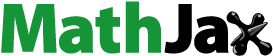
ABSTRACT
This investigation is a review of the potential of aerobic granular sludge membrane bioreactor (AGMBR) in wastewater treatment due to the advantage of combination of membrane and aerobic granules for reducing membrane fouling and enhancing removal performance. The AGMBR is the same as the membrane bioreactor (MBR), but the activated sludge is replaced by aerobic granular sludge. This technology combines the advantages of aerobic granular sludge, such as good settleability, strong ability to withstand shock-loadings and high organic loading rate, and capacity of simultaneous chemical oxygen demand (COD) and nitrogen removal, and advantages of membrane bioreactor (MBR) such as excellent effluent quality, high biomass content, low excess sludge production, and small land requirement. Therefore, it can be considered a promising option for efficient wastewater treatment. Most studies have shown that aerobic granules could control membrane fouling, which often occurs in MBR. The main fouling mechanism was determined to be surface fouling by floccular sludge in MBR but pore fouling by colloids and solutes in AGMBR. Aerobic granular sludge also removed COD and nitrogen simultaneously, with more than 60% total nitrogen removal efficiency. The formation and stability of aerobic granules in AGMBR with various operational modes are discussed in this study.
Introduction
The advancement of wastewater treatment methods has led to the emergence of the Membrane Bioreactor (MBR) technology, which seamlessly integrates the activated sludge process with membrane filtration [Citation1]. This innovation effectively segregates solids within mixed liquids and offers a host of advantages over the conventional Activated Sludge (CAS) process. Notably, MBR excels in generating superior effluent quality, occupying minimal space, and generating minimal excess sludge. A distinctive attribute of MBR lies in the submicron size of its membrane pores, enabling comprehensive retention of biomass, including bacteria and viruses. This unique trait also transforms the membrane into a disinfection mechanism. The exceptional permeate quality from MBR, characterized by its colorless nature, low Chemical Oxygen Demand (COD) levels, and absence of solid concentration, facilitates direct reuse without additional treatment.
MBR’s prowess in retaining biomass empowers it to operate under significantly higher biomass concentrations – exceeding 8,000 mg/l – beyond the customary 3,000–4,000 mg/l encountered in conventional biological wastewater treatment. However, a pivotal setback of MBR technology is membrane fouling, a phenomenon that hampers productivity and escalates operational and energy costs, thereby limiting the widespread application of MBR [Citation2].
The determinants influencing membrane fouling in MBR encompass sludge characteristics, reactor operational conditions, and membrane material properties. Among these, the properties of sludge wield a profound impact on the trajectory of membrane fouling. Consequently, manipulating sludge attributes emerges as a promising approach to mitigate fouling in MBR. Various strategies have been proposed, including the introduction of adsorption materials (such as activated carbon and zeolite) or coagulants (polyamide, polyaluminum chloride, diatomite) to adsorb colloids and solutes, along with enhancements to the sludge’s structure via carrier material additions to cultivate a biofilm [Citation3]. Nonetheless, such material augmentations often diminish sludge activity due to inert substances, precipitating inorganic fouling when the dosage surpasses limits and incurring substantial chemical and material costs [Citation4].
In the late 1990s, the emergence and application of aerobic granules, as introduced by Morgenroth [Citation5], ignited fervent research interest. Aerobic Granular Sludge (AGS) represents a distinct biofilm development, wherein microorganisms self-immobilize sans carriers or coagulation agents, yielding compact granules under suitable shear forces. AGS boasts several advantages over CAS, including a well-organized structure, heightened bioactivity, robust biomass retention, efficient settling velocity, and simultaneous nitrification-denitrification capabilities [Citation6,Citation7]. Notably, the granule settling velocity far surpasses CAS (10 m/h versus roughly 1 m/h), and the sludge volume index (SVI) diminishes by about 12 mL/g [Citation8]. Moreover, AGS systems can sustain organic loading rates (OLR) as high as 15 kg COD/m3] day [Citation9]. Capitalizing on these strengths, aerobic granules offer a potent avenue for transforming activated sludge to counter fouling in MBR. Yet, the AGS process faces challenges in meeting discharge standards due to elevated suspended solid concentrations in effluents, often necessitating sedimentation or membrane filtration. Thus, synergizing AGS with membrane filtration could surmount drawbacks, effectively managing fouling and enhancing removal efficiency for COD, nitrogen, and suspended solids.
Enter the Aerobic Granular Sludge Membrane Bioreactor (AGMBR) technology, seamlessly fusing AGS with membrane filtration and surpassing conventional biological approaches in numerous aspects. While most studies have thus far been confined to lab-scale setups employing synthetic wastewater, recent efforts encompass larger-scale investigations on real wastewater treatment [Citation10–12]. These endeavors underscore AGS’s pivotal role in both fouling mitigation and removal efficiency enhancement. This burgeoning field offers a promising avenue for supplanting conventional MBR technology in practical wastewater treatment and reuse scenarios. Given the nascent status of this technology, this comprehensive review aims to illuminate the contours of AGMBR technology and its latent potential in wastewater treatment. This study delves into intricate aspects, including (1) the dynamics of AGS formation and stability across various operational modes in AGMBR; (2) a nuanced comparison of membrane filterability and fouling phenomena between AGMBR and MBR; (3) the treatment efficacy of AGMBR concerning COD, nitrogen, and phosphorus.
Overview of AGMBR technology
The AGMBR technology combines an aerobic granular sludge process and membrane filtration, the same as MBR technology, but the difference is that activated sludge is replaced by aerobic granular sludge in this system. In AGMBR, bacteria decompose substrates in the influent; the membrane separates the microorganisms and some macromolecules, allowing the water and dissolved species to pass through. Like MBR, membrane modules are located inside or outside the bioreactor to form the submerged AGMBR and the external AGMBR. Submerged – MBR has widespread application in wastewater treatment due to the advantages of less energy requirement, lower cost of fabrication and maintenance due to lack of housing, higher permeability in full-scale operating conditions; the impact of shear on sludge properties and performance is minimized due to the modest suction pressure at 5–30 kPa; higher biomass concentration induces increased treatment efficiency.
The AGMBR technology has attracted the interest of researchers in practical wastewater treatment [Citation13]. The vast majority of the studies may have been harvested from the Scopus database (accessed on 27 March 2023), illustrated in :
Figure 1. Scopus number of published articles per year (a) and country (b) by AGMBR with the keywords “aerobic granular membrane bioreactor”.

shows that the total number of articles with the key ‘Aerobic granular membrane bioreactor’ retrieved from the Scopus database was 181. Due to the advantages of aerobic granules in membrane fouling reduction and removal enhancement, the number of studies conducted on AGMBR has steeply increased since 2005 with the number of published articles per year of about 10–18. The data analysis reveals that 38 countries have contributed to AGMBR wastewater research, and the contributions vary from country to country. China had the highest research output (50.3%), followed by the United States (9.9%), Taiwan (8.3%), Canada (6.1%), and South Korea (4.4%). The results also indicate that environmental science was determined to be of the highest interest within research areas, ranking around 35.2% (data not shown). In environmental science, remediation of nutrients, pharmaceuticals, and textiles in wastewaters may be among Scopus’ most frequently studied areas.
