ABSTRACT
Any effective nuclear disarmament regime will need to consider how to deal with the infrastructure that supported the development and maintenance of nuclear weapons. Such infrastructure carries within it the seeds for re-armament. This paper presents a framework of potential actions to be applied to facilities within nuclear weapons production complexes in a disarming and a disarmed world. This framework has two axes. The first identifies key capabilities and facilities that are integral to the production of both simple fission weapons and more advanced boosted and thermonuclear weapons. The second axis explores the range of actions that can be taken to constrain nuclear weapons production infrastructure which would either directly or indirectly influence the capacity for future reacquisition of nuclear weapons. In exploring these themes, the paper asks what are the crucial nuclear facilities or processes that are likely to be key in enabling potential re-armament? And what types of actions can be considered to constrain the re-armament potential of these facilities? Considering how to enhance the degree of disarmament irreversibility will entail posing and answering difficult questions on the fate of nuclear weapons complexes, and not just nuclear weapons.
Introduction
Nuclear disarmament can be thought of as simply the elimination of all nuclear weapons from national arsenals. However, such a disarmed world would retain the capability to rearm, building back nuclear weapons, in relatively short order. Any effective disarmament regime will therefore also need to consider what to do with the infrastructure that supported nuclear weapons development and maintenance and could likely enable future re-armament if left in place. In other words, any comprehensive disarmament regime would have to make choices regarding whether to apply controls on either some of, or all, the various existing nuclear facilities and infrastructure. This is particularly relevant to irreversibility as defined in the introduction to this special issue: as the capacity for re-armament conceived along a spectrum.Footnote1 Defining irreversibility in this way shifts its focus to re-armament dynamics and presents it in relative terms where irreversibility becomes a matter of degree rather than absolute binaries. Any restrictions imposed on nuclear infrastructure or facilities will therefore influence the degree of irreversibility in a disarmament regime.
In the absence of any pre-defined and widely acceptable blueprint for irreversible disarmament, this paper explores how a spectrum of irreversibility can be applied in designing a regime for nuclear disarmament. It does this by exploring the range of actions that can be taken to constrain nuclear infrastructure that would either directly or indirectly influence the capacity for future reacquisition of nuclear weapons post-disarmament. The paper therefore asks what are the crucial nuclear facilities or processes that are likely to be key in enabling potential re-armament? What type of actions can be considered to constrain the re-armament potential of these facilities?
In addressing these questions, this paper makes some analytical choices to guide its exploration. It limits its focus to the nuclear weapons production complex. The peaceful nuclear fuel cycle has been widely studied and its relationship with the capacity to build nuclear weapons scrutinized in many studies (Fuhrmann Citation2009; Miller Citation2017; Schneider and Ramana Citation2023; Stulberg and Fuhrmann Citation2013). However, in comparison, less attention has been accorded to the nuclear weapons production complex. The reasons for this may be obvious. Firstly, the secrecy surrounding the military nuclear fuel cycle limits the quantity of public domain information on it, particularly if compared with the much more widely reported civil nuclear fuel cycle (Bodansky Citation2007). Decades of implementation of nuclear safeguards agreements by the International Atomic Energy Agency (IAEA) have also engendered a tolerance for higher levels of openness and transparency in the peaceful nuclear fuel cycle, while information on the military fuel cycle remains relatively inaccessible (Petit Citation1994; Rockwood Citation2013). Furthermore, the nuclear non-proliferation literature has focused on studying the nuclear fuel cycle and facilities linked to it in states of proliferation concern.Footnote2 Focusing on new states that might be seeking nuclear weapons also meant that less attention was accorded to the studying of the already established, long-standing nuclear complexes. In focusing on the production processes of nuclear weapons in such programmes and options for their control, this paper directs its analytical lens to an understudied topic in nuclear studies.
To simplify the scope, the paper restricts its focus to the infrastructure within a nuclear complex required for the production, maintenance, recycling, and dismantlement of simple nuclear fission weapons. While more sophisticated thermonuclear weapon designs abound and are a commonly used type in nuclear weapons programmes globally today, fission remains core to such designs and therefore constraining the infrastructure required to produce fission weapons would also affect the production of more advanced nuclear weapons such as boosted or thermonuclear devices. Addressing capabilities linked to fission in this article therefore covers a core part of the complex and allows the elaboration of the framework in a focused manner that can later be also adaptable to other parts of the complex.
