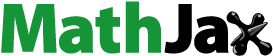
Abstract
Field performance and leaf productivity of silkworm feed plants or genotypes are essential issues to be considered at start of sericulture business. In addition, the growth, development and economic characteristics of silkworms are significantly influenced by the quality of the feed. Hence, this experiment (both in the field and laboratory) was conducted at Jimma Agricultural Research Center (Melko station) during the main cropping seasons. The study was carried out to evaluate different cassava genotypes for adaptability, leaf productivity and silk yields of Eri-silkworms (Samia cynthia ricini Boisduval) fed on different cassava genotypes. Ten cassava genotypes were arranged in a randomized complement block design (RCBD) to evaluate agronomic performance, and the feasibility of cassava genotypes as Eri-silkworm feed was also evaluated in a complete randomized design (CRD) in three replications. A significant difference (P ≤ 0.05) was recorded in the agronomic performance of cassava genotypes in the field and the rearing performance of Eri silkworms. The cassava genotypes, including Kulle (5.7 ton/ha), M94/0117 (5.0 ton/ha) and 869 (4.6 ton/ha), were found to have significantly higher fresh leaf yield compared to other genotypes. The variation in fresh leaf yield observed is due to genetic difference and leaf moisture content among cassava genotypes. In addition, the findings also show that the rearing performance of Eri-silkworm was significantly higher in terms of effective rate of rearing and approximate digestibility when fed on leaves of 869, Umbure and Kulle. The study revealed that Umbure and 869 genotypes gave the largest cocoon weights (2.5 g), shell weights (0.3 g) and shell ratios (15.8%). Higher crude protein content was also recorded from leaves of genotype Kulle, M94/0117 and 869, with mean values of 28.6%, 27.1% and 26.9%, respectively. In general, the findings demonstrated that 869, Umbure and M94/0117 were found to be better-performing genotypes in terms of agronomic performance, crude protein, moisture, dry matter and ash content of leaves, and rearing performance of Eri-silkworms when fed leaves of these genotypes.
REVIEWING EDITOR:
1. Introduction
Sericulture is an art and science of raising silkworms for silk production. It is an agricultural industry that originated in China (Dar et al., Citation2021; Sime & Siraj, Citation2020). Silkworm rearing entails a variety of activities, including soil plowing, planting of feed plant (mulberry, castor and cassava), cultivation, silk fiber processing (cocoon drying, cocoon cooking, silk fiber spinning or reeling) for manufacturing of silk thread and also the development of silk industry (Sime & Siraj, Citation2020).
Silk production has offered an economic source and employment for a number of developing countries (Kavane, Citation2014). Ethiopia is believed to offer a safe opportunity to use the technology for income generation and employment creation for people at various levels due to the country’s favorable agro-climatic conditions and the availability of the resources needed to produce silk (Sime & Siraj, Citation2020). Ethiopians are also thought to have an edge in adopting the technology because they are accustomed to spinning other materials such as cotton (Tesfa et al., Citation2014). Ethiopia produces silk by using Eri-silk worms and mulberry silkworms. Mulberry silk worms are monophagous in nature and only consume mulberry leaves, but Eri-silk worms are polyphagous in nature and consume leaves of different plants including castor and cassava (Hailu, Citation2016).
The Eri-silkworm (Samia cynthia ricini) is a multivoltine serigeneous insect that is the most exploited, domesticated, and commercialized non-mulberry feeder silkworm. It has many generations per year and feeds on several host plant species (Naik & Murthy, Citation2013). It is a domesticated silkworm and can be raised indoors. The main feed plants for Eri-silkworm are Castor (Ricinus communis L.). Castor plants lose their leaves in dry periods and so, presence of optional feed plants will be very essential. Therefore, cassava will serve as an alternative solution to alleviate this problem. Cassava (Manihot spp.) is second choice feed plant for Eri-silkworms for commercial silk worm production (Sakthivel, Citation2016).
Cassava belongs to the family Euphorbiaceae and the genus Manihot. The genus Manihot is a large family of flowering plants with about 8000 species (Jennings & Iglesias, Citation2000). Among these species that belong to Manihot, Manihot esculenta Crantz is the most economically important species and is widely cultivated for food and industrial applications. There is different cassava varieties cultivated depending on appropriate ecologies. Cassava are well adapted and grown in different parts of the country (Mulualem et al., Citation2020), which can be integrated with eri-silk worm production because it has extra foliage in nature (25–40%) to be used by Eri-worms without loss in tuber yield (Narzary & Saikia, Citation2022). Therefore, there is a great need to evaluate cassava genotypes for adaptability, leaf productivity, growth, and silk yields of Eri silkworms fed on different cassava genotypes.
