Abstract
Probiotic use is suggested as an alternative to antibiotics when the treatment of poultry gastrointestinal tract microbiota through antibiotic use has been banned in some countries. The objective of this study was to evaluate the effect of previously isolated Lactobacillus salivarius CEL1 and Lactobacillus reuteri CEC3 probiotics on the growth performance, caecal microbial population, and carcass weight of Rhode Island Red (RIR) male chickens. A total of 72, day-old RIR male chicks were randomly assigned into three experimental treatment groups in triplicate. The treatment groups were the control, L. salivarius CEL1 and L. reuteri CEC3. The control group was fed basal chicken feed, and 1 × 108 cfu of L. salivarius CEL1 and L. reuteri CEC3 cultures were added to the feed for the L. salivarius CEL1 and L. reuteri CEC3 groups, respectively. The Lactobacillus, total aerobic bacteria, and Escherichia coli populations in the ceca were also determined. Compared to the control group, supplementation of L. salivarius CEL1 and L. reuteri CEC3 significantly (p < 0.05) increased body weight and body weight gain and the number of E. coli and total aerobic bacteria in the cecum of chickens were reduced. In addition, L. salivarius CEL1 and L. reuteri CEC3 supplementation resulted in significantly high dressing and internal organ weights. However, there were no statistically significant differences in feed intake and feed conversion efficiency between treatments at 42 days of rearing. This study revealed that L. salivarius CEL1 and L. reuteri CEC3 could be considered good potential probiotics for chickens.
REVIEWING EDITOR:
SUBJECTS:
1. Introduction
Poultry is a major asset and key to poverty alleviation, income generation, and market participation, particularly for smallholders and the poor communities of the world (Birhanu et al., Citation2023). The growing population and urbanization drive the worldwide demand for meat and egg products (Mottet & Tempio, Citation2016). Even though the poultry industry is an important economic activity across the world, serious economic losses result when poultry are exposed to stressful conditions, problems related to diseases, and deterioration of environmental conditions. The emergence of a large variety of pathogens and antibiotic-resistant bacteria is the main limiting factor to the productivity of the poultry industry (Lutful Kabir, Citation2009).
Since the 1940s, antibiotics have been widely used at subtherapeutic levels in animal feed as animal growth promoters, as they improve growth and feed conversion rates in some animals and poultry (Chattopadhyay, Citation2014). However, in recent years, the emergence of antibiotic-resistant bacterial populations, the appearance of residual antibiotics in meats, and increasing public demand for organic production have increased interest in searching for alternatives to antibiotics. For these reasons, the management of the gastrointestinal tract (GIT) microflora of poultry through the use of antibiotics has been banned in some countries (Anadón, Citation2006; Harimurti & Hadisaputro, Citation2015).
Probiotics have been defined as ‘a live microbial feed supplement which beneficially affects the host by improving its intestinal microbial balance’. These live microorganisms are non-pathogenic and nontoxic in nature and are favourable to the host’s health when adequately administered through the digestive route (Azzaz et al., Citation2016; FAO/WHO, Citation2001). The use of probiotics is mentioned as an alternative to antibiotics (Alagawany et al., Citation2018; Shin et al., Citation2008). Probiotics research is one of the current alternative research areas to minimize the effect of antibiotics on animals and humans (Mehdi et al., Citation2018). The use of probiotic feed additives is mentioned for the prevention of disease, decrease in mortality, toleration of environmental stress, and enhancement of chicken productivity (Krysiak et al., Citation2021). The addition of probiotics to the diet of livestock and poultry has been found to improve the growth performance of the host, feed conversion efficiency, and immune responses and to help combat enteric pathogens (Dhama et al., Citation2011).
Different microbial species have been used as probiotics, including species of Bacillus, Bifidobacterium, Enterococcus, Escherichia, Lactobacillus, Lactococcus, Streptococcus, a variety of yeast species (mainly Saccharomyces), Pediococcus and undefined mixed cultures (Fijan, Citation2014). Lactobacillus species are commonly used in broiler chickens (Peng et al., Citation2016) in developed countries. Different strains belonging to similar species have different properties, so effects or benefits can be different from one strain to another within the same species (Jha et al., Citation2020).
