ABSTRACT
Live poultry markets (LPMs) are regarded as hubs for avian influenza virus (AIV) transmission in poultry and are a major risk factor in human AIV infections. We performed an AIV surveillance study at a wholesale LPM, where different poultry species were sold in separate stalls, and nine retail LPMs, which received poultry from the wholesale LPM but where different poultry species were sold in one stall, in Guangdong province from 2017 to 2019. A higher AIV isolation rate was observed at the retail LPMs than the wholesale LPM. H9N2 was the dominant AIV subtype and was mainly present in chickens and quails. The genetic diversity of H9N2 viruses was greater at the retail LPMs, where a complex system of two-way transmission between different poultry species had formed. The isolated H9N2 viruses could be classed into four genotypes: G57 and the three novel genotypes, NG164, NG165, and NG166. The H9N2 AIVs isolated from chickens and quails at the wholesale LPM only belonged to the G57 and NG164 genotypes, respectively. However, the G57, NG164, and NG165 genotypes were identified in both chickens and quails at the retail LPMs. We found that the replication and transmission of the NG165 genotype were more adaptive to both poultry and mammalian models than those of its precursor genotype, NG164. Our findings revealed that mixed poultry selling at retail LPMs has increased the genetic diversity of AIVs, which might facilitate the emergence of novel viruses that threaten public health.
Introduction
Avian influenza viruses (AIVs) not only inflict great economic losses on the poultry industry but also can be transmitted to humans, thus posing a threat to public health. The viruses of four severe influenza pandemics (i.e. the Spanish flu of 1918 [H1N1], the Asian flu of 1957 [H2N2], the Hong Kong flu of 1968 [H3N2], and the swine flu of 2009 [H1N1]) were all related to AIVs, which acquired virulence factors through adaptive mutation or reassortment with circulating human or swine viruses [Citation1,Citation2]. In recent decades, novel H7N9, H5N6, H10N3, H10N8, and H3N8 AIVs have repeatedly infected humans in China [Citation3–6]. The H7N9 virus, in particular, has caused 1,568 human infections and 616 deaths to date. Of note, some or even all the internal gene segments of most novel AIVs originate from H9N2 AIVs.
Several characteristics of H9N2 AIVs may have contributed to this phenomenon. First, in China, the H9N2 has been the dominant AIV subtype in poultry since 2016; thus, H9N2 has had many opportunities to undergo reassortment with other AIVs [Citation7]. Second, the adaptation of H9N2 AIVs to mammalian hosts is increasing. Since 2013, the number of human H9N2 infection cases has steadily risen, while the replication ability and transmissibility of H9N2 AIVs in mice and ferret models have also increased [Citation8,Citation9]. Third, there is a high genetic compatibility between H9N2 AIVs and other influenza viruses [Citation10]. Therefore, it is necessary to pay close attention to the evolution and reassortment of H9N2 AIVs.
In China, which is regarded as an epicenter for AIVs, the H9N2 virus has been detected in multiple avian species, including chickens, ducks, quails, pheasants, partridges, guinea fowl, pigeons, chukars, green peafowl, and wild birds [Citation11–14]. Duck species can shed and spread viruses from both the respiratory and intestinal tracts, while showing few or no disease signs. The absence of disease signs has led to the concept that ducks, with their surreptitious spread of viruses, are the “Trojan horses” of AIV transmission [Citation15]. Chickens have been reported to be major hosts for H9N2 AIVs [Citation16]. Meanwhile, because of their high susceptibility to aquatic bird viruses, quails are thought to serve as intermediate hosts for AIVs that are transmitted between aquatic and terrestrial birds [Citation17]. However, AIV surveillance in quails has been limited.
LPMs can serve as hubs for AIV transmission in poultry and are a major risk factor in human infection with AIVs such as H5N1, H7N9, H9N2, and H10N8 [Citation18–24]. Approximately 87% of people infected with H7N9 AIVs have had close contact with live poultry or were exposed to contaminated environments such as poultry markets [Citation25]. Moreover, LPM closures have reduced the incidence of human infections with H7N9 AIVs [Citation26]. Guangdong province in southern China is home to ≈ 10% of China’s domestic poultry industry and is thought to be a major epicenter of influenza A virus circulation [Citation27]. A survey in Guangzhou, the provincial capital of Guangdong, found that 80% of households reported purchasing poultry at LPMs at least once a year, with more frequent purchases also being common [Citation28]. Genetic studies have revealed that two-way interspecies transmission exists between different types of poultry at LPMs [Citation11]. The H5N1 influenza virus, which killed humans and poultry in 1997, resulted from a reassortant that possibly arose in a domestic poultry species sold at LPMs in Hong Kong [Citation29–31]. Therefore, determining the LPM-associated factors that contribute to AIV prevalence is important for the prevention and control of AIV.
LPMs in China can be divided into two types: wholesale LPMs (where no mixed selling of different poultry species occurs) and retail LPMs (which practice mixed selling) [Citation32]. This provides an ideal model for exploring the possible roles of vending processes at LPMs in the evolution of AIVs. Thus, we performed an AIV surveillance study at different types of LPMs located in Guangdong province. We found that the subtype diversity of AIVs was higher at retail LPMs than at the wholesale LPM, and that H9N2 was still the predominant subtype. Moreover, the genetic diversity of H9N2 viruses was higher at the retail LPMs and some novel reassortant H9N2 AIVs were identified only at these LPMs. Our findings highlight the public health risks associated with the mixed selling of different poultry species, which should be prohibited.
Materials and methods
Ethics statement
This study was carried out in strict accordance with the recommendations of the Guide for the Care and Use of Laboratory Animals of the Ministry of Science and Technology of the People’s Republic of China. The animal study protocols were approved by the Committee on the Ethics of Laboratory Animals of China Agricultural University (approval number: SKLAB-B-2010-003).
Cell lines
Madin–Darby canine kidney (MDCK) cells and human lung epithelial A549 cells were obtained from the Chinese National Infrastructure of Cell Line Resource. The cells were maintained in Dulbecco’s modified Eagle’s medium (DMEM) (Gibco) supplemented with 10% fetal bovine serum (Gibco), 100 U/mL penicillin, and 100 μg/mL streptomycin at 37 °C under a humidified atmosphere containing 5% CO2.
Sample collection and virus isolation
Between 2017 and 2019, oropharyngeal swabs were collected from chickens, ducks, geese, quails, pigeons, and partridges at LPMs in Guangdong province, China. Viruses were isolated using 10-day-old specific-pathogen-free (SPF) chicken eggs and identified serologically with antisera to each known influenza virus subtype. At least one isolate from each positive sampling occasion was sequenced. The viruses were stored in a −80 °C freezer, and the viral stocks were expanded in SPF chicken eggs.