2. Materials and methods
2.1. Study area description
The experiment was conducted under field conditions in Jimma Agricultural Research Center (Melko station) during the main cropping seasons of 2020–2022 under rain fed conditions. The soil type of the Center is Nitosol with a pH of around 5.1. The average annual rainfall is 1216.7 mm, with the main wet season from June to September. The maximum and minimum temperatures of Jimma were 27 °C and 10 °C, respectively (JARCM, Citation2022). The longitude and latitude of Jimma are 7°36’N and 36° 50′E, respectively, at an altitude of 1753 m above sea level. The study areas practice mixed farming systems of crop-livestock.
2.2. Experimental design and planting materials
The tested materials were ten cassava genotypes (). Stems with uniform thickness of 14 months old, pest and disease-free cassava genotypes were obtained from horticulture case team working at Jimma Agriculture Research Center (JARC). The treatments were arranged in a randomized complete block design (RCBD) in three replications to evaluate the agronomic performance of cassava genotypes. RCBD is used in experiments to limit variation by accounting for particular effects in the field. The cassava cuttings, 25–30 cm long (consisting of 5–6 buds) and 14-month-old cuttings were planted at a spacing of 1 m between rows and plants. The same age and size of cassava cuttings are important to generate uniform data during the experiment. If we use different-age cassava cuttings, older ones germinate slowly while younger ones germinate quickly. And when using different sizes of cassava cuttings, the number of buds per cutting is different. These result in data variations during the experimental period. The experimental plot size was 12 m2 (3 m × 4 m) for each treatment, leaving a free space of 1.5 m between plots. Each plot has four rows with 20 plants per plot. Blocks spaced at 2 m among each other. After planting cassava accessions, all field management activities (weeding, inter-cultivation, harvesting, etc.) were performed similarly in all plots during the experimental period.
Table 1. Cassava accessions used as treatment for experiment.
2.3. Experimental layout and silkworm rearing in the laboratory
The silkworm rearing room and equipment were cleaned, washed and disinfected with 2% formalin solution. The eggs of Eri-silkworm race were used to carry out the experiment as per recommendations by Dayashankar (Citation1982). A feeding experiment was carried out in the laboratory to select the best cassava genotypes as feed for Eri silkworms. Treatments were arranged in a complete randomized design (CRD) with three replications for each treatment. Leaves from each cassava genotypes were harvested separately from a field experiment and feed to silk worms in three replications. Then the effect of cassava leaves on rearing performance of Eri-silk worms was observed. Eri-silkworm eggs (200) were brushed into each replication tray and allowed to complete their life cycle. Silkworms at the larval stage were fed four times a day with tender leaves until the 3rd instar and mature leaves until the fifth instar. Rearing beds were cleaned every day before the first feeding. The room temperature and relative humidity were maintained based on recommendations (Rahmathulla, Citation2012). The grown-up worms were picked and left on the mountages for spinning. On the sixth to eighth day of spinning, the cocoons were harvested, counted, and weighed.
2.4. Nutritional value
Nutritional compositions were determined at Animal Nutrition Laboratory of Holeta Agricultural Research Center (HARC). To determine the chemical constituents, 500 g of fresh leaf samples were taken 14 old months after planting, stored in an airtight bag, and subjected to a forced air draft oven at 65 °C for 72 hours. The dried samples were then ground and passed through a 1 mm-wide sieve. All samples were subjected to chemical analysis for the determination of organic matter following the methods of AOAC. (Citation2000). Crude protein (CP) (Kjeldhal method), acid detergent fiber (ADF), neutral detergent fiber (NDF), and acid detergent lignin (ADL) were analyzed using the procedure of the Van Soest method (Van Soest et al., Citation1991). Ash was determined by igniting at 550 °C overnight (AOAC, Citation2000). Tilley and Terry (Citation1963) procedure was used to determine in vitro dry matter digestibility.