Potential probiotic bacteria can be isolated from the GIT of adapted chickens and can be used to formulate probiotics. The main objective of this study was to evaluate the effect of previously isolated (Tsega et al., Citation2023) Lactobacillus salivarius CEL1 and Lactobacillus reuteri CEC3 probiotic cultures on the growth performance and caecal microbial population of Rhode Island Red (RIR) male chickens. The in vitro potential probiotic characteristics of these Lactobacillus strains were determined, and these two strains were selected based on their high bile salt tolerance, low pH resistance, high surface hydrophobicity, good antimicrobial activity, and other desired properties of probiotics.
2. Materials and methods
2.1. Ethical approval
Before the beginning of the experiment, ethical approval was obtained from the Research Ethical Committee of the University of Gondar (Ethiopia). All aspects of animal care and experimentation performed in this study conformed to the Guide for Care and Use of Laboratory Animals of the National Institutes of Health (National Research Council, Citation2010) and followed the EEC directive of 2010/63/EU (Directive, Citation2010).
2.2. Probiotics supplemented feed preparation
2.2.1. Production of bacterial cells for probiotics
After in vitro characterization and identification by 16S rRNA gene sequencing, two Lactobacillus strains (L. salivarius CEL1 and L. reuteri CEC3) were selected based on their probiotic potential and propagated in a liquid culture medium. The 16S rRNA gene of the L. salivarius CEL1 and L. reuteri CEC3 strains was published in a public database (GenBank) under the accession number MK764683 and MK764685, respectively. After growing, the bacterial cells were separated from the culture medium by centrifugation at 5000 rpm for 10 min. Then, the pellets were washed two times by using a sterile saline solution. The clean pellet was weighed and resuspended in a sterile saline solution. The dilution of the pellet was 1 g/30 ml of saline solution.
2.2.2. Preparation of experimental feeds
The selected Lactobacillus strains were used to prepare the probiotic-supplemented feed by following the method described by Fajardo et al. (Citation2012). The probiotic-supplemented feed preparation contained 1 g probiotic L. salivarius CEL1 or L. reuteri CEC3 preparations per 1 kg diet (Mountzouris et al., Citation2010). These probiotic bacteria feed mixtures gave 107–109 colony-forming units (CFU). The probiotic-feed mixture was prepared daily. Up to the experimental chicks reaching 21 days old, the probiotic-feed mixture was prepared by using starter feed. From day 22–42 growers’ feed was used. The viability of the probiotic Lactobacillus cells in the feed mixture was checked twice a week by culturing a sample from the feed mixture on MRS Lactobacillus agar (Himedia, India). Age-dependent feeding of the starter and grower diet was performed according to nutrient recommendations for chickens based upon feeding a starter diet for 21 days, followed by a grower diet until 45 days of age (Saleh et al., Citation1997; Wecke et al., Citation2016). The basal feed composition is presented in .
Table 1. Composition of the basal starter and grower chicken feed.
2.3. Experimental design and chicken management
The experiment was carried out at the University of Gondar College of Veterinary Medicine Poultry Farm (Ethiopia). A total of 72-day-old RIR male chicks were obtained from Kombolcha Poultry Resource Development Enterprise. The sample size was calculated according to the method of Arifin and Zahiruddin (Citation2017). The chicks were randomly assigned into three experimental treatment groups with three replicates of eight chickens in each treatment. The treatments were the control, L. salivarius CEL1 and L. reuteri CEC3. Each replicate was housed separately in individual pens in a chicken room. The chickens were maintained in floor pens (1 m2) on clean wood shavings to simulate farm conditions. The pen was constructed from hard plastic sheets. Each pen was equipped with a feeder and a waterer. Feeders and waterers were of identical manufacture, type, size, colour, and other notable physical features. Chicks were individually identified using leg bands. The environmental temperature in the room was maintained at 33–35 °C in the first week and then gradually reduced to 23–25 °C until the end of the trial. Chicks were provided with a pre-weighed diet daily in the morning. Feed and water were provided ad libitum. The control chicks were fed the basal commercial diet alone. Whereas, the two groups were given a mixture of L. salivarius CEL1 and L. reuteri CEC3 with the basal diet. Chicks were vaccinated for Marek’s (on day 1), Newcastle (on days 5 and 30), infectious bursal diseases (on days 18 and 25), and Gumboro (on day 20). General flock conditions, temperature, lighting, water, feed, and mortality were observed twice daily.
