cultivation of other crops, the production of microalgae requires far less fertile soil, clean water, herbicides, or pesticides; hence, it will not compete with other crops for resources [Citation30,Citation31]. It has been reported that it is possible to cultivate microalgae using wastewater sources (like those from the palm oil industry or homes with sewage systems) because different microalgal species come from other subspecies families with similar properties and characteristics beneficial for commercial applications [Citation32].
This growing method primarily relies on naturally occurring water bodies to give the necessary conditions and nutrients (e.g. nitrogen and phosphate-based chemicals) for the microalgae to thrive appropriately and grow to their full potential. Open pond farming is one of the conventional methods to obtain bulk microalgae culture [Citation33]. Open ponds are frequently utilized in the industry as the overall cultivation process reduces expenses associated with their construction, maintenance, and day-to-day operation. The open pond system has numerous of benefits, including the ease with which it can be set up and maintained, the minimal energy requirements, and the straightforward nature of its extension [Citation34]. The circular pond was the first man-made water source used to cultivate microalgae. The growing method known as ‘circle farming’ gets its name from the circular configuration of the culture tank, which is between 30 and 70 centimeters in depth and 45 meters in width and features a rotating agitator in the pond’s exact center [Citation35]. The raceway pond is also becoming one of the most well-liked open pond designs for microalgae cultivation. It comprises several closed-loop channels that are 30 centimeters deep and paddlewheels that allow microalgal biomass to be circulated. This is done to ensure that nutrients are distributed evenly and to prevent sedimentation from occurring [Citation36]. As a result of the efficient use of energy, raceway ponds are often recognized as among the most effective designs for open pond horticulture that are now available. To appropriately agitate a raceway pond that is 5 hectares in size, all required is a single paddlewheel [Citation34].
2.3.1. Waste from industries
The production of biofuels could utilize the waste products obtained from various industries, most notably the food industry. Biofuel production by selecting a relatively inexpensive feedstock is one of the most critical steps [Citation37]. Throughout the production cycle, the food processing industry and the manufacturing sector generate various food waste and losses [Citation38]. It is likely that inefficient ways of packaging, storing, and transporting goods would lead to the production of trash. Last but not least, the retail system and market environments also contribute to food and food waste production [Citation39]. The conversion of food debris from food processing of such materials into biofuels remains an exciting approach that will be successful due to their price and the cost involved in the removal and treatment processes. In addition, the composition that includes hemicelluloses, cellulose, lignin, organic acids, lipids, proteins, and starch present in waste materials drawn from the food processing industry can be used as carbon and food sources as biofuels [Citation40].
2.3.2. Agricultural waste
Every day, more people are living in the world, which inevitably increases the volume and variety of waste products [Citation41,Citation42]. Several companies are spending money and effort on getting rid of wastes; however, this is not an efficient use of either resource. Rather than that, it would be preferable to generate bioenergy from agricultural wastes; Lignocellulosic biomass consists of four different types of plant matter: grass, grass clippings, hardwood, and softwood. In addition to municipal garbage, this classification also contains agricultural trash and wastewater from cultivable land and runoff waters, possibly creating an avenue for microalgal cultivation. These biomasses are typically thrown out without being treated, which can contaminate the ecosystem by altering the decaying microbial population and creating more stress on the already stressed environment. There are two main types of agricultural waste: those generated in the fields and those generated during processing. After harvesting, there are field trash such as stems, leaves, and stalks, and processing wastes such as seeds, peels, and husks. Biofuels can be produced from the lignocellulosic biomass contained in food waste. The components of this biomass are hemicellulose, cellulose, and lignin [Citation22]. Consumable features of crops include sugarcane, fruits, sugar beets, and corn. Beets are also a type of crop. In addition to corn cobs and stover, rice husk, orchard trimmings, and stalks, agricultural biomass consists of leaves, rice straw, and rice can also be considered to be the source of bioenergy [Citation43].
2.3.3. Microbial source
Due to the fact that biofuel may be produced by microorganisms directly from biomass and does not require any further chemical alterations, researchers have been investigating on various strain of bacteria that can produce biofuel. When producing biofuel, microbes rely heavily on synthetic biology technology (i.e. cell factories) [Citation44]. Ethanol can be made by Clostridium ljungdahlii sp., as this fact has been established. The rod-shaped bacteria could move, formed spores, produced acetic acid, and had motility [Citation45]. Cyanobacteria have many desirable characteristics, including high photosynthetic efficiency, quick growth, genetic tractability, and easy access to their genomes, which is a necessary step in generating biofuel [Citation46]. Current biofuel production includes various products, such as biogas, bioethanol, biohydrogen, biodiesel, and bioelectricity. Cyanobacteria carry out the process by storing the light energy that is acquired during the process of photosynthesis in fuel cells [Citation47]. Anabaena variabilis is a filamentous cyanobacterium that generates electrons for fuel cells in two ways: i) by making its own glycogen and ii) by oxidizing water through photosynthesis in the presence of light [Citation48].
2.3.4. Other feedstock sources for biofuel production
Three primary needs must be met for a human to survive: food, clothing and a place to live. Similar to secondary and tertiary consumers that require necessities such as energy, the environment, and medical treatment [Citation49]. Since fossil fuels is although a significant source of energy are running short, researchers are focusing more attention on alternate renewable energy forms. Studies have looked into the viability of using biomass as a fuel source for alternative power generation [Citation50]. Biomass resources can originate from various sources, such as aquatic biomass, animal wastes, agro-industrial residues, woody biomass, and the inedible portions of plants and animals, in non-addition to rubbish from commercial and residential establishments [Citation51]. Therefore, this section will focus on various commercial materials that could be used to make biofuels. has listed a schematic representation of multiple strategies and technologies for the biofuel production.
3. Microalgal biomass production strategies
The importance of cell density and biomass cannot be overstated when discussing microalgae’s commercial cultivation and utilization. This requires immense optimization in microalgae cultivation to generate a significant amount of biomass. This would be one of the solutions to overcome the problem of lowering the costs associated with using microalgae biomass [Citation50]. In addition to photosynthesis, which is undoubtedly one of the most critical aspects for the growth of algae, heterotrophy and mixotrophy cultivation in varying degrees and forms are both possible for algae to engage in [Citation52]. Due to the need for large-scale production in the microalgal energy business, the high-density culture mode, especially the mixotrophic culture mode, has been getting more attention in recent years. Autotrophic and heterotrophic cultures are better in some ways than mixotrophic cultures; a clear illustration of how the different types of culturing conditions favor biofuel production is provided in . It grows the fastest and builds up the most biomass. It also makes a lot of products with high added value that are triggered by dark conditions [Citation53]. Mixotrophic culture can also be used with measures to cut down on flue gas emissions to save money. The production of microalgal biodiesel can be accomplished through the employment of the following three primary cultivation methods: photoautotrophic cultivation, heterotrophic cultivation, and mixotrophic cultivation [Citation54].
3.1. Autotrophic culture
This strategy works best when implemented in the outdoor environment as there is abundant unfettered access to sunlight and inorganic CO2 substances. Microalgal cells that are cultivated in an environment that is photoautotrophic uses light as their source of energy and carbon dioxide as their supply of carbon; there is no easier way to grow microalgae than this technique [Citation55]. Growing autotrophic microalgae requires a significant investment in costly energy sources such as carbon and phosphorus due to the volume of material needed. Research into identifying low-cost sources of carbon and nitrogen for the development of microalgae, such as carbon and nitrogen pollution, has been an expanding field in recent years [Citation56]. It is possible to increase the amount of light in an area, either naturally or artificially, to a sufficient level to support rapid development and dense population growth. However, the photoautotrophic method has flaws, particularly in regions where the sun does not shine brightly enough throughout the year to allow photoautotrophic agriculture to be practiced continuously. Carbon is the primary food that is required by microalgal cells, and photoautotrophic microalgae can absorb CO2 from exhaust fumes, which reduces the cost of production [Citation57]. Microalgae are a unique group of an organism whose different parts allow them to use a wide range of metabolic processes. Most microalgae are grown through autotrophic growth, which uses carbon dioxide (CO2) as the carbon supply and sunlight as the energy source [Citation58]. The maximal photosynthetic efficiency, controlled by the total incident solar energy, significantly influences biomass productivity. However, this growth mode has a minimal effect on that productivity. Biomass productivity is extremely limited by maximal photosynthetic efficiency [Citation59].
3.2. Heterotrophic culture
Compared to the autotrophic culture of microalgae, heterotrophic culture utilizes a dark light condition, organic carbon sources such as glucose, other organic substances, and external carbon supply, potentially has a large amount of biomass of accumulation of lipids [Citation60]. The biomass and lipid productivities of the microalgae grown in heterotrophy under optimal culture conditions are usually much higher than those of other algae [Citation60,Citation61]. Heterotrophic cultures exhibit a more significant accumulation of biomass and lipids and faster rates of cell development compared to autotrophic cultures. In photoheterotrophic cultures, organic compounds cannot be used as a source of carbon without light. Still, in heterotrophic cultures, organic molecules like glucose, acetate, and glycerol can be used as a source of energy and carbon even when there is no light. In order to prevent bacterial contamination, it is essential to select heterotrophic algae species that have a high level of adaptability to their surrounding environment [Citation62]. When it comes to lipid buildup, nitrogen concentration has a considerable impact on all types of cultivation, including autotrophic and heterotrophic methods. It has been found that the nitrogen content of the medium affects the number of lipids present in microalgal cells. The high productivity of heterotrophic cultures has led to a significant rise in interest in the search for microalgal strains capable of producing high lipid content under circumstances that are optimal for heterotrophic development.