In exploring these themes, the paper advances several inter-related points. First, that since the military nuclear fuel cycle and its associated facilities are directly linked with nuclear weapons production, their operability will have a significant impact on the potential for re-armament, even more so than the civil fuel cycle. Therefore, an understanding of nuclear weapons production complexes is crucial as a first step for disarmament regimes aiming for higher levels of irreversibility. Second, many of the abstract processes for weapons production can be universally standardised; for example, the production of fissile material and the shaping of this into so-called “pits” will be part of any effort to produce nuclear weapons. However, the wide variety of methods, facilities, and configurations used to achieve these processes make each national weapons production complex unique. These varieties are shaped by history, resource availability, and internal bureaucratic politics among many other factors. Understanding these national complexes, including how each approaches the various processes for weapons production, is crucial in designing tailored and effective irreversibility measures. Third, international practice has given us only limited insights into the wide range of possible actions on weapons production complexes. However, a framework of measures can be devised by extrapolating from the patchwork of international experiences, exploring the variety of possible actions and the potential scale of their application, i.e. to which facilities the measures are applied and how comprehensive they are. This will be explored in more detail later in this paper. Finally, a paradox exists when addressing weapons production complexes during a dismantlement process. While higher degrees of irreversibility may be achieved by implementing wide ranging, crippling constraints across the nuclear weapons production capacity, the processes of safe nuclear warhead storage pending disassembly, weapon dismantlement and dispositioning/storage of waste streams would rely on the continued operation of certain aspects of weapons complexes. Therefore, some residual production capacity will need to exist due the need to retain facilities for dismantlement processes, if only for long-term safe and secure nuclear materials management.
The next section explains what a nuclear weapons complex is. It is followed by a section that outlines the key generic processes needed for the development of nuclear weapons and attempts to link these processes to facilities where they are likely to be housed. Connecting processes to facilities adds more texture to understanding of the nuclear weapons production complex and enables further conversation on control and verification measures. In doing so, the paper does not attempt to chart all the various permutations, either in terms of production processes or facilities, but instead develops a standard base line that can be used for illustrative purposes for control measures. The third section develops a framework for potential actions to constrain the operations, outputs, or existence of elements of the nuclear weapons production complex. The framework is presented as having two axes. The first defines the range of facilities within a nuclear weapons production complex which might be constrained. The second defines the range of possible constraining actions that can be applied to such facilities. Together, the two axes develop a set of facility-action pairs that can be considered by disarmament planners, and which will deliver various levels of irreversibility in a disarmament process.
Understanding Nuclear Weapons Complexes
The nuclear weapons production complex is defined here as the ecosystem of interconnected facilities that are primarily used for the production, maintenance, recycling and disposal of nuclear warheads.Footnote3 Since the start of the nuclear age, these complexes have been the overarching national organisations within which nuclear weapons have been developed and maintained; they have produced thousands of weapons across the world. They have benefited from generous government funding and employed many scientists, technicians, administrators, and bureaucrats to work in their ranks. While the scales of government funding, resource endowments and human capital involved in the complex have seen ups and downs through the years, they remain substantial. For example, Schwartz estimated that the United States had spent nearly $5.5 trillion on nuclear programmes between 1940 through to 1996 and the US Congressional Budget Office projects $756 billion over the 2023–2032 period (Congressional Budget Office Citation2023; Schwartz Citation1998). Other countries are less transparent about what they spend to sustain and maintain their nuclear complexes, yet such figures are likely to also be high.
The term “nuclear complex” is sometimes used in an expansive way. According to Bischak, it includes “weapons research laboratories, nuclear weapons material production facilities, the weapons fabrication facilities, the nuclear weapons test sites, and the treatment, storage and disposal of radioactive waste” (Bischak Citation1988). A reduced scope of the term is used analytically here to refer to all the facilities directly involved in the manufacturing and maintenance of nuclear weapons in a country, excluding nuclear testing and research and development (R&D) facilities which do not also contribute directly to weapons production. This reduced scope was chosen in part for pragmatic reasons to make this examination possible in an article-length format and in part because manufacturing and maintenance sites are at the core of weapons production or re-armament while R&D and testing largely occur at the front and back ends of this process.Footnote4 An examination of the links between R&D and production potential is further explored later in the article under the sub-section entitled “Target nuclear weapons R&D”.
The production complex can be managed within a single large bureaucratic or administrative unit within a state or divided across many government departments or units. It can also carry different names in the official discourse and can reflect different propensities as to what facilities and activities are included. In the United States, for example, it is formally called the “Nuclear Security Enterprise” in the jargon of the US Department of Energy. Example of the tasks carried out by the Nuclear Security Enterprise are mandated by the US Atomic Energy Act: “ensure … the [nuclear] stockpile is safe, secure, and reliable to perform as the Nation’s nuclear deterrent”.Footnote5 In the United Kingdom, the complex is formally called the “Defence Nuclear Enterprise” and is overseen and sponsored by the Defence Nuclear Organisation. It “includes all the organisations, programmes and people within government that sustain the UK’s strategic nuclear deterrent”.Footnote6 As another example, at a key juncture in the early 1980s, the Soviet nuclear weapons production complex included facilities concentrated in 10 closed cities serving as distinct administrative and production units (Podvig and Bukharin Citation2004).