Phylogenetic, genotype, and molecular analyses
The genomic RNA segments of viruses were extracted using an RNeasy mini kit (Qiagen, Valencia, CA, USA). Reverse transcription and PCR were carried out using primers specific for each viral gene. The exact primer sequences and amplification conditions are available from the authors upon request. PCR products from all eight viral segments were gel purified using the QIAquick PCR purification kit (QIAGEN, Valencia, CA, USA) and sequenced at the Beijing Genomics Institute, China. DNA sequences were assembled and edited using the Lasergene sequence analysis software package (DNASTAR, Madison, WI, USA) and multiple sequence alignment was performed using ClustalW. RAxML was used to construct maximum likelihood phylogenies for each segment [Citation33] via the CIPRES Science Gateway with 1,000 bootstrap replicates. GTRGAMMA + I was used as the nucleotide substitution model. Classification of the phylogenetic trees and genotypes was performed as described previously [Citation34,Citation35].
Antigenic analysis
The antigenic characterization of 29 H9N2 viruses, isolated in China in this and previous studies, was performed using hemagglutination inhibition (HI) assays. Antisera from chickens infected with H9N2 viruses of different hemagglutinin (HA) lineages and years of isolation were used to characterize the viruses; the following viruses were included: Qa/HK/G1/97, Sw/SD/3/03, Ck/SH/F/98, Ck/BJ/3/99, Ck/SD/ZB/07, Ck/GD/01/11, Ck/HeB/0617/07, Ck/JL/0519/12, Ck/JS/TS/10, Ck/SD/zc4/12, Ck/TJ/1/15, Ck/SD/0202-2/15, Ck/HeB/M0530-1/17, Qa/GD/8/17, and Ck/GD/23/18. The HI test was performed as previously described [Citation36,Citation37], with a starting serum dilution of 1:10. Antigenic cartography was performed with the program AntigenMap (https://sysbio.missouri.edu/software/AntigenMap), which uses matrix completion multidimensional scaling to map HI titers in three dimensions [Citation38].
Chicken and quail studies
Six-week-old SPF chickens were obtained from the Boehringer Ingelheim Animal Health Care Corporation in Beijing, China. Four-week-old quails (Deling Quail Farm, Beijing, China), which were serologically negative for influenza viruses, were used in this study. Six chickens or quails per group were infected intranasally with a 106 50% egg infectious dose (EID50) of each stock virus in a 200 μL volume and were observed for 7 days. At 1 d post inoculation (dpi), the inoculated chickens or quails were housed together with the same avian species. Tracheal swabs from each avian species were taken at 3, 5, and 7 dpi for viral titer quantification; the viral titer detection limit was 100.75 EID50/mL. Three chickens or quails per group were euthanized at 3 dpi, and the lungs were collected for viral titration.
Viral titration and growth kinetics in cells
The median tissue culture infective dose (TCID50) was determined in MDCK cells by inoculation of 10-fold serially diluted viruses at 37 °C for 72 h. The TCID50 value was calculated using the Reed–Muench method [Citation39]. Multistep replication kinetics were determined using A549 cells, which were infected with viruses at a multiplicity of infection (MOI) of 0.01. The cells were suspended in serum-free DMEM containing 1 μg/mL tosylsulfonyl phenylalanyl chloromethyl ketone-trypsin (Sigma-Aldrich) and incubated at 37 °C. The supernatants were sampled at 12, 24, 36, 48, 60, and 72 h post inoculation (hpi) and titrated by inoculating MDCK cells into 96-well plates. Three independent experiments were performed.
Ferret studies
Six-month-old female ferrets (Wuxi Cay Ferret Farm, Jiangsu, China), which were serologically negative for influenza viruses, were used in this study. The animals were anesthetized via intramuscular injection of ketamine (20 mg/kg of body weight) and xylazine (1 mg/kg) and infected intranasally with 106 TCID50 of each test virus in a 500 μL volume (250 μL per nostril). A group of three ferrets was infected intranasally with 106 TCID50 of each virus. Twenty-four hours later, the three inoculated animals were individually paired and cohoused with a direct-contact ferret. An aerosol-spread animal was also housed in a wire frame cage adjacent to the infected ferret. Nasal washes were collected at 2-day intervals (beginning at 2 dpi) and titrated in MDCK cells. The ambient conditions for these experiments were 20–22°C and 30%–40% relative humidity. The airflow in the isolator was horizontal at a speed of 0.1 m/s; the airflow direction was from the inoculated animals toward the exposed animals.
Statistical analyses
All statistical analyses were performed using GraphPad Prism software version 5.00 (GraphPad Software Inc., San Diego, CA, USA). Statistically significant differences between the experimental groups were determined by analysis of variance (ANOVA). Differences were considered statistically significant at a P-value of < 0.05.
Accession numbers
The viral nucleotide sequences are available from the Global Initiative on Sharing All Influenza Data database (GISAID) using the accession numbers EPI 2270654 to EPI2273109.
Results
H9N2 AIVs were dominant in chickens and minor poultry species at LPMs
During the study period (from June 2017 to October 2019), we performed oropharyngeal swab sampling (once a month) of poultry at a wholesale LPM and nine retail LPMs; the poultry sold at the retail LPMs originated from the wholesale LPM monitored in this study. A total of 1,648 samples (1,162 chickens, 201 quails, 163 ducks, 79 pigeons, 33 partridges, and 10 geese) were collected; 325 samples (19.7%) were identified as AIV-positive, with the highest isolation rate being observed during the winter season (December to February) (Figure S1). Four influenza virus subtypes were identified, H9N2 (n = 310), H5N6 (n = 6), H6N2 (n = 4) and H6N6 (n = 5), of which H9N2 was the dominant subtype. Additionally, the mean H9N2 isolation rates increased annually, from 9.1% in 2017 to 23.3% in 2019 ((A)). The AIV isolation rates were the highest for quails (64.7%; 64.2% H9N2 and 0.5% H6N6), followed by partridges (30.3%; 27.3% H9N2 and 3.0% H6N6), chickens (14.1%; 14.0% H9N2 and 0.1% H6N6), ducks (11.0%; 4.3% H9N2, 3.7% H5N6, 1.8% H6N2, and 1.2% H6N6), geese (10.0%; all H6N2), and pigeons (2.5%; all H9N2) ((B)). The AIV detection rate was much higher at the retail LPMs than at the wholesale LPM (24.6% [260/1059] vs. 10.9% [64/589]). The subtype diversity of AIVs was also higher at the retail LPMs than at the wholesale LPM. Moreover, H5N6 and H6N2 were only isolated at the retail LPMs. The Chinese government stipulates that areas designated for selling waterfowl should be separated from those used to sell other types of poultry at LPMs. However, we observed that some stalls (3/9) of retail LPMs practiced mixed selling of waterfowl and terrestrial poultry. Of note, H6N6 AIVs were only detected in ducks from the wholesale LPM, whereas H6N6 AIVs were identified in several poultry species (including ducks, chickens, quails, and partridges) at stalls of retail LPMs selling both waterfowl and terrestrial poultry. This indicated that the mixed selling of waterfowl and terrestrial poultry might cause the transmission of H6N6 AIVs from ducks to terrestrial poultry species.
