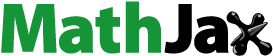
ABSTRACT
Reducing the preparation cost of alumina ceramics has received significant attention, as alumina is one of the most widely used structural ceramics. In this study, corundum-based composite ceramics with a white corundum-to-zircon powder mass ratio of 7:3 were selected as the matrix. CeO2 and Y2O3 doped corundum-based composite ceramics were prepared via pressureless sintering. The total content of CeO2-Y2O3 was set at 10 wt% to study the effects of different temperatures and CeO2-Y2O3 ratios on the properties of the corundum-based composite ceramics. Physical phase and microstructural analyses of the corundum-based composite ceramics were performed using X-ray diffraction and scanning electron microscopy, respectively. The results showed that the sintering temperature of the corundum-based composite ceramics could be effectively reduced by using a solid solution of CeO2 and Y2O3. The best sintering temperature was 1400°C, and the best composition was 5 wt% CeO2-5 wt% Y2O3. The physical properties of the corundum-based composite ceramics prepared under these technological conditions include a flexural strength of 386.2 MPa, hardness of 13.5 GPa, density of 3.9128 g/cm3, linear shrinkage of 16.31% and an apparent porosity of 0.62%.
1. Introduction
Corundum ceramics have a wide variety of potential applications in wear-resistant materials, refractory materials, and mechanical processing owing to their high melting points, high hardness, good wear resistance, good thermal shock resistance, and high physical and chemical stability [Citation1,Citation2]. The ionic and covalent properties of the Al-O bonds in alumina make it one of the most versatile refractory ceramic oxides. However, it is prone to anisotropy and abnormal grain growth during a long sintering process due to its high sintering temperature (above 1700°C) and low diffusion coefficient. In addition, alumina ceramics have reduced densities due to the presence of residual porosity, which is inevitable [Citation3–5]. Both these drawbacks illustrate that preparing low-cost, high-density alumina ceramics for commercial use in a simple and reliable manner remains a great challenge. Many researchers have studied the sintering behavior and microstructure of alumina ceramics in detail by adding sintering aids or by using various sintering techniques, such as hot-pressure [Citation6], spark plasma [Citation7], oscillatory pressure [Citation8], and microwave sintering [Citation9]. Currently, the addition of sintering aids is the most effective method for decreasing the sintering temperature and increasing the density of ceramics. The temperature required to sinter alumina ceramics has been minimized by adding oxide sintering aids, which aids in forming a liquid phase. The oxides that promoted the formation of a liquid phase include CaO, MgO, SiO2, B2O3, TiO2, CuO, MnO2, Y2O3, La2O3, and Nb2O5 [Citation10–19]. These oxides, alone or in combination, can form a glass-liquid phase, low-eutectic-point liquid phase, or lattice defects within the solid solution matrix; these are conducive to atomic diffusion, which accelerates the sintering process of alumina ceramics and is significant for sintering. After adding sintering additives, the sintering temperature of alumina ceramics was reduced to 1400°C-1450°C; however, the cooling effect was not significant [Citation20–24]. The addition of sintering aids also promotes the formation of anisotropic grains with a large width-to-diameter ratio, which significantly affects the final microstructure of alumina ceramics [Citation25].
Zirconium silicate has a high dielectric constant (~15), strong corrosion resistance, high melting point (>1670°C), low thermal conductivity and expansion, and excellent chemical and phase stability. Zircon powder (ZrSiO4) is generally used in refractory coatings; however, owing to a lack of resources, it has recently been compounded with white corundum to produce coatings for large castings. ZrSiO4 decomposes into ZrO2 and SiO2 at high temperatures. While ZrO2 can toughen Al2O3-based ceramics, SiO2 can be used as a sintering aid for Al2O3. However, Y2O3 can be used as both a sintering aid for Al2O3 and a ZrO2 stabilizer to prevent a phase change [Citation26–35]. Therefore, in this study, corundum-based composite ceramics were prepared by atmospheric pressure sintering method using white corundum and zircon powder as raw materials and CeO2-Y2O3 binary system as sintering aid. The effects of CeO2-Y2O3 sintering aids on the mechanical and sintering properties of corundum-based composite ceramics were investigated and the optimal CeO2-Y2O3 ratio and sintering temperature were determined.
