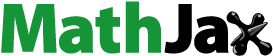
Abstract
Anthropogenic infrastructure in rivers (e.g. culverts, dams and weirs) can block the movements of fish and negatively impact their communities. Recent research has shown that fish passage at such barriers can be improved through the use of cylindrical bristle cluster (CBC) arrays. The relationship between the hydrodynamic environment created by different CBC array geometries and passage efficiency, the number of fish that passed as a percentage of those that attempted to do so, and swimming behaviour of a native wide spread European cyprinid species, the roach (Rutilus rutilus), was investigated. Passage efficiency was a function of cluster diameter and spacing; efficiency was highest (>80%) when the ratio of lateral cluster spacing (centre to centre) (Sc) to diameter was less than 5. Fish exhibited a range of swimming behaviours while manoeuvring through the CBC array to ascend the weir, the most common of which was zigzagging between two lines of clusters. Additionally, fish utilised lower velocity areas when swimming through a CBC array, often combining different swimming behaviours to successfully navigate at burst speeds. Fish passage efficiency can be improved by increasing the size of the wake behind clusters and the overall hydraulic resistance created within the array while ensuring sufficient space is available for fish to manoeuvre.
Introduction
Improving fluvial connectivity is a central challenge in the field of river restoration. A high density of barriers, such as low-head weirs, that impede the movement of fish has caused considerable ecological degradation of rivers in many countries by fragmenting critical habitat (Jones et al. Citation2019). For example, in Great Britain there is at least one artificial barrier for every 1.5 km of stream with only 3.3% of the river network fully connected (Jones et al. Citation2019). When considering Europe, fragmentation is even higher, with on average one artificial barrier for every 0.74 km of stream (Belletti et al., Citation2020). The impact these barriers create with respect to habitat fragmentation can be severe, impacting fish population numbers and diversity (Kemp Citation2015; Deinet et al. Citation2019). The hydraulic conditions at barriers can often render a structure impassable as water velocities are greater than the swim speeds of fish attempting to pass over them in an upstream direction (Kemp et al. Citation2008). Fish passes, therefore, play a crucial role in mitigating for the effects of habitat fragmentation as a result of artificial barriers. However, despite the existence of many fish pass designs, their efficacy is highly variable and often lower than expected, particularly for non-salmonids species (Bunt et al. Citation2012; Noonan et al. Citation2012).
Mitigating the impacts of low-head barriers is of particular interest as they are far more numerous than larger dams, yet can be a major obstruction to fish movement (Lucas et al. Citation2009). Low-head barriers encompass a large variety of man-made in channel structures and include watermills, fords, sluices and weirs. Fish passage solutions are being retrofitted to mitigate for the influence of these barriers on our waterways (Tummers et al. Citation2018; Lothian et al. Citation2019), with success dependent on a range of factors, and velocity reduction of key importance (Amaral et al. Citation2019). Low-head gauging weirs, such as Crump and Flat-V designs, create a particular challenge for fish passage retrofit as there is a need to maintain the gauging capabilities of the structure and as such any retrofit solution must not affect the measurement capability.
Recently, a staggered array of cylindrical bristle clusters (CBCs) was developed as a novel low-cost fish passage solution for low-head gauging weirs (Montali-Ashworth et al. Citation2020). The CBC array increases water depth and creates lower velocity areas in the wake of individual clusters that vary in width and length depending on cluster diameter (D) and spacing (Sc) (Zong and Nepf Citation2012). Consequently, the hydrodynamic environment within the CBC array is linked to the dimensionless parameter Sc/D (Nicolle Citation2009; ).
Figure 1. Different wake overlap states, from full overlap to no overlap, for spacing (Sc) to diameter (D) ratios ranging from less than 1.2 to greater than 4 (Nicolle Citation2009).