The combination of AGS and membrane filtration is carried out in two ways: (1) Cultivate aerobic granules into MBR alter for activated sludge and operate the reactor in continuous mode; (2) Install membrane modules into the granulation reactor and operate the reactor in batch mode. Operational mode affected the characteristic and structure of aerobic granules, leading to an effect on filterability and treatability of AGMBR. Therefore, the studies concentrated on evaluating filterability and substrate removal efficiency, along with the stabilization of aerobic granules. The causes and main foulants in the AGMBR were also estimated. Currently, studies of AGMBR technology are mostly carried out in lab scale with synthetic wastewater from glucose [Citation13–19], acetate [Citation20–26], methanol [Citation27], etc. … However, up to now there are some studies for real wastewater treatment such as paper mill wastewater [Citation28], Ilsan wastewater [Citation29], municipal wastewater [Citation6], real mixed wastewater [Citation30] and large scale such as pilot scale to treat domestic wastewater [Citation12], refractory wastewater [Citation10] and full scale for industrial wastewater treatment [Citation11]. The results show the role of aerobic granular sludge in membrane fouling control and removal efficiency improvement.
Formation and stabilization of AEROBIC GRANULES in AGMBR
The results in show that the formation and stabilization of aerobic granules in AGMBR depend on the operational mode of the reactor. Operating the lab-scale AGMBR in a batch mode similar to a sequencing batch reactor (SBR), researchers cultivated and maintained the stability of aerobic granules with a diameter of 0.5–1.0 mm and SVI of 40–50 mL/g [Citation14,Citation23,Citation31,Citation33]. In some cases, when the membrane module was installed in the SBR, the granules were destabilized and reduced in size but then stabilizes [Citation34]. For these reasons, the granular sludge is rubbed by the membrane, the oxygen diffusion in the reactor is uneven due to the effection of the membrane module, or the floc is trapped in the reactor and competes with the granular sludge. In the batch operational mode known as SBR but have no settling and directly discharge phases, Tay, J. H [Citation23] observed the aerobic granules to be unstable after an operational 20 days, while Tu, X [Citation18] showed aerobic granules to be able to stabilize at the diameter of 0.5–1.0 mm and SVI of 40 mL/g during 180 days.
Table 1. Formation and stabilization of AGS in AGMBR.
Operating the AGMBR in continuous mode as conventional MBR did not provide suitable conditions for stabilizing aerobic granules. Wang, J [Citation25] observed the disintegration of granules having a large size of more than 0.9 mm when inoculated into MBR at the first operational days and just maintained the small granules with a diameter of 0.18–0.9 mm in the reactor after operating 70 days. Li, X [Citation15,Citation16] also observed that the characteristics of granules were worse through the decrease in diameter from 3 mm down to 2 mm and the increase of SVI from 42 mL/g up to 70 mL/g after working 55 days. The size of the aerobic granules decreased significantly from 2.4 mm to 1.9 mm during the initial 6 days in a continuous reactor and then remained stable at around 1.2 mm after 30 operational days [Citation35]. The granules in continuous AGMBR also had a small size with a diameter of 0.7 mm [Citation36,Citation37]. The unstabilization was explained because the floccular sludge was not discharged and kept by the membrane in continuous AGMBR, so it competed with granules and did not enhance the granulation. Moreover, the continuous influent flow did not cause an alternative feast and famine conditions in the reactor, so it did not promote the release and use of extracellular polymeric substances (EPS), leading to the limitation of the granulation. It has been believed that appropriately bound EPS (bEPS) amounts of approximately 100–150 mg/gSS would increase the compact structure of granules. In contrast, EPS concentrations higher than 200 mg/gSS resulted in the occlusion of granule porosity and the limitation of nutrient transport from the bulk into granules and consequently the death of bacteria [Citation16].
The AGMBR model operated in batch mode showed suitable conditions for the stabilization of aerobic granules due to the batch influent making a different condition of substrate concentration in a cycle; direct effluent discharge to remove the flocs having bad settle ability [Citation14,Citation18,Citation20,Citation23,Citation27,Citation31]. Batch AGMBR created stressful cultural conditions for the production and consumption of EPS. EPS was mainly produced in the feast phase and then consumed in the famine phase when bacteria spent longer time in endogenous respiration conditions. Therefore, the EPS content in the reactor was always controlled appropriately to stabilize the granular structure. In addition, the frequent discharge of poorly settling flocs in the reactor also played an important role. The floccular sludge mainly includes heterotrophic aerobic bacteria, which have quick growth rates. Meanwhile, the bacteria inside the granules are more difficult to contact with the substrate, so they have lower growth rates and are easily competed by bacteria in the flocs. Therefore, the floccular sludge in the AGMBR should be withdrawn frequently to restrict the competition between flocculent and granular sludge for the long-term stabilization of AGS. Obviously, batch operation and floc removal maintained the stability of aerobic granules in the AGMBR.
Operational parameters also affect the stability of granular sludge. Some studies have maintained the stability of granules; however, the size of the granules was rather small, less than 1 mm, and the morphology was not uniform [Citation6,Citation14,Citation23,Citation31]. The experimental conditions disadvantaged the granulation in this literature, such as a low OLR of 0.25 kg TOC (total organic carbon)/m3] day [Citation6], low surface air velocity of 0.1 cm/s [Citation31] and high HRT 1 day [Citation14], resulting in a decrease in granule size. Suitable operational conditions in our previous study, such as high air velocity of 2.5 cm/s, high OLR of 2.5 kg COD/m [Citation3] day, and short HRT of 7.56 h, favored the stability of aerobic granules with the 1–2 mm granular particles accounting for 77.5% [Citation7]. The increase in the general surface gas velocity or gas flow creates a hydraulic disturbance, the major shear condition for granular formation [Citation41]. It has been reported that high OLR is beneficial for accumulating biomass by secreting more EPS and shortening the time required to reach a steady state [Citation42,Citation43]. At short settling and hydraulic retention times, floccular sludge is washed out to restrict competition with granular sludge to stimulate granulation.