3.3. Mixotrophic culture
Microalgae can drive photoautotrophic and heterotrophic; when grown in a mixotrophic environment, they can get carbon from both inorganic and organic sources, utilizing sunlight/dark light. Aerobic respiration is controlled by how much organic carbon is in the air [Citation63]. On the other hand, during photosynthesis, inorganic carbon is fixed, which is affected by the amount of light present [Citation64]. The primary difference between photoheterotrophy, a unique method of plant cultivation that makes use of The main difference between photoheterotrophy and mixotrophy is that photoheterotrophy gets most of its energy from light. In contrast, mixotrophy has the option of making use of organic substances instead [Citation65]. As a result of the fact that organic molecules can be utilized in mixotrophic farming, the growth of microalgae is not entirely reliant on photosynthesis. As a result, the availability of light is not necessarily a limiting factor for the development of microalgae. When the illumination levels are too low or too high, there is a possibility that photo limitation or photo inhibition will be reduced in mixotrophic cultures [Citation66]. The inorganic waste components can also function as economical sources of nutrients for mixotrophic microalgae. In order to boost the amount of biomass produced by mixotrophic cultures of Chlorella pyrenoidosa, significant amounts of ammonium and phosphate, which can be found in sewage sludge that has been aerobically digested were added. This method allowed the microalgae to grow and make more lipids by using the total nitrogen, phosphorus, and other nutrients in the mixed waste culture solution; there was no requirement for additional sources of nutrients [Citation67].
4. Microalgal biomass to bioenergy conversion
The conversion made through thermochemical and biochemical processes are two of the most crucial processes in the transformation of algal biomass into viable biofuels. The biochemical conversion, in which bacteria are used to create biofuels from biomass, is in contrast to the thermochemical conversion, which uses heat to break down the organic components of the biomass [Citation68]. Among several distinct approaches, Gasification is creating explosive gas combinations from biomass by heating the material at higher temperatures (800–1000°C) with less oxygen present. The method of converting biomass typically results in the formation of syngas, consisting of CO, hydrogen, CH3, and CO2 [Citation69]. Processing that occurs after production has already accounted for most of the total cost. As a result, the process used to cultivate algae and remove their lipids is the single most significant component in algal biofuel production. It is of the utmost importance to locate strains of algae that can produce oil quickly and are rich in lipids. In order to make the process of lipid extraction more effective, it is necessary to combine these strains with newly developed extraction methods. As a result of the low amount of energy, it has been utilized as a fuel for gas engines and turbines in residential settings. Several different syngas combination processes were used in order to transform microalgal biomass into biofuels in an effective manner; the overall representation of third-generation biofuel production from the algal sources has been depicted in the . As part of the conversion procedure, it is feasible to determine the desired product outputs based on the characteristics and composition of various microalgae species to successfully produce various sustainable microalgal biofuels [Citation70]. It also demonstrates the ability to accomplish high photosynthesis efficiency and high lipid content within an integrated process. Which is importantly giving possibilities to for the producing bioethanol, biodiesel, and biogas from a single substrate significantly improves the conversion efficiency of microalgae biomass using different processing methods [Citation71].
The thermochemical liquefaction process is yet another direct method for converting wet algal biomass into bio-oil. This method occurs at low temperatures and high pressure, and a catalyst is not required [Citation72]. Liquefaction has the potential to convert the proteins and carbohydrates that are present in biomass into crude oil in addition to lipids. The efficiency of this process depends on several variables, including temperature, type of catalysts used, length of the retention period, and the characteristics of the biomass (e.g. concentrations of lipids, carbohydrates, and proteins) [Citation73,Citation74]. Supercritical water with a high activity level can break down biomass into molecules with shorter chain lengths with greater energy density. The reaction results in various by-products, including solid, liquid, and gaseous phases. Similar to fossil fuels, biocrude has a similar energy density. Procedures for denitrogenating and deoxygenating the biocrude are necessary, however, to convert it into fuels that may be used [Citation75].
Biomass can be converted in different form, bio-oil, syngas, and charcoal, through a process known as pyrolysis [Citation76]. This process occurs in an oxygen-free environment at temperatures ranging from 350 to 700°C. Rapid pyrolysis with a bio-oil yield of 95% occurs at a moderate of 500°C range and a short duration with hot vapor residence [Citation77]. This combination creates the conditions for the reactions that are compared to slow and fast pyrolysis, a more efficient process requiring less energy and resulting in a greater quantity of bio-oil [Citation78]. Fast pyrolysis yields bio-oil that is better suited for use in liquid fuels due to its lower viscosity compared to bio-oil yielded by slow pyrolysis [Citation79].
Direct combustion, which results in heat generation, is the process of burning biomass while in contact with air. This requires temperatures greater than 800 degrees Celsius, typically in a furnace, boiler, or steam turbine [Citation80]. It is only possible to burn biomass that has a dry-weight moisture content that is less than 50%, and the heat energy that is produced must be used as soon as it is available. Operating a facility that uses direct burning is more expensive since the biomass must undergo additional pretreatment, such as drying and grinding. Despite this fact, significant energy facilities that are fueled by biomass have conversion efficiency that is on par with those of coal power plants.
A variety of metabolic conversions can be accomplished through the processes of anaerobic digestion and alcohol fermentation [Citation81]. Algae material is broken down by bacteria without oxygen, yielding methane, carbon dioxide, and small amounts of hydrogen sulfide [(bio)gas] [Citation82]. Anaerobic digestion describes this metabolic activity in which oxygen is not used. Biogas has a more effective energy content of 20–40% compared to the energy content of biomass, which has a lower heating value. Anaerobic digestion was applied to the processed biomass residue using sludge from an anaerobic digester that had been collected from a wastewater treatment plant [Citation83]. This allowed the biomass residue to be broken down further without the need for oxygen. The anaerobic digestion process is most successful when applied to biomass with higher moisture content. The production of methane by anaerobic digestion was intended to be increased [Citation84]. However, for the microbes to be able to devour the microalgal cells, the cell walls of the microalgae need to be shattered. Compared to physical and chemical processes, enzymatic procedures are currently less suitable for industrial-scale production [Citation85]. In general, the techniques for cell disruption that involve the use of physical means of grinding, ultrasonication, and bead-beating and chemical approaches like acid, base, and ozonation are found to be cost-effective methods.
5. Types of biofuels
5.1. Bioethanol
The production of bioethanol typically makes use of feedstocks from the first or second generation. First-generation bioethanol can be made from various cereals and legumes, including corn, wheat, barley, and sugar beets, all of which are also utilized as food sources [Citation86]. Yeast ferments sugars directly after being derived from first-generation feedstocks such as sugar cane, molasses, sugar beets, and fruits [Citation87]. This process is known as ‘direct yeast fermentation.’ Bioethanol, which has the chemical formula C2H5OH, is a type of liquid biofuel that can be produced by employing several different conversion processes with the help of microbes and biomass feedstocks [Citation88]. The capacity of bioethanol to ensure energy security on a worldwide scale has been recognized by the international community as a significant advantage. On a global basis, bioethanol production is picking up speed at an ever-increasing rate [Citation89]. The vast majority of photobioreactors are able to achieve regulated algal production by controlling variables such as the amount of light, pH level, and carbon dioxide levels, amongst others [Citation90]. Because they are closed systems, there is no evaporation, which eliminates a potential barrier to producing one-of-a-kind biological substances [Citation91]. Microalgae are an especially desirable candidate for use as a biodiesel feedstock because they can produce lipids and have a high photosynthetic efficiency [Citation92]. Macroalgae, valued for the carbohydrates they contain, bioethanol and biogas are produced by the utilization these compounds. The principal area of investigation for research into algae-based biofuels is the production of biodiesel [Citation93]. The structure of algae may contain carbohydrates, which, after undergoing a series of hydrolysis processes, can be converted into ethanol for use in industrial processes. The structural biopolymers hemicellulose and lignin, which are necessary for terrestrial plants but not for aquatic algae, such as algal cells, are not required [Citation94]. This is the case because terrestrial plants need hemicellulose, but marine algae do not. The processes that go into the production of bioethanol can be broken down into four categories: preparation of the feedstock, pretreatment with microbial enzymes (both bacterial and fungal), hydrolysis or saccharification, fermentation with a variety of microbes, including bacteria, fungi, and yeast, distillation, and dehydration as the final steps in the production process. In addition to the production of bioethanol, feedstock sources may also be utilized in the generation of lactic acid and various bio-based products [Citation95]. Increasing the amount of bioethanol that can be made from different substrates requires careful consideration of the pretreatment methods that will be used. The high ash and silica content of the substrate also plays a role in elevated ethanol production [Citation96].
5.2. Biogas production
The anaerobic digestion (AD) process has garnered a lot of interest recently since it can effectively treat a diverse range of substrates while at the same time producing renewable energy. During the AD process, an oxygen-free atmosphere is created, allowing for the breakdown of organic waste such as agricultural leftovers, animal feces, crop plants, sludge, and micro- and macroalgae [Citation97]. This process takes place. This process, which ultimately results in the production of biogas, involves several intricate biochemical reactions. Four basic processes are involved in the production of biomethane. These processes are hydrolysis, acidogenesis, acetogenesis/dehydrogenation, and methanogenesis [Citation98]. The substrate’s molecular makeup, such as the presence of proteins, lipids, carbohydrates, and lignocellulose, has an effect on the first step of the biomethane or biogas production process, which is called hydrolysis [Citation99]. During hydrolysis, fermenting bacteria (FB) like Clostridia, Bifidobacteria, and Bacteriocides can readily convert and complex with biopolymers such as carbohydrates, proteins, and lipids into soluble organic molecules. These bacteria break down complex biopolymers such as amino acids, sugar, and fatty acids. Water-soluble organic molecules are fermented with numerous fermentative bacteria and are used in the acidogenesis and acetogenesis process. These processes result in the production of biohydrogen, carbon dioxide, and acetate. During the final stage of the process, which is referred to as methanogenesis, CO2 is responsible for the production of biomethane and carbon dioxide, respectively. During hydrolysis, high-molecular-weight organic compounds such as lipids, polysaccharides, proteins, and nucleic acids are hydrolyzed into lower-molecular-weight liquid organic compounds [Citation100]. These compounds include lipids, polysaccharides, proteins, and nucleic acids (e.g. sugars, amino acids, and fatty acids). During the acidogenesis process, the hydrolyzed monomers are split even further. At this point, acidogenic bacteria, also known as fermentative bacteria, are responsible for the production of volatile fatty acids. Acetogens further degrade the by-products of the third stage of acidogenesis, producing acetic acid, carbon dioxide, and hydrogen gas as the final products. Methane is produced during the process of methanogenesis by two distinct species of archaea [Citation101].