2.5. Data collection
2.5.1. Agronomic parameters
Agronomic data on cassava accessions, such as number of leaves per plant, number of primary and secondary branches per plant, plant height, stand count, 50% emergency date, leaf moisture content and fresh and dry weight of leaf yield, were collected during the harvesting stage. The plant height was measured from five randomly selected plants from each plot using a steel tape from the ground level to the highest leaf. Fresh leaf yield was determined by counting the number of leaves from three sample plants and multiplying by the number of plants per plot. Then, the weight of leaves obtained from three sample plants was converted to plant population per hectare. Leaf moisture content was measured by taking fresh and dry weight of the leaf (Zhu et al., Citation2020).
Where MC: moisture content, FW: fresh weight, DW: dry weight
2.5.2. Larval parameters
All necessary data on the rearing performance of Eri-silkworm, including the number of eggs hatched, larval weight, cocoon weight, shell weight, number of cocoon harvested, survival rate and hatching percentage, were collected during the experimental period. Besides, amount of feed ingested, digested, approximated digestibility in percent, mean larval weight, efficient conversion of ingesta to larva and efficient conversion of digesta to larva traits were also recorded. The following formulae adopted by Singh and Benchamin (Citation2002) were used to evaluate rearing performance of silk worms.
Hatchability (%):- Hatchability was calculated by deducting the number of non-hatched eggs from the total number of eggs laid and dividing by the number of normal eggs (Singh & Benchamin, Citation2002).
Silk ratio: The silk ratio is the amount of silk present in a cocoon shell expressed as a percentage, as shown below.
Effective rate of rearing (ERR) (%):-The effective rate of rearing was measured by dividing the number of larvae spinning cocoons by the number of larvae brushed and expressing it in percentage (Singh & Benchamin, Citation2002).
Mean larval weight= (Final weight – Initial weight)/2 + Initial weight
Different nutritional parameters were calculated using the following formula (Rahmathulla & Suresh, Citation2012).
Ingesta = dry weight of leaf fed – dry weight of leftover leaf
Digesta = dry weight of leaf ingested – dry weight of feces
Approximate digestibility percentage (AD %) = (dry weight of food ingested– dry weight of feces)/dry weight of food ingested) × 100.
Efficient conversion of ingesta or digesta (ECI/ECD) to larva (%) = (dry weight gained by larvae during feeding period/dry weight of ingesta or digesta) × 100
2.6. Statistical analysis
The data were examined for homogeneity of variance and normality. Data that have normal distributions were subjected to the analysis of variance (ANOVA) appropriate to RCBD and CRD using SAS general linear model software. Mean fresh biomass yield, dry matter yield and agronomic parameters data were determined to compare treatment means. Least significant difference at 5% level of probability was used for comparison of means among treatments.
3. Results and discussion
3.1. Field performance of different cassava accessions
The agronomic performance of 10 cassava genotypes was evaluated at Jimma Agriculture Research Centers. The mean values of cassava genotypes for different agronomic traits such as 50% germination date, stand count, plant height, primary branches and leaf biomass yield were present in . The result indicated that 50% germination date, plant height, leaf fresh biomass and dry matter yield (t/ha) traits were significantly (P ≤ 0.05) affected by genotypes but number of stand count and number of branches per plant did not showed significant differences across genotypes. Analysis of variance showed that days to 50% germination from cuttings were significantly affected by different cassava genotypes (). The early germinating genotypes were Umbure, Kello, and 869, which took 153 days and 159 days to germinate after planting, respectively. Whereas 1554, high HCN, and 196/624 were late-germinating genotypes that germinated 185, 180 and 165 days after planting, respectively. The variation in germination found is attributed to differences in cassava genotypes used in the experiment. Significant variations were observed in plant height. The mean plant height for tested cassava genotypes ranged from 114 cm (High HCN) to 163 cm (Umbure) with a mean of 130.0 cm. Umbure genotype had the highest plant height. The variation in plant height obtained in the current findings indicates the presence of heterogeneity among cassava genotypes for this trait.
Table 2. Agronomic performance of cassava accessions at JARC.