The term complex however has not been used in either the NPT or the Treaty of the Prohibition of Nuclear Weapons (TPNW). The latter in instituting a blanket prohibition on nuclear weapons, used the term “nuclear weapon programme” to refer to the infrastructure that brought about these weapons. States which join the treaty are not allowed to possess nuclear weapons or hold on to nuclear weapon programmes. This however is not defined within the text of the treaty leaving room to interpret what forms part of a nuclear weapon programme and what can fall outside. In 2007, Costa Rica and Malaysia presented a model Nuclear Weapons Convention that used the term “facility” to refer to that infrastructure rather than complex or programme.Footnote7
Nuclear weapon complexes have been challenging to study because of various layers of secrecy surrounding them. As Wellerstein demonstrates, secrecy, rather than being incidental to the nuclear age, is constitutive to many of its practices from the start of the nuclear age till this day (Wellerstein Citation2021). This secrecy is sometimes enshrined in legal obligations, national security needs or as a function of the taboo surrounding nuclear weapons due to their extraordinary destructive power. Furthermore, this secrecy has evolved, with its logic, rationale and extent changing over time. In the early years of the nuclear era, secrecy was guided by the need to preserve a nuclear advantage, and was meant to prevent other state competitors from gaining useful knowledge about how to develop the scientific, technical, and industrial capacity to build nuclear weapons (Westwick Citation2000). Later, other layers were added, including the need to be in compliance with global instruments such as the Nuclear Non-Proliferation Treaty (NPT), which prohibits providing assistance in developing nuclear weapons, as well as the fear of nuclear terrorism, on top of traditional national security considerations.Footnote8 The combined effect of these and other factors contributed to a limited availability of information on nuclear weapon complexes in the public domain, making them more challenging to study.
Notwithstanding successful acts of nuclear espionage and cases of deliberate state-to-state cooperation,Footnote9 secrecy also led to the military nuclear complex and facilities of each country being developed separately and independently without the harmonizing influence derived from the relatively open exchange of technology in a permissive international market environment as enjoyed by today’s commercial civil nuclear energy, where civil nuclear technologies are sold internationally by a range of supplier states (Schneider and Froggatt Citation2023). This, as well as the specific demands and opportunities of various national contexts, resulted in a set of closed, nationally-styled nuclear weapons complexes that are challenging to study compared to the peaceful nuclear fuel cycle. It is also worth noting that while little information is available in the public domain on nuclear complexes, that scarcity is not equal everywhere. Some states, for example, the United States, are much more open about their nuclear weapons complex than others. These differences in approaches to secrecy between states are in part due to different political systems that might encourage or discourage the sharing of information and internal accountability, but it can also have some cultural components (Schaper and Müller Citation2009). More information about the US nuclear weapons production complex is available in the public domain than other states’ complexes, meaning that studies tend to draw on the US complex more heavily. This study will be no exception, with the explicit proviso that nuclear complexes in other countries might work differently, and that a disarmament approach designed for the United States will be unlikely to be as effective for other national complexes.
The nuclear weapons production complex, as defined in this paper, does not function in a vacuum. It is linked to other facilities and entities that contribute to the development of nuclear weapons, such as the military sites where handling and deployment takes place, testing facilities, and research and development infrastructure. The latter represent hubs of knowledge and skills related to the development of nuclear weapons, and their duties may include assessing the safety and effectiveness of deployed and stored nuclear weapons over time. That said, some laboratories within research facilities may allow for small-scale production of nuclear weapons materials, and therefore should not be discounted when considering disarmament.
The nuclear weapons complex might also draw, either directly or indirectly, on civil nuclear activities, most prominently nuclear power generation and the civil nuclear fuel cycle, and this relationship is worthy of deeper consideration. The dual-use nature of nuclear technology has been covered extensively in the nuclear literature and despite differences between the aims of military and peaceful applications there is significant overlap in the technologies and their utilisation (Forge Citation2010. A state may possess both a nuclear weapons complex of facilities and processes aimed at developing nuclear weapons, and a nuclear energy programme supported by a civil nuclear fuel cycle. The nuclear weapons complex and the peaceful fuel cycle may be entirely separated, with the key activities and facilities included in one not being shared with the other and each run or supervised administratively by a different government department. This is the case in the United Kingdom, for instance, where the Defence Nuclear Organisation falls under the control of the Ministry of Defence, uses a dedicated inventory of military nuclear material, and all activities occur within dedicated facilities (Comptroller and Auditor General Citation2018, 16; Mills Citation2023a, 13). Along the same lines, in 2001, Pakistan transitioned its programme from the control of the Pakistan Atomic Energy Commission to a separate, new organisation, the National Defense Complex (Guinnessy Citation2001). Other states manage their nuclear weapons production and maintenance through dedicated facilities with dedicated materials, but with a single government department responsible for both peaceful nuclear energy and nuclear weapons. For instance, the US Department of Energy and the French Alternative Energies and Atomic Energy Commission (CEA) have both civilian and military nuclear roles, although the peaceful and military activities are functionally separated within these high-level bodies (Kristensen, Korda, and Johns Citation2023; World Nuclear Association Citation2023). A third set of states may possess programmes with a degree of overlap, with some key facilities, materials and processes playing a function in both civil and military nuclear activities. Discussions of India-US nuclear deal in 2007 led to requests from the United States that India should separate its peaceful from military nuclear facilities and materials (Weiss Citation2007).