It is believed that algal biomass, which contains a significant number of organic compounds, has the potential to become a useful fuel for the production of biogas. It was also proposed that biogas produced by microalgae could be a suitable candidate. Producing biogas from the algal biomass that has been gathered from blooms that have occurred in lakes, ponds, and oceans can be used to help in the production of energy and bioremediation [Citation102]. On the other hand, the algae’s biomass contains a significant amount of sulfur, which leads to the creation of the caustic gas H2S. A fiber fraction that comprises indigestible biomass and a liquid fraction that contains salts and nitrogen are both included in the by-product of the biogas production process. This by-product is referred to as the liquid fraction. Although the fiber fraction is difficult to utilize due to its high levels of sulfur and nitrogen, the liquid fraction can be used as fertilizer [Citation103].
The production of biogas from microalgae can be accomplished in a manner that is analogous to that of the production of biogas from other carbon-rich feedstocks. Microalgae biomass may be exploited in conjunction with other carbonaceous feedstocks [Citation104]. There is no restriction on the types of microalgae that can be processed in anaerobic digesters; as a result of the technique, significant energy outputs are produced, and the microalgal biomass can be directly utilized without first being dried. Biogas production from microalgal species is hampered by several factors, including the need for a significant amount of energy to be spent on heating the digesters and high investment costs that include the costs of land and infrastructure [Citation105]. It was discovered that biogas production from microalgae requires a higher amount of energy when compared to the production of biodiesel from microalgae [Citation106].
5.3. Biodiesel
New technologies for the manufacture of biodiesel from photosynthetic microalgae have been to increase the amount of algal biomass that can be produced by relying on the components that have been the primary importance of nowadays research objectives by the global researcher [Citation102]. These components include cultivation, strain selection, biomass harvesting, lipid extraction, and biomass drying, with the transesterification process. The production of biodiesel can use a wide variety of oils, including those derived from plants and animals, as well as discarded cooking oil [Citation107]. To convert these oils into biodiesel, a process called transesterification must first take place. The conversion of oils and fats into biodiesel can be accomplished in three primary ways: first, by using a base catalyst for the transesterification of the oil; second, by using a direct catalyst for the transesterification of the oil; and third, by converting the oil to its fatty acids and then converting those acids into biodiesel [Citation90]. Biodiesel production is possible using various feedstocks, including soybeans, sunflowers, palm fruits, and jatropha. The ability of a species of microalgae to produce lipids is a significant indicator of whether or not it can produce biodiesel. The regulation of culture conditions, in particular the application of a variety of stress conditions, is a common method that can be used to improve the number of lipids produced by microalgae [Citation108]. The fact that the development of the microalgae and the accumulation of lipids do not always occur at the same time during cultivation. This factor reduces the overall amount of lipids that can be produced. Both biomass and lipid concentrations need to be managed carefully to ensure that the production of biofuels derived from microalgae can be economically viable [Citation109]. In addition to providing a workable technical solution to increase lipid contents and reduce biodiesel costs, microalgae treatment of environmental contaminants has made significant progress. This is because microalgae can simultaneously boost lipid contents and reduce production costs [Citation110]. The production of biofuel involves several challenging tasks, one of which is the development of efficient methods for collecting microalgal biomass from the culture broth to assist in lowering the cost of harvesting microalgae, as a result, the cost of producing biodiesel, numerous researchers are actively working on modifying the technologies that are used to harvest microalgae by combining magnetic separation technology, flocculation that is made by an increase in pH, and ultrafiltration [Citation111]. This is being done to help lower the cost of harvesting microalgae. The strain selection and their growth conditions for the efficient production of biofuel from the microalgal species have been listed in .
Table 1. Different culturing conditions and strain selection for the bioenergy production.
5.4. Biohydrogen energy
Biohydrogen is another form of environmentally friendly energy that has the potential to be derived from microalgae. The photobiology process results in hydrogen production by unicellular green algae [Citation110,Citation140]. In addition, various cutting-edge technologies were utilized to produce biohydrogen. These technologies include genomics, transcriptomics, proteomics, and metabolomics [Citation102].
Light-dependent mechanisms, as well as those that are light-independent, can both be used to manufacture biohydrogen. Photofermentation by the direct or indirect biophotolysis process by bacteria, including purple non-sulfur bacteria, are two methods used to produce hydrogen via light [Citation141]. Both of these systems have hydrogen. The dark fermentation process can’t make biohydrogen without the participation of heterotrophic organotrophic bacteria.
By understanding the process by which biomass is converted into hydrogen gas, it may be possible to reduce the negative impact that fuels derived from petroleum have on the surrounding environment [Citation142]. Researchers from China utilized a microwave plasma reactor operating at atmospheric pressure. They performed microwave-assisted pyrolysis at a range of reaction temperatures to investigate algae’s hydrogen production capabilities. The findings from their studies were subsequently utilized in the process of formulating plans for the generation of hydrogen [Citation108]. The production of hydrogen is a complicated process that is affected by a number of factors, one of which is the type of strain. It is possible that optimizing the bioprocess parameters is the key to successfully scaling up the operation of photobioreactors for hydrogen production [Citation107]. Until now, there haven’t been many attempts to illustrate how fuel cells and biohydrogen generation can work together to create something new, and recent advances in the hydrogen production was listed in the with types of organism that are effectively utilized with suitable growth condition for the efficient production. It would be interesting to investigate how effectively fuel cells could operate if biohydrogen production were maintained continuously [Citation140,Citation143].
Table 2. Types of microalgal organisms used for the production of hydrogen.
5.5. An emerging source of bioenergy-microbial fuel cells
In a microbial fuel cell (MFC), hydrogen can be produced by combining the metabolic processes of bacteria with electrochemistry. MFCs are considered to be part of the category of bio electrochemical systems. Oxidation-reduction reactions in a bio electrochemical system could be facilitated by the activity of microorganisms acting as catalysts [Citation147]. Because of the relationship between the metabolic behaviors of these microorganisms and the electrodes, they are often referred to as electroactive microorganisms [Citation148]. In MFCs, the bacteria known as anode-respiring bacteria or exoelectrogenic bacteria produce carbon dioxide, electrons, and protons through the process of oxidizing the organic molecules. When bacteria move an electron from their extracellular space to the anode in an anaerobic environment, the release of protons into the solution results [Citation149]. After that, hydrogen can be formed when the free protons in the solution and the electrons unite at a cathode using a wire. The top of the cathode chamber opened, causing the release of H2 gas, which was subsequently collected. Additionally, various strategies for optimizing the designs of MFCs have been developed. These strategies include increasing the membrane size about the electrode-projected surface area, utilizing anodic electrodes with more significant surface areas, reducing the distance between electrodes, designing various membrane-less two-chamber coupled systems, and developing MFCs that are powered by dye-sensitized solar cells [Citation93].
5.6. Biofuel production process from microalgae
The manufacturing of biofuels consists of three stages: the first stage, the cultivation of plants; the second stage, and the third stage, the fabrication of the finished product. The harvesting of the biomass of the microalgae is the stage that occurs in the middle of the process [Citation150]. Many different types of open-system and closed-system environments are suitable for developing autotrophic and heterotrophic microalgae, respectively. During the harvesting process, the medium containing the microalgae is concentrated, which increases the amount of biomass converted [Citation151]. The transformation of microalgal oil into biodiesel takes place through a process known as transesterification. Because microalgae oil has a more excellent viscosity than petroleum diesel, it must first have its initial viscosity reduced while simultaneously having its fluidity raised before it can be utilized in diesel engines. Fermentation of the cellulose, sugar, or starch present in the biomass of microalgae is the process that leads to bioethanol production [Citation152,Citation153].
5.6.1. Advantages of microalgal biofuel production
Compared to any other time in human history, the second half of the 20th century saw an unprecedented rate of environmental degradation by human activities. This led to an incredible level of habitat destruction and resource depletion. The current pattern of worldwide utilization of energy sources based on fossil fuels is under pressure from policies that are becoming increasingly stringent [Citation154]. Manufacturing with a more negligible impact on the environment and using more sustainable materials are challenges that businesses must overcome. The advancement of sustainable development is driven by several aspects, including difficulties relating to socioeconomics, politics, culture, and humans [Citation155]. It is abundantly apparent that these adverse and long-lasting impacts damage not only the world’s ecosystems but also its population and how they maintain their standard of living. When it comes to producing third-generation biofuels, microalgae are one of the many different types of biomass feedstock sources that are increasingly being utilized [Citation156]. Microalgae could serve as a source of feedstock for manufacturing biofuels and other high-value by-products. Microalgae are grown in water environments and can consist of single-cell or multicellular photosynthetic microorganisms. Microalgae are cultivated for their food value. They can convert the CO2 in the atmosphere into a kind of chemical energy that can then be stored as the lipids, carbs, and proteins that make up the microalgae biomass [Citation157]. As a consequence of this, the microalgae biorefinery process may become more economically efficient as a whole as a result of this. Recent advancements in genetic engineering and metabolic engineering are anticipated to bring about considerable improvements in whether or not producing ethanol from microalgae biomass is viable from a financial and ecological perspective [Citation21].