The results showed that highly significant (p < 0.001) differences in fresh and dry matter leaf yield among cassava genotypes or treatments. Significantly higher fresh leaf yield was obtained from Kulle, M94/0117, 869 and Umbure genotypes, with a rate of 5.7, 5.0, 4.6 and 3.4 tons/hectare, respectively. Whereas genotype high HCN gave lowest fresh leaf yield compared to other genotypes. The genotypes M94/0117, Kulle and 869 produced significantly higher leaf dry matter yield in comparisons to other genotypes. The variation in fresh and dry matter leaf yield observed is due to genetic variation among cassava genotypes. The current findings’ cassava leaf yield was slightly lower than that reported by Sakthivel and Qadri (Citation2017). On the other hand, the current findings of fresh leaf yield were significantly higher than those reported by Buamuangphia et al. (Citation2017). This variation is caused by cultivar differences, plant density, soil fertility, harvesting stage and the environment of the study location (Sakthivel, Citation2018). The branching nature of genotypes, shoot length, leaf area, and leaf moisture content all influence leaf yield (Adetoro et al., Citation2021).
3.2. The effect of different cassava genotypes on Eri silkworm rearing performance
The rearing performance of Eri-silkworm was evaluated by feeding different cassava genotypes. Hatchability of Eri-silkworm eggs ranges from 95.15 to 98.5% with a mean of 96.8 feeding on different cassava genotypes. These findings demonstrated that Eri-silkworm eggs had a high hatching percentage in all cassava genotypes; however, there was no significant (P ≥ 0.05) difference observed among all genotypes ().
Table 3. Cassava genotypes’ effects on Eri-silkworm rearing performance.
Silkworm fed on 10 different cassava genotypes showed significant differences in the effective rate of rearing. The highest ERR was recorded in Eri-silkworm fed on genotype 869, Umbure, and Kulle, with a rate of 91.7%, 88.8% and 85.2%, respectively. The variance in ERR of silkworms fed on different cassava genotypes is attributable to differences in leaf composition and nutritional availability, both of which contribute to silkworm growth and development. The variation observed due to difference of genetic makeup of the cassava accessions. The average ERR reported by Birari et al. (Citation2019) and Kumar and Elangovan (Citation2010) using cassava leaves was 87.85% and 88.0%, respectively. These findings were relatively higher than the current experimental results. This difference may be due to environmental conditions that affect larval survival rates.
3.3. Nutritional efficiency parameters
The quality and quantity of leaves given to silkworms influence their productivity (Putra et al., Citation2023). The current results showed that ingesta and digesta parameters have a significant difference (P ≤ 0.05) among cassava genotypes (). Significantly higher ingesta and digesta mean values were recorded by Eri-silkworms fed Umbure, 869 and Kulle genotypes of cassava. The amount of leaf ingested by Eri-silkworm ranged from 10.8 g (M94/0125) to 15.4 g (Umbure) with a mean value of 12.6 g. The amount of leaf digested by Eri-silkworm ranged from 3.4 g (M94/0125) to 7.4 g (869) with a mean value of 4.9 g. Silkworm ingested significantly more leaf when reared on genotypes Umbure (15.4 g/larva) and 869 (14.4 g/larva). Nutritional parameters have a strong influence on feed intake and silk output. The nutritional parameters such as ingesta, digesta, and approximate digestibility were significantly higher in silkworms that were fed with leaves of 869 and Umbure cassava genotypes (). This is due to the higher moisture, crude protein, ash, and dry matter content of the genotypes (). Feed consumption efficiency has a direct impact on the weight of larvae, cocoons, pupae, and cocoon shells. The high feed consumption efficiency of cassava leaves raises the weight of the final instar larvae, which influences the rise in single cocoon, pupal, and cocoon shell weight. The leaves of cassava genotypes, including Umbure, 869, and Kulle, were efficiently ingested and digested by Eri-silk worms, resulting in a higher cocoon weight, shell weight, and silk ration percentage (). Lower ingesta and digesta mean values, on the other hand, were observed for M94/0125 and 1630 (1) cassava genotypes during the experimental period. This results in lower silkworm performance and economic traits such as larval weight, silk ratio percentage, cocoon and shell weight, and/or the rearing performance of silk worms ().
Table 4. Single sample weight of economically important traits fed on cassava genotypes.
Table 5. Nutritional content of cassava genotypes.
Approximated digestibility percentage (AD %) also showed that significant difference (P ≤ 0.05) when leaves of different cassava genotypes were fed to Er-silkworm. The approximate digestibility of different genotypes of cassava fed by Eri-silkworm ranged from 55.6% (Acc-869) to 31.4% (Acc-M94/0125), with a mean value of 40.5%. Higher AD% was found in genotypes 869 (55.6%), Umbure (42.1%), and Kulle (41.1%), resulting in high silkworm rearing performance in terms of growth development and silk production. Whereas silkworms fed on genotypes M94/0125 showed lower AD (31.4%). Nutritional deficits, high fiber content, or a lack of water all have an impact on digestibility. Higher absorption efficiency or approximate digestibility is undoubtedly a racial trait, as increased feed intake does not always result in increased digestibility (Rahmathulla et al., Citation2004).