The separation or overlap between military and civilian fuel cycles is not uniform in all states and different configurations have existed in different historical periods. For example, a county might start with a focus on weapons production and never develop the capacity for nuclear power generation. Alternatively, a country can start with weapons production and then develop an interest in peaceful nuclear applications later, or vice versa. Identifying where military and civilian capabilities interact or separate is crucial in identifying the boundaries between nuclear weapons production complexes and other related but separate facilities. Are there facilities that jointly benefit a military and a civilian fuel cycle or are these facilities separated? A key consideration here is the production of fissile material and whether uranium enrichment facilities, or plutonium producing reactors and reprocessing facilities, handle material and activities strictly for military or military as well as civilian purposes. Where separation exists, the nuclear weapons production complex can be addressed with control measures without impacting on the peaceful uses of nuclear technology. Where integration rather than separation exists, any irreversibility-enhancing actions will likely impact both military and peaceful functions, and states will need to consider the level of impact they are willing to tolerate on their peaceful nuclear activities to enhance irreversibility in nuclear disarmament.
The separation or integration of the fuel cycle is driven by two competing factors. Economic factors will drive towards integration. For instance, enrichment and reprocessing plants are hugely complex and expensive facilities, both to construct and operate. As such, economic considerations would advocate using existing facilities where possible, rather than setting up independent, parallel military fuel cycle facilities to perform the same function. Secrecy and security considerations as well as international norms would pull in the other direction and towards separation. To perform military activities within a civilian facility creates a risk of sensitive knowledge transfer to civilian nuclear workers, while the facility’s existing security arrangements may not be suitable for nuclear weapons purposes, and the human reliability assessments and other changes necessary to allow this could lead to an undesirable/unacceptable interruption to civil nuclear operations. When considering the level of separation, each state will assess the relative importance of these and other factors according to its own unique situation and objectives.
It is worth noting that activities in the nuclear weapons production complex, including fissile material production, are out of the reach of any international transparency and verification activities. For example, they are not covered by traditional IAEA verification measures, known as safeguards. IAEA Comprehensive Safeguards Agreements are not applied to nuclear weapons holders. Some states choose to apply “voluntary offer agreements” that allow inspections on some facilities in nuclear weapon states, but these are limited in scope and do not apply to facilities linked to the nuclear weapons complex, only to those performing peaceful nuclear activities.Footnote10
Nuclear Complexes: Capabilities and Facilities
This section outlines some of the key capabilities that are common in any nuclear weapons production complex. These are expressed in terms of generic activities that aim to capture the crucial processes for nuclear weapons production. There are some caveats that need to be taken into consideration here. Building nuclear weapons involves a very wide range of activities that differ in both scope and significance. This paper does not seek to capture the entirety of the process but to focus on the key steps deemed to have a crucial role in weapons production and which consequently require investment to build and maintain the necessary enabling infrastructure. The section also considers the facilities that typically enable such capabilities. Doing so can help identify key facilities in the generic nuclear weapons production complex and contribute to understanding the range of measures available to constrain them to influence the degree of irreversibility in disarmament or arms control measures.
This paper posits that five critical capabilities are required for the purpose of producing fission weapons, supported by three sustaining capabilities required for the maintenance of credible nuclear weapons programmes in the long-term. More advanced designs, such as boosted and fusion weapons, will require additional critical capabilities beyond these, for instance to produce tritium and/or lithium deuteride.Footnote11 The five critical and three sustaining capabilities are presented in . A diagram is presented in showing the eight capabilities and how they interrelate.
Figure 1. Flowsheet illustrating the relationship between critical capabilities C1-C5 and sustaining capabilities S1-S3 within the nuclear weapons production complex. Processes whose boxes have dashed lined are not considered within this paper to be part of the nuclear weapons production complex but are included to show the complete weapons lifecycle. Note – pit storage (sustaining capability S2) is included as an optional process for illustrative purposes. Storage and staging facilities are key elements of nuclear weapons production complexes and can be inserted at any point, but only this one example is included here to simplify the diagram.
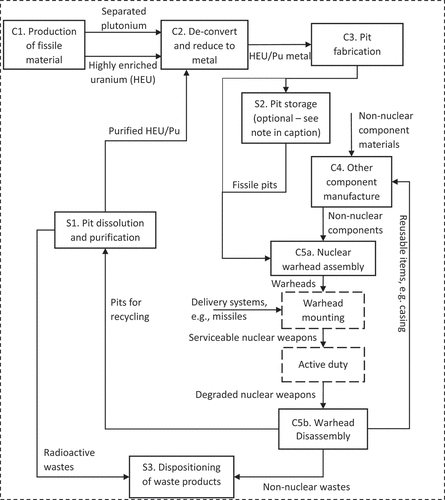
Table 1. Nuclear weapons production complex critical capabilities C1 to C5 and sustaining capabilities S1-S3.
The pit storage capability shown in box S2 in is included to illustrate that storage capabilities will play a key role. However, the capability to store and maintain stocks is important throughout the production complex, and this sustaining capability may occur between and within any process listed, not simply between pit fabrication and warhead assembly. States possessing inventories of nuclear materials and items within storage facilities might be able to use these as feedstocks to other capability processes downstream to continue producing weapons in the absence of upstream capabilities, meaning that it is crucial to identify not only the facilities but also any such inventories possessed by states. This will be particularly important in the consideration of ways to enhance irreversibility in nuclear disarmament plans. States may also circumvent the need for a given capability by collaborating with willing foreign partners who possess it and are willing to share or provide access to the capability.
