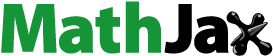
ABSTRACT
The impact of CuB2O4 additive used as a sintering aid on the microstructures and microwave dielectric characteristics of mixed phase (Mg0.95Zn0.05)Co0.05TiO3-Ca0.6(La0.9Y0.1)0.2667TiO3 ceramics was investigated. CuB2O4 additives can effectively lower the densification temperature of (Mg0.95Zn0.05)Co0.05TiO3-Ca0.6(La0.9Y0.1)0.2667TiO3 from 1450°C to the range of 1050°C ~ 1175°C. Doping CuB2O4 (0.25 ~ 2 wt%) in 0.875(Mg0.95Zn0.05)Co0.05TiO3-0.125Ca0.6(La0.9Y0.1)0.2667TiO3 ceramics can substantially enhance their microwave dielectric properties and density. With the addition of 1% and 0.25% CuB2O4, Q × f values of 43,000 GHz and 7700 GHz were obtained at 1050–1175°C, respectively. Different additions of CuB2O4 have a significant effect on the Q × f value. Temperature coefficient of resonant frequency of 0.875(Mg0.95Zn0.05)Co0.05TiO3-0.125Ca0.6(La0.9Y0.1)0.2667TiO3 (0.875MZCT–0.125CLYT) varied from 16 to 30.9 ppm/°C when adding different CuB2O4 (0.25 ~ 2 wt%).
KEYWORDS:
1. Introduction
As mobile communication technology enters the (5th generation mobile networks) 5 G era, the development of massive multi-input multi-output (MIMO) technology, coupled with the increasingly stringent requirements brought by base station integration, puts forward higher requirements for filters [Citation1,Citation2]. Microwave ceramic filter element media,which has received a lot of interest in the field of next-generation filters [Citation2,Citation3], has a comprehensive advantage of light weight and good temperature drift resistance under the premise of achieving the core performance requirements [Citation4–8].
The manufacture of powder raw materials and the improvement of ceramic properties of microwave dielectric are now the most challenging technical issues to overcome in order to better fulfill the development needs of ceramic filters. Permittivity(εr), quality factor (Q × f),and temperature coefficient of resonant frequency are the three main characteristics of microwave dielectrics. A low εr can shorten the signal transmission time, a high Q × f can increase the selectivity and longevity of microwave components, and a temperature coefficient of resonant frequency close to zero can allow electronic devices to operate reliably in a range of temperature environments [Citation9–18].
MgTiO3-based microwave dielectrics have been intensively studied for years with low dielectric loss. MgTiO3-CaTiO3 ceramics made of a mixture of modified magnesium titanate and perovskite structured calcium titanate have been applied in dielectric resonators and patch antennas. With a ratio of Mg:Ca = 95:5, 0.95 MgTiO3-0.05CaTiO3 mixed phase ceramic has an εr ~21, a Q×f ~ 56,000 (at 7 GHz), and a zero τf value [Citation19]. With the partial replacement (Mg0.7Zn0.3) by Co, (Mg0.7Zn0.3)0.95Co0.05TiO3 had excellent dielectric properties with an εr ~20, Q×f ~ 163,560 GHz, and a τf ~ −65 ppm/oC. To produce a temperature-stable material, Ca0.6(La0.9Y0.1)0.2667TiO3 which has a large positive τf value of +380 ppm/ oC, was added to (Mg0.7Zn0.3)0.95Co0.05TiO3.
There are three approaches to reducing the sintering temperature of microwave dielectric ceramics: low melting sintering aids addition [Citation20–22], chemical processing, and the use of smaller particles as the starting materials. Of these three, sintering aids addition is the most effective and least expensive. However, no liquid-phase sintering of xMZCT-(1-x)CLYT with sintering aids addition has been reported yet. Previously, CuB2O4 was researched and found to have good dielectric properties [Citation23].