Compared to fossil fuel consumption, biofuels made from microalgae can significantly reduce the quantity of carbon dioxide (CO2) emitted into the atmosphere [Citation155]. Studies and improvements are being made on numerous microalgae species with the potential to be used in the production of biofuels is a significant benefit to future efforts to cut carbon emissions and increase the sustainability of energy production and consumption [Citation7]. These efforts stand to gain a great deal from this research and development. Future technological advancements in the production and harvesting of microalgae may help to reduce both the global carbon footprint and the consumption of fossil fuels [Citation156].
6. Challenges and prospects
Fossil fuels are examples of conventional energy sources. Providing nutrients (e.g. nitrate and potassium) and fresh water for the microalgae industry is an expensive endeavor, and it is one of the main reasons why the price of producing advanced biofuels has increased when compared to the price of fossil fuel today, the cost of creating biodiesel from microalgae is significantly greater than that of conventional fuel sources [Citation95]. Studies indicate that the cost of manufacturing biodiesel from microalgae can be between 20 and 30%, accounted for by these factors [Citation140].
Because of this, the cost of producing biofuel and the logistics involved in its distribution are both directly influenced by microalgae cultivation. Runoff from fertilizers and pesticides is another risk associated with conventional farming, in addition to the increased risk of contaminating surface water and contributing to the eutrophication of lakes and streams. Environmental concerns like these should be prioritized in any future microalgae-based fuel development to ensure the process’s environmental and social sustainability [Citation30,Citation158]. The ongoing research into this field has laid a solid basis by doing an in-depth analysis of the technical viability and the decrease in production costs, both of which might be the initial stages in more in-depth research and development.
In spite of this, several high-margin secondary products can be produced alongside the energy because microalgae are a rich source of protein, carbs, fatty acids, vitamins, pigments, and so on. Given the capability of microalgae to store carbon, it is also a potentially viable option for energy companies to participate in carbon trading. Microalgae have been shown to be particularly effective in this regard [Citation65,Citation159]. As a result, potential directions for future study could center on the development and introduction of innovative farming techniques [Citation160].
The primary focus of future studies should be the effectiveness of producing genetically modified microalgae strains in a real-world culture setting and innovative approaches for altering the metabolic processes of microalgae. In addition, further research needs to be done to improve the likelihood of effectively expanding existing operations and using industrial modules to cultivate relevant microalgae species [Citation90]. Furthermore, the removal of biomass from microalgae is a significant obstacle. Self-flocculation, also known as bio-flocculation, is a technology that is currently being used extensively since it has been demonstrated to be effective and is available at a cost that is not prohibitive [Citation110].
In today’s world, the environment is under assault from a wide variety of problems, the most prominent of which are resource depletion, climate change, energy security, oil prices, and the price of oil. Significant headway has been made in the research and production of energy and fuels generated from biomass as a direct consequence of these challenges. Therefore, in this regard, it is believed that biofuels would be particularly advantageous, as they will be able to handle these concerns sustainably 158 successfully. It has been determined that using biofuels is the most effective and feasible method for reducing the amount of carbon dioxide emissions produced by the transportation sector. In addition, the production of biofuels can be made quite easily by making use of native resources that are easily accessible in the area. Recently, a lot of focus has been placed on algal biofuels, which are being heralded as the most potentially effective answer to the problem of insufficient energy supply around the world [Citation161]. The two primary advantages of employing algae are the ability of algae to potentially have high productivity and the absence of any competition for land and freshwater resources that they may have with food crops. The development of biofuels is currently the subject of a great number of studies that are being carried out all around the world. Research in the area of biofuels and the industry itself is unquestionably developing at a rapid pace. Significant technological advancements have been made in the manufacture of biofuels, and a comprehensive understanding of the processes employed to produce them has also been attained [Citation162]. The two successes are equally impressive. While biofuels show promise, they are still a long way from fully replacing fossil fuels in transportation. Several integrated engineering and biological approaches are needed to improve biofuel production at a commercial scale [Citation163]. If we are going to create a sustainable biofuels-based economy, we need to know how climate change will affect biofuel production. As a result, biofuels as a replacement for fossil fuels in the future will unquestionably be a top energy supplier in a sustainable way with the ability to increase supply security, which will undoubtedly result in a decrease in the total amount of vehicle emissions [Citation155].
It would be beneficial to develop lipid and product extraction methods that are both more applicable and more efficient to bring down the cost of manufacturing biofuel. Through the use of recycling technology, it may be possible to lower the cost of recovering the solvent once the extraction process has been completed. In order to successfully bring algal oil to the market, it is necessary to give downstream processing a higher priority, focusing on methods that require less energy but produce more products [Citation164]. Microalgae have a high market value as a source of protein even though lipids are lost in recovering the proteins. This is because the selling price for protein is higher than the selling price for biofuel per weight. Therefore, the primary emphasis of future research should be placed on the co-extraction or sequential extraction of multiple products from microalgal biomass, depending on which method is more successful [Citation162]. Irrespective of the sources that are being utilized for biofuel production, the concern about zero-waste management is also gaining more critical in the near future since the usage of waste as an effective source for bioenergy production is being motivated in the many developed and developing countries, for an instance to achieve zero waste various concept various impedance paraments are influenced [Citation165]. Since the amount of land they demand and the emissions they emit into the surrounding environment, landfills are a significant hurdle for policymakers and planners in the process of constructing intelligent and sustainable cities [Citation166]. A zero-waste city can only be built with the help of a wide range of research subjects and expertise. Green/smart mobility, urban resilience, smart cities, the circular economy, and complementary, enabling efforts such as the fab lab and makers movements are examples of these subjects and disciplines to put on into the actions [Citation167].
7. Conclusion
In addition to waste from agricultural practices, waste from industrial activities is the primary source of water contamination in the United States. Along with the depletion of fossil fuel supplies, there is a continuously rising demand for other energy sources. Compared to other physical-chemical therapy techniques, microalgae are the biological agents best suited to treating both WW and the energy crisis. Before algae can be used to produce commercial biofuels, three factors must be carefully considered. These are the species of microalgae that will be utilized, the habitats in which they will thrive, and the extraction methods that will be utilized. Finding reliable renewable energy sources is invariably the most important challenge associated with developing environmentally friendly technologies. Utilizing microalgae is a cutting-edge biotechnology approach that cleanses wastewater while lowering the amount of contamination caused by heavy metals. This method is beneficial to the environment. The most recent statistics on the status of research initiatives aimed at simplifying the process of extracting biofuels from microalgae were seriously considered in this particular study. As was pointed out in this specific study, a growing body of work is being done in the research field about the relationship between microalgae and environmentally friendly technology.
This study examined the benefits that can be obtained as well as the challenges that are currently associated with the production of a wide range of biofuels. These biofuels include biodiesel, bioethanol, biohydrogen, and biomethane, all of which can be made by employing microalgae as a source of biomass. Because wastewater is used as a nutrient in microalgae culture, these fuels can significantly reduce environmental pollution, which is one of the most significant advantages of these fuels, along with their capacity to contribute to sustainable development. However, it may also be advantageous to find and alter microalgae that are resilient enough to tolerate a variety of conditions and stresses within the framework of the water treatment infrastructure that is already in place. Additionally, using the current water treatment method in commercial manufacturing may result in cost reductions; nevertheless, this technology requires significant research before it can be utilized to its full potential. Additionally, researchers in this discipline can study other bioenergy sources and value-added commodities that can profit from microalgae.
Author Contributions
Thanigaivel Sundaram: Conceptualization, Literature review, Investigation, Writing-Original Draft.
Saravanan Rajendran: Conceptualization,Writing-Reviewing and Editing, Funding acquisition, Supervision.
Lalitha Gnanasekaran: Writing-Reviewing and Editing, Investigation, Literature review, Validation
Nova Rachmadona: Writing-Reviewing and Editing, Investigation, Literature review, Validation, Visualization
Jheng-Jie Jiang: Writing-Reviewing and Editing, Investigation, Literature review, Validation,
Kuan Shiong Khoo: Writing-Reviewing and Editing, Validation, Supervision.
Pau Loke Show: Writing-Reviewing and Editing, Validation, Supervision.
Disclosure statement
No potential conflict of interest was reported by the author(s).
Data availability statement
Data sharing not applicable – no new data generated
Additional information
Funding
References
- Azarpour A, Zendehboudi S, Mohammadzadeh O, et al. A review on microalgal biomass and biodiesel production through co-cultivation strategy. Energy Convers Manag. 2022;267:267. doi: 10.1016/j.enconman.2022.115757
- Kim GS, Choi SK, Seok JH. Does biomass energy consumption reduce total energy CO2 emissions in the US? J Policy Model. 2020;42(5):953–25. doi: 10.1016/j.jpolmod.2020.02.009
- Olawore YA, Nwinyi OC. Algae based biofuel and co-products-A review. ASJ Int J Adv Sci Res Rev [Internet]. 2019 [cited 2021 Jul 27];4:74–88. Available from www.academiascholarlyjournal.org/ijasrr/index_ijasrr.htm
- Vijayalakshmi S, Anand M, Ranjitha J. Microalgae Cultiv Biofuels Prod. 2020;251–263.