A nuclear-armed state must have access to all critical capabilities to produce a nuclear weapon, as well as all sustaining capabilities to maintain a functional and independent nuclear weapons programme. These capabilities are enabled through access to a range of specialist explicit and tacit knowledge embodied in workers and managers, suitable equipment, infrastructure and facilities, and sufficient sustained funding (MacKenzie and Spinardi Citation1995; Ritchie Citation2023). Absent any of these elements, the capability becomes unavailable, meaning that a critical step cannot be completed, resulting in disruption to the nuclear weapons production complex. Thus, states wishing to build irreversibility into their disarmament processes should consider how they can limit, constrain, or get rid of these capabilities. This will need to be informed by a detailed assessment of the full scope of relevant capabilities possessed by the state, how they might be used in a disarmament reversal scenario, and how they may be approached from an irreversibility enhancement perspective. It is not necessarily the case that states must focus on completely eliminating specific individual capabilities to achieve irreversibility; indeed, states can progressively enhance the irreversibility of their disarmament by taking a set of more or less readily reversible actions across the set of capabilities.
Critical Capability C1 – Production of Fissile Material
The production of fissile material in the right configuration has historically been the most costly and challenging part of producing components in nuclear weapons. Almost 80% of the costs of the historical Manhattan project were dedicating to mastering these processes (Zimmerman Citation1993). The production of new fissile material for weapons programmes is not currently undertaken and so current cost figures are not known, although the authors have no reason to believe that fissile material production processes have become less costly or challenging relative to the other processes required for nuclear weapons production. So prominent are fissile materials that Feiveson et al use them as a key gateway to analysing nuclear weapons dynamics and call our epoch the ’fissile material age’ (Feiveson et al. Citation2014, 1). This reflects their relative value and importance within the overall effort to produce nuclear weapons and also the potential enhancement to irreversibility in nuclear disarmament that can be achieved through action to control the capability to produce them. These materials are usually in the form of highly enriched uranium or separated plutonium.
The International Panel on Fissile Materials estimates the global stockpile of unirradiated highly enriched uranium that is in weapons or available for use in weapon programs to be 1,100 metric tons. For separated plutonium, this figure is 140 metric tons.Footnote12 Most nuclear-armed states do not currently produce new fissile material for weapons purposes, with the exception of India and Pakistan and possibly Israel and North Korea.Footnote13 For states no longer producing new nuclear materials, these can be drawn from existing military inventories where available or recycled around the loop made by capabilities C2-C5 and S1-S2 in . However, the production in these states may be resumed if national governments decide to grow their weapons stockpiles beyond their current levels, or if their existing inventories of military nuclear material become degraded beyond use and a decision is taken to replace them.
The production of highly enriched uranium takes place in facilities utilizing different enrichment techniques to increase the concentration of the uranium-235 isotope from the 0.7% level found in natural uranium to greater than 90%. Key enrichment techniques include gaseous diffusion methods and gas centrifugation methods, which involve the conversion of the uranium to a hexafluoride gas (UF6, known as “hex”) and then either forcing the gas through a semi-permeable membrane or spinning the gas at extremely high speeds within a centrifuge, respectively. Both processes only achieve a minute degree of separation, and often many hundreds or thousands of units are required to achieve an acceptable product and rate of production. These units must be supported by pumps, piping, and a range of other equipment (Wilks Citation2012). Enrichment facilities can be set up in a range of configurations depending on the type of equipment used and the scale of their operation, but they must be suitable for the operation of this sensitive, complex, and expensive process. While enrichment through gaseous diffusion and gas centrifuges have been the two most commonly used methods in military enrichment plants, other technologies exist for enrichment; electromagnetic separation approaches have previously been used in the Manhattan Project at industrial scale (although these were found to be less effective than gaseous diffusion approaches) (US Department of Energy Office of History and Heritage Resources Citationn.d.), and in the Iraqi programme albeit not on an industrial scale,Footnote14 as have approaches based on so-called stationary centrifugation in the South African programme (Kelley Citation2020).
The production of suitable plutonium would require irradiation of suitable nuclear fuel in a reactor followed by chemical reprocessing to separate the plutonium from other components of the irradiated fuel. The facilities for plutonium production are highly specialised, in part due to the major radiation hazards posed by irradiated nuclear fuel (Netter Citation2012). Both nuclear power plants and nuclear chemical reprocessing plants are very specific facilities with numerous unique features that make them unlike any other type of facility. Nuclear fuel reprocessing cannot be performed outside of a specially designed facility with suitable radiation shielding without very quickly exposing workers to lethal levels of radiation.Footnote15 Operations in civil reprocessing plants are performed within hot cells – enclosures of thick concrete and lead-doped glass, through which workers conduct operations using remote manipulators. Military reprocessing facilities very likely operate under the same principles.
The facilities described here for fissile material production can legitimately exist for peaceful uses, although if they are configured for weapons production this can have telltale signs such as the rearrangement of enrichment equipment units towards the production of a highly enriched product, the presence of enriched uranium above the level expected for civil purposes, the diversion of material from further civil activities, or the removal of or tampering with verification equipment. In states with strict separation of fissile material production between military and civilian purposes, applying restrictions on facilities earmarked for military purposes would block the most direct and straightforward route to the production of military fissile materials. However, the risk that facilities earmarked for civilian uses could be adapted to weapons production remains, which would indicate a need for controls similar to those currently applied to prevent proliferation.