In this paper, CuB2O4 was used as a sintering aid for reducing the sintering temperature of xMZCT-(1-x)CLYT ceramics. The effects of CB content on the densification, phase development and dielectric properties of 0.875MZCT–0.125CLYT ceramics are investigated. The analysis focused on the crystal phase, microstructure, and microwave dielectric properties of 0.875MZCT–0.125CLYT ceramics intermixed with CuB2O4.
2. Experimental
Samples for the 0.875MZCT–0.125CLYT were created using traditional solid-state techniques. The initial components are combined in accordance with the stoichiometric ratio. For CuB2O4 powder, high-purity powders of CuO and H3BO3 were weighed, mixed and calcined at 850°C for 3 h. As a sintering aid, a trace amount of CuB2O4 (0.25%– 2% weight) was used. The (Mg0.95Zn0.05)Co0.05TiO3 were synthesized using a solid-state mixed oxide route with starting materials of high-purity oxide powders (>99.9%): MgO, ZnO, CoO and TiO2. Because MgO is hygroscopic, it was first fired at 600°C to avoid moisture contain. The weighed raw materials were mixed by ball milling with agate media in distilled water for 24 h, and the mixtures were dried and calcined at 1100°C for 4 h. Samples of Ca0.6(La0.9Y0.1)0.2667TiO3 ceramics were processed using a conventional solid-state reaction from high-purity oxide powders (CaCO3, La2O3, Y2O3, and TiO2). After mixing, milling for 24 h, drying, and grinding, the mixtures were calcined at 1000 ◦C for 2 h.
The powder comprising (Mg0.95Zn0.05)Co0.05TiO3 (MZCT) and Ca0.6(La0.9Y0.1)0.2667TiO3 (CLYT) is weighed, and then ground with ZrO2 balls in distilled water for 12 h before being dried.
The powder calcination at different temperatures, then mix with massage fraction 0.875MZCT–0.125CLYT. The calcined powder was mixed with varying quantities of CuB2O4 additives serving as sintering aids to achieve the desired composition of 0.875MZCT–0.125CLYT. It was subsequently reground with Polyvinyl alcohol (PVA) solution as a binder for 5 h. Uniaxial pressing is used to press particles having a 1.1 cm diameter and 0.5 cm thickness. At 120 MPA, all samples were compressed. These particles are sintered in air for hours at a temperature between 1050 and 1175°C after being degreased.
X-ray diffraction (XRD) with CuKα1 radiation (XRD-7000,Shimadzu, Kyoto,Japan) was used to examine the phase purity and crystal structure of 0.875MZCT–0.125CLYT ceramics. The Archimedes method was used to calculate the bulk densities.
Using a scanning electron microscope (FE-SEM, Model S4800; Hitachi, Japan), microstructure images were taken. The Agilent E8362B network analyzer was used to measure the permittivity and quality factor values in the TE011 mode, following the Hakki–Coleman method [Citation24,Citation25]. The temperature coefficient of resonant frequency (τf) was measured for a temperature range of 25–85°C to determine the temperature coefficient of resonant frequency. The following equation was used to determine the value of τf:
Where f1 denotes the resonant frequency at T1 and f2 denotes the resonant frequency at T2.