- Milano J, Ong HC, Masjuki HH, et al. Microalgae biofuels as an alternative to fossil fuel for power generation. Renewable Sustainable Energy Rev. 2016;58:180–197. doi: 10.1016/j.rser.2015.12.150
- Arodudu O, Helming K, Wiggering H, et al. Towards a more holistic sustainability assessment framework for agro-bioenergy systems — a review. Environ Impact Assess Rev. 2017;62:61–75. doi: 10.1016/j.eiar.2016.07.008
- Dewangan A, Yadav AK, Mallick A. Current scenario of biodiesel development in India: prospects and challenges. Energy sources, part a recover. Util Environ Eff. 2018;40(20):2494–2501. doi: 10.1080/15567036.2018.1502849
- Kaniapan S, Hassan S, Ya H, et al. The utilisation of palm oil and oil palm residues and the related challenges as a sustainable alternative in biofuel, bioenergy, and transportation sector: a review. Sustain. 2021;13(6):3110. doi: 10.3390/su13063110
- Ahmad A, Banat F, Alsafar H, et al. Algae biotechnology for industrial wastewater treatment, bioenergy production, and high-value bioproducts. Sci Total Environ. 2022;806:150585. doi: 10.1016/j.scitotenv.2021.150585
- Alazaiza MYD, Albahnasawi A, Ahmad Z, et al. Potential use of algae for the bioremediation of different types of wastewater and contaminants: production of bioproducts and biofuel for green circular economy. J Environ Manage. 2022;324:116415. doi: 10.1016/j.jenvman.2022.116415
- Cheregi O, Ekendahl S, Engelbrektsson J, et al. Microalgae biotechnology in Nordic countries – the potential of local strains. Physiol Plant [Internet]. 2019 [cited 2023 Apr 28];166(1):438–450. 10.1111/ppl.12951
- Karpagam R, Jawaharraj K, Gnanam R. Review on integrated biofuel production from microalgal biomass through the outset of transesterification route: a cascade approach for sustainable bioenergy. Sci Total Environ. 2021;766:144236. doi: 10.1016/j.scitotenv.2020.144236
- Kim JY, Jung JM, Jung S, et al. Biodiesel from microalgae: recent progress and key challenges. Prog Energy Combust Sci. 2022;93:101020. doi: 10.1016/j.pecs.2022.101020
- Rana RL, Lombardi M, Giungato P, et al. Trends in scientific literature on energy return ratio of renewable energy sources for supporting policymakers. Adm Sci [Internet]. 2020 [cited 2022 Nov 30];10(2):21. 10.3390/admsci10020021
- Walters JP, Archer DW, Sassenrath GF, et al. Exploring agricultural production systems and their fundamental components with system dynamics modelling. Ecol Modell. 2016;333:51–65. doi: 10.1016/j.ecolmodel.2016.04.015
- Sulaiman C, Abdul-Rahim AS, Ofozor CA. Does wood biomass energy use reduce CO2 emissions in European Union member countries? Evidence from 27 members. J Clean Prod. 2020;253:253. doi: 10.1016/j.jclepro.2020.119996
- Gomiero T. Are biofuels an effective and viable energy strategy for industrialized societies? A reasoned overview of potentials and limits. Sustain. 2015;7(7):8491–8521. doi: 10.3390/su7078491
- Demirbas A, Fatih Demirbas M. Importance of algae oil as a source of biodiesel. Energy Convers Manag. 2011;52(1):163–170. doi: 10.1016/j.enconman.2010.06.055. Elsevier Ltd.
- Stephens E, Ross IL, King Z, et al. An economic and technical evaluation of microalgal biofuels. Nat Biotechnol. 2010;28(2):126–128. doi: 10.1038/nbt0210-126
- Wigmosta MS, Coleman AM, Skaggs RJ, et al. National microalgae biofuel production potential and resource demand. Water Resour Res [Internet]. 2011 [cited 2023 Jan 12];47(3). doi:10.1029/2010WR009966
- Schenk PM, Thomas-Hall SR, Stephens E, et al. Second generation biofuels: high-efficiency microalgae for biodiesel production. BioEnergy Res. 2008;1(1):20–43. doi: 10.1007/s12155-008-9008-8
- Ranjbar S, Malcata FX. Challenges and prospects for sustainable microalga-based oil: a comprehensive review, with a focus on metabolic and genetic engineering. Fuel. 2022;324:124567. doi: 10.1016/j.fuel.2022.124567
- Chisti Y, Yan J. Energy from algae: current status and future trends. Algal biofuels - a status report. Appl Energy. 2011;88(10):3277–3279. doi: 10.1016/j.apenergy.2011.04.038
- Tredici MR. Photobiology of microalgae mass cultures: understanding the tools for the next green revolution. Biofuels. 2010;1(1):143–162. doi: 10.4155/bfs.09.10
- Behera S, Singh R, Arora R, et al. Scope of algae as third generation biofuels. Front Bioeng Biotechnol. 2015;2:90. doi: 10.3389/fbioe.2014.00090
- AEMMR A, Shalaby EA, Shanab SMM. Enhancement of biodiesel production from different species of algae. Grasas y Aceites. 2010;61(4):416–422. doi: 10.3989/gya.021610
- Baruah J, Nath BK, Sharma R, et al. Recent trends in the pretreatment of lignocellulosic biomass for value-added products. Front Energy Res. 2018;6:6. doi: 10.3389/fenrg.2018.00141
- Kröger M, Müller-Langer F. Review on possible algal-biofuel production processes. Biofuels. 2012;3:333–349. doi: 10.4155/bfs.12.14
- Chew KW, Yap JY, Show PL, et al. Microalgae biorefinery: High value products perspectives. Bioresour Technol. 2017;229:53–62. doi: 10.1016/j.biortech.2017.01.006
- Gendy TS, El-Temtamy SA. Commercialization potential aspects of microalgae for biofuel production: an overview. Egypt J Pet. 2013;22(1):43–51. doi: 10.1016/j.ejpe.2012.07.001
- Johnson MB, Wen Z. Development of an attached microalgal growth system for biofuel production. Appl Microbiol Biotechnol. 2010;85(3):525–534. doi: 10.1007/s00253-009-2133-2
- Ghaffar I, Deepanraj B, Sundar LS, et al. A review on the sustainable procurement of microalgal biomass from wastewaters for the production of biofuels. Chemosphere. 2023;311:137094. doi: 10.1016/j.chemosphere.2022.137094
- Debeni Devi N, Chaudhuri A, Goud VV. Algae biofilm as a renewable resource for production of biofuel and value-added products: a review. Sustain Energy Technol. 2022;53:53. doi: 10.1016/j.seta.2022.102749 Assessments.
- Cho S, Luong TT, Lee D, et al. Reuse of effluent water from a municipal wastewater treatment plant in microalgae cultivation for biofuel production. Bioresour Technol. 2011;102(18):8639–8645. doi: 10.1016/j.biortech.2011.03.037
- Krimech A, Helamieh M, Wulf M, et al. Differences in adaptation to light and temperature extremes of Chlorella sorokiniana strains isolated from a wastewater lagoon. Bioresour Technol. 2022;350:350. doi: 10.1016/j.biortech.2022.126931
- Pittman JK, Dean AP, Osundeko O. The potential of sustainable algal biofuel production using wastewater resources. Bioresour Technol. 2011;102(1):17–25. doi: 10.1016/j.biortech.2010.06.035
- Ali SS, El-Sheekh M, Manni A, et al. Microalgae-mediated wastewater treatment for biofuels production: a comprehensive review. Microbiol Res. 2022;265:265. doi: 10.1016/j.micres.2022.127187
- Xu J, Yao Y, Ehyaei MA, et al. Performance assessment of biomass–geothermal configuration energy resources for cooling and power generation. Energy Sci Eng. 2022;10(9):3650–3666. doi: 10.1002/ese3.1215
- Lam MK, Khoo CG, Lee KT. Biofuels From Algae. 2019;475–506. doi: 10.1016/B978-0-444-64192-2.00019-6
- Ahn Y, Park S, Ji MK, et al. Biodiesel production potential of microalgae, cultivated in acid mine drainage and livestock wastewater. J Environ Manage. 2022;314:314. doi: 10.1016/j.jenvman.2022.115031
- Zahedi R, Ahmadi A, Gitifar S. Reduction of the environmental impacts of the hydropower plant by microalgae cultivation and biodiesel production. J Environ Manage. 2022;304:114247. doi: 10.1016/j.jenvman.2021.114247
- Voloshin RA, Rodionova MV, Zharmukhamedov SK, et al. Review: biofuel production from plant and algal biomass. Int J Hydrogen Energy. 2016;41(39):17257–17273. doi: 10.1016/j.ijhydene.2016.07.084
- Rattanapoltee P, Kaewkannetra P. Utilization of agricultural residues of pineapple peels and sugarcane bagasse as cost-saving raw materials in Scenedesmus acutus for lipid accumulation and biodiesel production. Appl Biochem Biotechnol. 2014;173(6):1495–1510. doi: 10.1007/s12010-014-0949-4
- Zhu L, Yan C, Li Z. Microalgal cultivation with biogas slurry for biofuel production. Bioresour Technol. 2016;220:629–636. doi: 10.1016/j.biortech.2016.08.111
- Stephens E, Ross IL, Mussgnug JH, et al. Future prospects of microalgal biofuel production systems. Trends Plant Sci. 2010;15(10):554–564. doi: 10.1016/j.tplants.2010.06.003
- Alavi M, Miller T, Erlandson K, et al. Bacterial community associated with Pfiesteria-like dinoflagellate cultures. Environ Microbiol. 2001;3(6):380–396. doi: 10.1046/j.1462-2920.2001.00207.x
- Yao S, Lyu S, An Y, et al. Microalgae-bacteria symbiosis in microalgal growth and biofuel production: a review. J Appl Microbiol [Internet]. 2019 [cited 2022 Dec 1];126(2):359–368. doi: 10.1111/jam.14095
- Barclay W, Apt K. Strategies for bioprospecting microalgae for potential commercial applications. In: Second. Handbook of microalgal culture: applied phycology and biotechnology. wiley;2013pp. 69–79. doi: 10.1002/9781118567166.ch4
- Mihic S, Golusin M, Mihajlovic M. Policy and promotion of sustainable inland waterway transport in Europe – Danube River. Sustain Energy Rev. 2011;15(4):1801–1809. doi: 10.1016/j.rser.2010.11.033
- Rezania S, Oryani B, Park J, et al. Review on transesterification of non-edible sources for biodiesel production with a focus on economic aspects, fuel properties and by-product applications. Energy Convers Manag. 2019;201:112155. doi: 10.1016/j.enconman.2019.112155
- Low SS, Bong KX, Mubashir M, et al. Microalgae cultivation in palm oil mill effluent (POME) treatment and biofuel production. Sustainability [Internet]. 2021 [cited 2022 Sep 22];13(6):3247. doi: 10.3390/su13063247