Different nutritional efficiency parameters were evaluated, including efficient conversion of ingesta to larva, efficient conversion of digesta to larva, and efficient conversion of ingesta and digesta to cocoon. Significantly higher efficient conversion of ingesta to larva was recorded from genotype Umbure, followed by genotype 869 and Kulle, compared to other genotypes. The highest and lowest efficient conversion of ingesta to larva were observed from genotypes Umbure (25.3%) and 196/624 (15.5%) when fed by Eri-silkworm, respectively. This variation in ECI to larva linked to variation in cassava genotypes. Even though there were no significant differences (P ≥ 0.05) in ECD to larva among cassava genotypes, 869 and M94/0117 had a relatively better efficiency conversion of digesta to larva. Significant variation was not observed in mature mean larva weight when Eri-silkworms were fed with ten different cassava genotypes. The feed conversion efficiency of Eri-silkworms in the current study () was higher than Putra et al. (Citation2023) findings, in which the average efficient conversion of ingesta and digesta into larvae was 22.01 and 17.7%, respectively. The more efficient conversion of ingesta and digesta to larvae was recorded from genotypes 869 and Umbure, which in turn resulted in increased larval, cocoon, and shell weight ( and ). Silkworm feed efficiency is important in converting consumed cassava leaves to silk production. A better economic metric in cocoon production is the efficiency of conversion of ingested and digested (ECI, ECD) cassava leaves into silk (Putra et al., Citation2023). Digestibility, the ratio of digested feed to coon, and the shell ratio are three important factors that determine the rate of leaf-silk conversion to shell (Putra et al., Citation2023).
Silkworm fed on ten different cassava genotypes showed significant differences in dry shell weight, silk ratio and the number of larva reaching full maturity (). A similar finding was reported by Sakthivel and Qadri (Citation2017), who observed variations in silk ratio and dry shell weight because of variations in cassava genotypes. The higher silk ratio weight was recorded in Eri-silkworm fed with genotype 869 (15.8%) and Umbure (13.3%). The current findings of silk ratio percentage are also in line with the findings of Umer et al. (Citation2016) and Buamuangphia et al. (Citation2017), when different cassava genotypes were fed as feed to Eri-silkworms. This is indicated as cassava genotypes can be an alternative feed for Eri silkworms. The higher shell weight (0.2 g) was recorded in Eri-silkworm fed with genotypes Umbure and Kulle. The remaining genotypes gave relatively lower dry shell weights when fed by Eri silkworms. A similar finding was reported by Buamuangphia et al. (Citation2017) in shell weight when fed different cassava genotypes. The variation in shell weight and silk ratio was due to variations in crude protein and moisture content cassava leaves of different genotypes. Economic traits such as cocoon weight, pupal weight did not show significant differences among cassava genotypes. However, relatively higher cocoon weight (2.4 g) was observed in 869 and Umbure genotypes (). The nutritional value of the feed plays a major role in larval and cocoon parameters. According to current findings, genotypes Umbure and 869 gave the largest cocoon weights (2.5 g), shell weights (0.3 g) and shell ratios (15.8%).
3.4. Nutritional composition of cassava genotypes
The leaf composition of cassava genotypes has shown significant variations among genotypes. The mean values of leaf compositions of cassava genotypes are presented in . The result revealed that moisture content percentage (MC%), absolute dry matter (DM), crude protein (CP), ash content, invitro dry matter digestibility (IVDMD) and dry organic matter digestibility (DOMD) traits have significant (P ≤ 0.05) differences among cassava genotypes (). However, neutral detergent fiber (ADF) and acid detergent parameters showed non-significant (P ≥ 0.05) differences. The variation in nutritional composition of cassava leaves is similar to the findings of Sakthivel (Citation2016). The nutrient content of leaves is influenced by genetic, physiological, edaphic and climatic differences with the stage of maturity. The stage of maturity is the major source of variation in nutrient content of leaves (Ravindran, Citation1995).