For solid cylinders, when Sc/D > 4, the wakes produced do not interact. When 3 < Sc/D < 4, the shear layer that forms due to flow separation around the upstream cylinder attaches to the boundary layer around the downstream cylinder. When Sc/D < 3, the wake created by the upstream cylinder overlaps with that produced by the one downstream. When cylinders are parallel and Sc/D < 1.2, they form a single bluff body (Nicolle Citation2009; ). For patches of vegetation or porous clusters, the ratio of Sc/D that produces wake overlap differs to that of solid cylinders because they generate longer wakes for the same diameter (Nepf Citation1999; Taddei et al. Citation2016). Taddei et al. (Citation2016) found that wake length varied depending on porosity, with near wake velocity dissipation occurring when Sc/D was greater than 5, suggesting that wake overlap could occur for ratios smaller than this if two cylinders were studied in tandem. While knowledge of the hydrodynamics around porous cylinder clusters has advanced in recent years, studies on arrays of these clusters and our understanding of how fish interact with them remains limited. Specifically, there is no information on how the ratio of Sc/D influences the swimming behaviour of fish or their passage over sloped weirs retrofitted with CBC arrays.
The interaction of fish with a complex hydrodynamic environment is a topic that has generated interest in recent years (e.g. Montgomery et al. Citation2003; Liao Citation2007; Cote and Webb 2015; Kerr et al. Citation2016). Under certain conditions, trout can utilise the hydrodynamics created by solid cylinders to reduce energetic costs (e.g. Kerr et al. Citation2016). Swimming behaviours that facilitate this include entrainment, where fish hold position behind a cylinder and orient their body based on spatial flow patterns so that the effect of hydrodynamic forces are reduced (Przybilla et al. Citation2010). Another behaviour, termed the Kármán gait, is characterised by large lateral body oscillations and a tail beat frequency that matches cylinder vortex shedding frequencies (Liao et al. Citation2003). Muscle activity is lower when a fish slaloms between vortices during Kármán gaiting compared to when swimming in less turbulent flow of comparable velocity (Liao Citation2004). Despite providing energetic benefits in some scenarios, vortices shed from solid cylinders can also destabilise and displace fish, particularly when their diameter is equal to the fish length (Cote and Webb 2015). Based on such evidence, it is likely that CBC design may be optimised to facilitate upstream passage at small-scale structures if appropriate conditions can be created, and undesirable conditions that destabilise fish avoided.
This study assessed how different arrangements of CBC array, retrofitted onto a sloped weir, influence hydrodynamics and the passage efficiency and swimming behaviour of roach (Rutilus rutilus). This was achieved by quantifying: (1) the influence of cluster spacing and diameter, and thus wake width and length, on fish passage (quantified as passage efficiency, time to pass and swim path length); and (2) swimming behaviour when navigating through CBC arrays (where different behaviours were identified as zigzagging, wall associating or lateral traversing). We hypothesised that: (a) passage efficiency is related to the availability of low velocity zones provided sufficient space existed for fish to manoeuvre through the clusters; and (b) fish adopt performance enhancing behaviours that enable them to utilise zones of low velocity when navigating the weir.
Materials and methods
Experimental setup
Experiments were conducted using a weir installed in a rectangular open channel flume (16 m long, 0.6 m wide and 0.8 m deep, ) at the International Centre for Ecohydraulics Research (ICER) laboratory, Boldrewood Innovation Campus, University of Southampton (UK). A staggered array of CBCs, attached to a base, was mounted on the downstream face of the weir (). The CBCs comprised of individual cylindrical elements 0.003 m in diameter (d).
Figure 2. (a) Plan and (b) section of the experimental setup used to quantify passage of roach (Rutilus rutilus) at a model gauging weir with CBC array installed. The weir and extent of the experimental area are shown (dimensions in mm).