Figure 1. Subtype proportions and host species distributions of AIVs in Guangdong province, China. (A) AIV-positive rates between 2017 and 2019. (B) Proportions of AIV subtypes in samples from different poultry species. The numbers of oropharyngeal swabs collected from chickens, quails, ducks, pigeons, partridges, and geese were 1162, 201, 163, 79, 33, and 10, respectively.
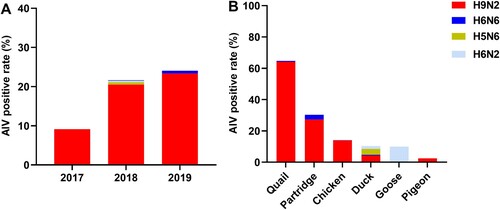
HA genes from BJ/94-like and G1-like lineages were co-circulating among chicken and quails
H9N2 was the dominant AIV subtype at LPMs, and therefore we further performed a phylogenetic analysis of the 310 H9N2 viruses for which full genome sequences were obtained using RAxML, along with all complete genomes of Chinese H9N2 submitted between 1994 and 2022 available in GenBank and GISAID (Figure S2). The HA phylogeny revealed that 165 of our H9N2 AIVs belonged to the BJ/94-like lineage (chickens [83.6%], quails [10.9%], ducks [3.0%], partridges [1.8%], and pigeons [0.6%]), while the remaining 145 isolates were closely related to the G1-like lineage viruses (quails [76.6%], chickens [17.2%], partridges [4.1%], ducks [1.4%], and pigeons [0.7%]) ((A)). Notably, the HA genes of most chicken H9N2 AIVs identified in previous studies and at the wholesale LPM in the present study belonged to the BJ/94-like lineage. Meanwhile, 25 of the 146 (17.1%) chicken H9N2 AIVs identified at the retail LPMs in this study were G1-like. The neuraminidase (NA) gene of the H9N2 AIVs that we had characterized in this study had also diverged into the BJ/94-like and G1-like lineages ((B)). The six internal genes of all our H9N2 isolates possessed the same constellation as the G57 genotype H9N2 AIVs, which were prevalent in chickens.
Figure 2. Phylogenetic analyses of H9N2 AIVs. (A) Host distribution of BJ/94-like and G1-like viruses; different colors represent different hosts. (B) Phylogenetic tree showing the HA (left) and NA (right) genes of the isolated H9N2 AIVs. 165 and 145 HA genes belonged to the BJ/94-like and G1-like lineages respectively. 207 and 103 NA genes belonged to the BJ/94-like and G1-like lineages, respectively. Red represents the BJ/94-like lineage and blue represents the G1-like lineage. Gray lines connect the HA and NA genes of the same isolate. (C) Host distribution of different genotypes at different LPMs; different colors represent different hosts.
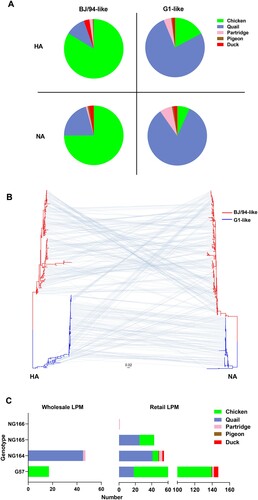
We next defined the AIV genotypes according to the lineage classification of phylogenetic trees. Four genotypes, including G57 and three novel genotypes (NG164, NG165, and NG166) which have not been recognized before, were identified (Table S1). The HA-NA gene combination of the G57 genotype was BJ/94::BJ/94, while that of the NG164 genotype was G1::G1.The HA-NA gene combination of the NG165 genotype was G1::BJ/94, which has not been reported previously, while that of NG166 was BJ/94::G1. The proportion of G57 was highest in the H9N2 strains (n = 164, 52.9%), including n = 138 from chickens, n = 18 from quails, n = 5 from ducks, n = 2 from partridges, and n = 1 from pigeons. NG164 (n = 102, 32.9%) was most prevalent in quails (n = 86), but it was also identified in chickens from the retail LPMs (n = 7), partridges (n = 6), ducks (n = 2) and pigeons (n = 1) ((C)). Notably, all NG165 viruses were isolated at retail LPMs and comprised 13.9% (n = 25 quails and n = 18 chickens) of all the H9N2 AIVs identified. Only one virus from a partridge at a retail LPM had a NG166 genotype.
These results suggested that chickens and quails were the major sources of H9N2 viruses at LPMs. Therefore, we next performed a genotypic evolution analysis of H9N2 viruses isolated from these hosts. In 2017, G57 and NG164 were the predominant genotypes detected in chickens and quails, respectively (). At the wholesale LPM, the H9N2 virus genotype isolated from chickens was G57 and that from quails was NG164. Of note, at retail LPMs, the proportion of quails infected with G57 viruses was 21.4% (n = 18) and that of chickens infected with NG164 viruses was 4.9% (n = 7). In 2018, the NA genes of some NG164 viruses at retail LPMs were replaced by those of the BJ/94-like lineage, resulting in the formation of an NG165 genotype. This then became the dominant genotype in quails at retail LPMs in 2019. Additionally, the isolation rate of NG165 in chickens at retail LPMs had gradually increased since 2018. These results indicated that H9N2 AIVs were frequently reassorted and were involved in a complex system of two-way transmission between different poultry species at retail LPMs.
Figure 3. Schematic of evolutionary pathways of isolated H9N2 AIVs. The eight gene segments (depicted using horizontal bars from the top to the bottom of the virion) encode: basic polymerase 2 (PB2), basic polymerase 1 (PB1), acidic polymerase (PA), HA, nucleoprotein (NP), NA, matrix (M), and nonstructural protein (NS); different colors represent different viral lineages and the arrow thickness is the approximate number of H9N2 isolates in each pathway.
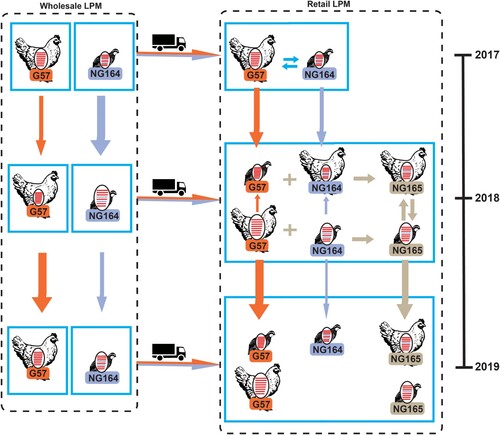
Genotypic diversity increased at retail LPMs
Hence, we found that the retail LPMs not only harbored more genotypes than the wholesale LPM, but the host ranges, even those of the same genotypes, were also more diverse at retail LPMs than those at wholesale LPM. Studies have demonstrated that influenza virus adaptation to new hosts involves a stepwise accumulation of potentiating mutations that favor the emergence of a particular adaptive mutation [Citation40,Citation41]. Thus, we further investigated whether the mixed selling of different poultry species at retail LPMs increased the genetic diversity of viruses, even those with the same genotype. A pairwise identity comparison of viral genes showed that both the G57 and NG164 genotypes from retail LPMs had a much greater genetic diversity than those from the wholesale LPM (). The genes from retail LPMs assumed more heterogeneous patterns, in which more genes possessed greater diversity (Figure S3). Our findings demonstrated that, even for gene segments belonging to the same lineage, the genetic diversity of H9N2 viruses was greater at the retail LPMs than at the wholesale LPM.