- Gupte AP, Basaglia M, Casella S, et al. Rice waste streams as a promising source of biofuels: feedstocks, biotechnologies and future perspectives. Renewable Sustainable Energy Rev. 2022;167:112673. doi: 10.1016/j.rser.2022.112673
- Zhang W, Wang C, Luo B, et al. Efficient and economic transesterification of waste cooking soybean oil to biodiesel catalyzed by outer surface of ZSM-22 supported different Mo catalyst. Biomass Bioenergy. 2022;167:167. doi: 10.1016/j.biombioe.2022.106646
- Ghosh N, Halder G. Current progress and perspective of heterogeneous nanocatalytic transesterification towards biodiesel production from edible and inedible feedstock: a review. Energy Convers Manag. 2022;270:270. doi: 10.1016/j.enconman.2022.116292
- Mohammad Mirzaie MA, Kalbasi M, Mousavi SM, et al. Investigation of mixotrophic, heterotrophic, and autotrophic growth of Chlorella vulgaris under agricultural waste medium. Prep Biochem Biotechnol. 2016;46(2):150–156. doi: 10.1080/10826068.2014.995812
- Abu-Ghazala AH, Abdelhady HH, Mazhar AA, et al. Valorization of hazard waste: efficient utilization of white brick waste powder in the catalytic production of biodiesel from waste cooking oil via RSM optimization process. Renewable Energy. 2022;200:1120–1133. doi: 10.1016/j.renene.2022.10.045
- Nordin N, Yusof N, Md Nadzir S, et al. Effect of photo-autotrophic cultural conditions on the biomass productivity and composition of Chlorella vulgaris. Biofuels. 2022;13(2):149–159. doi: 10.1080/17597269.2019.1652787
- Athar M, Zaidi S. A review of the feedstocks, catalysts, and intensification techniques for sustainable biodiesel production. J Environ Chem Eng. 2020;8(6):104523. doi: 10.1016/j.jece.2020.104523
- Cheirsilp B, Torpee S. Enhanced growth and lipid production of microalgae under mixotrophic culture condition: effect of light intensity, glucose concentration and fed-batch cultivation. Bioresour Technol. 2012;110:510–516. doi: 10.1016/j.biortech.2012.01.125
- Bellou S, Baeshen MN, Elazzazy AM, et al. Microalgal lipids biochemistry and biotechnological perspectives. Biotechnol Adv. 2014;32(8):1476–1493. doi: 10.1016/j.biotechadv.2014.10.003
- Vazhappilly R, Chen F. Heterotrophic production potential of omega-3 polyunsaturated fatty acids by microalgae and algae-like microorganisms. Bot Mar. 1998;41(1–6):553–558. doi: 10.1515/botm.1998.41.1-6.553
- Pinzi S, Leiva D, López-García I, et al. Latest trends in feedstocks for biodiesel production. Biofuels, Bioprod Bioref. 2014;8(1):126–143. doi: 10.1002/bbb.1435
- Abreu AP, Morais RC, Teixeira JA, et al. A comparison between microalgal autotrophic growth and metabolite accumulation with heterotrophic, mixotrophic and photoheterotrophic cultivation modes. Renewable Sustainable Energy Rev. 2022;159:112247. doi: 10.1016/j.rser.2022.112247
- Lari Z, Abrishamchi P, Ahmadzadeh H, et al. Differential carbon partitioning and fatty acid composition in mixotrophic and autotrophic cultures of a new marine isolate Tetraselmis sp. KY114885. J Appl Phycol. 2019;31(1):201–210. doi: 10.1007/s10811-018-1549-4
- Cai W, Wang L, Li L, et al. A review on methods of energy performance improvement towards sustainable manufacturing from perspectives of energy monitoring, evaluation, optimization and benchmarking. Renewable Sustainable Energy Rev. 2022;159:159. doi: 10.1016/j.rser.2022.112227
- Patel AK, Singhania RR, Di Dong C, et al. Mixotrophic biorefinery: a promising algal platform for sustainable biofuels and high value coproducts. Renewable Sustainable Energy Rev. 2021;152:111669. doi: 10.1016/j.rser.2021.111669
- Patel AK, Singhania RR, Sim SJ, et al. Recent advancements in mixotrophic bioprocessing for production of high value microalgal products. Bioresource Technology. 2021;320:124421. doi: 10.1016/j.biortech.2020.124421
- Dixit M, Gupta GK, Usmani Z, et al. Enhanced bioremediation of pulp effluents through improved enzymatic treatment strategies: a greener approach. Renewable Sustainable Energy Rev. 2021;152:152. doi: 10.1016/j.rser.2021.111664
- Patel AK, Choi YY, Sim SJ. Emerging prospects of mixotrophic microalgae: way forward to sustainable bioprocess for environmental remediation and cost-effective biofuels. Bioresour Technol. 2020;300:300. doi: 10.1016/j.biortech.2020.122741
- Hoang AT, Sirohi R, Pandey A, et al. Biofuel production from microalgae: challenges and chances. Phytochem Rev. 2022;1–38. doi: 10.1007/s11101-022-09819-y
- Escalante J, Chen WH, Tabatabaei M, et al. Pyrolysis of lignocellulosic, algal, plastic, and other biomass wastes for biofuel production and circular bioeconomy: a review of thermogravimetric analysis (TGA) approach. Renewable Sustainable Energy Rev. 2022;169:112914. doi: 10.1016/j.rser.2022.112914
- Hong W, Chen J, Ding Q, et al. Efficient thermochemical liquefaction of microalgae Haematococcus pluvialis for production of high quality biocrude with high selectivity over Fe/montmorillonite catalyst. J Energy Inst. 2021;97:73–79. doi: 10.1016/j.joei.2021.04.004
- Ong HC, Chen WH, Farooq A, et al. Catalytic thermochemical conversion of biomass for biofuel production: a comprehensive review. Renewable Sustainable Energy Rev. 2019;113:109266. doi: 10.1016/j.rser.2019.109266
- Velusamy K, Devanand J, Senthil Kumar P, et al. A review on nano-catalysts and biochar-based catalysts for biofuel production. Fuel. 2021;306:121632. doi: 10.1016/j.fuel.2021.121632
- Gnanasekaran L, Priya AK, Thanigaivel S, et al. The conversion of biomass to fuels via cutting-edge technologies: explorations from natural utilization systems. Fuel. 2023;331:331. doi: 10.1016/j.fuel.2022.125668
- Ağbulut Ü, Sirohi R, Lichtfouse E, et al. Microalgae bio-oil production by pyrolysis and hydrothermal liquefaction: mechanism and characteristics. Bioresour Technol. 2023;376:128860. doi: 10.1016/j.biortech.2023.128860
- Ayub HMU, Ahmed A, Lam SS, et al. Sustainable valorization of algae biomass via thermochemical processing route: an overview. Bioresour Technol. 2022;344:126399. doi: 10.1016/j.biortech.2021.126399
- Ramos A, Monteiro E, Rouboa A. Biomass pre-treatment techniques for the production of biofuels using thermal conversion methods – a review. Energy Convers Manag. 2022;270:270. doi: 10.1016/j.enconman.2022.116271
- Le V, Siriwatwechakul W. Sustainable vancomycin production from industrial residues and the effects of inoculum control to ensure reproducibility. Bioresour Technol Reports. 2021;15:15. doi: 10.1016/j.biteb.2021.100805
- Biswas WK, Barton L, Carter D. Biodiesel production in a semiarid environment: a life cycle assessment approach. Environ Sci Technol. 2011;45(7):3069–3074. doi: 10.1021/es1031807
- Onay M. Biobutanol from microalgae. In: 3rd generation biofuels: disruptive technologies to enable commercial production. Elsevier; 2022. pp. 547–569.
- Kandasamy S, Zhang B, He Z, et al. Microalgae as a multipotential role in commercial applications: current scenario and future perspectives. Fuel. 2022;308:122053. doi: 10.1016/j.fuel.2021.122053
- Akram F, Ul Haq I, Raja SI, et al. Current trends in biodiesel production technologies and future progressions: a possible displacement of the petro-diesel. J Clean Prod. 2022;370. doi: 10.1016/j.jclepro.2022.133479
- Bibi F, Jamal A, Huang Z, et al. Advancement and role of abiotic stresses in microalgae biorefinery with a focus on lipid production. Fuel. 2022;316:123192. doi: 10.1016/j.fuel.2022.123192
- Figueroa-Torres GM, Wan Mahmood WMA, Pittman JK, et al. Microalgal biomass as a biorefinery platform for biobutanol and biodiesel production. Biochem Eng J. 2020;153:107396. doi: 10.1016/j.bej.2019.107396
- Huang X, Bai S, Liu Z, et al. Fermentation of pigment-extracted microalgal residue using yeast cell-surface display: direct high-density ethanol production with competitive life cycle impacts. Green Chem. 2020;22(1):153–162. doi: 10.1039/C9GC02634G
- Tan XB, Zhang YL, Zhao XC, et al. Anaerobic digestates grown oleaginous microalgae for pollutants removal and lipids production. Chemosphere. 2022;308:308. doi: 10.1016/j.chemosphere.2022.136177
- Pinpatthanapong K, Khetkorn W, Honda R, et al. Effects of high-strength landfill leachate effluent on stress-induced microalgae lipid production and post-treatment micropollutant degradation. J Environ Manage. 2022;324:324. doi: 10.1016/j.jenvman.2022.116367
- Nagarajan D, Lee DJ, Varjani S, et al. Microalgae-based wastewater treatment – microalgae-bacteria consortia, multi-omics approaches and algal stress response. Sci Total Environ. 2022;845:157110. doi: 10.1016/j.scitotenv.2022.157110
- Xu L, Weathers PJ, Xiong XR, et al. Microalgal bioreactors: challenges and opportunities. Eng Life Sci. 2009;9(3):178–189. doi: 10.1002/elsc.200800111
- Ranganathan P, Pandey AK, Sirohi R, et al. Recent advances in computational fluid dynamics (CFD) modelling of photobioreactors: Design and applications. Bioresour Technol. 2022;350:126920. doi: 10.1016/j.biortech.2022.126920
- Prabhu C, Navaneetha Krishnan B, Prakash T, et al. Biodiesel unsaturation and the synergic effects of hydrogen sharing rate on the characteristics of a compression ignition engine in dual-fuel mode. Fuel. 2023;334:126699. doi: 10.1016/j.fuel.2022.126699
- Leo VV, Lallawmsangi, Lalrokimi, et al. Microbes as resource of biomass, bioenergy, and biofuel. In: Microbial interventions in agriculture and environment: volume 1 : research trends, priorities and prospects. Springer Singapore; 2019. pp. 241–260. doi:10.1007/978-981-13-8391-5_9.