Indeed, there are many factors that influence AGS formation, including operational parameters and the properties of wastewater. The effect of operating parameters on the formation and stability of AGS in the AGMBR is nearly similar to that in the SBR. High air velocity and OLR as well as short settling and hydraulic retention times favored the formation and stability of aerobic granules in AGMBR. In order to maintain the stability of the AGS, it is necessary to control OLR in the appropriate range of about 2.5–5 kgCOD/m [Citation3] day [Citation7,Citation42], the surface air velocity of 1–3 cm/s [Citation44–46], HRT of about 6–12 h [Citation5,Citation46,Citation47]. The settling time should be chosen so that the settling velocity of sludge particles is higher than 10 m/h [Citation48]. AGS is unstable at low DO concentrations (40% saturation) because when DO is low, filamentous bacteria will grow and cause the granular sludge to decompose [Citation49]. The suitable DO range (1.5–2.0 mg/L) was an important guarantee to maintain the stability of the structure of AGS in AGMBR [Citation36]. The property of wastewater also affects aerobic granulation. AGS can be grown on many different substrates with high organic matter and nutrient content such as synthetic wastewater (acetate, glucose, peptone, sucrose, …) [Citation42,Citation45,Citation50], domestic and industrial wastewater [Citation51–53]. However, the structure and microbial composition in AGS cultured with different substrates will be different. Remarkably, free ammonia is inhibitory to most bacterial species, so it affects the granules. At free ammonia concentrations higher than 23.5 mg/L, AGS cannot form [Citation54].
At present, very few studies have been able to maintain a large and stable granular sludge with an average size of about 2 mm [Citation7,Citation32]. Granules with a size smaller than 1 mm and not regular were often maintained even in batch-operated AGMBR. Meanwhile, the granules with small sizes are still maintained in modified batch-operated AGMBR with no sludge settling phase and no directly effluent discharge phase [Citation18] and in modified continuous-operated AGMBR with directly effluent discharge phase [Citation22], or no excess sludge discharge [Citation38,Citation39]. Moreover, it was possible that aerobic granules were cultivated from aerobic floccular sludge and preserved their morphology for a long time in continuous MBR if the operating parameters were well controlled to maintain a suitable EPS concentration [Citation16].
The batch AGMBR can be operated in two ways with the membrane module located inside or outside the bioreactor, corresponding to the submerged AGMBR and the external AGMBR. For the external AGMBR, the reactor is operated similarly to the SBR and the granular sludge is not affected by the membrane module. For submerged AGMBR, the membrane filtration affects the granular sludge due to the collision between the granular sludge and the membrane. Moreover, for some studies, the treated water is discharged through the membrane and there is no directly effluent discharge, so the flocculent sludge is not discharged regularly and competes with the granular sludge. However, experiments in different conditions showed inconsistent results. Operating the AGMBR with the same parameters and modes as the aerobic granular sludge SBR, it was possible to maintain the stability of the large-size granular sludge with an average size of about 2 mm even if the membrane module is submerged [Citation7,Citation32]. Meanwhile, AGS with a small size lower than 1 mm was maintained in external AGMBR [Citation10,Citation11,Citation40]. The reason is that large granules are difficult to stabilize in reactors having such a long anaerobic filling time and large scale.
In reality, the development and stability of the aerobic granules in AGMBR are still inconsistent with these studies due to their different operating modes. Therefore, it is necessary to conduct further studies to determine suitable operation modes to maintain the stability of aerobic granules with larger size and more compact structure for effective control of membrane fouling in AGMBR.
Membrane filterability and fouling in AGMBR
Membrane filterability of AGMBR
Comparing the filterability of Anaerobic Granular Sludge Membrane Bioreactor (AGMBR) with that of a Membrane Bioreactor (MBR), an analysis of the studies detailed in reveals a consistent trend favoring AGMBR’s superior filterability. Evident in short-term membrane filtration experiments, Zhou et al. [Citation55] and Liu et al. [Citation27] observed higher flux values in AGMBR compared to MBR. Moreover, findings from Li’s 16-day long-term filtration study (13) aligned with this outcome. In a continuous filtration scenario spanning 32 days, Li et al. [Citation13] demonstrated that cleaning fouled AGMBR membranes required only two cycles, whereas MBR demanded up to four cycles. Additionally, during a short-term filtration of 30 h, AGMBR exhibited a gradual decrease in flux alongside an increase in transmembrane pressure (TMP), further affirming its better performance.
Table 2. Comparison of the filterability of AGS and flocculent sludge.
Extended filtration periods strengthened these observations. A 180-day filtration experiment of Tu et al. [Citation18] established AGMBR’s favorable filterability, maintaining a specific flux exceeding 1.3 L/m2 h.kPa at a TMP below 8 kPa. Similarly, Wang et al. [Citation31] conducted at a high flux of 20 L/m2 h, correlated enhanced filterability with granule diameter. Critical TMP thresholds of 50 kPa were surpassed after 10, 14, 19, and 61 days for block sludge, floc, small granules, and large granules, respectively. Notably, an increase in granular sludge within the reactor led to elevated permeate flux and reduced fouling resistance [Citation33].
The application of full-scale AGS membrane filtration in industrial treatment provided further validation. Permeability in large granular reactors ranged from 158.9 to 190.8 L/m2 h.bar, surpassing that of small granular reactors (89.9 L/m2 h.bar) by 50% [Citation11]. This conclusion was corroborated by other researchers [Citation17,Citation24,Citation33,Citation35].
undertakes a comparative assessment of granular and floccular sludge filterability, gauged through the filterability ratio of granular to floccular sludge. Two approaches were employed for filtration experiments: (1) maintaining a constant TMP equivalent to the filterability ratio (granule flux/floc flux) or (2) upholding a constant flux equivalent to the filterability ratio (floc TMP/granule TMP). Various operational conditions – filtration time, TMP, flux – were manipulated along with distinct sludge characteristics, yielding diverse filterability outcomes. Notwithstanding these variables, a consistent trend emerged, establishing granular sludge’s higher filterability over floccular sludge. This phenomenon was attributed to granular sludge’s propensity to remain suspended rather than settling on the membrane surface, thus reducing the density of the sludge cake layer and enhancing filterability, particularly at high flux or low TMP [Citation23,Citation31].
Membrane fouling phenomena and mechanism in AGMBR
Membrane fouling phenomena and mechanism are the most heavily investigated areas in MBR publications. Most studies have shown that fouling is primarily caused by the larger soluble molecules plugging and narrowing the pores of membranes or the particles/colloids depositing on the membrane surfaces to form a cake layer. In the initial stage, substances having sizes less than membrane pores easily block the membrane pores; simultaneously, the greater-size substances tend to form cake layers on membranes. Long term, the foulants may fill in the voids of the formed cake layers or contribute to increasing the cake layers. Membrane fouling leads to increased TMP and frequent membrane cleaning, so it has high operation and maintenance costs.
There are two main fouling mechanisms: reversible fouling and irreversible fouling. Reversible fouling is caused by the cake layer, which is readily removable under a physical washing protocol. In contrast, irreversible fouling indicates that internal fouling is caused by the adsorption and/or deposition of soluble matter into the membrane pores, which is generally removed by chemical cleaning [Citation57]. Therefore, the total resistance (Rt) consists of net membrane resistance (Rm), reversible resistance (or cake – layer resistance – Rc) and irreversible resistance (or pore resistance – Rp) [Citation27,Citation31,Citation55,Citation58].