Table 1. Sequence identities of H9N2 AIVs isolated at different LPMs.
G1-like lineage H9N2 AIVs formed a new antigenic group
To understand the antigenic evolution of H9N2 AIVs, a total of 16 representative isolated H9N2 AIVs were investigated using a panel of polyclonal sera raised against H9 viruses with previously identified antigenic groups (Table S2). A numerical analysis of HI titers was conducted to visualize antigenic variation, revealing eight distinct groups (). One group included all of the BJ/94-like lineage H9N2 AIVs, which reacted well with the antisera against the Ck/HeB/M0530-1/17 or Ck/GD/23/18 viruses but showed low or moderate reactivity to the group A–F antisera. This reaction pattern was similar to that of the subgroup G2 viruses isolated in 2016 and 2017. Notably, these G1-like viruses (isolated in 2016 and 2017) exhibited little or no antigenic cross-reactivity to antiserum against Qa/HK/G1/97, indicating that the G1-like lineage H9N2 AIVs had significant antigenic variation and belonged to a new antigenic group (group H).
Figure 4. Antigenic cartography representation of HI data generated using a panel of antisera. Detailed HI data are presented in Table S2. The map was produced using AntigenMap (http://sysbio.cvm.msstate.edu/AntigenMap). One unit (cell) represents a two-fold change in the HI assay result.
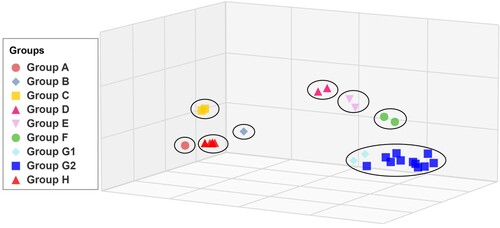
Replication and transmission of isolated H9N2 viruses in chickens and quails
After identifying the G57, NG164, and NG165 genotypes co-circulating in chickens and quails, we further evaluated their virulence and transmission in these avian species. Groups of six chickens or quails were intranasally inoculated with 106 EID50 of A/chicken/Zhejiang/HJ/2007(Ck/ZJ/HJ/07,G57), A/chicken/Beijing/xy1125/2014(Ck/BJ/xy1125/14,G57), A/chicken/Guangdong/53/2018(Ck/GD/53/18,G57), A/chicken/Guangdong/72/2019(Ck/GD/72/19,G57), A/quail/Guangdong/177/2018(Qa/GD/177/18,NG164), A/chicken/Guangdong/10/2018(Ck/GD/10/18,NG164), A/quail/Guangdong/254/2019(Qa/GD/254/19,NG165), or A/chicken/Guangdong/133/19 (Ck/GD/133/19,NG165). At 24 hpi, the birds were then cohoused with contact birds of the same species. We found that the replication ability of G57 H9N2 AIVs in the chickens and quails gradually increased ((A, B)). Shedding of the recently isolated G57 viruses (Ck/GD/53/18 and Ck/GD/72/19) from the trachea lasted for at least 7 days, while early G57 viruses (Ck/ZJ/HJ/07 and Ck/BJ/xy1125/14) shedding lasted for 5 days. At each time point of tracheal sampling, the recently isolated G57 viruses produced more progeny than the early G57 viruses; and the difference in virus output between the early viruses and the recently isolated viruses was over 100-fold at 3 and 5 dpi ((A, B)). There was a high level of replication by the recently isolated G57 viruses from the lungs of all infected chickens and quails at 3 dpi ((C, D)). Similar to its replication in the inoculated group, the recently isolated G57 genotypes also exhibited improved infection with higher viral titers in the contact group ((A, B)). These results suggested that the replication and transmission of the G57 viruses had significantly improved in chickens and quails in recent years.
Figure 5. Replication and transmission of G57 viruses in chickens and quails. The viral titers of G57 H9N2 AIVs were recovered from chicken or quail trachea (A and B, respectively) and lungs (C and D, respectively). Groups of six chickens or quails were inoculated with 106 EID50 of the indicated virus; tracheal swabs from three chickens or quails per group were taken at 3, 5, and 7 dpi and lungs were harvested from chickens or quails at 3 dpi for viral titration. All data are representative or presented as the mean ± standard deviation of the mean (SEM) of three independent experiments, unless specified otherwise. Statistical significance relative to Ck/ZJ/HJ/07 or Ck/BJ/xy1125/14 was assessed using two-way ANOVA (*P < 0.05, **P < 0.01).
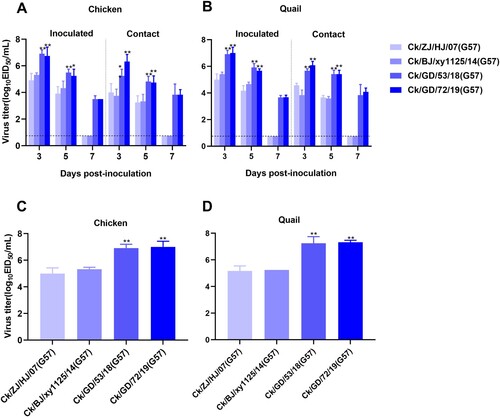
Genetic reassortment is advantageous to viruses because it can rapidly create high-fitness genotypes. To determine the effect of reassortment on the infectivity of G1-like lineage viruses, the replication and transmission of NG164 and NG165 viruses were compared in chickens and quails. After inoculation, all the tested viruses had a 100% isolation rate in the trachea ((A, B)). However, shedding of the two NG165 viruses from the trachea lasted for at least 7 days, while that of the two NG164 viruses lasted for 5 days. Moreover, the NG165 viruses produced significantly higher titers than the NG164 viruses (P < 0.05; (C, D)) in the lung tissue. The viral titers of the NG165 contact group were also significantly higher than those of the NG164 contact group (P < 0.01). Furthermore, both of the NG165 viruses were transmitted more efficiently than the NG164 viruses in chickens and quails ((C, D)). Collectively, these findings suggest that the improved replication and transmission of NG165 viruses (vs. NG164 viruses) in chickens and quails may have led to the replacement of NG164 viruses with NG165 ones at retail LPMs. Thus, alarmingly, the mixed selling of different poultry at retail LPMs has facilitated the emergence of novel AIVs.