2. Experimental procedure
In this study, α-Al2O3 analytical grade powder (purity, 99.9%; particle size 5 μm; Shanghai Yunfu Nano Technology Co., Ltd., Shanghai, China), zircon powder (particle size 20 μm; composition, ZrO2 >65 wt%, SiO2 ≤33 wt%, TiO2 <0.3 wt%, Al2O3 <1.8 wt%, P2O3 ≤0.2 wt%, Fe2O3 <0.2 wt%; Henan Yixiang Materials, Henan, China), Y2O3, and CeO2 (purity, 99.0%; particle size 5 μm; Shanghai Maclin Biochemical Technology Co., Ltd., Shanghai, China)were used. Based on the results from preliminary tests, alumina, and zircon powder were mixed in a 7:3 ratio to form a composite corundum. The total content of the sintering aids (CeO2 and Y2O3) was set to 10 wt%. The influence of the sintering aids on the sample properties was studied by changing the mass ratios of CeO2 and Y2O3, as shown in .
Table 1. The formula compositions of samples (wt%).
The raw materials were weighed proportionally and placed in a polytetrafluoroethylene ball-milling tank. Al2O3 balls, polyvinyl alcohol (5 wt%), and ethanol were selected as the grinding balls, binder, and ball-milling medium, respectively. The ball milling time was 4 h, and the ball-to-powder ratio was 2:1. The prepared slurry was dried in an oven at 60°C for 12 h and then passed through a 100-mesh screen. The sieved powder (25 g) was placed in a steel mold (45 mm diameter) and pressed under a uniaxial pressure of 20 MPa for 10 min to obtain a green body (φ45 × H7 mm). Subsequently, using a pressureless sintering technique, the raw billets were heated in a Muffle furnace at 2°C/min to 600°C and held for 1 h to burn out the organic additives. The temperature was then increased to the target temperature and maintained for 2 h, and the sintering temperatures were 1300°C, 1350°C, 1400°C, 1450°C, and 1500°C. Finally, the temperatures were cooled to room temperature.
The bulk density and apparent porosity of the samples were determined using Archimedes’ method. The flexural strengths of the corundum-based composite ceramics were measured using an electronic universal testing machine (CMT5105; Shenzhen Xinsanji Material Testing Co., Ltd.). The samples were processed into standard specimens measuring 4 mm × 3 mm × 36 mm by diamond wire cutting, their surfaces were polished and their four corners were chamfered with sandpaper (to a depth of approximately 0.3 mm). The samples were placed on a beam with a span of 30 mm and a loading rate was 0.5 mm/min. The hardness of the samples was measured using a Wechsler hardness tester. A diamond tetragonal cone indenter with an angle of 136° on both sides was used to apply pressure to the samples, which were held at a pressure of 1 kg for 15 s. X-ray diffraction (XRD, Japan Science Smartlab SE) was used to analyze the physical phase compositions. The microstructures of the samples were analyzed using scanning electron microscopy (SEM, Zeiss GeminiSEM360 UK) and X-ray energy-dispersive spectroscopy (EDS).