3. Results and discussion
shows the XRD patterns of the 0.875MZCT–0.125CLYT ceramic sintered at various temperatures for 4 h. The XRD patterns showed that peaks indicating the presence of (Mg0.95Zn0.05)Co0.05TiO3 (MZCT)(ICDD-PDF #01-073-7752) as the main crystalline phase, in association with Ca0.6(La0.9Y0.1)0.2667TiO3 (CLYT) (ICDD-PDF #22–0153) as minor phases. It is understood that crystal structures of (Mg0.95Zn0.050)Co0.05TiO3 and Ca0.6(La0.9Y0.1)0.2667TiO3 are trigonal crystal structures and orthorhombic crystal structures, respectively and the fact that the average ion radii of Ca2+, La3+, and Y3+ were bigger than those of Mg2+(72 pm) the solid solution could not be produced. MgTi2O5 usually formed as an intermediate phase. According to the XRD patterns, the (Mg0.95Zn0.05)Co0.05TiO3 phase exists in these specimens. X-ray diffraction patterns of 0.875MZCT–0.125CLYT ceramics system have not been changed significantly with sintering temperatures in the range 1050–1175°C. The XRD patterns show peaks indicating the presence of (Mg0.95Zn0.05)Co0.05TiO3 as the main crystalline phase, a minor phase of Ca0.6(La0.9Y0.1)0.2667TiO3. displays sintered at various temperatures for 4 h. Four SEM images of 0.875MZCT–0.125CLYT ceramics that include CuB2O4 additions of 1 weight percent. As the sintering temperature increased, it was evident that the grain size also increased for the 0.875MZCT–0.125CLYT ceramics. However,when 1 wt% CuB2O4 was added, the ceramics did not achieve high density and no grain growth was observed at 1050°C, as indicated by SEM results for different temperatures. As shown in , the liquid-phase effect caused an increase in grain size as the sintering temperature. CuB2O4 impact on the liquid phase could be significant. At 1100°C, significant grain development was seen, and for specimens sintered at 1125°C, the holes were virtually completely obliterated.
Figure 1. X-ray diffraction patterns of 0.875MZCT–0.125CLYT ceramics with 1 wt% CuB2O4 additives sintered at:(a) 1050°C, (b) 1075°C, (c) 1100°C, (d) 1125°C, (e) 1150°C, and (f) 1175°C for 4 h. (✧ : MZCT, +: CLYT, ✰: MgTi2O5).
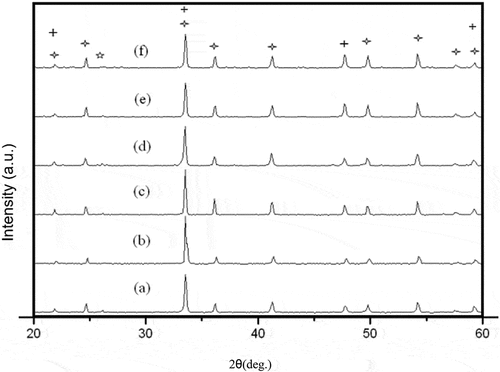
Figure 2. SEM images were taken of 0.875MZCT–0.125CLYT ceramics containing 1 wt% CuB2O4, sintered at different temperatures for a duration of four hours, including (a) 1050°C, (b) 1075°C, (c) 1100°C, (d) 1125°C, (e) 1150°C, and (f) 1175°C.
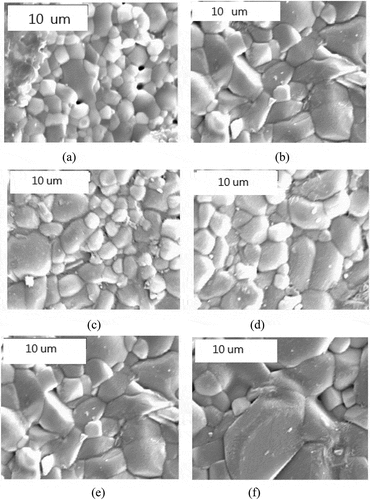
depicts the densities of 0.875MZCT–0.125CLYT ceramics doped with varying quantities of CuB2O4 throughout a 4-h period at varied sintering temperatures. As shown in the figure, due to the increase in grain size, the density increases to 1 wt% with the increase of sintering temperature and the increase of CuB2O4 additives. The rise in bulk density with a higher sintering temperature may be attributed to the reduction in pore numbers,as depicted in , whereas the reduction in bulk density could arise from a typical grain growth. Even with so much CuB2O4 doping, it seems like the bulk density has reached saturation at 1100°C. The maximum density of 0.875MZCT–0.125CLYT with 1 wt% CuB2O4 is 4.3 g/cm [Citation3] sintered at 1100°C for 4 h. Therefore, the inclusion of CuB2O4 additives can significantly lower the sintering temperature required for 0.875MZCT–0.125CLYT ceramics sintering temperature in the CuB2O4-doped 0.875MZCT–0.125CLYT ceramic. Excessive will reduce the density because CB is a glass phase and the CB density is lower than 0.875MZCT–0.125CLYT, and excessive CB addition over 2 wt.% will reduce the density.