The results in show that the main fouling mechanism in MBR is due to the suspended solid having a small size adhered to the membrane surface and increased cake-layer resistance (Rc = 60–70% Rt). The main fouling mechanism in AGMBR is due to colloids and solutes filling the pore of the membrane and increased pore fouling resistance (Rp = 45–70% Rt). These data also showed that MBR’s total resistance (Rt) was 2–3 times higher than that of AGMBR due to the cake-layer resistance (Rc) of MBR being 4–13 times higher than that of AGMBR. This explains why aerobic granules with a large diameter and compact structure led to reduced adherence to the membrane surface and formed a thin and porous cake layer, reducing Rc [Citation6,Citation7,Citation31]. These results are also proven by the Carman-Kozeny equation, which states that the specific cake-layer resistance has an inverse relationship with particle size [Citation55]. In addition, soluble EPS (sEPS), considered key fouling factors, were synthesized into granules for stable granulation, resulting in the reduction of membrane foulant amount in the AGMBR three times compared with the MBR [Citation7].
Table 3. Comparison of filtration resistance of AGS and floccular sludge.
also shows the fouling mechanisms of the MBR and AGMBR. The deposition of fine particles in activated sludge on the membrane surface may induce a dense surface image of the MBR. Under the high suction force of membrane filtration, fine particles would easily and quickly accumulate on the membrane surface to form a compact cake layer. The substances smaller than the membrane’s pore size entered into the pore and caused blocking. The thick cake layer that developed on the membrane surface in the MBR could serve as a barrier to restrict the deposit of pore-blocking substances, such as solutes and colloids, into the membrane pores. This is why the MBR had a lower pore resistance than that of the AGMBR [Citation7,Citation55]. In the AGMBR fouled membrane, the cake layer formed more porosity compared with the MBR. The aerobic granules had a large size and compact structure and did not easily attach to or clog on the membrane surface. Thus, the formed cake layer had a loose structure, which was beneficial for maintaining permeability [Citation7,Citation23]. Indeed, in order to reduce membrane fouling in AGMBR, it is necessary to maintain stable granular sludge because of the benefit of granules in membrane filterability. Moreover, when granular sludge is disintegrated, many damaged cells, EPS, and soluble substances are released into mixed liquor. These small particles and soluble substances swiftly cover or fill the membrane pores, resulting in the loss of membrane filterability [Citation23].
Figure 2. Schematic representation of membrane fouling in an MBR with floccular sludge (A) and in an AGMBR with aerobic granular sludge (B) [Citation60].
![Figure 2. Schematic representation of membrane fouling in an MBR with floccular sludge (A) and in an AGMBR with aerobic granular sludge (B) [Citation60].](/cms/asset/5af8bae6-32cc-430f-8bbc-273770dac36b/kbie_a_2260139_f0002_oc.jpg)
It can be disclosed from the resistance and SEM analysis findings that the key fouling mechanism of the MBR was cake layer fouling, while that of the AGMBR was pore blocking [Citation6,Citation7,Citation31,Citation55,Citation59,Citation60]. The cake layer resistance of AGMBR is obviously lower than that of MBR. However, there has not yet been a high agreement when comparing pore blocking resistance between AGMBR and MBR. Many studies have determined that the pore blocking resistance of AGMBR was lower than that of MBR [Citation11,Citation23,Citation27,Citation33]. The results of full – scale AGS membrane filtration for industrial treatment also showed that the pore blocking resistance for reactor 3 was 2.5 times larger than reactor 2 and 7.5 times larger than reactor 1 corresponding to small granules 70.4 μm, slow-settling granules 144.68 μm, and good-settling granules 158.62 μm in reactor 3, 2, 1, respectively [Citation11]. Flocculent sludge exhibits a more serious pore blocking, because of adherence of EPS and soluble microbial products (SMP), as main foulants, to the compact flocculent sludge fouling layer, enhancing the density degree of the cake layer and pore blocking. In contrast, during the AGS formation process, partial EPS and SMP are immobilized into AGS and are degraded by anaerobic bacteria in the granules, abating their composition in fouling layer and membrane pores; thus, its cake layer and pore blocking are smaller [Citation33]. In addition to the membrane foulants consumption of the granular sludge, the membrane fouling mitigation mechanism of AGS is also due to AGS scouring, AGS structure, hydraulic stress possessed a significant contribution rate 39.9%, 50.3%, 9.7% % for fouling mitigation, respectively [Citation33]. Meanwhile, some other studies estimated that the pore blocking resistance of AGMBR was higher and occupied a large ratio of total resistance [Citation31,Citation55]. However, the membrane filtration experiments to determine the resistance-in-series in these studies were conducted in a short time of just 3 h and 30 min, respectively, so the membrane blocking properties are different from those of long time filtration experiments in lab-scale model. Therefore, further studies need to be conducted to demonstrate the ability to reduce pore clogging of granules.
The fouling mechanism of the external AGMBR is different from that of the submerged AGMBR. For external AGMBR, filtration is applied to the effluent of the AGS reactor which has a lot of flocs, so the fouling mechanism is similar to that of the filtration in MBR, with the predominant cake layer clogging. Some studies confirmed that membrane fouling is mitigated and cake layer formation is the dominating fouling mechanism when using polyethersulfone ultrafiltration membrane to filtrate AGS effluent [Citation46] or using modified microbial flocculant to pre-coagulate the AGS effluent before dead-end filtration [Citation3]. The results also show that the total resistance of the effluent of the AGS reactor is smaller than that of the flocculent sludge due to EPS and SMP immobilization and degradation by granules, abating their composition in fouling layer and membrane pores [Citation33].
Indeed, aerobic granules have shown many benefits in reducing membrane fouling. The total and cake-layer resistances of the AGS membrane filtration were determined to be smaller than that of the flocculent sludge membrane filtration. However, up to now, the studies have mainly been carried out on the lab – scale for synthetic wastewater treatment, with the exception of AGS membrane filtration in a full-scale industrial plant, which also identified the total and pore resistance of aerobic granular sludge filtration is lower than that of flocculent sludge filtration [Citation11]. Therefore, further studies with larger scale for practical wastewater treatment need to be carried out to demonstrate the potential for reducing membrane fouling of AGS in practice.