Critical Capability C2 – Deconversion and Reduction to Metal
Nuclear materials incoming from enrichment and reprocessing facilities may come in a range of chemical forms depending on upstream processing. These include solids of uranium as various oxides or fluorides, or plutonium as a nitrate, peroxide, or oxide, in solid form or in solution. Nuclear weapon pits, however, are in the form of metals or alloys (Murray and Holbert Citation2015, 495–502). Consequently, the weapons-grade fissile material must be converted to the metal/alloy form to produce functional pits. The radiation hazard for these materials is much lower than for irradiated nuclear fuel, though still present, and facilities must control for this. Thus, chemical engineering facilities suitable for the handling of hazardous radioactive materials will be required for this process. The metal must initially be produced through chemical purification processes under conditions suitable to prevent unacceptable radiation exposure of personnel (Ackland Citation2002, 130–135). Again, the type of facility will also be highly dependent on the scale of operations to be performed. This facility may also be used for the conversion of purified material from recycled pits back to metal for fabrication into new pits.
Critical Capability C3 – Fabrication of Fissile Material into Suitable Pits
To develop nuclear weapons, metallic fissile materials produced in the above step must be shaped to optimise their explosive potential. The shaped metal products are known as “pits”. A pit generally takes the form of a hollow sphere of uranium and/or plutonium metal, commonly produced by casting the molten metal as two hemispherical shells, which are then welded together (Medalia Citation2015, 25–26). These are challenging processes. Highly skilled machine tool operators are required to shape the shells and sphere to its final form in suitable workshop facilities. Quality control on fissile materials and fabricated pits is critical, as the slightest deviation from accepted parameters can lead to significant changes in weapon performance (Coggeshall, Grant, and Spivey Citation2021). The processes of casting, machining, welding, and quality control may take place within a single facility or be divided between multiple facilities. In the United States, for example, during the Cold War, the industrial capacity to produce plutonium pits resided in a pit manufacturing plant (the Rocky Flats Plant), which was separate from fissile material production (Woolf and Werner Citation2021, 10). The production of pits in the Rocky Flats Plant has now been discontinued. Some capacity also exists within research facilities at Los Alamos National Laboratory at the PF-4 facility, and the United States is seeking to expand this capacity (Medalia Citation2015, 1). Commonly in the United States, multiple facilities are collocated into a small number of secure sites.
Uranium is more forgiving than plutonium in terms of shaping, and there is no requirement to machine a perfect hollow sphere, although this does produce the greatest yield from a given mass of fissile material. It is possible to achieve a nuclear explosion with highly enriched uranium using a gun-type device, as originally demonstrated in the nuclear warhead used against Hiroshima during the Second World War (Murray and Holbert Citation2015, 496). Uranium is also metallurgically more straightforward with only three relatively separated allotropes compared to plutonium’s often close or co-existing seven, allowing it to be cast and machined more easily (Baker, Hecker, and Harbur Citation1983). This means that uranium fabrication facilities can be comparatively simpler, though appropriate safety precautions will still be required to protect workers from the radiation hazard.
Overall, facilities for nuclear pit production will have the hallmarks of both metallurgical casting and precision machining workshops, and radioactive materials laboratories. They will need to be fitted out such that these hazardous materials can be processed under suitable protection, principally sealed glove boxes or hot cell environments designed to work under conditions of extreme temperature.
Critical Capability C4 – Manufacturing of Non-Nuclear Elements of Nuclear Warheads
Warheads can range in complexity depending on their design and intended purpose. At minimum, they must include a physics package, composed of a pit and neutron source surrounded by suitably shaped high-explosives, a detonation system, and an outer shell.Footnote16 There may also be systems for safing, boosting, and guidance and/or manoeuvring, in the form of a range of electrical and mechanical systems, as well as structural components (Plummer and Greenwood Citation1998, 1, 5–6). These must all be held within a suitable casing. These numerous components may be all manufactured in separate facilities before being brought together for assembly, and given their nature, many of the types of components have non-nuclear uses in, e.g. conventional arms. Taking the US nuclear complex as an example, the Kansas City National Security Campus is responsible for the manufacturing of nonnuclear mechanical, electronic, and engineered material components for nuclear weapons (Woolf and Werner Citation2021, 18).
The nature of these non-nuclear components is highly varied, ranging from highly sensitive but suitably robust sensors used in arming and firing the weapon, to power systems to initiate the detonators, to neutron reflecting shell materials such as beryllium (Deputy Assistant Secretary of Defense for Nuclear Matters Citation2020). As a result, production facilities for non-nuclear are also highly varied, though will take the form of secure laboratories and/or workshops. The individual components may be brought together into one or more subassemblies prior to the installation of nuclear material. These materials and components are likely to be secret and may also be toxic, e.g. beryllium.