Figure 3. Bulk density curves were generated for 0.875MZCT–0.125CLYT ceramics with varying amounts of CuB2O4 additives sintered at different temperatures for four hours.
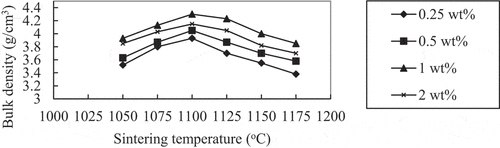
When the sintering temperature rises, the energy rises meaning that the grain boundary moves better and it is easy for the small grains to dissolve, so that the grain growth is more uniform and the pores decrease with the increase in the sintering temperature, and densification occurs. In addition, when various additives are added to the 0.875MZCT–0.125CLYT ceramics to form liquid-phase sintering, this is more conducive to small-grain dissolution, an increase in the wetting boundary movement rate, and grain rearrangement to achieve densification. This can be compared with the SEM in for the 0.875MZCT–0.125CLYT ceramics with multiple sintering aids CB that were sintered at 1050°C for 4 h, where the grains were smaller, more pores appeared, and the density was lower; when the sintering temperature rose to 1100°C, the grain size was consistent, there were fewer pores, and the density was higher. It can be concluded that liquid-phase sintering occurs when using different additives, and when the additive exceeds 2 wt%, it will cause a slight decrease in the density. Generally, in cases of liquid-phase sintering, using appropriate amounts of sintering aids can effectively improve the phase-forming capacity and reduce the sintering temperature. However, when the sintering aid content is excessive, the grains grow excessively, and with a larger particle size, the rearrangement will be subject to greater resistance and there will be a small number of pores in the boundary, meaning it is not easy to discharge and other factors will affect the change in the density and radio-frequency dielectric performances.
The dielectric constant marginally rises as the sintering temperature rises. Additionally, larger CuB2O4 concentration raises the density value, which translates to higher εr as shown in .
Figure 4. The dielectric constant curves for 0.875MZCT–0.125CLYT ceramics with different amounts of CuB2O4 additives at various sintering temperatures were recorded over a period of four hours.
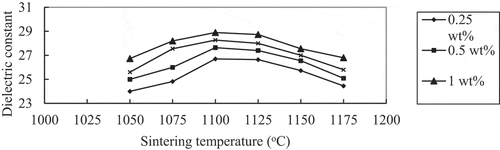
Lichterecker [Citation26] proposed an intermediate form between the serial and parallel mixing rules form called logarithmic mixing rule (in the case where α → 0) [Citation26]:
where and
are the relative dielectric constants of the high-dielectric phase and low-dielectric phase, respectively, Vh and Vl the volume fractions of the high dielectric phase and low-dielectric phase (Vh+Vl = 1),
the effective dielectric constant of the composite, and α a parameter that determines the type of mixing rule.
The addition of 1 wt% CuB2O4 to the 0.875MZCT–0.125CLYT ceramic, accompanied by a rise in sintering temperature from 1050°C to 1100°C, resulted in an increase of εr value from 26.73 to 28.9. Microwave dielectric loss is influenced by various factors, which can be classified into intrinsic and extrinsic losses. The lattice vibration mode is the primary cause of intrinsic loss, whereas second phase, oxygen vacancy, grain size, and densification or porosity are the main contributors to extrinsic loss [Citation27]. It is believed that porous materials are significantly influenced by interfacial polarization [Citation28].