Foulants in AGMBR
To better control fouling phenomena, the studies also estimated the main foulants in the AGMBR. It has been considered that microbial flocs, individual microbial cells, microbial metabolic products (EPS) and soluble microorganism products (SMPs), and non-degraded matter from wastewater are considered potential foulants. Among these, small flocs and macromolecules classified as SMP or EPS play a major role in membrane fouling [Citation14,Citation61]. This notion is supported theoretically since small particles tend to deposit on the membrane surface due to low back-transport velocity, increasing cake-layer resistance [Citation62]. In comparing fouling phenomena between MBR and AGMBR, Li, X [Citation13] showed that the main foulants are the flocs deposited on the membrane surface in MBR, but colloids and solutes adhered to the membrane pore in AGMBR. Most other studies have also provided the same conclusions [Citation21,Citation23,Citation27,Citation31,Citation55]. Estimating the fouling causes in AGMBR, most studies concluded that polysaccharides (PS) and proteins (PN) in mixed liquids play the main role in fouling. Sajad, M [Citation17] concluded that proteins, polysaccharides in SMP, fine particles, and surplus Mg2+ are the main foulants. Analysis of EPS in a fouled membrane, Zhou, J [Citation55] and Zhang, B [Citation59] showed that the main foulants are protein (PN) and polysaccharide (PS). Many authors have concluded that soluble polysaccharide (sPS) is the main foulant [Citation7,Citation24,Citation27,Citation29,Citation56,Citation63]. However, other authors have estimated sPN as the main foulant [Citation6,Citation26]. Although there is no agreement in estimating the causes of fouling, most studies have concluded that SMP is the main foulant, among which sPS plays the main role [Citation7,Citation35,Citation56,Citation57]. Besides, pollutants in wastewater also cause membrane fouling. Therefore, the higher the concentration of pollutants, the greater the risk of membrane fouling. AGS is capable of decomposing organic matter, nitrogen, phosphorus [Citation33] and even non-biodegradable substances [Citation64] as well as adsorbing heavy metals [Citation65] thereby reducing the risk of membrane clogging due to these components.
Currently, there is no high agreement on the causes of membrane fouling and main foulants. The mechanism of the membrane fouling phenomenon is not clear. The reason is that the studies were carried out under different experimental conditions of the compositions and properties of wastewater, the configuration of reactors, and the operation parameters and modes. It cannot interpolate biofiltration from one circumstance to the others because the different types of wastewater cause different membrane fouling phenomena. Up to now, the studies have mostly been carried out in synthetic wastewater, except for some studies in real wastewater, such as paper mill wastewater [Citation28], Ilsan wastewater [Citation29], and municipal wastewater [Citation6]…. Therefore, more work is needed to estimate the membrane fouling mechanism and main foulants for various types of wastewater.
Removal capacity of AEROBIC GRANULAR sludge in AGMBR
Aerobic granular sludge can withstand high organic and nitrogen loading rates of 2.5–10 kg COD/m3 day [Citation9,Citation66] and 0.5–2.5 kgN/m [Citation3] day [Citation67] with the removal efficiency of COD and total nitrogen (TN) more than 90% and about 60–70%, respectively. Nitrification and denitrification are simultaneous in the reactor due to the microbial multi-layer structure of aerobic granules. TN removal efficiency can be improved more than 90% by modifying operation conditions, such as the alternate anoxic and aerobic environment in the reactor; maintaining the low dissolved oxygen (DO); supply further carbon for heterotrophic bacteria of denitrification; anaerobic filling [Citation8,Citation68]. The total phosphorus (TP) removal efficiency of AGMBR may reach about 55–70% and even up to 90% by alternating anoxic and aerobic conditions in the reactor [Citation69–71]. The anoxic condition can be provided by filling without aeration or light mixing. Phosphorus is released in an anoxic filling phase, then utilized quickly during the aerobic phase, and removed through an excess sludge discharge [Citation72]. Really, AGS can remove COD, nitrogen, and phosphorus simultaneously even if aerobic condition is maintained in the reactor. Treatability depends on the structure and diameter of granules, concentration of DO, extra carbon supply, and the density of bacteria in granules.
The above advantages allow AGS to improve substrate removal efficiency in AGMBR. Most of the studies listed in show that the organic removal efficiency in AGMBR reached around 80–98% [Citation13,Citation14,Citation20,Citation23,Citation25,Citation27,Citation59]. Due to the oxygen mass transfer resistance in the compact layered structure of granules, nitrification and denitrification happened simultaneously and improved the removal efficiency of NH4-N and TN up to 90–99% and 50–70%, respectively [Citation14,Citation18,Citation22,Citation25,Citation27,Citation59]. The TN treatment efficiency can be improved to over 90% when the large granular sludge is stabilized in the reactor. The large granules facilitate the simultaneous nitrification and denitrification process and TN removal was further enhanced to 90.6% for the granule size of 2.5–2.8 mm [Citation46] and 80% for the granule size of 0.2–1.7 mm [Citation38].
Table 4. COD, nitrogen, and phosphorus removal efficiency of AGS in AGMBR.
The TP removal efficiency varied according to the phosphorus concentration in the influent. When the concentration of phosphorus in the influent was lower compared with the ratio COD:P = 100:1, the TP removal efficiency was about 90% [Citation32,Citation75]. That is, most of the studies were carried out in synthetic wastewater, and phosphorus concentration was just enough for the growth of bacteria. On the contrary, when the concentration of phosphorus in the influent was higher compared with the ratio COD:P = 100:1, the TP removal efficiency was only about 30–60% [Citation33,Citation74]. Some studies have shown that the TP removal efficiency fluctuated in the range of 30–70% [Citation38].
Advance studies for improving TN and TP removal efficiency were also carried out by alternating anoxic and aerobic conditions or controlling DO concentration in AGMBR. Setting the aeration/non-aeration phase in 100–240 min/60–90 min in granular sludge SBR for real mixed wastewater treatment, the removal efficiencies of TOC, TN, and TP were 92.1%, 76.1%, and 82.2%, respectively, with the DO of 3.2 mg/L and granular size of 1–3 mm [Citation30]. Operational mode with 60 min of anaerobic filling and the low concentration of TN in the influent enhanced TN removal efficiency up to 96–99% [Citation40]. Alternating anoxic and aerobic conditions in a cycle with 100 min anoxic and 240 min aerobic, TN removal efficiency reached up to 96% [Citation18]. The real wastewater treatment studies on pilot and full-scale also show high organic matter removal efficiency of over 90% [Citation10,Citation11] and TN treatment efficiency of about 60% [Citation10].
The results showed the simultaneous COD, TN, and TP removal capacity of AGS due to the bacterial multi-layer structure of the granules. However, studies on real wastewater and large-scale wastewater are limited. Therefore, this area needs more studies in the future on pilot and full scale for real wastewater treatment to verify the real removal efficiency of AGMBR and assess the influence of real wastewater properties on performance.
Conclusions and recommendation
Our collective body of research consistently indicates that Anaerobic Granular Sludge (AGS) demonstrates robust stability within Anaerobic Granular Sludge Membrane Bioreactor (AGMBR) systems. This stability translates to noteworthy reductions in membrane fouling, an increase in flux rates and enhanced efficiency in nitrogen and phosphorus removal. Consequently, AGMBR technology emerges as a promising contender for wastewater treatment applications, capitalizing on the amalgamation of AGS processes and membrane filtration.
In direct comparison with conventional Membrane Bioreactor (MBR) technology, AGMBR offers distinct economic advantages, underscored by its multifaceted benefits:
Mitigation of membrane fouling results in elevated membrane filtration efficiency and reduced cleaning cycles, thereby curtailing operational expenditures and extending membrane lifespan.
Simultaneous treatment of COD, nitrogen, and phosphorus, even under high organic loading rates. This holistic approach minimizes spatial requirements owing to diminished reactor volume necessities. Furthermore, the omission of secondary sedimentation, disinfection, and advanced nitrogen and phosphorus removal processes contributes to space and cost savings.