2.4. Growth performance analysis of experimental chickens
The growth performance of chickens was determined. Data on initial and final body weight (BW), body weight gain (BWG), feed intake (FI), and feed conversion ratio (FCR) during the 42-day experimental period of experimental chickens were recorded. The body weight of each chick was recorded every week after 12 h of fasting. the body weight gain was calculated as the difference in final and initial body weight. Feed intake was calculated by the difference in food offered and refusal. The feed conversion ratio (FCR) was calculated by dividing feed intake by BWG (Radikara et al., Citation2016).
2.5. Caecal bacteria counting
Caecal bacteria counting was performed using the standard plate count method at 42 days of age, fifteen chickens from each treatment group (five from each replicate) were randomly selected and isolated into empty pens, feed was withdrawn overnight, and sacrificed by cervical dislocation. The chickens were eviscerated manually, and the GIT was collected aseptically. Then, 1 g of caecal contents was taken aseptically from the slaughtered chickens and serial dilutions were made with sterilized saline solution. Afterwards, one hundred microliters of sample were taken from selected dilutions and were plated over sterilized Plate Count agar and Eosine Methile Blue agar. The caecal samples were diluted 10-fold with sterilized saline solution and vortexed for 2 min. One hundred microliters of the supernatant were spread onto plate count agar and eosin methylene blue agar. After 48 h of incubation at 37 °C, the total aerobic and E. coli bacteria were counted. Similarly, 100 µl of supernatant was spread onto Lactobacillus MRS agar to calculate the total Lactobacillus population (Yeh et al., Citation2018). All agars were obtained from Himedia (India). The results were expressed as the number of CFU per gram (log10 cfu/g) of caecal content.
2.6. Measurement of carcass traits
The weights of the liver, heart, gizzard, abdominal fat (from the gizzard to celiac fat), breast (with bone and skin), back, drumstick, and thigh (with bone and skin) were measured (Radikara et al., Citation2016). After the end of the experiment, all carcasses were incinerated.
2.7. Data analysis
Data on feed intake, BWG, FCR, mortality rate, and number of colonies forming units, were presented in terms of means, standard deviations, and percentages. Data were analysed by one-way analysis of variance (ANOVA) using IBM SPSS Statistics (version 21, IBM, Armonk, NY, USA). Duncan’s multiple-range test was used to examine the similarities among all experimental groups. The level of significant difference was set (p < 0.05).
3. Results
3.1. Growth performance
Results of body weight, Feed intake, BWG, FCR, and mortality rate of the control, L. salivarius CEL1, and L. reuteri CEC3 dietary treatments of RIR male chickens are presented in . There was no statistically significant difference (p > 0.05) between treatments in body weight (at day 1), feed intake day 21–42, BWG (g) at day 22–42, and FCR day 1–21 and 1–42. The chick fed with the two Lactobacilli cultures had better body weight gain at day 21 and day 42 of rearing and feed intake at days 1–21 and 1–42 than those fed the control group.
Table 2. Results of body weight, feed intake, body weight gain (BWG), feed conversion ratio (FCR), and mortality rate of the control, Lactobacillus salivarius CEL1, and Lactobacillus reuteri CEC3 dietary treatments of RIR male chickens.
The mean body weights (g) of chickens under the control, L. salivarius CEL1 and L. reuteri CEC3 dietary treatment groups were 547.06, 562.63, and 588.45, respectively, at day 21 of rearing. At day 42, the mean body weights (g) of chickens in the control, L. salivarius CEL1 and L. reuteri CEC3 dietary treatment groups were 904.56, 964.90, and 997.36, respectively. The final body weight at 42 days of rearing of chickens fed with the two Lactobacillus bacteria was a distinct significant difference (p < 0.01) between the groups in the control group.