The quality factor (Q × f) value of 0.875MZCT–0.125CLYT ceramics that incorporate different CuB2O4 additives at varying sintering temperatures is presented in .
Figure 5. The quality factor values were measured for 0.875MZCT–0.125CLYT systems with varying amounts of CuB2O4 additives, sintered at different temperatures for four hours.
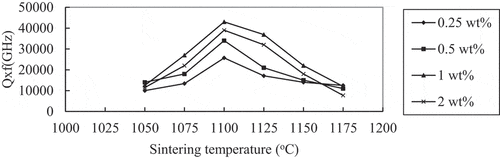
Internal factors, such as lattice vibration mode, impact the Q × f value of microwave loss. The presence of cation ordering can often result in an increase in Q × f, thus its absence may frequently lead to a decrease in Q × f [Citation29].
The decrease of Q × f in mixed systems has been associated by some with the loss of cation ordering in ordered cation compounds [Citation30].
However, it should be noted that the reduction of the Q × f value can be due to either intrinsic (i.e. attice related) or extrinsic mechanisms. At microwave frequencies, the unloaded quality factor is said to be dependent on extrinsic factors like secondary phases, density, and oxygen vacancies. The Q × f value increases to its maximum value and subsequently decreases as the sintering temperature rises. At 1100°C, the maximum Q × f value of 0.875MZCT–0.125CLYT ceramic containing 1 wt% CuB2O4 additive is 43,000 GHz. Abnormal grain growth at higher sintering temperatures is responsible for the degradation of the Q × f value, as demonstrated. Conversely, due to the enhancement in densification of the specimen by the liquid phase, CuB2O4-doped 0.875MZCT–0.125CLYT exhibits a single maximum Q × f value at lower sintering temperatures. Dielectric losses in microwave materials are primarily due to lattice vibration patterns, as well as pores,impurities, second phases,or lattice defects. The controlling role of relative density in these losses has been demonstrated in other types of microwave dielectric materials as well [Citation31,Citation32].
Due to the addition of sintering aids, the Q × f values of the samples in this work are approximately half of those of the samples sintered at 1450°C. However, adding sintering aids can reduce the sintering temperature.
Ceramics containing 0.5 wt%of CuB2O4 additive in 0.875MZCT–0.125CLYT were found to lower the sintering temperature compared to pure 0.875MZCT–0.125CLYT ceramics sintered at 1450°C (Q × f ~90000 at 8 GHz).
displays the temperature coefficients of resonant frequency (τf) for 0.875MZCT–0.125CLYT ceramics with different CuB2O4 additives and sintering temperatures. The relationship between the temperature coefficient of resonant frequency (TCF) and the temperature coefficient of dielectric constant (TCK) as well as the thermal expansion coefficient (a) is stated in EquationEquation 4(4)
(4) [Citation33]
Figure 6. The resonant frequency values of 0.875MZCT–0.125CLYT system,with various CuB2O4 additives sintered for four hours at different temperatures, were analyzed for their temperature coefficients.
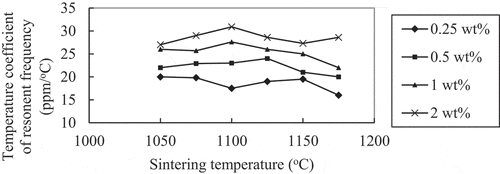
The linear thermal expansion coefficient,denoted as L,is a constant in ceramics and directly affects the temperature coefficient of capacitance (TCF) through its relationship with TCK. The temperature dependence of the dielectric constant (TCK) can be expressed as three terms(A,B,and C) in EquationEquation (4)(4)
(4) [Citation34]:
Where and V denote the polariz ability and volume,respectively. The term A (commonly negative) represents the direct dependence of the polarizability on temperature. B and C represent the increase of the polarizability and the decrease of the number of polarizable ions in the unit-cell, respectively; the unit cell volume increased with an increase in temperature. The B and C terms are normally the largest ones but have similar value with opposite signs. Hence, (B+C) has a small positive value. TCK is increased by an increase of the tilting of oxygen octahedra in the perovskite structure, which correspond to a decrease of TCF by EquationEquation (4)(4)
(4) .