Demonstrable high removal efficiency, positioning AGMBR as a viable option for wastewater reclamation and reuse.
Nonetheless, despite its promise, AGMBR remains underutilized and gaps within the existing body of research persist. To pave the way for practical application and further refinement of this technology, addressing the following limitations is imperative:
The size and structural regularity of aerobic granules within AGMBR require thorough investigation. The prevailing maintenance of small-diameter granules (less than 1 mm) with irregular structures prompts the need to identify optimal operational conditions that sustain larger, more uniform, and compact granular sludge.
While AGMBR exhibits notable filterability, instances of pore fouling dominate. A comprehensive understanding of pore fouling, particularly in comparison to MBRs, necessitates dedicated exploration, offering potential insights into this intricate aspect of fouling dynamics.
Presently, research predominantly relies on synthetic wastewater conditions. To ascertain the true membrane fouling mechanisms and performance of AGMBR technology on a practical scale, rigorous examination in pilot and full-scale real wastewater treatment scenarios is indispensable.
Given the prevailing nature of continuous operation in practical biological reactors, the applicability of AGS in continuous reactors requires further inquiry. This involves determining stable operational conditions for AGS in continuous modes or devising novel operational strategies for new batch-based reactors.
While AGMBR technology holds immense potential, its effective deployment hinges upon addressing the outlined research gaps. With concerted efforts to refine its operational parameters, understand fouling dynamics, and validate its performance under real-world conditions, AGMBR can emerge as a transformative tool in advancing sustainable wastewater treatment practices.
Highlights
A systematic review of AGMBR was conducted
The formation and stabilization of aerobic granules in AGMBR
Membrane fouling phenomena and mechanism during AGMBR process
Removal capacity of aerobic granular sludge occurred in AGMBR
Author contributions
Hong Truong Thi Bich: writing, review and editing, analysis, and interpretation of the data. Ha Manh Bui: Conceptualization, methodology, resources, data curation, supervision. All authors agreed to be accountable for all aspects of the work.
Disclosure statement
No potential conflict of interest was reported by the author(s).
Data availability statement
The authors confirm that the data supporting the findings of this study are available within the article [and/or] its supplementary materials.
Additional information
Funding
References
- Rahman TU, Roy H, Islam MR, et al. The advancement in membrane bioreactor (MBR) technology toward sustainable industrial wastewater management. Membranes (Basel). 2023;13(2):181. doi: 10.3390/membranes13020181
- Park H-D, Chang I-S, Lee K-J. Principles of membrane bioreactors for wastewater treatment. CRC Press; 2015.
- Zhang B, Mao X, Tang X, et al. Pre-coagulation for membrane fouling mitigation in an aerobic granular sludge membrane bioreactor: a comparative study of modified microbial and organic flocculants. J Membr Sci. 2022;644:120129. doi: 10.1016/j.memsci.2021.120129.
- Nguyen T-T, Chiemchaisri C, Bui X-T, et al. Membrane bioreactor processes. In: Bui X-T, Nguyen DD, Nguyen P-D, Ngo HH, Pandey A, editors. Current developments in biotechnology and bioengineering advances in biological wastewater treatment system. Vol. 1, Netherlands: Elsevier; 2022. p. 155–17. doi:10.1016/B978-0-323-99874-1.00026-9.
- Morgenroth E, Sherden T, Van Loosdrecht M, et al. Aerobic granular sludge in a sequencing batch reactor. Water Res. 1997;31(12):3191–3194. doi: 10.1016/S0043-1354(97)00216-9
- Li J, Liu Y, Li X, et al. Reactor performance and membrane fouling of a novel submerged aerobic granular sludge membrane bioreactor during long-term operation. J Water Reuse Desalin. 2017;8(1):1–9. doi: 10.2166/wrd.2017.019
- Hong T, Phuong T, Ha M. Integration of aerobic granular sludge and membrane filtration for tapioca processing wastewater treatment: fouling mechanism and granular stability. J Water Supp. 2018;67(8):846–857. doi: 10.2166/aqua.2018.104
- De Kreuk M, Pronk M, Van Loosdrecht M. Formation of aerobic granules and conversion processes in an aerobic granular sludge reactor at moderate and low temperatures. Water Res. 2005;39(18):4476–4484. doi: 10.1016/j.watres.2005.08.031
- Moy BP, Tay JH, Toh SK, et al. High organic loading influences the physical characteristics of aerobic sludge granules. Lett Appl Microbiol. 2002;34(6):407–412. doi: 10.1046/j.1472-765X.2002.01108.x
- Guo T, Ji Y, Zhao J, et al. Coupling of fe-C and aerobic granular sludge to treat refractory wastewater from a membrane manufacturer in a pilot-scale system. Water Res. 2020;186:116–331. doi: 10.1016/j.watres.2020.116331.
- Tsertou E, Caluwe M, Goossens K, et al. Performance of an aerobic granular sludge membrane filtration in a full-scale industrial plant. Water Sci Technol. 2023;87(12):76–98. doi: 10.2166/wst.2023.176
- Ali M, Singh Y, Fortunato L, et al. Performance evaluation of a pilot-scale aerobic granular sludge integrated with gravity-driven membrane system treating domestic wastewater. ACS ES&T Water. 2023;3(8):78–90. doi: 10.1021/acsestwater.3c00178
- Li X, Gao F, Hua Z, et al. Treatment of synthetic wastewater by a novel MBR with granular sludge developed for controlling membrane fouling. Sep Purif Technol. 2005;46(1–2):19–25. doi: 10.1016/j.seppur.2005.04.003
- Jang A, Jang N, Ren X, et al. Performance enhancement of MBR operated with aerobic granules on membrane filterability improvement. Desalin Water Treat. 2012;43(1–3):323–331. doi: 10.1080/19443994.2012.672222
- Li X, Li Y, Liu H, et al. Characteristics of aerobic biogranules from membrane bioreactor system. J Membr Sci. 2007;287(2):294–299. doi: 10.1016/j.memsci.2006.11.005
- Li X, Li Y-J, Liu H, et al. Correlation between extracellular polymeric substances and aerobic biogranulation in membrane bioreactor. Sep Purif Technol. 2008;59(1):26–33. doi: 10.1016/j.seppur.2007.05.024
- Sajjad M, Kim KS. Influence of Mg2+ catalyzed granular sludge on flux sustainability in a sequencing batch membrane bioreactor system. Chem Eng J. 2015;281:404–410. doi: 10.1016/j.cej.2015.06.112.
- Tu X, Zhang S, Xu L, et al. Performance and fouling characteristics in a membrane sequence batch reactor (MSBR) system coupled with aerobic granular sludge. Desalination. 2010;261(1–2):191–196. doi: 10.1016/j.desal.2010.03.034
- Vijayalayan P, Thanh BX, Visvanathan C. Simultaneous nitrification denitrification in a batch granulation membrane airlift bioreactor. Int Biodeterior Biodegrad. 2014;95:139–143. doi: 10.1016/j.ibiod.2014.05.020.
