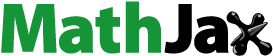
ABSTRACT
Oversize rock fragments are highly undesired in a sublevel caving (SLC) operation as they affect the production cycle, equipment, and infrastructure. In this study, a field test was carried out in Malmberget mine to analyse the impact of oversize fragments on the production cycle and the costs of different procedures for handling such fragments. The tests involved monitoring of dumping oversize fragments in two orepasses, one with a grizzly and the other one without a grizzly, using cameras. The cycle times of load-haul-dump (LHD) machines were determined for both orepasses. The results indicate that the grizzly increased the availability and productivity of the orepass despite increasing the cycle time of the LHD machines. Moreover, installation of a boulder breaker system along with the grizzly can further increase the productivity and the cost of such a system will be paid off in a shorter time in terms of enhanced productivity.
Introduction
Oversize fragments, or boulders, are the most undesired rock fragmentation in the mining industry. An oversize rock fragment can be defined as ‘any fragment produced from primary blasting, that cannot be adequately handled by the standard loading, hauling and crushing equipment, used in an operation’ (Singh and Narendrula Citation2010). These fragments mostly require secondary breakage and have various implications for mining operations, for example, delays in loading, increased production cycle times, increased damage risk and wear of loading and hauling equipment, secondary breakage costs, and additional maintenance costs for crushers if such fragments are not handled before crushing (Kumar Citation1997; Singh and Narendrula Citation2010). These lead to production losses and increased costs of material handling. As the production disturbances from oversize fragments vary from site to site depending on the capacity and dimensions of the loading, hauling, and crushing equipment (Singh and Narendrula Citation2010), a general size recommendation cannot be given that suits all operations. Instead, recommendations need to be site-specific. The purpose of this paper is to show the impact of oversize fragments on the production cycle of SLC, looking at orepasses with and without a grizzly. It estimates the costs of boulder handling at LKAB’s SLC mine in Malmberget, Sweden.
Oversize fragments in sublevel caving
In sublevel caving, oversize fragments are highly undesired because they can obstruct the material flow in the blasted rings and cause hazardous hang-ups, leading to safety issues and reduced ore recovery if the hang-ups cannot be removed (Danielsson et al. Citation2017). They can significantly affect the production cycle and create problems in efficient handling of the ore through subsequent stages (Kumar Citation1997). They can be characterized based on several different factors, for example, draw point dimensions, load-haul-dump (LHD) bucket dimensions, and orepass dimensions.
Oversize fragments are commonly observed at draw points in all caving methods. The authors earlier studied the occurrence and frequency of oversize fragments in Swedish SLC mines. Manzoor et al. (Citation2022a) studied the boulder occurrence for 20 production rings and found that more than 15% of the total LHD buckets loaded from the draw points contained oversize fragments. In another study, Manzoor et al. (Citation2022b) found that the percentage of oversize fragments increases with the increasing material extraction from the rings. The oversize fragments do not occur often when material is being extracted from a recently blasted ring, but at higher excavation ratios, up to 25% of buckets can contain boulders. shows the variation in oversize fragment occurrence with increasing material extraction from the ring.
Figure 1. Occurrence of oversize fragments (modified from Manzoor et al., Citation2022b).

Orepasses
Orepasses transfer broken rock from higher to lower levels in a mine (Skawina et al. Citation2022; Sredniawa et al. Citation2022; Szwedzicki, Citation2007) and their proper functioning is important to a production cycle in any SLC operation. A primary reason for orepass hang-ups is the dumping of large rock fragments into the orepasses. The bigger fragments can form interlocking arches or domes and cause stoppages in the orepasses (Szwedzicki, Citation2007). The risk of hang-ups also increases when the orepasses that are being used are fully filled as compared to when they are empty or partially filled (Skawina et al. Citation2022).
However, there is often a risk of orepass failure or stoppage during material transportation that can affect operational capacity and productivity (Bunker et al. Citation2015; Skawina et al. Citation2018, Citation2021), and in particular, hang-ups or blockages, which make the orepasses unavailable (Szwedzicki, Citation2007). Therefore, the orepasses are bottlenecks for the production cycle as the mine production is reduced if they are no longer functional (Skawina et al. Citation2018; Szwedzicki, Citation2007). This may also disturb or stop the LHD machines (Gustafson et al. Citation2014) if trucks cannot be used to transport the material from the production area or the LHD machines cannot be moved to another mining area.