- Abd Elaziz M, Abualigah L, Issa M, et al. Optimal parameters extracting of fuel cell based on Gorilla troops optimizer. Fuel. 2023;332:126162. doi: 10.1016/j.fuel.2022.126162
- Sathya AB, Thirunavukkarasu A, Nithya R, et al. Microalgal biofuel production: potential challenges and prospective research. Fuel. 2023;332:126199. doi: 10.1016/j.fuel.2022.126199
- Terbish N, Popuri SR, Lee CH. Improved performance of organic–inorganic nanocomposite membrane for bioelectricity generation and wastewater treatment in microbial fuel cells. Fuel. 2023;332:126167. doi: 10.1016/j.fuel.2022.126167
- Siqueira JC, Braga MQ, Ázara MS, et al. Recovery of vinasse with combined microalgae cultivation in a conceptual energy-efficient industrial plant: analysis of related process considerations. Renewable Sustainable Energy Rev. 2022;155:155. doi: 10.1016/j.rser.2021.111904
- Brentner LB, Eckelman MJ, Zimmerman JB. Combinatorial life cycle assessment to inform process design of industrial production of algal biodiesel. Environ Sci Technol. 2011;45(16):7060–7067. doi: 10.1021/es2006995
- Weiss A, Schebek L. The net energy ratio of microalgae biofuels production based on correlated cultivation parameters in flat plate photobioreactors. J Clean Prod. 2021;287:125073. doi: 10.1016/j.jclepro.2020.125073
- Cordero T, Marquez F, Rodriguez-Mirasol J, et al. Predicting heating values of lignocellulosics and carbonaceous materials from proximate analysis. Fuel. 2001;80(11):1567–1571. doi: 10.1016/S0016-2361(01)00034-5
- Sztancs G, Juhasz L, Nagy BJ, et al. Co-Hydrothermal gasification of Chlorella vulgaris and hydrochar: the effects of waste-to-solid biofuel production and blending concentration on biogas generation. Bioresour Technol. 2020;302:122793. doi: 10.1016/j.biortech.2020.122793
- Cabuk B, Duman G, Yanik J, et al. Effect of fuel blend composition on hydrogen yield in co-gasification of coal and non-woody biomass. Int J Hydrogen Energy. 2020;45(5):3435–3443. doi: 10.1016/j.ijhydene.2019.02.130
- Solé-Bundó M, Garfí M, Ferrer I. Pretreatment and co-digestion of microalgae, sludge and fat oil and grease (FOG) from microalgae-based wastewater treatment plants. Bioresour Technol. 2020;298:122563. doi: 10.1016/j.biortech.2019.122563
- Cuellar-Bermudez SP, Magdalena JA, Muylaert K, et al. High methane yields in anaerobic digestion of the cyanobacterium pseudanabaena sp. Algal Res. 2019;44:101689. doi: 10.1016/j.algal.2019.101689
- Cherubini F. The biorefinery concept: using biomass instead of oil for producing energy and chemicals. Energy Convers Manag. 2010;51(7):1412–1421. doi: 10.1016/j.enconman.2010.01.015
- Torres A, Padrino S, Brito A, et al. Biogas production from anaerobic digestion of solid microalgae residues generated on different processes of microalgae-to-biofuel production. Biomass Conv Bioref. 2021;13(6):4659–4672. doi: 10.1007/s13399-021-01898-9
- de Jesus SS, Ferreira GF, Moreira LS, et al. Biodiesel production from microalgae by direct transesterification using green solvents. Renewable Energy. 2020;160:1283–1294. doi: 10.1016/j.renene.2020.07.056
- Ferreira Mota G, Germano de Sousa I, Luiz Barros de Oliveira A, et al. Biodiesel production from microalgae using lipase-based catalysts: current challenges and prospects. Algal Res. 2022;62:102616. doi: 10.1016/j.algal.2021.102616
- Wong WY, Lim S, Pang YL, et al. Synthesis of renewable heterogeneous acid catalyst from oil palm empty fruit bunch for glycerol-free biodiesel production. Sci Total Environ. 2020;727:138534. doi: 10.1016/j.scitotenv.2020.138534
- Faruque MO, Razzak SA, Hossain MM. Application of heterogeneous catalysts for biodiesel production from microalgal oil—a review. Catalysts2020. 2020;10(9):1–25. doi: 10.3390/catal10091025
- Li G, Zhang J, Li H, et al. Towards high-quality biodiesel production from microalgae using original and anaerobically-digested livestock wastewater. Chemosphere. 2021;273:128578. doi: 10.1016/j.chemosphere.2020.128578
- Gim GH, Kim SW. Optimization of cell disruption and transesterification of lipids from botryococcus braunii LB572. Biotechnol Bioprocess Eng. 2018;23(5):550–556. doi: 10.1007/s12257-018-0277-6
- Pandey S, Kumar P, Dasgupta S, et al. Gradient strategy for mixotrophic cultivation of Chlamydomonas reinhardtii: small steps, a large impact on biofuel potential and lipid droplet morphology. BioEnergy Res. 2022;16(1):163–176. doi: 10.1007/s12155-022-10454-w
- Patel A, Krikigianni E, Rova U, et al. Bioprocessing of volatile fatty acids by oleaginous freshwater microalgae and their potential for biofuel and protein production. Chem Eng J. 2022;438:438. doi: 10.1016/j.cej.2022.135529
- Chen X, Ma X, Chen L, et al. Hydrothermal liquefaction of Chlorella pyrenoidosa and effect of emulsification on upgrading the bio-oil. Bioresour Technol. 2020;316:123914. doi: 10.1016/j.biortech.2020.123914
- Paz A, Chalima A, Topakas E. Biorefinery of exhausted olive pomace through the production of polygalacturonases and omega-3 fatty acids by crypthecodinium cohnii. Algal Res. 2021;59:102470. doi: 10.1016/j.algal.2021.102470
- Peter AP, Koyande AK, Chew KW, et al. Continuous cultivation of microalgae in photobioreactors as a source of renewable energy: current status and future challenges. Renewable Sustainable Energy Rev. 2022;154:111852. doi: 10.1016/j.rser.2021.111852
- Saad MG, Dosoky NS, Zoromba MS, et al. Algal biofuels: current status and key challenges. Energies. 2019;12(10):1920. doi: 10.3390/en12101920
- Hussain ZN, Jazie AAAH. Fucus vesiculosus algae oil deoxygenation and cracking: reaction parameter optimization using response surface methodology [Internet]. In: AIP Conference Proceedings. American Institute of Physics Inc.; 2022 [cited 2022 Dec 2]. page 40024. doi: 10.1063/5.0068718
- Onay M. Bioethanol production via different saccharification strategies from H. tetrachotoma ME03 grown at various concentrations of municipal wastewater in a flat-photobioreactor. Fuel. 2019;239:1315–1323. doi: 10.1016/j.fuel.2018.11.126
- Zaki MA, Ashour M, Heneash AMM, et al. Potential applications of native cyanobacterium isolate (Arthrospira platensis NIOF17/003) for biodiesel production and utilization of its byproduct in marine Rotifer (Brachionus plicatilis) production. Sustainability [Internet]. 2021 [cited 2022 Dec 2];13(4):1769. . Doi: 10.3390/su13041769
- Farrokheh A, Tahvildari K, Nozari M. Biodiesel production from the Chlorella vulgaris and Spirulina platensis microalgae by electrolysis using CaO/KOH-Fe3O4 and KF/KOH-Fe3O4 as magnetic nanocatalysts. Biomass Conv Bioref. 2022;12(2):403–417. doi: 10.1007/s13399-020-00688-z
- Sahabudin E, Lee J, Asada R, et al. Isolation and characterization of acid-tolerant Stichococcus-like microalga (Tetratostichococcus sp. P1) from a tropical peatland in Malaysia. J Appl Phycol. 2022;34(4):1881–1892. doi: 10.1007/s10811-022-02762-7
- Mutaf T, Oz Y, Kose A, et al. The effect of medium and light wavelength towards Stichococcus bacillaris fatty acid production and composition. Bioresour Technol. 2019;289:121732. doi: 10.1016/j.biortech.2019.121732
- Goswami RK, Agrawal K, Verma P. Microalgae Dunaliella as biofuel feedstock and β-carotene production: an influential step towards environmental sustainability. Energy Convers Manag X. 2022;13:100154. doi: 10.1016/j.ecmx.2021.100154
- Chen S, Qu D, Xiao X, et al. Biohydrogen production with lipid-extracted Dunaliella biomass and a new strain of hyper-thermophilic archaeon thermococcus eurythermalis A501. Int J Hydrogen Energy. 2020;45(23):12721–12730. doi: 10.1016/j.ijhydene.2020.03.010
- Nishshanka GKSH, Liyanaarachchi VC, Nimarshana PHV, et al. Haematococcus pluvialis: a potential feedstock for multiple-product biorefining. J Clean Prod. 2022;344:131103. doi: 10.1016/j.jclepro.2022.131103
- Hosseini A, Jazini M, Mahdieh M, et al. Efficient superantioxidant and biofuel production from microalga Haematococcus pluvialis via a biorefinery approach. Bioresour Technol. 2020;306:123100. doi: 10.1016/j.biortech.2020.123100
- Amit A, Kumar Ghosh U. Utilization of kinnow peel extract with different wastewaters for cultivation of microalgae for potential biodiesel production. J Environ Chem Eng. 2019;7(3):103135. doi: 10.1016/j.jece.2019.103135
- Vinoth Arul Raj J, Bharathiraja B, Vijayakumar B, et al. Biodiesel production from microalgae Nannochloropsis oculata using heterogeneous poly ethylene glycol (PEG) encapsulated ZnOMn2+ nanocatalyst. Bioresour Technol. 2019;282:348–352. doi: 10.1016/j.biortech.2019.03.030
- Turkkul B, Deliismail O, Seker E. Ethyl esters biodiesel production from Spirulina sp. and Nannochloropsis oculata microalgal lipids over alumina-calcium oxide catalyst. Renewable Energy. 2020;145:1014–1019. doi: 10.1016/j.renene.2019.06.093
- Bumbiere K, Gancone A, Pubule J, et al. Ranking of bioresources for biogas production. Environ Clim Technol. 2020;24(1):368–377. doi: 10.2478/rtuect-2020-0021
- Kendir E, Ugurlu A. A comprehensive review on pretreatment of microalgae for biogas production. Int J Energy Res. 2018 [cited 2022 Dec 2];42(12):3711–3731. 10.1002/er.4100.