The temperature coefficient of the resonant frequency (τf) is typically determined by the composition and phase within the ceramic, while demonstrating insensitivity across all sintering temperature ranges. With the increase of CuB2O4 additives, the τf value becomes more positive. As the sintering temperature varies, the CuB2O4 additive increases from 0.25 wt% to 2 wt% resulting in a variation of 17.5 to 30.9 ppm/oC. Increasing the amount of CLYT can result in achieving zero τf, as the τf values for (Mg0.95Zn0.05)Co0.05TiO3 and CLYT are −50 and + 310 ppm/oC,respectively.
The temperature coefficient of resonant frequency (τf) for ceramics was related to composition and existing phases. Regardless of sintering temperature range, τf was not affected. When CuB2O4 additives were increased, the τf value became more positive and ranged from 17.5 to 30.9 ppm/oC. Increasing CLYT content could achieve zero τf since (Mg0.95Zn0.05)Co0.05TiO3 and CLYT had different τf values of −50 and +310 ppm/oC, respectively.
4. Conclusion
The study focused on the dielectric properties of 0.875MZCT–0.125CLYT ceramics containing CuB2O4. Secondary phases such as MgTi2O5 were mixed with 0.875MZCT–0.125CLYT ceramics, with MgTi2O5 serving as the primary phase. The addition of CuB2O4 in 0.875MZCT–0.125CLYT ceramics not only lowers the sintering temperature effectively but also enhances its microwave dielectric properties. At a temperature of 1100°C, ceramics with a composition of 0.875MZCT–0.125CLYT and an addition of 1 wt% CuB2O4 exhibit exceptional microwave dielectric characteristics including εr of approximately 28.9, Q × f value of approximately 43,000 (at 8 GHz),and τf value of approximately 27.6 ppm/oC.
Author contributions
Yuan-Bin Chen: Preparation of materials, methodology, and software. Preparation of materials. Data curation and writing – original draft preparation. Writing – reviewing and editing. Software, theoretical calculation, and validation.
Declarations
Conflict of interest: The authors declare that they have no known competing financial interests or personal relationships that could have appeared to influence the work reported in this paper.
Disclosure statement
No potential conflict of interest was reported by the author(s).
Data availability statement
The data that support the findings of this study are openly available to the reviewers.
References
- Xiang H-C, Li C-C, Jantunen H, et al. Ultralow loss CaMgGeO4 Microwave dielectric ceramic and its chemical compatibility with silver electrodes for low-temperature cofired ceramic applications. ACS Sustainable Chem Eng. 2018;6(5):6458–6466. doi: 10.1021/acssuschemeng.8b00220
- George S, Sebastian M-T. Microwave dielectric properties of novel temperature stable high Q Li2Mg1−Zn Ti3O8 and Li2A1−Ca Ti3O8 (A = Mg, Zn) ceramics. J Eur Ceram Soc. 2010;30(12):2585–2592. doi: 10.1016/j.jeurceramsoc.2010.05.010
- Sebastian M-T. Dielectric materials for wireless communication. Oxford, UK: Elsevier Publishers; 2008.