The mean feed intake (g) of chickens fed the control, L. salivarius CEL1- and L. reuteri CEC3-containing feed at day 1–21 and 22–42 was 726.16 and 1946.89, 830.58 and 2366.12, and 856.92 and 2356.10, respectively. Similarly, the body weight gain at days 1–21 and 1–42 were statistically significant differences (p < 0.01) between treatment groups. The highest was recorded on chicken feed with L. reuteri CEC3. The feed conversion ratio was not a significant difference (p > 0.05) on days 1–21 and 1–42. However, FCR chicken at days 22–42 was a significant difference (p < 0.01), the higher in L. salivarius CEL1- (2.91 and L. reuteri CEC3- (2.90). The control group had a better feed conversion ratio than the L. salivarius CEL1- and L. reuteri CEC3-treated groups. The mortality rate (%) of the control group was 4.1%, and 0% mortality was recorded in the L. salivarius CEL1 and L. reuteri CEC3 treatment groups.
3.2. Caecal bacterial count
As shown in , supplementation of the two potential Lactobacillus probiotics to the feed of chicks significantly influenced the number of E. coli in the cecum of chickens. Giving either L. salivarius CEL1 or L. reuteri CEC3 in the feed resulted in the lowest number of E. coli compared to the control group. In addition, L. salivarius CEL1 and L. reuteri CEC3 significantly (p < 0.05) inhibited the total aerobic bacteria count.
Figure 1. Effects of Lactobacillus salivarius CEL1 and Lactobacillus reuteri CEC3 dietary supplementation on populations of cecal Lactobacilli, total aerobes, and Escherichia coli of RIR male chickens at 42 days of age enumerated on spread plate agar medium and expressed as log10CFUg−1. Columns represent the means of 15 birds in each treatment group ± standard deviation. The error bars indicate significant differences between treatments (p < 0.05).
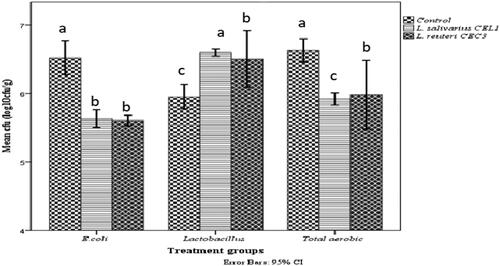
3.3. Measurement of carcass traits
The mean values of live weight, dressing weight, breast weight, back weight, thigh weight, and drumstick weight are presented in . The mean live and dressing weights (g) of the treatment groups of control (890.11, 637.98), L. salivarius CEL1 (963.76, 706.61), and L. reuteri CEC3 (1003.74, 736.3), respectively were significant differences (p < 0.05). All three groups had significant differences (p < 0.01) in abdominal fat. The L. reuteri CEC3 treatment groups had the highest (6.62%) abdominal fat content. The three treatment groups also gave different results for internal organ weights (liver, heart, and gizzard).
Table 3. Effect of Lactobacillus salivarius CEL1 and Lactobacillus reuteri CEC3 treatments on live, dressing, and organ weights of Rhode Island Red Male chickens at day 42.
4. Discussion
In this study, the addition of L. salivarius CEL1 and L. reuteri CEC3 bacteria to the feed of chickens influenced the growth performance of male RIR chickens during the starter (1–21 days) and grower phases (22–42 days). Lactobacillus salivarius CEL1 and L. reuteri CEC3 supplementation improved the body weight of chicks by increasing the number of Lactobacillus and decreasing the number of E. coli in the ceca. From the two Lactobacillus treatment groups, L. reuteri CEC3 showed a statistically significant difference (p < 0.05) in chicken body weight and body weight gain. As shown in , the influence of the two Lactobacillus treatments was achieved without a significant difference in feed intake, and there were statistically significant differences in feed conversion efficiency at day 42 of rearing. This shows using the two Lactobacillus strains as feed additives would be preferred.
The properties of Lactobacillus spp. to inhibit the growth of undesirable microorganisms in the gastrointestinal tract of chickens have been described in many previous studies. Administration of Lactobacillus plantarum K KKP 593/p and Lactobacillus rhamnosus KKP 825 via feed or drinking water reduced the number of E. coli in ROSS 308 Broiler chickens (Kuprys-Carus et al., Citation2019). Yu et al. (Citation2007) reported a significant effect of the L. reuteri Pg4 strain on increasing body weight gain and reducing harmful bacteria in chickens.