Along with production losses, orepass hang-ups can cause safety issues, as the arches suddenly can crash down (Szwedzicki, Citation2007). Oversize fragments can damage the orepass walls and lead to increased orepass maintenance costs even if they don’t block the orepass (Kumar Citation1997). They can also get stuck in the chute and disturb the material transportation at the haulage level (Gustafson et al. Citation2016a). Blasting is commonly used to remove hang-ups caused by oversize fragments, but this can damage the orepass walls or the chute (Bunker et al. Citation2015; Skawina et al. Citation2022). Furthermore, orepass restoration is an expensive, challenging, and time-consuming process (Hadjigeorgiou and Stacey Citation2013).
The orepass diameter (D) is critical for the free flow of material through the orepass. shows guidelines given in the literature based on the relations between the orepass diameter and the size of the largest rock fragment (d).
Table 1. Orepass dimension for free flow of material.
If the D/d ratio is less than the ones mentioned in , the flow of material is uncertain; the probability of material not flowing through the orepass further increases with increasing fragment size.
Screening at orepasses
To prevent the dumping of oversize fragments into orepasses, screening devices can be installed at the entrance of the orepass (Gustafson et al., Citation2016b; Hadjigeorgiou et al. Citation2005; Hadjigeorgiou and Stacey Citation2013; Koivisto Citation2017). A screening device can be a grizzly, a scalper, or a mantle (Gustafson et al., Citation2016b; Hadjigeorgiou et al. Citation2005; Hadjigeorgiou and Stacey Citation2013; Koivisto Citation2017). The primary difference between these screening devices is the shape of the opening that the material passes through. The grizzly has a square, the scalper has a rectangular while the mantle has a circular opening (Hadjigeorgiou et al. Citation2005). A grizzly is considered the best device for this purpose because of its greater durability and lesser demands on maintenance (Gustafson et al., Citation2016b; Hadjigeorgiou et al. Citation2005; Koivisto Citation2017). However, several researchers (Gustafson et al., Citation2016b; Hadjigeorgiou et al. Citation2005; Koivisto Citation2017) have concluded that if the size of the grid is incorrect, it can have a negative impact on production. Sjöberg et al. (Citation2003) recommended screening devices to increase orepass stability for a mine with SLC operation in Sweden. Although screening increases the operational reliability of the orepass, Hadjigeorgiou and Stacey (Citation2013) found mining operations were often reluctant to invest in such devices, and workers did not accept them, claiming a negative impact on the production rate.
If an oversize fragment is dumped on the grizzly without any boulder breaking system close by, the boulder must be removed and transported to another location (e.g. a boulder drift) to be broken down into smaller pieces, thus leading to increased cycle time for the LHD machines (Koivisto Citation2017). Stewart et al. (Citation1999) and Costello and Knights (Citation2012) reported that some mines have abandoned the use of grizzlies because of their negative impact on productivity. Alternatively, the LHD operator can separate and remove the oversize fragments at the muck pile (Hadjigeorgiou et al. Citation2005; Koivisto Citation2017) and transport them directly to the boulder drift for secondary breaking, but this will naturally slow down the production.
In any SLC mine, the material and production cycle on a loading level is a trade-off between production rate, damage to infrastructure, and operators’ training or company investment in boulder control measures. To correctly perform this trade-off, it must be possible to estimate the cost of boulder handling.
Procedure for handling oversize fragments at LKAB
LKAB operates two SLC mines in Sweden: Malmberget mine and Kiirunavaara mine. The procedure for handling oversize fragments in these mines is quite different. At the mine, oversize fragments are defined as fragments larger than 1 m × 1 m × 1 m (Gustafson, et al., Citation2016a); see .
Kiirunavaara mine
In Kiirunavaara mine, grizzlies, in combination with remotely controlled boulder breakers, are used in all orepasses. When the LHD machine dumps the material over the grizzly, a person sitting in a remote-control room monitors the grizzly through camera surveillance (Drakenberg Citation2007). If an oversize fragment is identified at the grizzly, the person in the control room breaks the fragment using the remotely controlled boulder breaker (Drakenberg Citation2007). Also, the orepasses are grouped together so if an orepass is unavailable, the LHD machine will dump the material to the nearby orepass.
In this case, the LHD operator does not need to sort out oversize fragments during the loading process and does not need to wait to break the oversize material at the grizzlies. Therefore, the production will not be disturbed, and the operational reliability will be high.