To quantify the impact of cluster spacing and diameter on fish swimming, only the diagonal spacing between clusters (Sd) and cluster diameter (D) was intentionally varied between treatments, with the majority of other variables remaining constant. Trials accommodated all combinations of cluster spacings and diameters, resulting in six treatments overall (). Other parameters that may influence fish passage (traverse length, cluster porosity, water depth and velocity) remained constant, with minor adjustments to weir slope to ensure that water depth (and therefore averaged velocity) remained constant between treatments (). To assess whether the change in slope affected fish passage, by reducing the work done by the gravitational force exerted on the fish as it moves from downstream to the crest, the ratio between the work of the drag and gravitational forces for different slopes was estimated. Such a ratio was found to be very large (>20x) for all tests. Therefore, changes in the slope were assumed to have a negligible effect on passage efficiency.
Table 1. Configurations tested during experimentation and their diameters (D), diagonal spacings (Sd), slope (So), temperature (T), average water depth (y), velocity (V) and Froude number (Fr), percentage of “low” velocity area within the array (% LVA) and lateral spacing (c/c)/diameter ratio (Sc/D).
The height of the bristles was 0.1 m. A cluster density φ = Nc d2/D2 (where Nc is the number of elements within the cluster, d is the internal diameter of individual bristle elements and D is the cluster diameter) of 0.1 was selected because this produces the longest wake without creating recirculation immediately downstream of the cluster (Taddei et al. Citation2016). The space between clusters was greater than the body amplitude of the fish to ensure manoeuvrability was not hampered.
An inflow discharge of 0.06 m3 s−1 was maintained constant for all trials. A tilting gate located approximately 8 m downstream of the weir crest was adjusted to ensure the position of the hydraulic jump (and therefore the distance fish were required to swim on the downstream weir face) remained constant (1.67 m) between treatments.
Velocity mapping in the CBC array was undertaken using an electromagnetic flow meter (Valeport Model 801) with a cylindrical sampling range of 20 mm in diameter and a height of 10 mm above the electromagnetic heads (Valeport Limited 1999) at approximately 50% water depth. Data was sampled over a period of 30 seconds producing time-averaged velocity outputs. To capture the hydraulic heterogeneity, velocity was measured at equally spaced lateral and longitudinal intervals that spanned the width and length of the downstream weir face, respectively (see ). Water depth was measured using a point gauge at the same location as for velocity. Time and space averaged water velocity within the array, and more specifically in the wake and in-between the clusters, was calculated. As depth and velocity data violated the assumption of normality (tested using a Shapiro-Wilk test) and could not be corrected through transformation, differences between treatments were compared using a Kruskal-Wallis test. Dunn tests were carried out post hoc to determine where differences occurred between treatments. Time and space averaged velocity throughout the whole array did not differ between treatments (F = 2.13, df = 5, p = 0.060; ), but did so when using data specifically for the wakes [F = 3.95, df = 5, p < 0.010; differences between Low D—High Sd and Intermediate D—Low Sd as well as Low D—Low Sd and Intermediate D—Low Sd] or in-between clusters (F = 20.93, df = 5, p < 0.001; differences were between Low D—High Sd and all other treatments). For all treatments, the mean velocity in the wake of clusters was lower than those in-between (e.g. ). The areas behind and in-between clusters are, therefore, subsequently defined as “low” and “high” velocity, respectively.
Figure 3. Representative (Intermediate D—High Sd treatment) water velocity profile (m s−1) within a CBC array from the crest (0) to the top of the hydraulic jump. Flow direction is denoted by the black arrow, while black dots and purple crosses indicate locations of the clusters (not actual size) and velocity measurements, respectively. The colour map was created by interpolating between these points.

Mean Froude number, calculated using spatially averaged water depths and velocities throughout the array, was greater than or equal to unity for all experiments (). Average (mean) water temperature ranged from 16.9 to 19.3 °C.