A similar study that involved the use of mixed Lactobacillus culture supplemented feed (1 g Lactobacillus culture/1 kg feed) to feed pure Hubbard and pure Shaver chicks from day 21 to 42 resulted in greater weight gain and heat stress tolerance in comparison to controls (Zulkifli et al., Citation2000). Similarly, the addition of probiotics into feed resulted in better growth and better quality meat (Bansal et al., Citation2011). Supplementing Kenyan indigenous chickens with Lactobacillus probiotics in drinking water also significantly improved their weight gain (Atela et al., Citation2015). In contrast to the present study, the findings of Wang et al. (Citation2017) showed that the use of the mixed probiotic Pediococcus pentosaceus and Bacillus subtilis, Issatchenkia orientalis and Bacillus macerans in the diet reduced the feed conversion ratio of Qingjia Ma chickens.
The synthesis of antimicrobial compounds by lactic acid bacteria species, such as Lactobacillus spp., Pediococcus acidilactici, Lactococcus lactis, and Enterococcus faecium, is one mechanism to prevent colonization by pathogens. These antimicrobial products include bacteriocins, short-chain fatty acids, hydrogen peroxide, and others. These antimicrobial compounds inhibit or kill other related or unrelated bacteria, including E. coli, Salmonella, Typhimurium, Staphylococcus aureus, Clostridium perfringens, Bacillus spp., Liseria spp., Klebsiella spp., and Proteus spp. by binding to specific receptors and causing cell damage (Cisek & Binek, Citation2014).
According to Shokryazdan et al. (Citation2017), supplementation of chicken feed with a mixture of L. salivarius strains improved the body weight, body weight gain, and FCR of Cobb500 broiler chickens. In addition, it increased populations of beneficial bacteria (Lactobacilli) and decreased harmful bacteria, such as E. coli and total aerobes. Administration of the poultry probiotic lactic acid bacteria reduces enteric pathogens through competitive exclusion in the poultry intestinal tract. This improves the intestinal health of poultry (Harimurti & Hadisaputro, Citation2015). In addition, L. salivarius and Pediococcus pentosaceus supplementation significantly increased BW and average daily gain and decreased FCR. In addition, these probiotic strains increased the number of Lactobacillus and significantly decreased the number of E. coli in ceca (Chen et al., Citation2017). Similar to the results of the present study were in line with the study of Harimurti and Hadisaputro (Citation2014) the administration of indigenous probiotic lactic acid bacteria to Lohmann strain broiler chickens improved carcass, liver, gizzard, and heart weight. The significant difference in dressing and internal organ weight between treatments may be related to the greater live weight and amount of feed consumption of those groups.
According to Estrada et al. (Citation2001), the administration of Bifidobacterium bifidum significantly increased the number of lactobacilli in the intestinal tract of broiler chickens. Kalavathy et al. (Citation2008) also found better feed conversion efficiency by giving mixed cultures of Lactobacillus to male broiler Hubbard chickens at days 28 and 35 of the experiment. The difference in the feed intake, feed conversion ratio, and body weight between the present study and others may be due to differences in the chicken breed, the basal feed composition, and the species type of additive probiotic bacteria used.
5. Conclusion
The results of the present study indicated that supplementation of chicken feed with L. salivarius CEL1 and L. reuteri CEC3 improved body weight and body weight gain. The addition of these Lactobacillus feed supplements increased populations of beneficial Lactobacillus bacteria and decreased harmful bacteria, such as E. coli and total aerobes. Further studies are recommended to evaluate the effects of these two Lactobacillus strains on egg quality, meat quality, immune status, and poultry health.
Ethical approval
Ethical approval was obtained from the Research Ethical Committee of the University of Gondar (Ref. No IOB/486/07/2019).
Consent for publication
Not applicable.
Author contributions
KTS, KJM, and NBT conceived and designed the experiments; KTS and SAM performed the experiments and carcass analysis, analyzed and interpreted the data; KTS contributed reagents, materials, analysis tools or data; KTS, KJM, NBT, and SAM wrote the paper. All authors read and approved the final manuscript.