Moisture content percentage: The findings revealed that there was significant (P ≤ 0.01) variation in leaf moisture content among cassava accessions (). The higher moisture content was recorded in the leaves of accessions M94/0117 (76.5%), Kulle (75.4%) and 1554 (76.3%), with a mean of 73.2%. On the other hand, the lower moisture content was recorded from accessions high HCN (68.5%), M94/0125 (71.4%), and Kello (70.2%). Less evapotranspiration loss from the leaves and an increase in hydrogen ion concentration in plant sap are contributing factors to the rise in leaf moisture content in fewer genotypes (Eaton, Citation1942).
Ash content: The ash content of cassava leaves varied significantly among accessions (). The leaves of accession 869 (11.5%), Kulle (10.9%), and Umbure (9.9%) had the highest ash content, whereas accession 1554 and M94/0125 had the lowest (15.385%) ash content. Derara et al. (Citation2004) observed significant differences in ash content between different cassava genotypes, respectively. The variance in ash concentration is related to genetic differences between cassava accessions and differences in metal composition.
Crude protein content (CP): A significant difference (P ≤ 0.05) in crude protein content was recorded in the leaf among cassava genotypes (). Significantly higher crude protein content was recorded from leaves of accession Kulle, M94/0117, and 869, with a mean value of 28.6%, 27.1%, and 26.9%, respectively. The result indicates that these accessions are suitable for Eri silkworm feeding. The current findings of crude protein content in leaves are slightly higher than the findings reported by Sakthivel and Qadri (Citation2017) and Sakthivel (Citation2016). This variation in crude protein content is attributed to genetic differences among cassava accessions used for the experiment, the maturity stage of the leaves, and environmental factors. The protein content is higher at the early stage of cassava leaves, rendering them more suitable for feeding Eri-silkworm. The nutritional quality of leaf feed plants determines the economic characteristics of silkworm yield based on moisture, crude protein content, and other minerals (Bongale & Chaluvachari, Citation1993). Leaf quality is the major factor that influences the growth and development of silkworms and the production quality of cocoons. In terms of silkworm nutrition, Eri-silkworm fed with more moisture content, protein, sugar and carbohydrate and fewer minerals and crude fiber is the best (Krishnaswami, Citation1978). The highest absolute dry matter percentage was recorded from genotypes 869 (94.2%), M94/0117 (92.3%) and Umbure (91.2%) cassava genotypes, with a mean of 84.3%.
Invitro dry matter and organic matter digestibility: In vitro dry matter digestibility (IVDMD) and organic digestibility (OMD) showed significant differences (P ≤ 0.05) among cassava genotypes. Significantly higher IVDMD was recorded from accession 869 (55.0%), followed by M94/0117 (53.3%) and Umbure (51.2%). These accessions also had significantly higher OMD compared to other accessions. Nutritional content and digestibility of cassava genotypes are significantly affected by environment and harvesting stage (Rahmathulla, Citation2012; Ravindran & Ravindran, Citation1988). The higher nutritional values and digestibility of the 869, Umbure and M94/0117 genotypes attributed the superior economic traits, including cocoon yield, silk ratio, and shell weight, and were found to be most suitable for Eri-silk worms compared to other cassava genotypes, which have lower nutrient contents in leaves ( and ).
4. Conclusion and recommendation
Ten different cassava genotypes were evaluated to examine agronomic performance, nutritional content and rearing performances of Eri silkworms fed on cassava leaves. The study was focused on the selection of cassava genotypes that have higher leaf biomass yields from field evaluation and cocoon traits, leaf quality and the leaf conversion efficiency of Eri silkworms from laboratory studies. The findings showed wide variation in the quantitative and qualitative traits of cassava genotypes. These differences resulted in significant variation in rearing performance and feeding efficiency of Eri silkworms when fed leaves of these genotypes. In conclusion, the current findings demonstrated that 869, Umbure and M94/0117 were found to be better performing genotypes in terms of fresh and dry matter leaf yield, crude protein, moisture, dry matter, ash content of leaves and rearing performance of Eri-silkworms when fed leaves of these genotypes. As a result, these genotypes are preferable for use as feed in Eri-silkworm rearing and sericulture development for commercial production.
Contribution of the author
Tesfa Mossie: draft manuscript preparation, writing the whole manuscript, analysis and interpretation of data,
Hayat Worku: study conception and design, data collection and data curation
Kasa Biratu: Revising and validating the manuscript
All authors reviewed the results and approved the final version of the manuscript to be published.