Critical Capability C5 – Warhead Assembly and Disassembly
This is the most step in the production of nuclear warheads, in that states which cannot do this cannot produce nuclear weapons themselves. It involves the closure of the physics package around the pit and the emplacement of this into the warhead shell with the other non-nuclear components. Historical designs also allowed for pit insertion to an assembled warhead (Weaver Citation2015, 2). These operations take place in highly secure facility. Such facilities would require suitable equipment to bring together the various components and sub-assemblies, as well as to handle radioactive pits, should these be added at this point.
These facilities may also be used for disassembly and refurbishment operations, given that disassembly will proceed as the reverse operation of the warhead assembly process. Maintenance/refurbishment will likely be required to maintain operational nuclear weapons over a period of years, due to the need to replace the pit if radioactive decay product ingrowth has made it unserviceable, check for any corrosion, and replace degraded non-nuclear components (Medalia Citation2015, 1). The old pit would be stored or dissolved and recycled through capability S1 below. This process involves weapons being taken from active duty, dismantled, and components being reused, repaired, or replaced. Facilities capable of supporting this process will be required during disarmament processes, at least until all weapons are dismantled to their individual components which can then be managed individually.
Some non-nuclear components of nuclear weapons may be suitable for reuse, possibly following a period of maintenance. This will vary according to the component, with longer-lived items in good condition potentially continuing their use, while other components may require maintenance or replacement. Maintenance may be conducted at the location of the dismantlement in specialised workshop facilities, or else components may be transferred to their original manufacturer for this purpose, using the same or similar facilities to those described in critical capability C4 above. Items which cannot be repaired, or are declared obsolete, must be replaced. How each component is managed will vary according to the policies of the individual nuclear weapons programme.
The value of these facilities in supporting rearmament thus increases as disarmament proceeds, given their status as facilities where new weapons can be assembled and where maintenance and refurbishment can be done. But as pointed out earlier, such facilities will be needed throughout any disarmament process to undertake disassembly of warheads. In planning for irreversibility in disarmament, thought should be given to how such facilities can be approached such that disassembly can be performed, but assembly becomes more difficult. In the United States, all assembly and disassembly operations since 1975 took place at one site, the Pantex Plant (Woolf and Werner Citation2021, 21). The United States does not currently produce new warheads but has plans underway to change that (US Department of Defense Citation2022, 20–22). France is also currently engaged in implementing new warheads for its submarine-launched missiles (Kristensen, Korda, and Johns Citation2023). The United Kingdom has plans to deploy a new warhead from the late 2030s (Mills Citation2023b, 4–5). All three states will require warhead assembly and disassembly capabilities to support these transitions.
Sustaining Capability S1 – Pit Dissolution and Purification
Over time, pits undergo radioactive decay, corrosion, and self-irradiation, leading to a build-up of undesirable decay products, such as americium-241 in plutonium pits, and other chemical and structural changes (Martz and Schwartz Citation2003). To remove these, pits must be periodically purified through chemical processing and then new pits are formed from the purified product. In the United States and United Kingdom, plutonium pits have been purified through several steps, including molten salt extraction and electrorefining methods (Lashley et al. Citation1999). The United States has historically done this at the PF-4 plant, though this process is not currently being performed (Medalia Citation2015, 18–21). Facilities for these processes will be akin to those used in spent fuel reprocessing, with the key difference being that the levels of radioactivity are much lower, which translates into a reduced, though not eliminated, requirement for radiation shielding. Nevertheless, the processes used involve high temperature treatment of radioactive substances in liquid and powder forms, using a range of highly toxic chemicals, and the facilities that perform this role are highly specialised. This process cannot be readily performed absent such facilities. As a radioactive material, highly enriched uranium also undergoes radioactive decay, but at a much slower rate, meaning that uranium pits are much longer lasting than plutonium pits without a need for repurification (Holden Citation1981). The product arrow in shows purified fissile material being returned to the deconversion and reduction to metal process, but this process may be carried out as part of the pit purification, meaning that the product may pass directly into pit fabrication.
Sustaining Capability S2 – Storage of Nuclear Materials and Other Weapon Components
Storage, or staging, facilities will likely form part of any nuclear weapons production complex, either to store products or raw materials temporarily pending further processing, or to keep a surplus inventory of materials or components available should the need for them arise. The nature of these facilities will vary according to the nature of the stored materials or items, though high levels of security will be required. Fissile materials, including pits, will need to be stored in a suitable configuration to prevent criticality events and to protect people and the environment from radiation. Non-nuclear components will not require these protections, though will still require a suitable facility to keep them in good condition. Storage facilities suitable for nuclear materials will be required during disarmament processes to store pits removed from weapons until such time as they can be transferred to appropriate dispositioning routes, e.g. conversion to a waste form and direct disposal, or conversion to a form suitable for use in the civil nuclear fuel cycle.
Sustaining Capability S3 – Radioactive Waste Management
The production of nuclear weapons generates a range of radioactive wastes that must be safely managed. While shows a simplified flowsheet indicating that waste streams come from only capabilities C5 and S1 (warhead disassembly, and pit dissolution and purification), in truth radioactive wastes will arise from any facility involved in the processing of radioactive materials. Radioactive waste management facilities will be of two broad types: waste packaging facilities, and waste storage and disposal facilities. Waste packaging facilities will include the necessary equipment for the conversion of wastes in the form received from other facilities to “waste forms” suitable for long-term storage or disposal. Storage and disposal facilities are likely to be highly hardened structures, potentially built underground, with a suitable layout inside to allow the emplacement of waste forms (International Atomic Energy Agency Citation2020, 3-11).