Acknowledgements
The authors would like to give special thanks to Pan African University for the sponsorship of this research work. We are also grateful to the University of Gondar, Ethiopia, for this research laboratory work.
Disclosure statement
No potential conflict of interest was reported by the author(s).
Data availability statement
Data included in article/supplementary material/referenced in the article.
Additional information
Funding
Notes on contributors
Kibrnesh Tegenaw Tsega
Kibrnesh Tegenaw Tsega (MSc) is a lecturer and researcher at the University of Gondar. She studied Molecular Biology and Biotechnology. Currently, she works at the University of Gondar, Institute of Biotechnology, Ethiopia. She has published more than four articles in reputable journals. Her research interests include the production of food and feed supplements (either probiotics or prebiotics), microbial conversion of biomass to valuable products, genomics and proteomics, metabolic pathway analysis, immunological response mechanisms to microbes, and plant/animal interaction with microorganisms.
J. Maina Kagira
J. Maina Kagira BVM, MSc, PhD working at Jomo Kenyatta University of Agriculture and Technology (JKUAT), his research interest mainly I. Development of diagnostic tools and novel drugs for the treatment of tropical human and livestock diseases II. Development of animal models for neglected tropical diseases III. Research on epidemiology, economic importance, and control of tropical human and livestock diseases IV. Research on the ‘One Health’ approach in the management and control of diseases.
Nega Berhane Tessema
Nega Berhane Tessema BSc, MSc, PhD working at the University of Gondar and director of the Institute of Biotechnology. He is a Medical Biotechnologist.
Shewangzaw Addisu Mekuria
Shewangzaw Addisu Mekuria BSc, MSc, PhD working at the University of Gondar College of Veterinary Medicine and Animal Sciences. He is an assistant professor in Animal Nutrition and PhD in Food Science and Nutrition.
References
- Alagawany, M., Abd El-Hack, M. E., Farag, M. R., Sachan, S., Karthik, K., & Dhama, K. (2018). The use of probiotics as eco-friendly alternatives for antibiotics in poultry nutrition. Environmental Science and Pollution Research International, 25(11), 1–8. https://doi.org/10.1007/s11356-018-1687-x
- Anadón, A. (2006). WS14 The EU ban of antibiotics as feed additives (2006): Alternatives and consumer safety. Journal of Veterinary Pharmacology and Therapeutics, 29(s1), 41–44. https://doi.org/10.1111/j.1365-2885.2006.00775_2.x
- Arifin, W. N., & Zahiruddin, W. M. (2017). Sample size calculation in animal studies using resource equation approach. The Malaysian Journal of Medical Sciences, 24(5), 101.
- Atela, J., Tuitoek, J., Onjoro, P., & Kibitok, N. (2015). Effects of probiotics feeding technology on weight gain of indigenous chicken in Kenya. IOSR Journal of Agriculture and Veterinary Science, 8(11), 33–36.
- Azzaz, H. H., Morsy, T. A., & Murad, H. A. (2016). Microbial feed supplements for ruminant’s performance enhancement. Asian Journal of Agricultural Research, 10(1), 1–14. https://doi.org/10.3923/ajar.2016.1.14
- Bansal, G., Singh, V., & Sachan, N. (2011). Effect of probiotic supplementation on the performance of broilers. Asian Journal of Animal Sciences, 5(4), 277–284. https://doi.org/10.3923/ajas.2011.277.284
- Birhanu, M. Y., Osei-Amponsah, R., Yeboah Obese, F., & Dessie, T. (2023). Smallholder poultry production in the context of increasing global food prices: Roles in poverty reduction and food security. Animal Frontiers: The Review Magazine of Animal Agriculture, 13(1), 17–25. https://doi.org/10.1093/af/vfac069
- Chattopadhyay, M. K. (2014). Use of antibiotics as feed additives: A burning question (Vol. 5, p. 334). Frontiers Media SA.