Acknowledgments
We are grateful to the Ethiopian Institute of Agriculture Research for the financial support of the study.
Disclosure statement
No potential conflict of interest was reported by the author(s).
Declaration of interest’s statement
The authors declare no competing interests.
Data availability statement
Data will be made available on request.
Additional information
Funding
References
- Adetoro, N. A., Oworu, O. O., Nassir, A. L., Bello, A., Parkes, E., Ogunbayo, S. A., Akinwale, M. G., Aina, O. O., Afolabi, A., Iluebbey, P., Sanni, L. O., Maziya-Dixon, B., Dixon, A., & Kulakow, P. (2021). Evaluation of improved cassava genotypes for yield and related traits for a better breeding strategy under different agroecologies in Nigeria. Euphytica, 217(73), 1–19. https://doi.org/10.1007/s10681-021-02855-3
- AOAC. (2000). Methods of analysis. Association of Official Agricultural Chemists. (7th ed). AOAC International.
- Birari, V. V., Siddhapara, M. R., & Patel, D. H. (2019). Biology of eri silkworm, Samia ricini (Donovan) on castor, Ricinus communis L. ENTOMON, 44(3), 1–9. https://doi.org/10.33307/entomon.v44i3.469
- Bongale, U. D., & Chaluvachari, G. (1993). Evaluation of four mulberry varieties by leaf biochemical analysis and bioassay with Bombyx mori L. Journal of Indian Botanical Society, 72, 59–62.
- Buamuangphia, C., Sirimungkararat, S., Saksirirat, W., & Srijankam, K. (2017). Cassava leaf harvesting management and positive impact on growth and yields of eri silkworm. Asia-Pacific Journal of Science and Technology, 22(4), 1–10.
- Dar, K. A., Auqib, H. G., & Ganie, N. A. (2021). Jammu and Kashmir silk industry: Problems and prospects. The Pharmacology, 10(6), 369–371.
- Dayashankar, K. N. (1982). Performance of eri silkworm, Samia cynthia ricini Boisduval on different host plants and economics of rearing on castor under Dharwad conditions . M. Sc. Thesis, University of Agricultural Sciences, Bangalore, 60–86.
- Derara, Y., Waktole, S., Amsalu, N., & Felka, M. (2004). Effect of nitrogen rates on leaf quality of two Cassava (Manihot Esculenta Crantz) varieties as a feed for Eri-Silkworm (Samia Cynthia Ricini Boisduval) cocoon production at Jimma, Southwest Ethiopia. International Journal of Current Research and Acadamic Review, 7(9), 23–32.
- Eaton, F. M. (1942). Toxicity and accumulation of chloride and sulfate salts in plants. Journal of Agricultural Research, 64(7), 357–399.
- Hailu, A. (2016). Assessment of growth and performance of silk worms (Bombyx mori L.) on mulberry leaves at Jimma, South West Ethiopia. Journal of Biology, Agriculture and Healthcare, 6(9), 10–16.
- JARCM. (2022). Jimma Agriculture Research Center Metrology data source.
- Jennings, D. L., & Iglesias, C. (2000). Breeding for crop improvement. In Cassava: Biology, Production and Utilization. ; Hillocks, R.J., Thresh, A.M., Bellotti, A.C., Eds.; CABI. 2000; pp. 149–166.
- Kavane, R. P. (2014). Terminalia Arjuna-A new host of Philosamia ricini eri silkworm under Western Maharashtra condition. International Journal of Pharmacology and Biological Sciences, 6(1), 787–792.
- Krishnaswami, S. (1978). Mulberry cultivation in South India. Bulletin CSRI Myore, 1, 1–19.
- Kumar, R., & Elangovan, V. (2010). Assessment of the volumetric attributes of eri silkworm (Philosamia ricini) reared on different host plants. International Journal of Natural Sceince, 1(2), 156–160.
- Mulualem, T., Semman, N., Etana, G., & Alo, S. (2020). Evaluation of Cassava Manihot esculenta genotypes for total cyanide content, storage tuber and starch yield in South Western Ethiopia. International Journal of Biomedical Materials Research, 8(2), 14. https://doi.org/10.11648/j.ijbmr.20200802.11
- Naik, M., & Murthy, C. (2013). Evaluation of new host plant species for ericulture. International Journal of Plant Protection, 6(2), 444–448.