3. Results and discussions
3.1. Phase composition
shows the phase compositions of the corundum-based composite ceramics with a CeO2 content of 5 wt% sintered at different temperatures for 2 h. At sintering temperatures of 1300°C and 1350°C, in addition to the main phases Al2O3 and ZrSiO4, small amounts of mullite, t-ZrO2, Zr0.9Ce0.1O2, and Zr0.8Y0.2O1.9 solid solution phases were produced in corundum-based composite ceramics. After the sintering temperature reached 1400°C, the peak of ZrSiO4 completely disappeared from the diagram and a large number of t-ZrO2, mullite, Zr0.9Ce0.1O2, and Zr0.8Y0.2O1.9 solid solution phases appeared. It was shown that ZrSiO4 decomposed completely at 1400°C, decomposing into SiO2 and ZrO2. At temperatures higher than 1400°C, ZrO2 existed mainly in the crystalline form of the tetragonal phase. The formation of t-ZrO2 could improve the mechanical properties of composites. SiO2 with Al2O3 produced mullite and the added CeO2 and Y2O3 sinter aids were solidly dissolved in ZrO2 to form the Zr0.9Ce0.1O2 and Zr0.8Y0.2O1.9 solid solution phases. Although the actual decomposition temperature of ZrSiO4 was 1550°C, the introduction of the CeO2-Y2O3 system as a sintering aid led to a lower decomposition temperature. The reactions involved in the sintering process could be expressed by the following:
shows the phase composition of the corundum-based composite ceramics with different sintering aids sintered for 2 h at 1400°C. As shown in , the primary crystalline phases in the corundum-based composite ceramics were Al2O3,t-ZrO2, mullite, Zr0.9Ce0.1O2, and Zr0.8Y0.2O1.9. However, no CeO2 or Y2O3 phases were observed in the XRD pattern, indicating that CeO2 and Y2O3 were completely dissolved into the composite corundum. As shown in , the increase in CeO2 content gradually shifted the 2θ value of the (110) diffraction peak to a smaller angle; using the Bragg equation, the lattice constant of Al2O3 increased. The Bragg equation is expressed as follows:
CeO2 has the fluorite structure in the cubic crystal system. Because the diameter of Ce4+ is larger than that of O2-, the face-centered cubic lattice is assumed to be composed of Ce4+, which occupy 1/2 of the octahedral voids, while O2- occupies all four tetrahedral voids. Owing to the difference in the ionic radii and valence states, Ce4+ and Y3+ must replace Al3+ to form cationic vacancies to maintain the neutrality of the corundum-based composite ceramics. The decrease in the binding energy near the vacancy results in an increase in the lattice constant and alumin lattice distortion. This lattice activation facilitates atomic diffusion and promotes the densification of ceramics. The reaction of point defects can be expressed as follows:
where Ce and Y represent the positions at which Ce4+ and Y3+ replace Al3+, respectively (the upper-right mark indicates the charge remaining after substitution), and V represents the Al3+ vacancy. According to Eq. (5), the equilibrium constant K of the Frankel defect reaction can be calculated as follows:
From the above equations, the diffusion coefficient is determined to be proportional to the vacancy concentration(V), which is proportional to the 3/4 power of the CeO2 concentration. As the sintering temperature increases, the diffusion coefficient increases, promoting a nonlinear increase in the sintering rate. This is an external manifestation of the internal mechanism of solid-phase reaction sintering.
3.2. Physical properties
shows the physical properties of the corundum-based composite ceramics with a CeO2 content of 5 wt% sintered at different temperatures for 2 h. From , a sintering temperature of 1300°C yields the smallest density and linear shrinkage and the highest apparent porosity. Subsequently, the density, linear shrinkage, and apparent porosity of the samples changed slightly with increasing temperature. When the sintering temperature reached 1400°C, the density and linear shrinkage of the sample reached their maximum values, and the apparent porosity reached its minimum. From , the flexural strength and hardness of the corundum-based composite ceramics first increased sharply and then had small fluctuations as the sintering temperature increased, reaching their maximum values at 1400°C. Combined with the XRD pattern in , the sample was not completely sintered at 1300°C. At temperatures above 1300°C, the activation energy required for the sintering of corundum-based composite ceramics was reached and the samples began to densify and increase in strength. This indicates that the introduction of the CeO2-Y2O3 system into the corundum-based composite ceramics promoted the formation of Zr0.9Ce0.1O2 and Zr0.8Y0.2O1.9 solid solutions, which further reduced the sintering temperature of the samples.