Figure 6. Replication and transmission of NG166 and NG184 viruses in chickens and quails. The viral titers of H9N2 AIVs were recovered from chicken or quail trachea (A and B, respectively) and lungs (C and D, respectively). Groups of six chickens or quails were inoculated with 106 EID50 of the indicated virus; tracheal swabs from three chickens or quails per group were taken at 3, 5, and 7 dpi, and lungs were harvested from chickens or quails at 3 dpi for viral titration. All data are representative or presented as the mean ± standard deviation of the mean (SEM) of three independent experiments, unless specified otherwise. Statistical significance relative to Qa/GD/177/18 or Ck/GD/10/18 was assessed using two-way ANOVA (*P < 0.05, **P < 0.01).
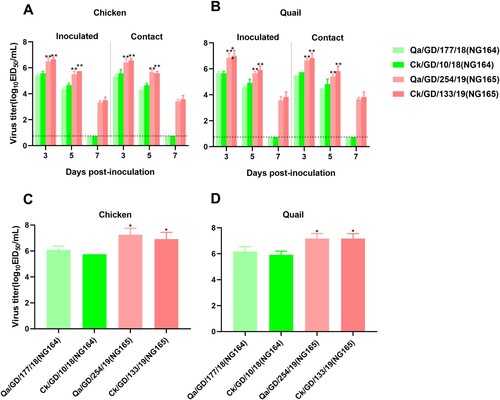
Replication and transmission of isolated H9N2 viruses in mammals
To compare the adaptation of H9N2 AIVs belonging to different genotypes to mammalian species, we first performed a multistep replication kinetics assays using Ck/ZJ/HJ/07 (G57), Ck/BJ/xy1125/14(G57), Ck/GD/53/18(G57), Ck/GD/72/19(G57), Qa/GD/177/18(NG164), Ck/GD/10/18(NG164), Qa/GD/254/19(NG165), and Ck/GD/133/19(NG165) with an MOI of 0.01 in alveolar epithelial (A549) cells. Two G57 viruses (isolated in 2018 and 2019) produced more viable viral progeny at 24–72 hpi than the earlier G57 strains (P < 0.01) ((A)). NG165 viruses produced more infectious viruses than the NG164 viruses at 24–60 hpi (P < 0.01), reaching a peak titer of 107.25 TCID50/mL. By contrast, the two NG164 viruses had peak titers of 105.75 TCID50/mL ((A)).
Figure 7. Mammalian adaptation of isolated H9N2 viruses. (A) Multistep growth curves of H9N2 AIVs in A549 cells inoculated at an MOI of 0.01. (B) Horizontal transmission and respiratory droplet transmission of H9N2 AIVs in ferrets; the dashed lines indicate the lower limit of viral detection. At 1 dpi, one inoculated ferret was moved to a clean isolator containing a naive ferret for direct contact. Another naive ferret was placed in the same isolator but separated so that no direct contact was possible, with transmission instead only permissible via the aspiration of aerosol droplets. To monitor viral shedding, nasal washes were collected from all animals every other day for 14 days. In cases when no virus was isolated from any of the three ferrets, no data were displayed. Each color bar represents the viral titer of an individual animal. All data are representative or presented as the mean ± standard deviation of the mean (SEM) of three independent experiments. Statistical significance relative to Ck/ZJ/HJ/07 and Ck/BJ/xy1125/14 or Qa/GD/177/18 and Ck/GD/10/18 was assessed using two-way ANOVA (*P < 0.05, **P < 0.01).
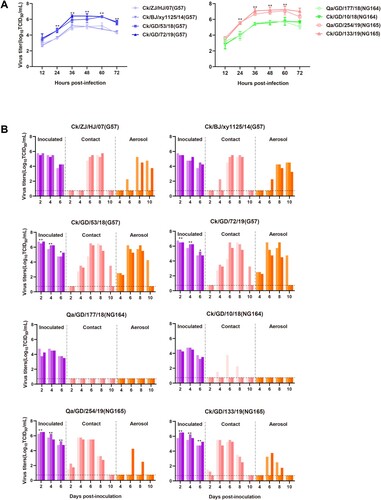
Ferrets have been widely used as experimental models to study human infection and the transmission of influenza viruses in mammals. A group of three ferrets was infected intranasally with 106 TCID50 of the above viruses. Twenty-four hours later, the three inoculated animals were individually paired and cohoused with a direct-contact ferret. In addition, another ferret was housed in a wire frame cage adjacent to the infected ferret to monitor AIV transmission via aerosol droplets. Nasal washes from the directly infected and transmission animals were collected every 2 days (beginning at 2 dpi) for the detection of viral shedding. The peak titers of Ck/GD/53/18(G57) and Ck/GD/72/19(G57) were significantly higher than those of Ck/ZJ/HJ/07(G57) and Ck/BJ/xy1125/14 (G57) in the inoculated ferrets (P < 0.05) at 2, 4, and 6 dpi ((B)). Viruses were detected in nasal washes from the three contact ferrets at 4–10 dpi in the Ck/GD/53/18(G57) and Ck/GD/72/19(G57) groups. By contrast, the Ck/ZJ/HJ/07(G57) virus was first detected in nasal washes of the three contact ferrets at 6 dpi, whereas Ck/BJ/xy1125/14(G57) was detected in only one contact ferret at 4 dpi ((B)). Of note, viruses were detected in all three aerosol-spread ferrets in the Ck/GD/53/18(G57) and Ck/GD/72/19(G57) groups at 4 dpi. By contrast, viruses were detected in only two of three aerosol-spread ferrets in the Ck/ZJ/HJ/07(G57) group and in only one aerosol-spread ferret in the Ck/BJ/xy1125/14(G57) group at 6 dpi ((B)).
Viruses with HA genes belonging to the G1-like lineage were next compared. We found that the viral titers in the nasal washes of the Qa/GD/254/19(NG165)- or Ck/GD/133/19(NG165)-infected ferrets were higher than those of Qa/GD/177/18(NG164)- or Ck/GD/10/18(NG164)-infected ferrets at 2, 4, and 6 dpi (P < 0.01). No viruses were detected in the Qa/GD/177/18 (NG164) contact ferrets. However, they were detected in one direct contact ferret in the Ck/GD/10/18 (NG164) group. Of note, these viruses are not transmittable by aerosol respiratory droplets. The Qa/GD/254/19(NG165) and Ck/GD/133/19(NG165) viruses were detected in all three direct contact ferrets and in one and two of the aerosol-spread ferrets, respectively ((B)). Analysis of known mammalian adaptation markers of these viruses showed that more amino acid changes related to the enhanced ability of the H9 subtype to bind to human receptors and the increased replication or virulence of AIVs in mammals were detected in the G57 viruses isolated in 2018 and 2019 (Table S3). The NG164 viruses had NA genes belonging to the G1-like lineage, while the NA genes of the NG165 viruses belonged to clade 2 of BJ/94-like lineage, which possessed deletion in the stalk region of NA protein (amino acids 62–64) associated with mammalian adaption [Citation42]. These results suggested that the mammalian adaptation of G57 viruses had improved in recent years, and that the NG165 viruses were more adapted to mammalian hosts than the NG164 strains.