- Saleem M, Hanif MU, Bahadar A, et al. The effects of Hot water and Ultrasonication pretreatment of microalgae (Nannochloropsis oculata) on biogas production in anaerobic co-digestion with Cow Manure. Processes [Internet]. 2020 [cited 2022 Dec 2];8(12):1558. doi: 10.3390/pr8121558.
- Kalaimurugan K, Karthikeyan S, Periyasamy M, et al. Emission analysis of CI engine with CeO2 nanoparticles added neochloris oleoabundans biodiesel-diesel fuel blends. In: Materials Today: Proceedings, Tamilnadu, India. Elsevier Ltd; 2020. page 2877–2881.
- Pushpakumari Kudahettige N, Pickova J, Gentili FG. Stressing algae for biofuel production: biomass and biochemical composition of Scenedesmus dimorphus and selenastrum minutum grown in municipal untreated wastewater. Front Energy Res. 2018 cited 2022 Dec 2;6:132. DOI:10.3389/fenrg.2018.00132
- Atmanli A. Experimental comparison of biodiesel production performance of two different microalgae. Fuel. 2020;278:118311. doi: 10.1016/j.fuel.2020.118311
- Bouras S, Katsoulas N, Antoniadis D, et al. Use of biofuel industry wastes as alternative nutrient sources for DHA-Yielding schizochytrium limacinum production. Appl Sci [Internet]. 2020 [cited 2022 Dec 2];10(12):4398. doi: 10.3390/app10124398.
- Ido AL, de Luna MDG, Ong DC, et al. Upgrading of Scenedesmus obliquus oil to high-quality liquid-phase biofuel by nickel-impregnated biochar catalyst. J Clean Prod. 2019;209:1052–1060. doi: 10.1016/j.jclepro.2018.10.028
- Mona S, Kumar SS, Kumar V, et al. Green technology for sustainable biohydrogen production (waste to energy): a review. Sci Total Environ. 2020;728:138481. doi: 10.1016/j.scitotenv.2020.138481
- Bhatia SK, Mehariya S, Bhatia RK, et al. Wastewater based microalgal biorefinery for bioenergy production: progress and challenges. Sci Total Environ. 2021;751:751. doi: 10.1016/j.scitotenv.2020.141599
- Goswami RK, Mehariya S, Obulisamy PK, et al. Advanced microalgae-based renewable biohydrogen production systems: a review. Bioresour Technol. 2021;320:320. doi: 10.1016/j.biortech.2020.124301
- Nagarajan D, Chang JS, Lee DJ. Pretreatment of microalgal biomass for efficient biohydrogen production – Recent insights and future perspectives. Bioresour Technol. 2020;302:122871. doi: 10.1016/j.biortech.2020.122871
- Rashid N, Rehman MSU, Memon S, et al. Current status, barriers and developments in biohydrogen production by microalgae. Renewable Sustainable Energy Rev. 2013;22:571–579. doi: 10.1016/j.rser.2013.01.051
- El-Dalatony MM, Zheng Y, Ji MK, et al. Metabolic pathways for microalgal biohydrogen production: current progress and future prospectives. Bioresour Technol. 2020;318:124253. doi: 10.1016/j.biortech.2020.124253
- Nagarajan D, Lee DJ, Kondo A, et al. Recent insights into biohydrogen production by microalgae – from biophotolysis to dark fermentation. Bioresour Technol. 2017;227:373–387. doi: 10.1016/j.biortech.2016.12.104
- Sovacool BK, Cabeza LF, Pisello AL, et al. Decarbonizing household heating: reviewing demographics, geography and low-carbon practices and preferences in five European countries. Renewable Sustainable Energy Rev. 2021;139:139. doi: 10.1016/j.rser.2020.110703
- Liu Y, Lyu Y, Tian J, et al. Review of waste biorefinery development towards a circular economy: from the perspective of a life cycle assessment. Renewable Sustainable Energy Rev. 2021;139:110716. doi: 10.1016/j.rser.2021.110716
- Behera HT, Mojumdar A, Das SR, et al. Microbial intervention in sustainable production of biofuels and other bioenergy products [Internet]. In: Environmental and agricultural microbiology. Wiley; 2021 cited 2022 Dec 2. pp. 361–381. 10.1002/9781119525899.ch17
- Poompavai T, Kowsalya M. Control and energy management strategies applied for solar photovoltaic and wind energy fed water pumping system: a review. Renewable Sustainable Energy Rev. 2019;107:108–122. doi: 10.1016/j.rser.2019.02.023
- Dancs G, Kakucska G, Dobrányi S, et al. Efficient method for the determination of the neutral lipid content of oil-producing microalgae strains required for biodiesel. Fuel. 2023;331:331. doi: 10.1016/j.fuel.2022.125831
- Menegazzo ML, Fonseca GG. Biomass recovery and lipid extraction processes for microalgae biofuels production: a review. Renewable Sustainable Energy Rev. 2019;107:87–107. doi: 10.1016/j.rser.2019.01.064
- Debnath C, Bandyopadhyay TK, Bhunia B, et al. Microalgae: sustainable resource of carbohydrates in third-generation biofuel production. Renewable Sustainable Energy Rev. 2021;150:111464. doi: 10.1016/j.rser.2021.111464
- Xue J, Balamurugan S, Li T, et al. Biotechnological approaches to enhance biofuel producing potential of microalgae. Fuel. 2021;302:121169. doi: 10.1016/j.fuel.2021.121169
- Khan S, Naushad M, Iqbal J, et al. Production and harvesting of microalgae and an efficient operational approach to biofuel production for a sustainable environment. Fuel. 2022;311:122543. doi: 10.1016/j.fuel.2021.122543
- Ebhodaghe SO, Imanah OE, Ndibe H. Biofuels from microalgae biomass: a review of conversion processes and procedures. Arab J Chem. 2022;15(2):15. doi: 10.1016/j.arabjc.2021.103591
- Singh D, Sharma D, Soni SL, et al. A review on feedstocks, production processes, and yield for different generations of biodiesel. Fuel. 2020;262:116553. doi: 10.1016/j.fuel.2019.116553
- Kumar MS, Buddolla V. Future prospects of biodiesel production by microalgae: a short review. In: Recent developments in applied microbiology and biochemistry. Elsevier; 2018. pp. 161–166. doi:10.1016/B978-0-12-816328-3.00012-X.
- Gourvenec S, Sturt F, Reid E, et al. Global assessment of historical, current and forecast ocean energy infrastructure: Implications for marine space planning, sustainable design and end-of-engineered-life management. Renewable Sustainable Energy Rev. 2022;154:154. doi: 10.1016/j.rser.2021.111794
- Hossain N, Mahlia TMI. Progress in physicochemical parameters of microalgae cultivation for biofuel production. Crit Rev Biotechnol. 2019;39(6):835–859. doi: 10.1080/07388551.2019.1624945
- Olabi AG, Shehata N, Sayed ET, et al. Role of microalgae in achieving sustainable development goals and circular economy. Sci Total Environ. 2023;854:854. doi: 10.1016/j.scitotenv.2022.158689
- Kim B, Heo HY, Son J, et al. Simplifying biodiesel production from microalgae via wet in situ transesterification: a review in current research and future prospects. Algal Res. 2019;41:101557. doi: 10.1016/j.algal.2019.101557
- Jeyakumar N, Hoang AT, Nižetić S, et al. Experimental investigation on simultaneous production of bioethanol and biodiesel from macro-algae. Fuel. 2022;329:125362. doi: 10.1016/j.fuel.2022.125362
- Chamkalani A, Zendehboudi S, Rezaei N, et al. A critical review on life cycle analysis of algae biodiesel: current challenges and future prospects. Renewable Sustainable Energy Rev. 2020;134:110143. doi: 10.1016/j.rser.2020.110143
- Ali SS, Mastropetros SG, Schagerl M, et al. Recent advances in wastewater microalgae-based biofuels production: a state-of-the-art review. Energy Rep. 2022;8:13253–13280. doi:10.1016/j.egyr.2022.09.143
- Awasthi AK, Cheela VRS, D’Adamo I, et al. Zero waste approach towards a sustainable waste management. Resour Environ Sustain. 2021;3:100014. doi: 10.1016/j.resenv.2021.100014
- Iqbal MW, Kang Y, Jeon HW. Zero waste strategy for green supply chain management with minimization of energy consumption. J Clean Prod. 2020;245:245. doi: 10.1016/j.jclepro.2019.118827