shows the physical properties of the corundum-based composite ceramics with different sintering aids sintered at 1400°C for 2 h. From , the density and linear shrinkage of the samples tended to increase, decrease, and then increase with increasing CeO2 concentration, whereas that of the pore size behaved in the opposite manner. When the CeO2 content reached 5 wt%, the density and linear shrinkage of the samples were at their largest, and the apparent porosity was at this smallest. However, when the CeO2 content was 6 wt%, a sudden change occurred. As shown in , the flexural strength and hardness of the corundum-based composite ceramics first increased and then decreased with increasing CeO2 content. The flexural strength and hardness of the sample reached their maximum values when the CeO2 content reached 5 wt%. This is because when the CeO2 content was less than 5 wt%, Y2O3 played an important role. On the one hand, Y2O3 can stabilize ZrO2 and prevent phase change, and on the other hand, it can refine the grains and at the same time increase the amount of liquid phase and filling the interstices of the grains, thus increasing the density of the corundum ceramics, and the strength increases. CeO2 and Y2O3 act together when the CeO2 content was 5 wt%, at this time density, porosity, line shrinkage and mechanical properties were highest. However, once the CeO2 content exceeded 5 wt%, the individual grain size began to increase and the increased interstitial space had a negative effect on both densification and strength. As CeO2 continued to increase, it could be seen in conjunction with the SEM in that the grains began to grow as a whole and the proportion of transgranular fracture increased, so the strength would increase again.
3.3. Microstructure
shows the SEM images of sections of the corundum-based composite ceramics with a CeO2 content of 5 wt% sintered for 2 h at different temperatures. Because the sample was not fully sintered at 1300°C (), the structure was loose, resulting in charge accumulation and fuzzy imaging. As the temperature increased, the corundum-based composite ceramics began to sinter, the grains gradually grew, the pores were filled and the structure became denser. shows that the porosity has been significantly reduced. When the temperature reached 1400°C, a large number of dimples appear in corundum-based composite ceramics. The crystal broke mainly by transgranular fracture and grain stripping, which is also the main reason for the increase in strength. However, when the temperature exceeded 1400, small particles tended to dissolve in the matrix while large particles tended to grow. Excessive grain growth would lead to abnormal grain growth and its fracture would be dominated by along-crystal fracture, leading to a reduction in strength.
Figure 5. SEM of the sample containing 5 wt % CeO2 sintered at 1300°C-1500°C for 2 h: (a) 1300°C; (b) 1350°C; (c) 1400°C; (d) 1450°C; (e) 1500°C.

shows the SEM images of cross-sections of corundum-based composite ceramics with different sintering aids sintered at 1400°C for 2 h. When the CeO2 content increased to 3 wt%, the percentage of grain pullout gradually decreased, and the percentage of abnormal grains started to increase (), indicating that Y2O3 can inhibit abnormal grain growth. This CeO2 concentration corresponded to the beginning of decreasing intensity, as shown in . When the CeO2 content was increased to 5 wt%, the grains started to decrease in size and the percentage of grain pullout increased (), indicating that CeO2 started to play a role. As the CeO2 content continued to increase, CeO2 dominated and the proportion of transgranular fractures began to decrease (). This is also the main reason for the consequent increase in intensity ().
Figure 6. SEM of different samples after sintering at 1400°C for 2 h: (a) 1 wt% CeO2; (b) 2 wt% CeO2; (c) 3 wt% CeO2; (d) 4 wt% CeO23; (e) 5 wt% CeO2; (f) 6 wt% CeO2; (g) 7 wt% CeO2; (h) 8 wt% CeO2; (i) 9 wt% CeO2.

shows the EDS diagram of a section of corundum-based composite ceramics with the section of the 5 wt% CeO2-5 wt% Y2O3 sintered at 1400°C for 2 h. As can be seen from the figure, SiO2 combined with part of Al2O3 to produce a small amount of mullite, and there is still part of ZrSiO4 not completely decomposed. Y2O3, CeO2 combined with ZrO2, and according to the XRD pattern, it can be analyzed that most of Y2O3 and CeO2 were soluted into ZrO2, forming a solid solution and reducing the sintering temperature.