Discussion
Here, we performed an AIV surveillance study at two types of LPMs in Guangdong province from 2017 to 2019 to elucidate the role of poultry vending practices in the prevalence and evolution of AIVs. We identified higher AIV isolation rates and increased subtype diversity at retail LPMs than at the wholesale LPM. The H9N2 was still the AIV predominant subtype at LPMs, with chickens and quails being its primary hosts. The genetic diversity of the H9N2 viruses was higher at the retail LPMs, where they participated in a complex system of two-way transmission between different poultry species. The reassortant genotypes—NG165 and NG166—were only identified at the retail LPMs. Additionally, the results of experiments in poultry and mammalian models showed that the replication and transmission of the G57 genotype H9N2 AIVs had increased in recent years. Moreover, we found that the NG165 viruses were more adaptive than the NG164 viruses to both avian and mammalian hosts. These results indicate that the mixed selling of different poultry species at retail LPMs might facilitate the emergence of novel AIVs that pose a higher threat to public health.
Since the first reported outbreak of H9N2 AIVs in chickens from Guangdong province in 1992 [Citation43], H9N2 viruses have spread across China, where they are currently endemic. This has resulted in great economic losses (e.g. from reduced egg production) or high mortality rates (especially due to co-infections with other pathogens) [Citation36,Citation44]. Our research and other studies have found that, since 2016, H9N2 has replaced H5N6 and H7N9 to become the dominant AIV subtype in both chickens and ducks in China [Citation7]. Phylogenetic studies have revealed that the HA genes of H9N2 AIVs can be categorized into the Eurasian or American avian lineages. The Eurasian avian lineage encompasses three distinct sub-lineages, including A/chicken/Beijing/1/94-like (BJ/94-like), A/quail/Hong Kong/G1/97-like (G1-like), and A/duck/Hong Kong/Y439/97 (Y439-like) [Citation9]. Studies have demonstrated that the HA and NA genes of most H9N2 viruses in China belong to the BJ/94-like (Ck/Beijing/1/94-like) and G1-like (Qa/HK/G1/97-like) lineages, which have become the predominant lineages in chickens and minor poultry species (mainly quails), respectively, while the internal genes show greater diversity [Citation11,Citation34–36,Citation45]. However, the surveillance of AIVs in minor poultry species after 2005 has been sporadic. In our study, three novel genotypes were identified: NG164, NG165, and NG166. NG164 and NG165 were the predominant H9N2 AIV genotypes in quails, while only one virus isolated from a partridge at a retail LPM had a NG166 genotype. Moreover, the antigenicity of currently prevalent G1-like lineage H9N2 AIVs was significantly different from that of A/quail/Hong Kong/G1/97.
LPMs are recognized as major sites for the maintenance, transmission, amplification, and dissemination of AIVs [Citation46–48]. Thus, they pose a public health risk of zoonotic AIV spill-over to humans through contact with infected poultry or environmental contamination [Citation49,Citation50]. The RNA detection rates of AIVs from environmental samples are much higher at retail markets than at wholesale markets [Citation51]. At retail LPMs, H5 and H7 viruses are most frequently detected in the poultry selling and slaughtering areas, whereas the highly prevalent H9 viruses are detected in poultry holding, slaughtering, and selling areas [Citation32]. Moreover, the transmission risk of AIVs progressively increases along the poultry supply chain toward retail LPMs. Retail LPMs are the endpoints of live poultry trading. Poultry workers in these settings have a higher seroprevalence of H9 AIV than those in other sections of the poultry supply chain [Citation52]. Previous studies have revealed that two-way interspecies transmission occurs between different types of poultry and that reassortment events among established viral lineages (e.g. H5N1 and H9N2 viruses) are mostly responsible for the high genetic diversity of H9N2 and H5N1 variants in southern China [Citation11]. However, the role of mixed selling of different poultry species at LPMs in the emergence of novel reassortant AIVs has not been demonstrated. Therefore, we selected a wholesale LPM and nine retail LPMs (which sourced poultry from the wholesale LPM monitored in this study) to perform an AIV surveillance study and analyze the evolution and epidemiology of AIVs in these different settings. The comparison of multiple wholesale LPMs and their associated retail LPMs in parallel would have strengthened our findings. However, during the study planning phase, we noticed that some retail LPMs purchased poultry from several wholesale LPMs which might have introduced sampling bias, and only one wholesale LPM and their associated nine retail LPMs can meet our requirements. We found the AIV detection rate to be much higher at the retail LPMs than at the wholesale LPM. We further performed a genotypic evolution analysis of H9N2 viruses isolated from the different LPMs. We found that the H9N2 AIVs isolated from chickens and quails at the wholesale LPM only belonged to the G57 and NG164 genotypes, respectively. However, the G57, NG164, and NG165 viruses were identified in both chickens and quails at the retail LPMs. The reassortant genotypes NG165 and NG166 were identified only at retail LPMs. Moreover, a pairwise identity comparison of viral genes revealed that the genetic diversity of viruses (for both G57 and NG164 genotypes) from retail LPMs was much greater than that of viruses from the wholesale LPM. Thus, our findings confirmed the suspicion that the mixed selling of different poultry species facilitated two-way interspecies transmission and increased the genotypic diversity of AIVs. However, since the data is from only the one wholesale LPM and nine retail LPMs which may limit the reliability of the conclusion. Thus, the role of the mixed selling of different poultry species at LPMs need to be further confirmed.
Since the H7N9 outbreak in 2013, human infections with novel subtypes of AIVs, including outbreaks of H6N1, H10N8, H5N6, H7N4, H10N3, and H3N8, have frequently occurred in China. Moreover, the number of novel AIV subtypes identified in humans in the past decade (i.e. between 2013 and 2022) is three times higher than that before 2013. Notably, most of the emerging AIVs found infecting humans have share a common feature: almost all their internal gene segments were derived from the H9N2 G57 genotype. Moreover, H9N2 AIVs have already acquired the ability to break through species barriers and directly invade humans without intermediate hosts. Accordingly, human H9N2 infection cases have continued to increase since 2013 [Citation8]. H9N2 AIVs isolated from poultry have acquired specificity human-like receptor-binding specificity, and their replication ability and transmissibility in mammalian models have also increased [Citation53]. We found that two G57 viruses isolated in 2018 and 2019 showed significantly increased replication ability in A549 cells and ferrets, compared with earlier G57 strains. Notably, these recent G57 viral isolates were transmitted to all three exposed ferrets via respiratory droplets, whereas only one of the three aerosol-spread ferrets became infected with earlier G57 strains. Molecular characterization showed that the G57 H9N2 viruses isolated in 2018 and 2019 had acquired more known mammalian adaptation markers. Additionally, the replication levels of NG165 in A549 cells and infected ferrets were higher than those of NG164, and transmission via aerosol was observed in the NG165 group but not the NG164 group of animals. Moreover, the NG164 viruses had NA genes belonging to G1-like lineage, while the NA genes of the NG165 viruses belonged to clade 2 of BJ/94-like lineage, which has a deletion in the stalk region of the NA protein (amino acids 62–64) associated with mammalian adaption[Citation42]. Thus, the mixed selling of different poultry species at retail LPMs has also contributed to the emergence of novel reassortant AIVs that are more adaptive to mammalian hosts.