Experimental fish and husbandry
Due to the general bias towards strong swimming anadromous species in fish passage research and development i.e. salmonids (Noonan et al. Citation2012), roach were selected for this study because they have comparatively lower swimming capabilities compared to salmonids and are a common easily sourced non-salmonid species found in British rivers. Approximately 200 fish were caught using seine nets on 27 March (2018), 10 days prior to experimentation, from a lake at Caddington (Bedfordshire, UK); and transported in oxygenated tanks containing lake water to the ICER facility. Fish were then distributed equally between three 1200 L holding tanks. A small number of additional fish were delivered in case of mortality.
Water quality was monitored for ammonia, nitrite and nitrate and maintained within suitable levels for fish health (nitrite < 1 mg L−1 and nitrate < 50 mg L−1) through regular part exchange with dechlorinated water. Holding tank temperatures (mean ± SD) were 12.5 ± 0.37 °C. Fish fork length (mean ± S.D: 14.6 ± 0.6 cm, F = 0.99, df = 5, p = 0.42) and mass (mean ± S.D: 47 ± 9 g, X2 = 8.12, df = 5, p = 0.15) did not differ between treatments when tested using an ANOVA and Kruskal-Wallis test, respectively.
Experimental procedure
Experiments were undertaken from the 6 April–4 May 2018 between 08:00 and 23:00 using individual fish. The variation in time of day during which trials were undertaken was equal for all treatments to avoid result bias. A total of 30 replicates were completed for each of the six treatments using one randomly selected fish per trial with individual roach used once only to avoid any potential influence of learned behaviour. Fish were acclimatised to the water temperature of the flume (mean ± SD: 17.7 ± 0.82 °C) for at least 1 hour prior to the start of each trial by being placed within a low water velocity holding area upstream of the weir. On initiation of each trial one fish was released at the farthest extent of the experimental area (approximately 2 m downstream of the base of the weir) and upstream progress through the array was recorded using an overhead Logitech webcam (C920; HD 10809; 30 frames per second; Logitech Pro, Switzerland). Trials were terminated once the fish passed the crest of the weir or after a period of one hour had elapsed. External lighting remained at a constant ambient level with visual cues minimised through the use of a blackout screen installed around the flume. At the end of a trial, fish were removed, measured and weighed to the nearest millimetre and gram, respectively.
Fish passage
Passage efficiency was calculated for each treatment as the number of fish that passed the weir as a percentage of those that attempted (), where an attempt is defined as when the whole body-length of the fish passed upstream of the hydraulic jump. A Fisher’s exact test of association determined the influence of CBC array (treatment) on passage efficiency, comparing number of fish that passed and failed. Data was analysed post hoc using the pairwise Fisher’s exact test.
Table 2. Metrics used to determine the influence of cluster spacing and diameter, and thus wake width and length, on passage efficiency and swimming behaviour of roach (Rutilus rutilus) when navigating through CBC arrays installed on an experimental sloped weir.
Length of fish swim path and time to navigate the weir face were calculated using post processed videography data (Logger Pro). Swim paths were produced by recording the position of the fish’s head in each video frame, generating two-dimensional (x, y) co-ordinates. These were subsequently used to determine the time to pass the weir (from the hydraulic jump to crest) and swim path length (). Data of swim path length and time to pass for all fish that successfully passed under the different treatments was tested for normality using a Shapiro-Wilk test. As data violated the assumption of normality and could not be corrected through transformation, treatments were compared using a Kruskal-Wallis test.
Swimming behaviour
The co-ordinates obtained from the video tracking were used to identify swimming behaviours exhibited by fish when navigating the CBC arrays. Swimming behaviours were categorised as either: (a) zigzagging, (b) wall association or (c) a combination of behaviours a, b or lateral traverse (). No fish pathways exhibited purely lateral traverse through the pass and therefore this behaviour was combined with (c). The percentage of time spent occupying the “low” or “high” velocity regions while navigating the array was calculated to quantify time spent in low velocity zones.