4. Conclusion
Corundum-based composite ceramics doped with 10 wt% CeO2 and Y2O3 were prepared. The effects of different temperatures and ratio of CeO2-Y2O3 on the sintering properties, physical properties, and microstructure were investigated. The main conclusions are as follows:
Corundum-based composite ceramics were prepared using a 5 wt% CeO2-5 wt% Y2O3 binary sintering aid at sintering temperatures of 1300°C-1500°C. A combination of XRD, SEM, and physical property analyses shows that the samples were not completely sintered at 1300°C. With an increase in temperature, the sample began to sinter, the grains grew gradually, and its mechanical performance initially increased and then decreased. When the temperature reached 1400°C, the performance was at its best. Binary CeO2-Y2O3 sintering aids effectively reduced the sintering temperature.
The effects of different ratios of CeO2-Y2O3 on the properties of corundum-based composite ceramics were studied at 1400°C. With increasing CeO2 content, the grains’ fracture mode was mainly transgranular pulling out. The best performance was obtained with the 5 wt% CeO2-5 wt% Y2O3 system. The flexural strength was 386.2 MPa, the hardness was 13.5 GPa, the density was 3.9128 g/cm3, the linear shrinkage was 16.31%, and the apparent porosity was 0.62%.
During sintering with the binary sintering aids, CeO2 and Y2O3 solid solutions were added to the corundum-based composite ceramics, forming Zr0.9Ce0.1O2 and Zr0.8Y0.2O1.9 solid solutions, which promoted the decomposition of ZrSiO4 and the sintering of the corundum-based composite ceramics.
Acknowledgments
Authors appreciate the financial supported by the National Natural Science Foundation of China (Grant No. 51872118), the Key Research and Development Program of Shandong Province (Grant No. 2020JMRH0401), the Shandong Provincial Natural Science Foundation (Grant No. ZR2023ME127, ZR2019MEM055). The project supported by State Key Laboratory of Advanced Technology for Materials Synthesis and Processing (Wuhan University of Technology). This work was financially supported by the Taishan Scholars Program, and the Case-by-Case Project for Top Outstanding Talents of Jinan.
Disclosure statement
No potential conflict of interest was reported by the author(s).
Additional information
Funding
References
- Maletsky AV, Belichko DR, Konstantinova TE, et al. Structure formation and properties of corundum ceramics based on metastable aluminium oxide doped with stabilized zirconium dioxide. Ceram Int. 2021;47(14):19489–19495. doi: 10.1016/j.ceramint.2021.03.286
- Xu XH, Zhang QK, Wu JF, et al. Preparation and characterization of corundum-based ceramics for thermal storage. Ceram Int. 2021;47(16):23620–23629. doi: 10.1016/j.ceramint.2021.05.081
- Yin ZB, Huang CZ, Zou B, et al. High temperature mechanical properties of Al2O3/TiC micro–nano-composite ceramic tool materials. Ceram Int. 2013;39(8):8877–8883. doi: 10.1016/j.ceramint.2013.04.081
- Yin YK, Xu JY, Ji M, et al. A critical review on sintering and mechanical processing of 3Y-TZP ceramics. Ceram Int. 2023;49(2):1549–1571. doi: 10.1016/j.ceramint.2022.10.159
- Lao XB, Xu XY, Jiang WH, et al. Effect of aluminum on performances of cordierite-SiCw composite ceramics for high-temperature sensible thermal storage. J Alloys Compd. 2019;780:378–387. doi: 10.1016/j.jallcom.2018.11.351