- Juang Y-C, Adav S, Lee D-J, et al. Biodiversity in aerobic granule membrane bioreactor at high organic loading rates. Appl Microbiol Biotechnol. 2009;85(2):383–388. doi: 10.1007/s00253-009-2227-x
- Juang Y-C, Lee D-J, Lai J-Y. Fouling layer on hollow-fibre membrane in aerobic granule membrane bioreactor. J Chin Inst Chem Eng. 2008;39(6):657–661. doi: 10.1016/j.jtice.2008.08.003
- Li WW, Wang Y-K, Sheng G-P, et al. Integration of aerobic granular sludge and mesh filter membrane bioreactor for cost-effective wastewater treatment. Biores Technol. 2012;122:22–26. doi: 10.1016/j.biortech.2012.02.018
- Tay JH, Yang P, Zhuang WQ, et al. Reactor performance and membrane filtration in aerobic granular sludge membrane bioreactor. J Membr Sci. 2007;304(1–2):24–32. doi: 10.1016/j.memsci.2007.05.028
- Wang JF, Qiu ZG, Chen ZQ, et al. Comparison and analysis of membrane fouling between flocculent sludge membrane bioreactor and granular sludge membrane bioreactor. PLoS One. 2012;7:08–19. doi: 10.1371/journal.pone.0040819
- Wang JF, Wang X, Zhao Z, et al. Organics and nitrogen removal and sludge stability in aerobic granular sludge membrane bioreactor. Appl Microbiol Biotechnol. 2008;79(4):679–685. doi: 10.1007/s00253-008-1466-6
- Wang X, Zhang B, Shen Z, et al. The EPS characteristics of sludge in an aerobic granule membrane bioreactor. Biores Technol. 2010;101(21):8046–8050. doi: 10.1016/j.biortech.2010.05.074
- Liu Y-J, Sun DD. Comparison of membrane fouling in dead-end microfiltration of denitrifying granular sludge suspension and its supernatant. J Membr Sci. 2010;352(1–2):100–106. doi: 10.1016/j.memsci.2010.02.005
- Morais ILH, Silva CM, Borges CP Aerobic granular sludge to treat paper mill effluent: organic matter removal and sludge filterability. Desalination and Water Treatment 2015:15–36.
- Sajjad M, Kim IS, Kim KS. Development of a novel process to mitigate membrane fouling in a continuous sludge system by seeding aerobic granules at pilot plant. J Membr Sci. 2016;497:90–98. doi: 10.1016/j.memsci.2015.09.021.
- Lee H, Hyun K. Effect of sequencing batch reactor (Sbr)/granular activated carbon (GAC) bed and membrane hybrid system for simultaneous water reuse and membrane fouling mitigation. Environ Eng Res. 2021;26(1):223–230. doi: 10.4491/eer.2019.500
- Wang Y, Zhong C, Huang D, et al. The membrane fouling characteristics of MBRs with different aerobic granular sludges at high flux. Biores Technol. 2013;136:488–495. doi: 10.1016/j.biortech.2013.03.066
- Wang X-C, Shen J-M, Chen Z-L, et al. Removal of pharmaceuticals from synthetic wastewater in an aerobic granular sludge membrane bioreactor and determination of the bioreactor microbial diversity. Appl Microbiol Biotechnol. 2016;100:8213–8223. doi: 10.1007/s00253-016-7577-6.
- Zhang W, Liang W, Zhang Z, et al. Aerobic granular sludge (AGS) scouring to mitigate membrane fouling: performance, hydrodynamic mechanism and contribution quantification model. Water Res. 2021;188:116–518. doi: 10.1016/j.watres.2020.116518.
- An Z, Zhang X, Bott CB, et al. Long-term stability of nitrifying granules in a membrane bioreactor without hydraulic selection pressure. Processes. 2021;9(6):1024. doi: 10.3390/pr9061024
- Wang Y, Chen Q, Lin Y, et al. Performance enhancement of a submerged forward osmotic membrane bioreactor by aerobic granules. Sep Purif Technol. 2021;254:117–608. doi: 10.1016/j.seppur.2020.117608
- Yang Z, Bin L, Huang S, et al. Revealing the stability of aerobic granular sludge in a membrane bioreactor under different DO values by proteomics analysis. Bioresour Technol Rep. 2021;14:100673. doi: 10.1016/j.biteb.2021.100673
- Xiao X, Ma F, You S, et al. Direct sludge granulation by applying mycelial pellets in continuous-flow aerobic membrane bioreactor: performance, granulation process and mechanism. Biores Technol. 2022;344:126233. doi: 10.1016/j.biortech.2021.126233
- Liu Q, Wu C, Bin L, et al. Distribution characteristics of phosphorus-containing substances in a long running aerobic granular sludge-membrane bioreactor with no sludge discharge. Biores Technol. 2022;347:126694. doi: 10.1016/j.biortech.2022.126694
- Qiu B, Liao G, Wu C, et al. Rapid granulation of aerobic granular sludge and maintaining its stability by combining the effects of multi-ionic matrix and bio-carrier in a continuous-flow membrane bioreactor. Sci Total Environ. 2022;813:152644. doi: 10.1016/j.scitotenv.2021.152644
- Iorhemen OT, Hamza RA, Zaghloul MS, et al. Simultaneous organics and nutrients removal in side-stream aerobic granular sludge membrane bioreactor (AGMBR). Water Proc Eng. 2018;21:127–132. doi: 10.1016/j.jwpe.2017.12.009.
- Liu Y, Tay JH. The essential role of hydrodynamic shear force in the formation of biofilm and granular sludge. Water Res. 2002;36(7):1653–1665. doi: 10.1016/S0043-1354(01)00379-7
- Liu YQ, Tay JH. Fast formation of aerobic granules by combining strong hydraulic selection pressure with overstressed organic loading rate. Water Res. 2015;80:256–266. doi: 10.1016/j.watres.2015.05.015.
- Zheng YM, Yu HQ, Liu SJ, et al. Formation and instability of aerobic granules under high organic loading conditions. Chemosphere. 2006;63(10):1791–1800. doi: 10.1016/j.chemosphere.2005.08.055
- Zhu L, Zhou J, Yu H, et al. Optimization of hydraulic shear parameters and reactor configuration in the aerobic granular sludge process. Environ Technol. 2015;36(13):1605–1611. doi: 10.1080/09593330.2014.998717
- Tay J-H, Liu Q-S, Liu Y. The effects of shear force on the formation, structure and metabolism of aerobic granules. Appl Microbiol Biotechnol. 2001;57(1–2):227–233. doi: 10.1007/s002530100766
- Yang Z, Tran QN, Jin X. Ultrafiltration of aerobic granular sludge bioreactor effluent: fouling potentials and properties. Water Proc Eng. 2022;47:102805. doi: 10.1016/j.jwpe.2022.102805.