In early 2013, after H7N9 was identified as the causative agent of the emerging flu epidemic, the source of the virus was immediately traced to LPMs. The Chinese government reacted quickly by calling for the immediate shutdown of LPMs in several cities to control the spread of the virus. However, since their long-term closure was deemed economically unviable, the markets soon reopened in some regions [Citation25]. LPMs still exist in China, especially in certain provinces of southern China, where the inhabitants prefer to buy live poultry [Citation54]. Owing to LPMs being transmission sources and potential incubators of human AIVs, the Ministry of Agriculture, the Ministry of Health, and the State Administration for Industry and Commerce jointly issued measures to separate waterfowl selling areas from those used to sell other types of poultry. However, we observed that the mixed selling of waterfowl and terrestrial poultry was still practiced at some stalls of retail LPMs. Of note, the H6N6 AIVs from terrestrial poultry (including chickens, quails, and partridges) were only collected from these stalls. Therefore, mixed poultry selling at retail LPMs provides a favorable environment for the transmission of AIVs from waterfowl to terrestrial poultry species. Whether reassortment between waterfowl and terrestrial poultry AIVs could occur in this setting needs further validation. Additionally, although government-led measures to separate aquatic and terrestrial poultry can effectively prevent the transmission of AIVs from waterfowl to terrestrial poultry, they cannot prevent the transmission of AIVs among different terrestrial poultry species. Indeed, our study demonstrated that the practice of selling a mix of terrestrial poultry species facilitated the emergence of novel reassortant AIVs, which had more efficient replication and transmission ability in both avian and mammalian hosts. Thus, we recommend that the areas selling different poultry species are adequately separated or adopting other measures to prevent the transmission of AIVs between different poultry species and the emergence of novel viral mutants threating public health.
Author contributions
Z.W., H.L., Y.L., ZL.W., H.A., Q.T., L.L., H.S., J.P., and Y.S. conducted the experiments; Z.W., M.Z., L.R, M.B, W.F, J.L., and Y.S. analyzed the data; Z.W. and Y.S. wrote the manuscript.
Supplemental Material
Download Zip (27.9 MB)Acknowledgements
We thank the authors and laboratories who submitted sequences to the GISAID EpiFlu Database and NCBI Database.
Disclosure statement
No potential conflict of interest was reported by the authors.
Additional information
Funding
References
- Rajagopal S, Treanor J. Pandemic (avian) influenza. Semin Respir Crit Care Med. 2007 Apr;28(2):159–170.
- Long JS, Mistry B, Haslam SM, et al. Host and viral determinants of influenza A virus species specificity. Nat Rev Microbiol. 2019 Jan;17(2):67–81.
- Zhang J, Ye H, Liu Y, et al. Resurgence of H5N6 avian influenza virus in 2021 poses new threat to public health. Lancet Microbe. 2022 Aug;3(8):e558.
- Yang R, Sun H, Gao F, et al. Human infection of avian influenza A H3N8 virus and the viral origins: a descriptive study. Lancet Microbe. 2022 Nov;3(11):e824–e834.
- Bi Y, Li J, Shi W. The time is now: a call to contain H9N2 avian influenza viruses. Lancet Microbe. 2022 Nov;3(11):e804–e805.
- Zhang J, Huang L, Liao M, et al. H9N2 avian influenza viruses: challenges and the way forward. Lancet Microbe. 2023 Feb;4(2):e70–e71.
- Bi Y, Li J, Li S, et al. Dominant subtype switch in avian influenza viruses during 2016-2019 in China. Nat Commun. 2020 Nov 20;11(1):5909.
- Song W, Qin K. Human-infecting influenza A (H9N2) virus: A forgotten potential pandemic strain? Zoonoses Public Health. 2020 May;67(3):203–212.
- Carnaccini S, Perez DR. H9 influenza viruses: An emerging challenge. Cold Spring Harb Perspect Med. 2020 Jun 1;10(6):a038588.
- Sun Y, Qin K, Wang J, et al. High genetic compatibility and increased pathogenicity of reassortants derived from avian H9N2 and pandemic H1N1/2009 influenza viruses. Proc Natl Acad Sci U S A. 2011 Mar 8;108(10):4164–4169.
- Xu KM, Smith GJ, Bahl J, et al. The genesis and evolution of H9N2 influenza viruses in poultry from southern China, 2000 to 2005. J Virol. 2007 Oct;81(19):10389–10401.
- Wang B, Chen Q, Chen Z. Complete genome sequence of an H9N2 avian influenza virus isolated from egret in Lake Dongting wetland. J Virol. 2012 Nov;86(21):11939.
- Guo J, Wang Y, Zhao C, et al. Molecular characterization, receptor binding property, and replication in chickens and mice of H9N2 avian influenza viruses isolated from chickens, peafowls, and wild birds in eastern China. Emerg Microbes Infect. 2021 Dec;10(1):2098–2112.
- He WT, Hou X, Zhao J, et al. Virome characterization of game animals in China reveals a spectrum of emerging pathogens. Cell. 2022 Mar 31;185(7):1117–1129.
- Kim JK, Negovetich NJ, Forrest HL, et al. Ducks: the “Trojan horses” of H5N1 influenza. Influenza Other Respir Viruses. 2009 Jul;3(4):121–128.
- Zhou X, Li Y, Wang Y, et al. The role of live poultry movement and live bird market biosecurity in the epidemiology of influenza A (H7N9): A cross-sectional observational study in four eastern China provinces. J Infect. 2015 Oct;71(4):470–479.
- Yamada S, Shinya K, Takada A, et al. Adaptation of a duck influenza A virus in quail. J Virol. 2012 Feb;86(3):1411–1420.
- Wan XF, Dong L, Lan Y, et al. Indications that live poultry markets are a major source of human H5N1 influenza virus infection in China. J Virol. 2011 Dec;85(24):13432–8.
- Fournie G, Guitian J, Desvaux S, et al. Interventions for avian influenza A (H5N1) risk management in live bird market networks. Proc Natl Acad Sci U S A. 2013 May 28;110(22):9177–9182.
- Lam TT, Wang J, Shen Y, et al. The genesis and source of the H7N9 influenza viruses causing human infections in China. Nature. 2013 Oct 10;502(7470):241–244.
- Liu D, Shi W, Shi Y, et al. Origin and diversity of novel avian influenza A H7N9 viruses causing human infection: phylogenetic, structural, and coalescent analyses. Lancet. 2013 Jun 1;381(9881):1926–1932.
- Chen H, Yuan H, Gao R, et al. Clinical and epidemiological characteristics of a fatal case of avian influenza A H10N8 virus infection: a descriptive study. Lancet. 2014 Feb 22;383(9918):714–721.