- Chen, F., Zhu, L., & Qiu, H. (2017). Isolation and probiotic potential of Lactobacillus salivarius and Pediococcus pentosaceus in specific pathogen free chickens. Revista Brasileira de Ciência Avícola, 19(2), 325–332. https://doi.org/10.1590/1806-9061-2016-0413
- Cisek, A., & Binek, M. (2014). Chicken intestinal microbiota function with a special emphasis on the role of probiotic bacteria. Polish Journal of Veterinary Sciences, 17(2), 385–394. https://doi.org/10.2478/pjvs-2014-0057
- Dhama, K., Verma, V., Sawant, P., Tiwari, R., Vaid, R., & Chauhan, R. (2011). Applications of probiotics in poultry: Enhancing immunity and beneficial effects on production performances and health–A review. Journal of Immunology and Immunopathology, 13(1), 1–19.
- Directive (2010). 2010/63/EU of the European Parliament and of the Council on the protection of animals used for scientific purposes. 276 C.F.R.
- Estrada, A., Wilkie, D., & Drew, M. (2001). Administration of Bifidobacterium bifidum to chicken broilers reduces the number of carcass condemnations for cellulitis at the abattoir. Journal of Applied Poultry Research, 10(4), 329–334. https://doi.org/10.1093/japr/10.4.329
- Fajardo, P., Pastrana, L., Méndez, J., Rodríguez, I., Fuciños, C., & Guerra, N. P. (2012). Effects of feeding of two potentially probiotic preparations from lactic acid bacteria on the performance and faecal microflora of broiler chickens. The Scientific World Journal, 2012, 1–9. https://doi.org/10.1100/2012/562635
- FAO/WHO (2001). Health and nutritional properties of probiotics in food including powder milk with live lactic acid bacteria. Prevention, 5(1), 1–10.
- Fijan, S. (2014). Microorganisms with claimed probiotic properties: An overview of recent literature. International Journal of Environmental Research and Public Health, 11(5), 4745–4767. https://doi.org/10.3390/ijerph110504745
- Harimurti, S., & Hadisaputro, W. (2014). Effect of indigenous probiotics lactic acid bacteria on performance, intestinal length and weight of internal organs of broiler chicken [Paper presentation]. Paper presented at the International Conference on Beneficial Microbes, Penang, Malaysia.
- Harimurti, S., & Hadisaputro, W. (2015). Probiotics in poultry. In M.-T. Liong (Ed.), Beneficial microorganisms in agriculture, aquaculture and other areas (pp. 1–19). Springer International Publishing. https://doi.org/10.1007/978-3-319-23183-9_1
- Jha, R., Das, R., Oak, S., & Mishra, P. (2020). Probiotics (direct-fed microbials) in poultry nutrition and their effects on nutrient utilization, growth and laying performance, and gut health: A systematic review. Animals, 10(10), 1863. https://doi.org/10.3390/ani10101863
- Kalavathy, R., Abdullah, N., Jalaludin, S., Wong, C., & Ho, Y. (2008). Effect of Lactobacillus cultures and oxytetracycline on the growth performance and serum lipids of chickens. International Journal of Poultry Science, 7(4), 385–389. https://doi.org/10.3923/ijps.2008.385.389
- Krysiak, K., Konkol, D., & Korczyński, M. (2021). Overview of the use of probiotics in poultry production. Animals, 11(6), 1620. https://doi.org/10.3390/ani11061620
- Kuprys-Carus, M., Michalczuk, M., Chablowska, B., & Stefanska, I. (2019). Comparison of the effect of lactic acid bacteria added to feed or water on growth performance, health status and gut microbiota of chickens broilers. Annals of Warsaw University of Life Sciences-SGGW-Animal Science, 58(1), 55–67.