Results
Fish passage
Passage efficiency differed between treatments (p = 0.002, Fisher’s exact test) with differences between High D—Low Sd (85.7%) and both Intermediate D—High Sd (12.5%) and Low D—High Sd (0%). Passage was highest under treatment High D—Low Sd, when the area of “low” water velocity was greatest and Sc/D was lowest (, ). The diagonal dashed line illustrates the relationship between passage efficiency and spacing to diameter ratio. Wake overlap is assumed to occur for Sc/D ratios less than five (illustrated with a vertical dashed line). Time to pass and swim path length did not differ between treatments (Kruskal-Wallis X2 = 8.48, df = 4, p = 0.075 and Kruskal-Wallis X2 = 5.93, df = 4, p = 0.205, respectively). Of the fish that negotiated the hydraulic jump, none passed through the CBC array when clusters were 0.03 m in diameter (the lowest size tested) and spaced 0.15 m apart (Low D—High Sd), even though the mean array velocity was comparable to the other treatments. The wake width, and as a consequence the area of low velocity created, was lowest under this treatment ().
Figure 4. Passage efficiency for each treatment tested is depicted, where passage efficiency is quantified as the number of roach that successfully passed an experimental gauging weir, expressed as a percentage of those that attempted for different array arrangements. The six alternative treatments based on characteristics of a CBC array, are High D—Low Sd (HDLS), Intermediate D—Low Sd (IDLS), Low D—Low Sd (LDLS), High D—High Sd (HDHS), Intermediate D—High Sd (IDHS), Low D—High Sd (LDHS). Sd is the diagonal spacing between clusters, Sc is the lateral spacing between clusters (c/c) and D is cluster diameter in metres. The dashed diagonal line depicts the trend line between passage efficiency and the spacing to diameter ratio.

Table 3. Numbers of roach that attempted, passed and failed, and corresponding passage efficiency for each of the treatments tested.
Swimming behaviour
Swimming behaviour was variable, with 41.4% of fish zigzagging between two longitudinal lines of clusters when passing through the array (). Of the fish that passed, 3.4% exhibited entirely wall-associated behaviours () and 55.2% exhibited combinations of swimming behaviours. This might either be a fish pathway that deviated outside the two longitudinal lines of clusters when zigzagging or one in which they swam diagonally from cluster to cluster, sometimes laterally traversing the entirety of the pass before reaching the edge of the flume and exhibiting localised wall association (as an example track provided in demonstrates). It was noted that the use of lateral traverse during ascent was more frequent for the array configurations with the larger spacing (0.15 m). For fish that passed the High D—Low Sd treatment (for which the most data was available) the percentage of time spent in low velocity zones ranged from 61–90%. As insufficient numbers of fish passed under the other treatments, use of low velocity regions was not calculated.
Figure 5. Examples of swim paths exhibited by roach as they ascended an experimental gauging weir with CBCs installed on the face. The crosses indicate swimming behaviour paths for zigzagging (a) wall association (b) and combination including lateral traversing (c). The arrow indicates the direction of flow.

Discussion
As hypothesised, CBC treatments that generated larger wakes improved passage efficiency of roach at a low-head experimental weir. Passage efficiency was highest for the largest diameter clusters tested (0.07 m) regardless of how far apart they were spaced, suggesting that the larger wakes produced facilitated upstream passage. The suggestion that fish use the wakes is reasonable as they seek to reduce their energy expenditure in a way that is similar to entraining behind cylinders to save energy during upstream movement (Beal et al. Citation2006). In this case, fish did not entrain behind the clusters as their movement through the array was rapid, but the swim paths recorded suggest a similar energy saving behaviour. Similar mechanisms for velocity reduction have been described in other studies that used roughness elements (e.g. Baker and Boubée 2006; Hintermann and Hassinger 2010), although their approach to fish pass design had a greater focus on velocity reduction created through energy loss, and less on the influence of fine scale hydrodynamic connectivity. This body of work highlights the importance of utilisation of low velocity areas in the wake of structures, as supported by Przybilla et al. (Citation2010) and Muraoka et al. (Citation2017).