Malmberget mine
In Malmberget mine, no grizzlies or other infrastructure are routinely used to prevent oversize fragments from being loaded into the orepass. Instead, the system is dependent on the LHD operator’s skills and discipline to visually identify and separate boulders for secondary breaking. The procedure for the loading operation at Malmberget is shown in .
In the mine, the orepass diameter is 3 m which gives a D/d of 3 considering oversize dimension of 1 m3. With this low D/d ratio as compared to , compliance with the boulder limit is essential and can be achieved either by screening at orepasses or by the strict discipline of LHD operators. Therefore, the operator’s decision at the loading level is important for overall operational efficiency. shows the relations between the boulder limit and the machine and drift dimensions; the figure emphasizes the difficulty of defining boulders in this operational environment.
The reason for the major difference in the mines’ boulder handling may be the difference in the total ore volume per level of the orebodies. The orebodies in Malmberget mine are normally scattered and have smaller volumes per level, while Kiirunavaara mine has one massive orebody. The relative cost for the installation of the remotely controlled system used in Kiirunavaara may be considered too high for smaller ore bodies.
Methodology
This study analyses the impact of oversize fragments on the production cycle for orepasses with and without a grizzly. The research was carried out at LKAB’s Malmberget iron ore mine and included a literature review, installation of cameras over two orepasses, installation of a grizzly over one of the orepasses, analysis of LHD loading data, and comparative cost analysis. The limitation of the test layout is that it compares an orepass without a grizzly to an orepass with a grizzly, but without an appropriate boulder breaker.
Test description
Malmberget mine (owned and operated by LKAB) is the second largest underground iron ore mine in the world (Shekhar et al. Citation2019). The mining area includes 20 orebodies divided into eastern and western fields. The eastern field contains massive magnetite deposits, while the western field contains smaller magnetite-hematite orebodies (Lund Citation2013). The mine has 18 orepasses, 17 of which are located in the eastern field (2 have been abandoned) and 1 in the western field. The mine uses the SLC mining method. The loading operation uses LHD machines with an average loading capacity of 1 tonnes.
This study was done in the eastern field, in one of the largest ore bodies, ‘Alliansen’, at production level 1082 m; see . The fragmentation statistics given by Manzoor et al. (Citation2022a, Citation2022b) for another level of the same orebody suggest that more than 15% of the loaded LHD buckets contain oversize fragments which increases to approximately 25% during the later stages of material extraction from the rings. The production level is served by two orepasses, orepass 216 and orepass 217; both are used to transport material to the main haulage level at 1250 m. Both orepasses are inclined at 60° with a diameter of 3 m. However, the wear and tear and rock stresses tend to change the orepass diameter over the operational life of the orepass (Gustafson et al., Citation2016a). A boulder drift between the orepasses is specifically assigned as a place to dump oversize fragments; they are then broken down by secondary blasting before being dumped into the orepasses.
During the test, orepass 216 was covered by a grizzly to stop oversize fragments from entering into the orepass. The mesh size of the grizzly was the same as the defined oversize fragment size limit, 1 × 1 m. shows the orepass covered with the grizzly (a) and a sketch of the grizzly from above (b). Orepass 217 was open without a grizzly, according to the normal operating procedure at the mine; thus, it was possible to dump oversize fragments into the orepass.
Oversize monitoring at the orepasses
To record the material being dumped into the orepasses, cameras were installed over the orepasses as shown in . A third camera was installed in the boulder drift to record the number of oversize fragments taken to the boulder drift. The cameras were set to record a short video whenever there was a movement under them. The filming area was properly illuminated using LED lights to yield better-quality videos in the dark mining environment.
Test period
The test was performed between May 2018 and the end of 2019. Several modifications and repairs were done on the grizzly during this period. The filming set-up described above also had some initial technical problems and was improved and modified. The selected measurement period for the analysis, presented in this paper, started in autumn 2019, when all technical issues were solved, and the operation was used to the system. The analysis is based on 12 weeks of LHD loading data out of which 6 weeks had continuous video recordings, resulting in more than 12,000 videos. Due to the time constraints the video recordings for the first 3 weeks were analysed manually to observe the occurrence and impact of oversize fragments. Some examples of oversize fragments observed in this period are shown in .