- Hou Y, Zhang GH, Chou KC. Comparison of hot pressing sintering and conventional powder-sintering in preparation of CaO-Al2O3-SiO2-Fe3O4-R2O glass ceramics. J Non-Cryst Solids. 2021;564:120829. doi: 10.1016/j.jnoncrysol.2021.120829
- Pristinskiy Y, Pinargote NWS, Smirnov A. The effect of MgO addition on the microstructure and mechanical properties of alumina ceramic obtained by spark plasma sintering, Mater. Today Proc. 2019;19:1990–1993. doi: 10.1016/j.matpr.2019.07.058
- Yuan Y, Fan JY, Li JS, et al. Oscillatory pressure sintering of Al2O3 ceramics. Ceram Int. 2020;46(10):15670–15673. doi: 10.1016/j.ceramint.2020.03.117
- Huang WW, Qiu HJ, Zhang YQ, et al. Microstructure and phase transformation behavior of Al2O3–ZrO2 under microwave sintering. Ceram Int. 2023;49(3):4855–4862. doi: 10.1016/j.ceramint.2022.09.376
- Liang YJ, Shi CB, Huang Y, et al. Effect of CaO/SiO2 mass ratio and Li2O on structure and phase precipitation behaviors of CaO-SiO2-MgO-Al2O3 oxide inclusions. J Non-Cryst Solids. 2022;597:121911. doi: 10.1016/j.jnoncrysol.2022.121911
- Gao CX, Zhao XX, Li B. Influence of Y2O3 on microstructure, crystallization, and properties of MgO-Al2O3-SiO2 glass-ceramics. J Non-Cryst Solid. 2021;560:120728. doi: 10.1016/j.jnoncrysol.2021.120728
- Wang M, Zheng QS, Chen AY, et al. Crystallization, thermal expansion and hardness of Y2O3–Al2O3–SiO2 glasses. Ceram Int. 2021;47(17):25059–25066. doi: 10.1016/j.ceramint.2021.05.236
- Wei MJ, He F, Cao XH, et al. Structure and sintering behavior of BaO–SrO–B2O3–SiO2 sealing glass for Al2O3 ceramic substrates. Ceram Int. 2022;48(19):27718–27730. doi: 10.1016/j.ceramint.2022.06.072
- Xu XX, Zhang QK, Wu JF, et al. Preparation and characterization of corundum ceramics doped with Fe2O3 and TiO2 for high temperature thermal storage. Ceram Int. 2022;48(2):1820–1826. doi: 10.1016/j.ceramint.2021.09.263
- Yang Y, Ma MS, Zhang FQ, et al. Low-temperature sintering of Al2O3 ceramics doped with 4CuO-TiO2-2Nb2O5 composite oxide sintering aid. J Eur Ceram Soc. 2020;40:5504–5510. doi: 10.1016/j.jeurceramsoc.2020.06.068
- Ucar V, Ozel A, Mimaroglu A, et al. Influence of SiO2 and MnO2 additives on the dry friction and wear performance of Al2O3 ceramic. Mater Des. 2001;22(3):171–175. doi: 10.1016/S0261-3069(00)00069-8
- Wang XY, Wang XL, Sun XW, et al. Microstructure and properties evolution of plasma sprayed Al2O3-Y2O3 composite coatings during high temperature and thermal shock treatment. J Rare Earths. 2021;39:718–727. doi: 10.1016/j.jre.2020.09.008
- He W, Ai YL, Liang BL, et al. Effects of La2O3 and Nb2O5 dopants on the microstructural development and fracture toughness of Al2O3 ceramic, Mater. Sci Eng A. 2018;723:134–140. doi: 10.1016/j.msea.2018.03.057
- Chen PC, Cheng CW, Kao IC, et al. Effect of co-doping NiO and Nb2O5 on phase stability and mechanical properties of Y2O3-stabilized ZrO2/Al2O3 composites. Adv Powder Technol. 2016;27:877–881. doi: 10.1016/j.apt.2016.01.017
- Yu WJ, Zheng YT, Pan JY, et al. Phase evolution and microstructure analysis of Al2O3–ZrO2(Y2O3) eutectic powders with ultra-fine nanostructure. Ceram Int. 2019;45(17):23423–23430. doi: 10.1016/j.ceramint.2019.08.046