Passage efficiency was lower for the 0.05 m diameter clusters (the intermediate size tested), with a marked improvement when they were closer together (i.e. spaced 0.1 m rather than 0.15 m apart). This may have been because the wakes did not overlap under the treatment with larger spacings (see Taddei et al. Citation2016), resulting in near wake velocity dissipation and subsequent increase in velocity occurring prior to reaching the next downstream cluster. Under this scenario, there would be less low velocity space available for ascending fish to exploit downstream of each cluster, possibly explaining the lower passage efficiency observed [e.g. Intermediate D—High Sd (D = 0.05, Sd = 0.15)]. Therefore, it is apparent that there needs to be sufficient wake overlap to enable low velocity areas to be interconnected in such a way as to provide a continuous route upstream over the weir. Indeed, previous observations have indicated that without such fine-scale hydrodynamic connectivity, fish passage can be impeded. For example, sculpin (Cottus pollux) struggled to pass through a rock ramp fish pass, a nature like array of roughness elements, when areas of low velocity were not interlinked (Muraoka et al. Citation2017). Our research can be used to show that when continuous low velocity routes are not created, fish passage is compromised. This is supported by Magaju et al. (2020) who tested varying boulder arrangements and heights on fish swimming performance and recommend that consideration of the need to link areas of low velocity is required.
Fish passage over modified weirs is compromised when the density of CBC arrays is high, as fish manoeuvrability is reduced (Montali-Ashworth et al. Citation2020). In this study, fish manoeuvrability was not hampered during weir ascent, even under higher CBC densities, because the ratio between CBC spacing and fish body length remained greater than 1. This suggests there is an optimal design between high and low CBC density that facilitates fish passage at small gauging weirs. Passage is compromised above or below these threshold densities, either because fish are unable to manoeuvre between the clusters or there is insufficient availability of lower velocity areas. Further research is needed to identify the CBC characteristics that will optimise fish passage for different species and body size.
Aggregating zigzagging and combinations of behaviours, 97% of swim paths were sinuous, with some fish spending a large proportion of time positioned behind the clusters with the variation in time spent in lower velocity zones likely dependent on fish swimming ability and the combination of modes used to navigate the array. For example, if fish mainly associate with the wall boundary when navigating the array then they will spend a higher proportion of time in lower velocity zones. Lateral movements during passage through the cluster array were uncommon. Successful ascent over the weir was typically achieved by zigzagging between two lines of clusters; the most direct route to ascend the weir while taking advantage of low velocities behind cylinders. Fish that ascended the weir face in association with the flume wall were able to take advantage of the lower water velocity and shear towards the boundary (e.g. similar to Johnson et al. Citation2019), a strategy that presumably reduced the energy needed to pass. Likewise, fish that moved laterally through the array may also have exploited the low velocity regions in the wake of the bristle clusters. Analysis of swim paths selected indicates that there is a range of strategies that fish adopt to enhance their performance in a complex and challenging hydrodynamic environment. However, further work is needed to quantify the magnitude of relative benefits accrued by employing these different energy saving tactics.
In addition to the advantages of creating CBC wake overlap, array treatments also influenced the overall hydrodynamics of the weir through reductions in bulk velocity. The magnitude of hydraulic resistance created by cluster arrays are influenced by a range of mechanisms, where the most influential are sheltering, delayed separation, and the blockage effect (Etminan et al. Citation2017). Sheltering can be prevalent when densities are high and greater wake overlap occurs, reducing the cumulative energy loss (Nepf Citation1999) and velocity reduction potential of the array. The velocity reduction potential is greatly reduced at low cluster densities in which the large spacings prevent formation of overlapping wakes. Therefore, there should be consideration of optimising design to maximise cumulative energy loss, and as a consequence, velocity reduction potential. This will ultimately increase the passage efficiency of structures as the water velocity will be reduced.