Results and discussion
Productivity comparison
If the procedure described in always worked, there would be no difference between the open orepass (217) and the orepass equipped with a grizzly (216). However, if material exceeding the boulder limit is brought to orepass 216, it will be obstructed by the grizzly. This will require additional time, either to handle the boulder at the grizzly or to transport it to the boulder drift.
Ideally, oversize fragments that cannot pass the grizzly should be picked up and transported back to the boulder drift. However, the video recordings show that boulders are frequently broken down over the grizzly, with the LHD bucket used as a hammer. In the short term, this may improve productivity, if boulders can be broken quickly with low force. However, if this is not the case, the cycle time may increase significantly. During the 3-week detailed manual analysis of recordings, on two occasions, the oversize fragment got stuck in the grizzly when it was forced into the orepass by the LHD bucket. The orepass was unavailable for approximately 18 h, while the boulder was drilled and blasted. The use of LHD bucket as a hammer should not occur, as this could result in costly damage and increase wear and tear on both the grizzly and the LHD machine. During the analysed test period, around 14% of the buckets dumped in orepass 216 and more than 20% of the buckets dumped in orepass 217 contained oversize fragments.
To estimate the actual productivity difference between loading to the orepasses with and without the grizzly, the cycle times for each scenario were compared. shows a cumulative density plot for the cycle times for each scenario.
The low end of the graph is similar for the orepasses with and without a grizzly. Thus, for fine or medium fragmented ore without oversize fragments, there should be no difference between the two orepass alternatives as the grizzly does not obstruct the flow of material for such fragmentation. However, between 70% and 90% (the magnified part of the figure), the cycle time is significantly longer for the orepass with a grizzly than for the one without a grizzly. As mentioned previously, up to 25% of the buckets in the mine may contain oversize fragments, and the observed loading procedure for the orepass with the grizzly described above is likely to be the reason for the longer cycle time.
For the entire test period (cycle times between 0 and 20 min) the average cycle time for the orepass without a grizzly was 4 min and 1 s, and for the orepass with the grizzly, it was 4 min and 21 s. This means the grizzly alone reduces the productivity by almost 10% because of the extra time spent on removing the oversize fragments from the grizzly or pushing them through the grizzly with the bucket. With a boulder breaker, like the system used in Kiirunavaara, the operator will be less affected by problems caused by oversize fragments; thus, there should be little or no reduction in productivity. However, for areas with a single orepass, waiting times can occasionally occur, if the boulder breaking time is longer than the LHD cycle time.
The shorter registered cycle time for the orepass without a grizzly depends on the absence of a grizzly at the entrance of the orepass. Bucket loads containing oversize fragments, that should have been separated and brought to the boulder drift for secondary breakage, were instead dumped in to the orepass, leading to short cycle times and very efficient operation at the loading level. However, loading oversize fragments into the orepass may lead to orepass breakage or hang-ups that stop ore flow and prevent loading in the orepass for longer periods.
The frequency of dumping oversize fragments into the orepass without a grizzly (217) was observed from the video recordings and compared to longer stoppages of the orepass. shows the frequency of dumping oversize fragments before and after a major stoppage.
As the figure shows, 22 out of the last 34 buckets dumped into the orepass before the work stoppage on 27-10-2019 contained oversize fragments (marked red in the figure). These 22 buckets contained 27 oversize fragments (some buckets contained several oversize fragments). Of these 27 oversize fragments, 13 were bigger than 2 × 2 m; this means a D/d ratio of less than 1.5 and a very high probability of hang-up. In this case, ore flow stopped at 06:50 pm on 27-10-2019, and the stoppage continued until 05:37 am on 29-10-2019.
On another occasion, the dumping was stopped at the beginning of the morning shift and resumed four days later. Before the stoppage, 14 buckets containing four oversize fragments were dumped into the orepass. Two of these four oversize fragments were bigger than 2 × 2 m, again resulting in a D/d ratio less than 1.5. In general, longer delays between successive dumps were more frequently observed for orepass 217 (without a grizzly) than for orepass 216 (with a grizzly). It is important to note that all the oversize fragments do not cause hang-ups. There are various factors which contribute towards a hang-up such as size, shape, and number of the oversize fragments. Therefore, the occurrence of a hang-up is described in probability which gets higher if the fragments are bigger or many oversize fragments are dumped successively as shown in .
Impact of grizzly on production
To compare the two alternatives, orepasses with and without a grizzly, different cost parameters were estimated as listed below.