- Lutful Kabir, S. (2009). The role of probiotics in the poultry industry. International Journal of Molecular Sciences, 10(8), 3531–3546. https://doi.org/10.3390/ijms10083531
- Mehdi, Y., Létourneau-Montminy, M.-P., Gaucher, M.-L., Chorfi, Y., Suresh, G., Rouissi, T., Brar, S. K., Côté, C., Ramirez, A. A., & Godbout, S. (2018). Use of antibiotics in broiler production: Global impacts and alternatives. Animal Nutrition, 4(2), 170–178. https://doi.org/10.1016/j.aninu.2018.03.002
- Mottet, A., & Tempio, G. (2016). Global poultry production: Current state and future outlook and challenges. World’s Poultry Science Journal, 73(2), 245–256. https://doi.org/10.1017/S0043933917000071
- Mountzouris, K., Tsitrsikos, P., Palamidi, I., Arvaniti, A., Mohnl, M., Schatzmayr, G., & Fegeros, K. (2010). Effects of probiotic inclusion levels in broiler nutrition on growth performance, nutrient digestibility, plasma immunoglobulins, and cecal microflora composition. Poultry Science, 89(1), 58–67. https://doi.org/10.3382/ps.2009-00308
- National Research Council (2010). Guide for the care and use of laboratory animals. Retrieved from www.nap.edu
- Peng, Q., Zeng, X. F., Zhu, J. L., Wang, S., Liu, X. T., Hou, C. L., Thacker, P. A., & Qiao, S. Y. (2016). Effects of dietary Lactobacillus plantarum B1 on growth performance, intestinal microbiota, and short chain fatty acid profiles in broiler chickens. Poultry Science, 95(4), 893–900. https://doi.org/10.3382/ps/pev435
- Radikara, M. V., Moreki, J. C., Mareko, M. H. D., & Kgwatalala, P. M. (2016). Effect of feeding commercial broiler diets on growth performance of Tswana and Orpington chickens reared upto 18 weeks of age under intensive system.
- Saleh, E., Watkins, S., & Waldroup, P. (1997). Changing time of feeding starter, grower, and finisher diets for broilers 3. Birds grown to 3.3 kg. Journal of Applied Poultry Research, 6(3), 290–297. https://doi.org/10.1093/japr/6.3.290
- Shin, M., Han, S., Ji, A., Kim, K., & Lee, W. (2008). Isolation and characterization of bacteriocin-producing bacteria from the gastrointestinal tract of broiler chickens for probiotic use. Journal of Applied Microbiology, 105(6), 2203–2212. https://doi.org/10.1111/j.1365-2672.2008.03935.x
- Shokryazdan, P., Faseleh Jahromi, M., Liang, J. B., Ramasamy, K., Sieo, C. C., & Ho, Y. W. (2017). Effects of a Lactobacillus salivarius mixture on performance, intestinal health and serum lipids of broiler chickens. PLOS One, 12(5), e0175959. https://doi.org/10.1371/journal.pone.0175959
- Tsega, K. T., Maina, K. J., & Tesema, N. B. (2023). Characterization of potential probiotics Lactobacillus species isolated from the gastrointestinal tract of Rhode Island Red (RIR) chicken in Ethiopia. Heliyon, 9(7), e17453. https://doi.org/10.1016/j.heliyon.2023.e17453
- Wang, Y., Sun, J., Zhong, H., Li, N., Xu, H., Zhu, Q., & Liu, Y. (2017). Effect of probiotics on the meat flavour and gut microbiota of chicken. Scientific Reports, 7(1), 6400. https://doi.org/10.1038/s41598-017-06677-z
- Wecke, C., Pastor, A., & Liebert, F. (2016). Validation of the lysine requirement as reference amino acid for ideal in-feed amino acid ratios in modern fast growing meat-type chickens. Open Journal of Animal Sciences, 6(3), 185–194. https://doi.org/10.4236/ojas.2016.63024
- Yeh, R. H., Hsieh, C. W., & Chen, K. L. (2018). Screening lactic acid bacteria to manufacture two-stage fermented feed and pelleting to investigate the feeding effect on broilers. Poultry Science, 97(1), 236–246. https://doi.org/10.3382/ps/pex300
- Yu, B., Liu, J., Chiou, M., Hsu, Y., & Chiou, P. (2007). The effects of probiotic Lactobacillus reuteri Pg4 strain on intestinal characteristics and performance in broilers. Asian-Australasian Journal of Animal Sciences, 20(8), 1243–1251. https://doi.org/10.5713/ajas.2007.1243
- Zulkifli, I., Abdulllah, N., Azrin, N. M., & Ho, Y. W. (2000). Growth performance and immune response of two commercial broiler strains fed diets containing Lactobacillus cultures and oxytetracycline under heat stress conditions. British Poultry Science, 41(5), 593–597. https://doi.org/10.1080/713654979