Based on the findings presented, we recommend that a large cluster with a large spacing where Sc/D < 5 would be suitable for multispecies fish passage. Wakes are proposed to overlap when Sc/D < 5 (Taddei et al. Citation2016) with near wake velocity dissipation occurring when Sc/D was greater than 5. This is supported by Nepf (Citation1999), in which sparse vegetation, where no wake overlap occurs, can be characterised as vegetation with density ad (dimensionless population density) <0.1, this translates to a Sc/D ratio > 3.16.
This work has implications for improving passage and understanding fish interactions with their hydrodynamic environment when swimming through a staggered array of bristle clusters. To further improve passage efficiency, it is recommended that the Sc/D ratio is tailored to allow increased availability of low velocity areas created by wake overlap behind each cluster, while also reducing velocity in the array and allowing greater space for fish to manoeuvre. Future work should test prototype CBC arrays in the field and with a range of species and sizes to determine whether the recommendation drawn from this research remains valid under more realistic settings.
Data availability
All data accompanying this paper can be downloaded from the University of Southampton repository at: doi:10.5258/SOTON/D1359
Acknowledgements
We thank Toru Tsuzaki and the ICER team and friends for their technical assistance during experimental periods.
Additional information
Funding
References
- Amaral SD, Quaresma AL, Branco P, Romão F, Katopodis C, Ferreira MT, Pinheiro AN, Santos JM. 2019. Assessment of retrofitted ramped weirs to improve passage of potamodromousfish. Water. 11(12):2441.
- Baker CF, Boubée JA. 2006. Upstream passage of inanga Galaxias maculatus and redfin bullies Gobiomorphus huttoni over artificial ramps. J Fish Biology. 69(3):668–681.
- Beal DN, Hover FS, Triantafyllou MS, Liao JC, Lauder GV. 2006. Passive propulsion in vortex wakes. J Fluid Mech. 549(1):385–402.
- Belletti B,Garcia De Leaniz C,Jones J,Bizzi S,Börger L,Segura G,Castelletti A,Van De Bund W,Aarestrup K,Barry J, et al. 2020. More than one million barriers fragment Europe's rivers. Nature. 588(7838):436–441. doi:10.1038/s41586-020-3005-2. 33328667
- Bunt CM, Castro-Santos T, Haro A. 2012. Performance of Fish passage structures at upstream barriers to migration. River Res Appl. 28(4):457–478.
- Cote AJ, Webb PW. 2015. Living in a turbulent world - a new conceptual framework for the interactions of fish and eddies. Integr Comp Biol. 55(4):662–672.
- Deinet S, Scott-Gatty K, Rotton H, Twardek WM, Marconi V, McRae L, Baumgartner LJ, Brink K, Claussen JE, Cooke SJ, et al. 2019. The Living Planet Index (LPI) for migratory freshwater fish - Technical Report. World Fish Migration Foundation, The Netherlands.
- Etminan V, Lowe RJ, Ghisalberti M. 2017. A new model for predicting the drag exerted by vegetation canopies. Water Resour Res. 53(4):3179–3196.
- Hintermann M, Hassinger R. 2010. Water Policy Office Haus Der Demokratie Und Menschenrechte Greifswalder. Bristled Fish Pass – the Au-Schönenberg Hydroelectric Dam Pilot project, fish migration assistance, hydropower, biological permeabilityStr. 4, D - 10405 Berlin: GRUNE LIGA e.V.
- Johnson K, Wait LE, Monk SK, Rader R, Hotchkiss RH, Belk MC. 2019. Effects of substrate on movement patterns and behavior of stream fish through culverts: an experimental approach. Sustainability. 11(2):470.
- Jones J, Börger L, Tummers J, Jones P, Lucas M, Kerr J, Kemp P, Bizzi S, Consuegra S, Marcello L, et al. 2019. A comprehensive assessment of stream fragmentation in Great Britain. Sci Total Environ. 673:756–762.