- Narzary, P.R., & Saikia. M. (2022). By-product utilization of Eri silkworm host plantTapioca (Manihot esculenta Crantz.): A review.Ecology, Environment and Conservation, 28(04), 1993–1996. https://doi.org/10.53550/EEC.2022.v28i04.050
- Putra, S., Fuah, A. M., & Endrawati, Y. C. (2023). Production performance of Eri Silkworm (Samia cynthia ricini) with cassava leaves feed (Manihot utilissima) on different mountages media. Jurnal Ilmu Produksi Dan Teknologi Hasil Peternakan, 11(3), 170–175.
- Rahmathulla, V., Mathur, V., & Geetha Devi, R. (2004). Growth and Dietary Efficiency of Mulberry Silkworm (Bombyx mori L.) Under Various Nutritional and Environmental Stress Conditions. Philippine Journal of Science, 133(1), 39–43.
- Rahmathulla, V. K. (2012). Management of climatic factors for successful silkworm (Bombyx mori L.) crop and higher silk production: A review. Psyche (London), 2012, 1–12. https://doi.org/10.1155/2012/121234
- Rahmathulla, V. K., & Suresh, H. M. (2012). Seasonal variation in food consumption, assimilation, and conversion efficiency of Indian bivoltine hybrid silkworm, Bombyx mori. Journal of Insect Science (Online), 12(82), 82–14. https://doi.org/10.1673/031.012.8201
- Ravindran, V. (1995). Preparation of cassava leaf products and their use in animal feed. In: Roots, tubers, plantains and bananas in animal feeding, FAO Animal Production and Health Paper. 11–116.
- Ravindran, G., & Ravindran, V. (1988). Changes in the nutritional composition of cassava (Manihot esculenta Crantz) leaves during maturity. Food Chemistry, 27(4), 299–309. https://doi.org/10.1016/0308-8146(88)90014-3
- Sakthivel, N. (2016). Evaluation of cassava varieties for eri silkworm, Samia Cynthia Ricini. Mun. Ent. Zool, 11(March), 165–168.
- Sakthivel, N. (2018). Standardization of Cassava leaf harvest in relation to ericulture cum tuber Production. International Journal of Agriculture Innovations and Research, 6(5), 2319–1473.
- Sakthivel, N., & Qadri, S. M. H. (2017). Utilization of Cassava foliage for large scale production of eri silk. International Journal of Sceince, Environment and Technology, 6(4), 2521–2534.
- Sime, D., & Siraj, Z. (2020). Sericulture in Ethiopia : Production status, opportunities, challenges and potential areas. Journal of Entomology and Zoology Studies, 8(11), 01–10.
- Singh, K. C., & Benchamin, K. V. (2002). Biology and ecology of the eri–silk moth, Samia ricini Donovan (Saturniidae). Bulletin of Indian Academy of Sericulture, 6(1), 20–33.
- Tesfa, A., Kerealem, E., Aseresu, Y., & Habtemariam, A. (2014). Assessment of value chain of sericulture products in Amhara region, Ethiopia. International Journal of Environmental Engineering and Natural Resources, 1(2), 61–69.
- Tilley, J. M. A., & Terry, R. A. (1963). A two‐stage technique for the in vitro digestion of forage crops. Grass and Forage Science, 18(2), 104–111. https://doi.org/10.1111/j.1365-2494.1963.tb00335.x
- Umer, B., Sori, W., & Getachew, M. (2016). Evaluation of castor (Ricinus communis L.) accession as feed for eri-silkworm (Samia cynthia RICINI Boisduval) at Jimma, South West Ethiopia. Sericologia, 56(4), 219–228.
- Van Soest, P. J., Robertson, J. B., & Lewis, B. A. (1991). Methods for dietary fiber, neutral detergent fiber, and nonstarch polysaccharides in relation to animal nutrition. Journal of Dairy Science, 74(10), 3583–3597. https://doi.org/10.3168/jds.S0022-0302(91)78551-2
- Zhu, Z., Li, T., Cui, J., Shi, X., Chen, J., & Wang, H. (2020). Non-destructive estimation of winter wheat leaf moisture content using near-ground hyperspectral imaging technology. Acta Agriculturae Scandinavica, Section B—Soil & Plant Science, 70(4), 294–306. https://doi.org/10.1080/09064710.2020.1726999