- Shu G-J, Yang F, Hao L, et al. Low-firing and microwave dielectric properties of a novel glass-free MoO3-based dielectric ceramic for LTCC applications. J Mater Sci Mater Electron. 2019;30(8):7485–9. doi: 10.1007/s10854-019-01061-1
- Zhang J, Yue Z-X, Luo Y, et al. Novel low-firing forsterite-based microwave dielectric for LTCC applications. J Am Ceram Soc. 2016;99(4):1122–4. doi: 10.1111/jace.14132
- Song X-Q, Lei W, Zhou Y-Y, et al. Ultra-low fired fluoride composite microwave dielectric ceramics and their application for BaCuSi2O6-based LTCC. J Am Ceram Soc. 2019;103(2):1–9. doi: 10.1111/jace.16795
- Chen Y-W, Li E-Z, Duan S-X, et al. Low temperature sintering kinetics and microwave dielectric properties of BaTi5O11 ceramic. ACS Sustainable Chem Eng. 2017;5(11):10606–13. doi: 10.1021/acssuschemeng.7b02589
- Zhou D, Pang LX, Wang D-W, et al. High permittivity and low loss microwave dielectrics suitable for 5G resonators and low temperature co-fired ceramic architecture. J Mater Chem C. 2017;5(38):10094–8. doi: 10.1039/C7TC03623J
- Varghese J, Siponkoski T, Teirikangas M, et al. Structural, dielectric and thermal properties of Pb free molybdate based ultra-low temperature glass. ACS Sustainable Chem Eng. 2016;4(7):3897–3904. doi: 10.1021/acssuschemeng.6b00721
- Xiao M, Sun H-R, Zhou Z-Q, et al. Bond ionicity lattice energy, bond energy, and microwave dielectric properties of Ca1-xSrxWO4 ceramics. Ceram Int. 2018;44(17):20686–20691. doi: 10.1016/j.ceramint.2018.08.062
- Kim E-S, Kim S-H, Lee B-I. Low-temperature sintering and microwave dielectric properties of CaWO4 ceramics for LTCC applications. J Eur Ceram Soc. 2006;26(10–11): 2101–4. doi: 10.1016/j.jeurceramsoc.2005.09.064
- Krzmanc M-M, Logar M, Budic B, et al. Dielectric and microstructural study of the SrWO4, BaWO4, and CaWO4 scheelite ceramics. J Am Ceram Soc. 2011;94(8):2464–72. doi: 10.1111/j.1551-2916.2010.04378.x
- Kim ES, Kim SH. Effects of structural characteristics on microwave dielectric properties of (1−x)CaWO4 –xLanbo4 ceramics. J Electroceram. 2006;17(2–4):47–77. doi: 10.1007/s10832-006-8571-7
- Hu X-Q, Jiang J, Wang J-Z, et al. A new additive-free microwave dielectric ceramic system for LTCC applications: (1 − x)CaWO4 − x(Li0.5Sm0.5)WO4. J Mater Sci Mater Electron. 2020;31(3):2544–2550. doi: 10.1007/s10854-019-02791-y
- Bian J-J, Ding Y-M. Structure, sintering behavior, and microwave dielectric properties of (1-x)CaWO4-xYLiF4 (0.02. Mater Res Bull. 2015;67:245–250. doi:10.1016/j.materresbull.2014.09.078
- Zhang S, Su H, Zhang H-W, et al. Microwave dielectric properties of CaWO4–Li2TiO3 ceramics added with LBSCA glass for LTCC applications. Ceram Int. 2016;42(14):15242–15246. doi: 10.1016/j.ceramint.2016.06.161
- Jeon C-J, Kim E-S. Low-temperature sintering of 0.85CaWO4–0.15LaNbO4 ceramics. Ceram Int. 2008;34(4):921–924. doi: 10.1016/j.ceramint.2007.09.058
- Liao Q-W, Wang Y-L, Jiang F, et al. Ultra-low fire glass-free Li3FeMo3O12 microwave dielectric ceramics. J Am Ceram Soc. 2014;97(8):2394–6. doi: 10.1111/jace.13073
- Huang C-L, Chen Y-B, Tasi C-F. Influence of V2O5 additions to 0.8(Mg0.95Zn0.05)TiO3–0.2Ca0.61Nd0.26TiO3 ceramics on sintering behavior and microwave dielectric properties. J Alloys Compd. 2008;454(1–2):454–459. doi: 10.1016/j.jallcom.2006.12.125
- Yang C, Chen Y, Tzou W, et al. Sintering and microwave dielectric characteristics of MCAS glass-added 0.84 Al2O3–0.16 TiO2 ceramics. Mater Lett. 2003;57:2945.