- Kemp PS. 2015. Impoundments, barriers and abstractions: impact on fishes and fisheries, mitigation and future directions. In: J. F. Craig, editor. Freshwater fisheries ecology. Chichester, GB: Wiley. p. 717–769.
- Kemp PS, Russon IJ, Waterson B, O’Hanley JR, Pess GR. 2008. Recommendations for a “Coarse-Resolution Rapid-Assessment" methodology to assess barriers to fish migration and associated prioritization tools - Final report. Tech. rep., Scottish Environment Protection Agency, Stirling, UK.
- Kerr JR, Manes C, Kemp PS. 2016. Assessing hydrodynamic space use of brown trout, Salmo trutta, in a complex flow environment: a return to first principles. J Exp Biol. 219(21):3480–3491.
- Liao JC. 2004. Neuromuscular control of trout swimming in a vortex street: implications or energy economy during Kármán gait. J Exp Biol. 207(20):3495–3506.
- Liao JC. 2007. A review of fish swimming mechanics and behaviour in altered flows. Philos Trans R Soc Lond B Biol Sci. 362(1487):1973–1993.
- Liao JC, Beal DN, Lauder GV, Triantafyllou MS. 2003. The Kármán gait: novel body kinematics of rainbow trout swimming in a vortex street. J Exp Biol. 206:1059–1073.
- Lothian AJ, Gardner CJ, Hull T, Griffiths D, Dickinson ER, Lucas MC. 2019. Passage performance and behaviour of wild and stocked cyprinid fish at a sloping weir with a Low Cost Baffle fishway. Ecol Eng. 130:67–79.
- Lucas MC, Bubb DH, Jang M, Ha K, Masters JEG. 2009. Availability of and access to critical habitats in regulated rivers: effects of low-head barriers on threatened lampreys. Freshw Biol. 54(3):621–634.
- Magaju D, Montgomery J, Franklin P, Baker C, Friedrich H. 2020. A new framework for assessing roughness elements in promoting fish passage at low-head instream structures. J Ecohydr. 5(2):152–164.
- Montali-Ashworth D, Vowles AS, De Almeida G, Kemp P. 2020. Use of Cylindrical Bristle Clusters as a novel multispecies fish pass to facilitate upstream movement at gauging weirs. Ecol Eng. 143:105634.
- Montgomery JC, Mcdonald F, Baker CF, Carton AG, Ling N. 2003. Sensory integration in the hydrodynamic world of rainbow trout. Proc Biol Sci. 270(2):195–197.
- Muraoka K, Nakanishi S, Kayaba Y. 2017. Boulder arrangement on a rocky ramp fishway based on the swimming behaviour of fish. Limnologica. 62:188–193.
- Nepf HM. 1999. Drag, turbulence and diffusion in flow through emergent vegetation. Water Resour Res. 35(2):479–489.
- Nicolle A. 2009. Flow through and around groups of bodies [thesis]. University College London.
- Noonan MJ, Grant JWA, Jackson CD. 2012. A quantitative assessment of fish passage efficiency. Fish Fish. 13(4):450–464.
- Przybilla A, Kunze S, Rudert A, Bleckmann H, Brucker C. 2010. Entraining in trout: a behavioural and hydrodynamic analysis. J Exp Biol. 213 (17):2976–2986.
- Taddei S. 2016. Turbulent flows interacting with groups of obstacles [thesis]. University of Southampton.
- Taddei S, Manes C, Ganapathisubramani B. 2016. Characterisation of drag and wake properties of canopy patches immersed in turbulent boundary layers. J Fluid Mech. 798:27–49.
- Tummers JS, Kerr J, O'Brien P, Kemp P, Lucas MC. 2018. Enhancing the upstream passage of river lamprey at a microhydropower installation using horizontally-mounted studded tiles. Ecol Eng. 125:87–97.
- Zong L, Nepf H. 2012. Vortex development behind a finite porous obstruction in a channel. J Fluid Mech. 691:368–391.