- Beun J, Hendriks A, Van Loosdrecht M, et al. Aerobic granulation in a sequencing batch reactor. Water Res. 1999;33(10):2283–2290. doi: 10.1016/S0043-1354(98)00463-1
- Beun J, Van Loosdrecht M, Heijnen J. Aerobic granulation in a sequencing batch airlift reactor. Water Res. 2002;36(3):702–712. doi: 10.1016/S0043-1354(01)00250-0
- Mosquera-Corral A, De Kreuk M, Heijnen J, et al. Effects of oxygen concentration on N-removal in an aerobic granular sludge reactor. Water Res. 2005;39(12):2676–2686. doi: 10.1016/j.watres.2005.04.065
- Tay J, Liu Q, Liu Y. Characteristics of aerobic granules grown on glucose and acetate in sequential aerobic sludge blanket reactors. Environ Technol. 2002;23(8):931–936. doi: 10.1080/09593332308618363
- Wang SG, Liu XW, Gong WX, et al. Aerobic granulation with brewery wastewater in a sequencing batch reactor. Biores Technol. 2007;98(11):2142–2147. doi: 10.1016/j.biortech.2006.08.018
- Nguyen PTT, Nguyen PV, Truong HTB, et al. The formation and stabilization of aerobic granular sludge in a sequencing batch airlift reactor for treating tapioca-processing wastewater. Polish J Environ Stud. 2016;25(5):1–10. doi: 10.15244/pjoes/62736
- Weber S, Ludwig W, Schleifer K-H, et al. Microbial composition and structure of aerobic granular sewage biofilms. Appl environ microbiol. 2007;73(19):6233–6240. doi: 10.1128/AEM.01002-07
- Yang S-F, Tay J-H, Liu Y. Inhibition of free ammonia to the formation of aerobic granules. Biochem Eng J. 2004;17(1):41–48. doi: 10.1016/S1369-703X(03)00122-0
- Zhou J, Yang F-L, Meng F-G, et al. Comparison of membrane fouling during short-term filtration of aerobic granular sludge and activated sludge. J Environ Sci. 2007;19(11):1281–1286. doi: 10.1016/S1001-0742(07)60209-5
- Wang Y, Li J, Zhu J. Comparative analysis of membrane fouling mechanisms induced by operation modes of membrane bioreactors with aerobic granular sludge. Heliyon. 2023;9(7):e17973. doi: 10.1016/j.heliyon.2023.e17973
- Thanh BX. Fouling behavior and nitrogen removal in the aerobic granulation membrane bioreactor. Thailand: Asian Institute of Technology; 2009. p. 141.
- Thanh BX, Visvanathan C, Aim RB. Fouling characterization and nitrogen removal in a batch granulation membrane bioreactor. Int Biodeterior Biodegrad. 2013;85:491–498. doi: 10.1016/j.ibiod.2013.02.005.
- Zhang B, Huang D, Shen Y, et al. Treatment of municipal wastewater with aerobic granular sludge membrane bioreactor (AGMBR): performance and membrane fouling. J Clean Prod. 2020;273:123124. doi: 10.1016/j.jclepro.2020.123124.
- Liébana R, Modin O, Persson F, et al. Integration of aerobic granular sludge and membrane bioreactors for wastewater treatment. Crit Rev Biotechnol. 2018;38(6):1–16. doi: 10.1080/07388551.2017.1414140
- Wu B, Yi S, Fane AG. Microbial behaviors involved in cake fouling in membrane bioreactors under different solids retention times. Biores Technol. 2011;102(3):2511–2516. doi: 10.1016/j.biortech.2010.11.045
- Yoon S-H. Membrane bioreactor processes: principles and applications. 1st ed. Boca Raton: CRC Press; 2015. p. 452. doi:10.1201/b18631.
- Thanh BX, Visvanathan C, Spérandio M, et al. Fouling characterization in aerobic granulation coupled baffled membrane separation unit. J Membr Sci. 2008;318(1–2):334–339. doi: 10.1016/j.memsci.2008.02.058
- Yi S, Zhuang W-Q, Wu B, et al. Biodegradation of p-nitrophenol by aerobic granules in a sequencing batch reactor. Environ Sci Technol. 2006;40(7):2396–2401. doi: 10.1021/es0517771
- Ahn KH, Hong SW. Characteristics of the adsorbed heavy metals onto aerobic granules: isotherms and distributions. Desalin Water Treat. 2015;53(9):2388–2402. doi: 10.1080/19443994.2014.927125
- Truong HTB, Van Nguyen P, Nguyen PTT, et al. Treatment of tapioca processing wastewater in a sequencing batch reactor: mechanism of granule formation and performance. J Environ Manage. 2018;218:39–49. doi: 10.1016/j.jenvman.2018.04.041
- Morales N, Figueroa M, Fra-Vázquez A, et al. Operation of an aerobic granular pilot scale SBR plant to treat swine slurry. Process Biochem. 2013;48(8):1216–1221. doi: 10.1016/j.procbio.2013.06.004
- Chen FY, Liu YQ, Tay JH, et al. Operational strategies for nitrogen removal in granular sequencing batch reactor. J Hazard Mater. 2011;189(1–2):342–348. doi: 10.1016/j.jhazmat.2011.02.041
- Long B, Xuan X, Yang C, et al. Stability of aerobic granular sludge in a pilot scale sequencing batch reactor enhanced by granular particle size control. Chemosphere. 2019;225:460–469. doi: 10.1016/j.chemosphere.2019.03.048.
- Cheng W, Ma C, Bao R, Yang X, Zhou X, Liu Y, et al. Effect of influent ammonia nitrogen concentration on the phosphorus removal process in the aerobic granular sludge reactor. J Environ Chem Eng. 2023;2023(5):110–476. doi: 10.1016/j.jece.2023.110476
- Cheng L, Wei M, Hu Q, et al. Aerobic granular sludge formation and stability in enhanced biological phosphorus removal system under antibiotics pressure: performance, granulation mechanism, and microbial successions. J Hazard Mater. 2023;454:131–472. doi: 10.1016/j.jhazmat.2023.131472
- Zhang H-L, Fang W, Wang Y-P, et al. Phosphorus removal in an enhanced biological phosphorus removal process: roles of extracellular polymeric substances. Environ Sci Technol. 2013;47(20):11482–11489. doi: 10.1021/es403227p
- Rui C Ammonium nitrogen wastewater treatment by aerobic granular sludge membrane bioreactor. Bioinformatics and Biomedical Engineering, 2008 ICBBE 2008 The 2nd International Conference; Shanghai, China. IEEE; 2008. p. 3469–3471.
- Liu H, Li Y, Yang C, et al. Stable aerobic granules in continuous-flow bioreactor with self-forming dynamic membrane. Biores Technol. 2012;121:111–118. doi: 10.1016/j.biortech.2012.07.016
- Zhao X, Chen Z-L, Wang X-C, et al. Ppcps removal by aerobic granular sludge membrane bioreactor. Appl Microbiol Biotechnol. 2014;98:9843–9848. doi: 10.1007/s00253-014-5923-0.