Average bucket capacity of LHD machine at the mine = 21 tonnes
Target profit (approximately) per tonne of ore at mine = 274 SEK ∼ 26.5 USD
Average cycle time for orepass without grizzly = 4.02 min
Average cycle time for orepass with grizzly = 4.35 min
The hourly production of an LHD can be calculated as given in Equation (1).
(1)
(1) Number of buckets per hour = 60 min/cycle time for the LHD
BC = average bucket capacity of the LHD
Using Equation (1), the hourly production of an LHD for the orepass without the grizzly, was 313 tonnes and with the grizzly, was 290 tonnes. This means that the grizzly reduced the hourly production of the orepass by approximately 7%.
However, more buckets were dumped in orepass 216 than orepass 217 over a period of 12 weeks. A total of 12760 buckets were dumped in orepass 216, while 8747 buckets were dumped in orepass 217. One possible reason of higher productivity of one orepass than the other, can be the proximity of the orepass to the active production drifts. However, no such pattern could be clearly seen in the loading data and there was no available plan for prioritizing one orepass over the other. Therefore, two scenarios were considered for the cost estimation of the impact of having a grizzly over the orepass.
Case 1: Both of the orepasses were equally good for dumping the material without any preference and the lower productivity of orepass 217 was assumed to be due to the more frequent disturbances and stoppages at the orepass.
Case 2: Orepass 216 had priority over the orepass 217 for dumping the material as it was closer to the production drifts so orepass 217 had a lower productivity.
Cost estimation for installing a boulder handling system: Case 1
Considering that both orepasses were equally good for dumping, more buckets in orepass 216 suggest that the installation of the grizzly reduced the orepass stoppages and could result in an increased production of the orepass. The increase in production is calculated as below.
Difference in buckets for 12 weeks = 12,760–8747 = 6987 buckets
Difference in buckets per week = 4013/12∼334 buckets
Production difference = 334 × 21 = 7014 tonnes per week
If the grizzly is used together with a boulder breaker and the LHDs do not need to wait for boulder breaking at the orepass, short-term productivity loss could be avoided and would result in production improvement of more than 7014 tonnes per week. It would result in an extra profit of 185,871 USD per week (7014 × 26.5 USD per tonne). An oversize handling system for an orepass (grizzly and remote-controlled boulder breaker) like the one used in Kiirunavaara costs approximately 1.45 million USD (personal communication, 2022). Given the production losses noted above, the cost of such a system could be paid off in Malmberget mine in less than two months assuming the production improvements are solely due to the grizzly.
Cost estimation for installing a boulder handling system: Case 2
Considering priority of orepass 216 for dumping the material, the number of buckets dumped in both orepasses cannot be used for cost estimation. Therefore, the loss in production due to handling the oversize fragments over the grizzly was calculated. The extra time spent by LHD machines in breaking down the oversize fragments was noted down from the video recordings. During three weeks of analysed video recordings, a total of 3736 buckets were dumped into the orepass 216 out of which 527 contained oversize fragments. The LHD machines spent approximately two minutes on average to break down the oversize fragments with some of them taking lesser and some of them taking more time depending on the size and material composition of the fragment. This resulted in 18 h and 26 min (18.43 h) without production in just three weeks since the LHD was handling boulders instead.
Considering a normal LHD cycle time of 4.02 min i.e. without grizzly, an LHD loads 15 buckets in an hour which results in approximately 315 tonnes of ore per hour. So, for three weeks of production, almost 5806 (i.e. 18.43 × 315) tonnes of ore were not loaded because the LHD spent that time on hammering the oversize fragments over the grizzly. It results in an estimated production loss of 153 859 USD (i.e. 5806 × 26.5 USD per tonne) for these three weeks. Given this production loss, the cost of an oversize handling system could be paid off in Malmberget mine in approximately 6.6 months with the assumption that the LHDs does not need to wait for the boulder breaker to clear the orepass i.e. it takes less than 4 min to clear the orepass from the oversize fragment.
Additionally, during the 3-week detailed analysis, the orepass became unavailable on two occasions for a total of approximately 18 h due to oversize fragments being stuck in the grizzly and needed to be drilled and blasted over the grizzly. In that case, the LHD needs to travel to the next orepass, which may affect the production if that orepass is assigned for dumping the material from another area. Also, if the other orepass becomes unavailable due to any issue like hang-up or collapse, etc., the production can come to halt resulting in a substantial financial impact.