- Zhang T, Bi Y, Tian H, et al. Human infection with influenza virus A(H10N8) from live poultry markets, People’s Republic of China, 2014. Emerg Infect Dis. 2014 Dec;20(12):2076–2079.
- Peacock THP, James J, Sealy JE, et al. A global perspective on H9N2 avian influenza virus. Viruses. 2019 Jul 5;11(7):620.
- Gao GF. Influenza and the live poultry trade. Science. 2014 Apr 18;344(6181):235.
- Yu H, Wu JT, Cowling BJ, et al. Effect of closure of live poultry markets on poultry-to-person transmission of avian influenza A H7N9 virus: an ecological study. Lancet. 2014 Feb 8;383(9916):541–548.
- Wu J, Lu J, Faria NR, et al. Effect of live poultry market interventions on influenza A(H7N9) virus, guangdong, China. Emerg Infect Dis. 2016 Dec;22(12):2104–2112.
- Liao Q, Lam WT, Leung GM, et al. Live poultry exposure, Guangzhou, People’s Republic of China, 2006. Epidemics. 2009 Dec;1(4):207–212.
- Guan Y, Shortridge KF, Krauss S, et al. H9N2 influenza viruses possessing H5N1-like internal genomes continue to circulate in poultry in southeastern China. J. Virol. 2000 Oct;74(20):9372–9380.
- Guan Y, Shortridge KF, Krauss S, et al. Molecular characterization of H9N2 influenza viruses: were they the donors of the “internal”. genes of H5N1 viruses in Hong Kong? Proc Natl Acad Sci U S A. 1999 Aug 3;96(16):9363–9367.
- Lin YP, Shaw M, Gregory V, et al. Avian-to-human transmission of H9N2 subtype influenza A viruses: relationship between H9N2 and H5N1 human isolates. Proc Natl Acad Sci U S A. 2000 Aug 15;97(17):9654–9658.
- Cheng KL, Wu J, Shen WL, et al. Avian influenza virus detection rates in poultry and environment at live poultry markets, Guangdong, People’s Republic of China. Emerg Infect Dis. 2020 Mar;26(3):591–595.
- Stamatakis A. RAxML version 8: a tool for phylogenetic analysis and post-analysis of large phylogenies. Bioinformatics. 2014 May 1;30(9):1312–1313.
- Pu J, Wang S, Yin Y, et al. Evolution of the H9N2 influenza genotype that facilitated the genesis of the novel H7N9 virus. Proc Natl Acad Sci U S A. 2015 Jan 13;112(2):548–553.
- Li C, Wang S, Bing G, et al. Genetic evolution of influenza H9N2 viruses isolated from various hosts in China from 1994 to 2013. Emerg Microbes Infect. 2017 Nov 29;6(11):e106.
- Sun Y, Pu J, Jiang Z, et al. Genotypic evolution and antigenic drift of H9N2 influenza viruses in China from 1994 to 2008. Vet Microbiol. 2010 Dec 15;146(3-4):215–225.
- Wei Y, Xu G, Zhang G, et al. Antigenic evolution of H9N2 chicken influenza viruses isolated in China during 2009-2013 and selection of a candidate vaccine strain with broad cross-reactivity. Vet Microbiol. 2016;182:1–7.
- Cai Z, Zhang T, Wan XF. A computational framework for influenza antigenic cartography. PLoS Comput Biol. 2010 Oct 7;6(10):e1000949.
- Nemeth B, Fasseeh A, Molnar A, et al. A systematic review of health economic models and utility estimation methods in schizophrenia. Expert Rev Pharmacoecon Outcomes Res. 2018 Jun;18(3):267–275.
- Cauldwell AV, Long JS, Moncorge O, et al. Viral determinants of influenza A virus host range. J Gen Virol. 2014 Jun;95(Pt 6):1193–1210.
- He WT, Wang L, Zhao Y, et al. Adaption and parallel evolution of human-isolated H5 avian influenza viruses. J Infect. 2020 Jun;80(6):630–638.
- Sun Y, Tan Y, Wei K, et al. Amino acid 316 of hemagglutinin and the neuraminidase stalk length influence virulence of H9N2 influenza virus in chickens and mice. J Virol. 2013 Mar;87(5):2963–2968.
- Sun Y, Liu J. H9N2 influenza virus in China: a cause of concern. Protein Cell. 2015 Jan;6(1):18–25.
- Zhao M, Liu Q, Sun Q, et al. Full genome sequence of a natural reassortant H9N2 avian influenza virus isolated from domestic ducks in Jiangsu province, China. Genome Announc. 2013 Jul 18;1(4):e00463–13.
- Pu J, Sun H, Qu Y, et al. M gene reassortment in H9N2 influenza virus promotes early infection and replication: contribution to rising virus prevalence in chickens in China. J Virol. 2017 Apr 15;91(8):e02055–16.
- Fournie G, Guitian FJ, Mangtani P, et al. Impact of the implementation of rest days in live bird markets on the dynamics of H5N1 highly pathogenic avian influenza. J R Soc Interface. 2011 Aug 7;8(61):1079–1089.
- Henning J, Hesterberg UW, Zenal F, et al. Risk factors for H5 avian influenza virus prevalence on urban live bird markets in Jakarta, Indonesia-Evaluation of long-term environmental surveillance data. PLoS One. 2019;14(5):e0216984.
- Zhang R, Lei Z, Liu C, et al. Live poultry feeding and trading network and the transmission of avian influenza A(H5N6) virus in a large city in China, 2014-2015. Int J Infect Dis. 2021 Jul;108:72–80.
- Kung NY, Morris RS, Perkins NR, et al. Risk for infection with highly pathogenic influenza A virus (H5N1) in chickens, Hong Kong, 2002. Emerg Infect Dis. 2007 Mar;13(3):412–418.
- Wang X, Wang Q, Cheng W, et al. Risk factors for avian influenza virus contamination of live poultry markets in Zhejiang, China during the 2015-2016 human influenza season. Sci Rep. 2017 Mar 3;7(2045-2322):42722.
- Yuan J, Lau EH, Li K, et al. Effect of live poultry market closure on avian influenza A(H7N9) virus activity in Guangzhou, People’s Republic of China, 2014. Emerg Infect Dis. 2015 Oct;21(10):1784–1793.
- Wu JY, Lau EH, Yuan J, et al. Transmission risk of avian influenza virus along poultry supply chains in Guangdong, China. J Infect. 2019 Jul;79(1):43–48.
- Li X, Shi J, Guo J, et al. Genetics, receptor binding property, and transmissibility in mammals of naturally isolated H9N2 Avian Influenza viruses. PLoS Pathog. 2014 Nov;10(11):e1004508.
- Peiris JS, Cowling BJ, Wu JT, et al. Interventions to reduce zoonotic and pandemic risks from avian influenza in Asia. Lancet Infect Dis. 2016 Feb;16(2):252–258.