- Lu SG, Kwok KW, Chan HLW, et al. Structural and electrical properties of BaTi4O9 microwave ceramics incorporated with glass phase. Mater Sci Eng B. 2003;99(1–3):491. doi: 10.1016/S0921-5107(02)00506-8
- Surendran KP, Mohanan P, Sebastian MT. The effect of glass additives on the microwave dielectric properties of Ba(Mg1/3Ta2/3)O3 ceramics. J Solid State Chem. 2004;177(11):4031. doi: 10.1016/j.jssc.2004.07.018
- Chu Y-J, Jean J-H. Low-fire processing of microwave BaTi4O9 dielectric with crystalline CuB2O4 and BaCuB2O5 additives. Ceram Int. 2013;39(5):5151–5158. doi: 10.1016/j.ceramint.2012.12.011
- Hakki BW, Coleman PD. A dielectric resonator method of measuring inductive capacities in the millimeter range. IEEE Trans Microwave Theory Tech. 1960;8(4):402. doi: 10.1109/TMTT.1960.1124749
- Courtney WE. Analysis and evaluation of a method of measuring the complex permittivity and permeability microwave insulators. IEEE Trans Microwave Theory Tech. 1970;18(8):476. doi: 10.1109/TMTT.1970.1127271
- Lichtenecker K. Dielektrizitatskonstante naturlicher und kunstlicher mischkorper[J]. Physikalische Zeitschrift. Phys Z. 1926;27:115.
- Wang Y, Jiqing L, Wang J, et al. Lattice vibrational characteristics, crystal structure and dielectric properties of Ba2MgWO6 microwave dielectric ceramic. Ceramics Int. 2021;47(12):17784–17788. doi: 10.1016/j.ceramint.2021.02.224
- Zhang C, Luo K, Liu J, et al. Realizing optimized interfacial polarization and impedance matching with CNT-confined Co nanoparticles in hollow carbon microspheres for enhanced microwave absorption. J Mater Sci Tech. 10 March 2024;175:1–9. doi: 10.1016/j.jmst.2023.07.034
- Kim Y-I, Woodward PM. Crystal structures and dielectric properties of ordered double perovskites containing Mg2+ and Ta5+. J Solid State Chem. 2007 October;180(10):2798–2807.
- Kreller CR, Uberuaga BP. The role of cation ordering and disordering on mass transport in complex oxides. Curr Opin Solid State Mater Sci. 2021 April;25(2):100899. doi: 10.1016/j.cossms.2021.100899
- Wesselinowa JM. Phonon damping in ferromagnetic semiconducting thin films. J Magn Magn Mater. 2004 August;279(2–3):276–282. doi: 10.1016/j.jmmm.2003.12.1425
- Bing-Jing L, Wang S-Y, Liao Y-H, et al. Dielectric properties and crystal structure of (Mg1−xCox)2(Ti0.95Sn0.05)O4 ceramics. J Ceram Soc Japan. 2014;122(11):955–958. doi: 10.2109/jcersj2.122.955
- Reaney IM, Colla EL, Setter N. Dielectric and structural characteristics of Ba- and Sr-based complex perovskites as a function of tolerance factor. Jpn J Appl Phys Part. 1994;33(33):3984. doi: 10.1143/JJAP.33.3984
- Zhang S, Sahin H, Torun E, et al. Fundamental mechanisms responsible for the temperature coefficient of resonant frequency in microwave dielectric ceramics. J Am Ceram Soc. 2017 April;100(4):1508–1516. doi: 10.1111/jace.14648