Comparing payoff time for both cases
The payoff time for an oversize handling system is calculated for two scenarios. It is important to note the assumptions behind these calculations. The cost of an oversize handling system could be paid off in a maximum time of approximately 6.6 months (case 2). However, the production loss when the grizzly was blocked with the oversize fragments was not included in the calculations. So, the actual payoff time may be shorter than the calculated. Similarly, the payoff time for case 1 is shorter i.e. less than 2 months but the calculations were based on the assumption that the productivity difference, with 6987 more buckets dumped in orepass 216, was solely due to the grizzly. However, higher productivity of the orepass 216 could also be due to the proximity of the orepass to the production drifts although no such evidence was found in this study. So, the actual payoff time may be longer than the calculated 2 months. Also, the target profit per tonne of ore was calculated from economic reports of the mine which could change depending upon the price variations and other factors such as inflation, taxation, etc. Therefore, the payoff time may be different for any future installations, if the target profit changes.
Finally, it is important to underline that this study only dealt with the production consequences of orepass screening. A screening device would also protect orepasses and prevent wear and damage, leading to less costly maintenance and restoration and associated production disturbances. A reduction of LHD maintenance costs is also likely if the boulders are not broken by the LHD bucket.
Conclusions
This study evaluated the impact of dumping oversize fragments in orepasses with and without a screening infrastructure. Based on the findings, the following conclusions can be drawn:
Orepasses without grizzlies require skilled and disciplined operators to identify and separate oversize fragments at the muck pile and bring them to the boulder drift. Otherwise, there are increased productivity risks, such as orepass stoppages and hang-ups caused by oversize fragments.
Screening devices over the orepasses are important, as they reduce the probability of hang-ups and increase the orepass availability and operational reliability.
Despite the high cost of installing an oversize handling system, the cost for Malmberget mine could be paid off in a shorter time based solely on productivity improvements.
Acknowledgements
The authors acknowledge LKAB, and the staff and management of Malmberget and Kiirunavaara mines for valuable input and support during the study. Vinnova, the Swedish Energy Agency, and Formas are acknowledged for financing the project ‘Face-to-Surface II’ through the SIP-STRIM program. Finally, the authors would like to acknowledge the support from the Centre for Advanced Mining and Metallurgy (CAMM2), Sweden.
Disclosure statement
No potential conflict of interest was reported by the author(s).
Additional information
Funding
References
- Aytaman V. 1960. Causes of hanging in ore chutes and its solution. Can Min J. 81:77–81.
- Bunker K, Campbell A, O’Toole D, Penney A. 2015. Guidelines for orepass design in a sublevel cave mine. Proceedings of the International Seminar on Design Methods in Underground Mining, Australian Centre for Geomechanics, Perth, p. 585–600. doi:10.36487/ACG_rep/1511_37_Bunker.
- Costello C, Knights P. 2012. Grizzly modifications at Ridgeway Deeps Block Cave Mine. Mining Education Australia (MEA) Student Conference, Kalgoorlie, WA, Australia, p. 13–18.
- Danielsson M, Ghosh R, Navarro J, Johansson D, Schunnesson H. 2017. Utilizing production data to predict operational disturbances in sublevel caving. 26th International Symposium on Mine Planning and Equipment Selection (MPES2017), Luleå, Sweden, p. 139–144.
- Drakenberg M. 2007. An analysis of the boulder-handling system at the Kiruna mine, LKAB [Master’s Thesis]. Luleå University of Technology, Sweden.
- Gustafson A, Johansson D, Schunnesson H. 2016b. Face to Surface: a fragmentation study [Research Report]. Luleå University of Technology, Luleå, Sweden.
- Gustafson A, Jonsson K, Johansson D, Schunnesson H. 2016a. “From Face to surface” – a fragmentation study. Sydney, Australia, 7th International Conference and Exhibition on Mass Mining (MassMin 2016), 9-11 May 2016. Sydney, Australia, p. 555–562.
- Gustafson A, Lipsett M, Schunnesson H, Galar D, Kumar U. 2014. Development of a Markov model for production performance optimisation. Application for semi-automatic and manual LHD machines in underground mines. Int J Min Reclam Environ. 28(5):342–355. doi:10.1080/17480930.2013.862026.
- Hadjigeorgiou J, Lessard JF. 2007. Numerical investigations of ore pass hang-up phenomena. Int J Rock Mech Min Sci. 44(6):820–834. doi:10.1016/j.ijrmms.2006.12.006.