- Fang YH, Chen N, Du GP, et al. Effect of Y2O3-stabilized ZrO2 whiskers on the microstructure, mechanical and wear resistance properties of Al2O3 based ceramic composites. Ceram Int. 2019;45:16504–16511. doi: 10.1016/j.ceramint.2019.05.184
- Wang ZJ, Liu LL, Du QF, et al. Enhanced microwave dielectric properties of CeO2–TiO2 ceramics by adding Al2O3 for microstrip antenna application. Ceram Int. 2022;48(10):14378–14385. doi: 10.1016/j.ceramint.2022.01.329
- Lyu XX, Zhao ZY, Sun HL, et al. Influence of Y2O3 contents on sintering and mechanical properties of B4C-Al2O3 multiphase ceramic composites. J Mater Res Technol. 2020;9(5):11687–11701. doi: 10.1016/j.jmrt.2020.08.072
- Zhai SY, Liu JC, Lan DH, et al. High temperature tensile strength of large size Al2O3/ZrO2(Y2O3) directionally solidified eutectic ceramics. Mater Lett. 2022;307:130950. doi: 10.1016/j.matlet.2021.130950
- Yu YD, Pan JY, Yuan YC, et al. Effect of Y2O3 contents on microstructural, mechanical, and antioxidative characteristics of Al2O3-ZrO2-Y2O3 coatings, Appl. Surf Sci. 2022;590:153096. doi: 10.1016/j.apsusc.2022.153096
- Zhang Y, Ma XG, Li XY, et al. Crystallization kinetics of Al2O3-26mol%Y2O3 glass and full crystallized transparent Y3Al5O12-based nanoceramic. J Eur Ceram Soc. 2021;41:1557–1563. doi: 10.1016/j.jeurceramsoc.2020.09.036
- Wei D, Xu XQ, Wang YL, et al. Quasi-elastic deformation with rebound resilience in bulk amorphous Al2O3–ZrO2–Y2O3 at moderate temperature. Ceram Int. 2020;46(18):29352–29355. doi: 10.1016/j.ceramint.2020.08.103
- Ma YH, Ouyang JH, Wang ZG, et al. Insights into intragranular precipitation and strengthening effect in Al2O3/SmAlO3 ceramic with eutectic composition, Mater. Sci Eng A. 2019;754:382–389. doi: 10.1016/j.msea.2019.03.091
- Song XW, Xie M, Zhou F, et al. High-temperature thermal properties of yttria fully stabilized zirconia ceramics. J Rare Earths. 2011;29(2):155–159. doi: 10.1016/S1002-0721(10)60422-X
- Wang X, Xue ZL, Zhou ZM, et al. Influences of ZrSiO4 doping on microstructure and mechanical properties of Y2SiO5–ZrSiO4 ceramics. Ceram Int. 2022;48(1):1277–1284. doi: 10.1016/j.ceramint.2021.09.212
- Ivanov DA, Sitnikov AI, Val′yano GE, et al. Alumina ceramics sintered from hollow corundum microspheres with Al2O3 and ZrO2–Y2O3 as sintering activators. Ceram Int. 2023;49(1):1496–1501. doi: 10.1016/j.ceramint.2022.09.320
- Yu WJ, Zhang EL, Yu YD, et al. Effects of CeO2 on the phase, microstructure and mechanical properties of Al2O3-ZrO2(CeO2) nanocomposite ceramics (AZC-NCs) by solid solution precipitation. Ceram Int. 2022;48:34454–34464. doi: 10.1016/j.ceramint.2022.08.025
- Kumatani N, Suda A, Morikawa A, et al. Synthesis of a nanosized homogeneous Al2O3–CeO2–ZrO2 composite as an oxygen-storage material for highly improved thermal durability. Ceram Int. 2023;49(11):19265–19272. doi: 10.1016/j.ceramint.2023.03.053
- Santos C, Coutinho IF, Amarante JEV, et al. Mechanical properties of ceramic composites based on ZrO2 co-stabilized by Y2O3-CeO2 reinforced with Al2O3 platelets for dental implants. J Mech Behav Biomed Mater. 2021;116:104372. doi: 10.1016/j.jmbbm.2021.104372
- Yao YJ, Li CC, Wang L, et al. Mechanical behaviors of alumina ceramics doped with rare-earth oxides. Rare Met. 2010;29:456–459. doi: 10.1007/s12598-010-0149-5