- Hadjigeorgiou J, Lessard JF, Mercier-Langevin F. 2005. Ore pass practice in Canadian mines. J S Afr Inst Min Metall. 105:809–816.
- Hadjigeorgiou J, Stacey TR. 2013. The absence of strategy in orepass planning, design, and management. J S Afr Inst Min Metall. 113:795–801.
- Hambley DF, Singh MM. 1983. Guidelines for open-pit ore pass design. Volume 2. Design Manual. Washington, DC.
- Joughin A, Stacey TR. 2004. The behaviour of ore passes in deep level tabular mines. Second International Seminar on Deep and High Stress Mining, SAIMM, Johannesburg, p. 395–411.
- Koivisto M. 2017. Ore Pass Design and Placement [Master’s Thesis]. Delft, Netherlands: Delft University of Technology.
- Kumar U. 1997. Study of problems caused by oversized boulders in a mine production system: a case study. Int J Surf Mining, Reclamation Environ. 11(2):69–73. doi:10.1080/09208119708944062.
- Kvapil R. 1965. Gravity flow of granular material in Hoppers and Bins in mines – II coarse material. Int J Rock Mech Min Sci. 2:277–304.
- Lund C. 2013. Mineralogical, chemical and textural characterisation of the Malmberget iron ore deposit for a geometallurgical model [PhD dissertation]. Luleå, Sweden: Luleå University of Technology.
- Manzoor S, Gustafson A, Johansson D, Schunnesson H. 2022b. Rock fragmentation variations with increasing extraction ratio in sublevel caving: a case study. Int J Min Reclam Environ. 36(3):159–173. doi:10.1080/17480930.2021.2000826.
- Manzoor S, Gustafson A, Schunnesson H, Tariq M, Wettainen T. 2022a. Rock fragmentation measurements in sublevel caving: field tests at LKAB’s Malmberget mine. Caving 2022: Fifth International Conference on Block and Sublevel Caving, Perth, p. 381–392. doi:10.36487/ACG_repo/2205_26.
- Peele R. 1947. Mining engineers’ handbook. 3rd ed., Vol. 1. New york: John Wiley & Sons Inc.
- Shekhar G, Gustafson A, Hersinger A, Jonsson K, Schunnesson H. 2019. Development of a model for economic control of loading in sublevel caving mines. Min Technol. 128(2):118–128. doi:10.1080/25726668.2019.1586371.
- Singh SP, Narendrula R. 2010. Causes, implications and control of oversize during blasting. 9th International Symposium on Rock Fragmentation by Blasting, Granda, Spain, p. 311–317.
- Sjöberg J, Lundman P, Nordlund E, Quinteiro C. 2003. Stability analysis of ore passes in the Kiirunavaara mine. ISRM 2003–Technology Roadmap for Rock Mechanics, South African Institute of Mining and Metallurgy, Sandton Convention Centre, 2003, p. 1093–1098.
- Skawina B, Greberg J, Salama A, Gustafson A. 2018. The effects of orepass loss on loading, hauling, and dumping operations and production rates in a sublevel caving mine. J South Afr Inst Min Metall. 118(4):409–418. doi:10.17159/2411-9717/2018/v118n4a11.
- Skawina B, Salama A, Gunillasson J, Strömsten M, Wettainen T. 2022. Comparison of productivity when running filled, near-empty, or flow-through orepass using discrete event simulation. Mining. 2(2):186–196. doi:10.3390/mining2020011.
- Skawina B, Shekhar G, Strömsten M, Wettainen T. 2021. Analysing reduced conveying capability of the ore passes systems in Malmberget mine. Proceedings of the Underground Operators Conference, Perth, Australia.
- Sredniawa W, Skawina B, Rapp J, Shekhar G, Gunillasson J. 2022. Longevity chart for planning production and the renovation of orepasses. Caving 2022: Fifth International Conference on Block and Sublevel Caving, Australian Centre for Geomechanics, Perth, p. 369–380. doi:10.36487/ACG_repo/2205_25.
- Stewart BM, Iverson SR, Beus MJ. 1999. Safety considerations for transport of ore and waste in underground orepasses. Min Eng. 51(3):53–60. https://www.cdc.gov/niosh/mining/works/coversheet284.html.
- Szwedzicki T. 2007. Formation and removal of hang-ups in ore passes. Min Technol. 116(3):139–145. doi:10.1179/174328607X191047.