Unlike most other capabilities in the nuclear weapons production complex, it will likely not be possible nor desirable to take major actions to constrain the operation of radioactive waste management facilities. Former weapons materials in a disarming or disarmed state will require long-term management to ensure that they remain safe, secure and that the public and the environment remain protected from the hazards within. However, some action may be taken to prevent retrieval of the materials, following suitable chemical and other processing to reduce their appeal in a disarmament reversal scenario.
Developing a Framework for Irreversibility Enhancement
This section develops a framework that lays out the range of possible actions on nuclear weapons production complex facilities that might be considered to enhance irreversibility in disarmament and the potential impacts of these actions. The purpose of this framework is to provide a tool that can be used to structure an analysis of the potential for irreversibility resulting from actions applied to nuclear weapons production complexes. Each action, applied to each facility within a complex, would have a different impact on the potential for disarmament irreversibility, as well as its own affiliated challenges.
As already indicated, any attempt to irreversibly disarm a state through action aimed at its nuclear weapons production complex must be informed by a complete understanding of the state’s capabilities, including currently in-use facilities, facilities under construction or planned, as well as facilities which could be drawn into the service of military ends at need, such as civilian fuel cycle facilities and conventional arms manufacturing facilities. Building from the generic flowsheet of , which does not indicate specific facilities, the information above will allow the generation of a complete set of possible flowsheets for the production of nuclear weapons within the state. This should include all realistic alternative routes to achieve each capability, for example, drawing material from storage rather than producing it, changing to another processing method or eliminating the need for a given capability, or switching to uranium pits rather than plutonium or vice versa.
As suggested above, the status of nuclear materials inventories must also be considered when developing the flowsheets, as material stockpiles can function as the feedstock to mid-stream processes, meaning that action against upstream facilities would give little value in terms of irreversibility. Furthermore, as stated above, fissile materials within nuclear weapons can be recycled for many years, and even without access to fissile material production, established weapons programmes can be sustained for decades given access to a suitable inventory of fissile material.
There are thus many potential actions that can be taken on different facilities to constrain a nuclear weapon production complex. Each of these facility-action pairs would have a unique impact on the potential for irreversibility in a given state’s disarmament programme. This article cannot encompass the full range of potential impacts from every pairing of facility and action, however, the framework developed here seeks to systematically lay out the potential actions that can be applied and facilities that can be acted upon to enable consideration of their feasibility, utility, and potential for irreversibility enhancement. While the framework aims to cover the full spectrum of potential facilities and actions, it does not claim to be exhaustive. The framework is developed along two separate axes, which are represented visually in . First, it considers which facilities could be selected for potential action to constrain or control their capacity. Second, it lays out the range of potential actions that could be taken regarding these facilities to achieve this. The nature of the facilities chosen and actions taken will determine how difficult, time-consuming and/or costly it will be to regain the capacity, and thus the degree to which the disarmament is made irreversible.
Table 2. Visual representation of the irreversibility framework as a table. The axes give either rows or column headers, which are defined within this paper. Each cell within the table defines a given facility-action pairing and its potential for irreversibility enhancement and its feasibility. The detail of the cells as shown here is not included in this paper, beyond some examples used to illustrate the axes of the framework.
A representation of the potential irreversibility enhancement that can broadly by achieved at each step along both axes is provided below, as well as a consideration of utility and some potential challenges. Further differentiation of the potential irreversibility enhancement impact and specific challenges at the level of each pairing of action and facility is considered to be a useful next step for this research.
First Axis: Selection of Facilities for Action
The first axis considers which facilities may be acted upon to enhance irreversibility in nuclear disarmament. These are presented here in broad categories; however, a detailed assessment of individual facilities will be required in a subsequent step to determine the individual facility level measures that might be applied. The individual critical and sustaining capabilities above do not necessarily correspond to single facilities; a given facility may be instrumental in the delivery of several capabilities, while a given capability may require several facilities. Additionally, there may be multiple redundant facilities capable of achieving similar outcomes, for instance older facilities which have been partially or even fully replaced but have not yet been decommissioned.
No Target: Retention of Full Production and Re-Constitution Capacity
This can be considered a disarmament minimum, under which the warheads are dismantled but the production capabilities remain intact without intervention. Under this baseline position, a decision is taken that no facilities will be selected for constraining action. This does not prevent disarmament according to a basic understanding of it that targets primarily the weapons and their delivery systems, potentially alongside dedicated effort to downplay the salience of nuclear weapons. However, all facilities in the weapons production complex under this type of action will remain unchanged. While this target selection option represents no enhancement of irreversibility in nuclear disarmament through action on weapons production capabilities, it has been advocated under the banner of “virtual nuclear arsenals” where weapons are eliminated but production capabilities are retained unchanged to allow re-constitution under agreed terms and common expectations, such as if one party were to cheat (Mazarr Citation1995; Schell Citation2000).
Target Redundant Production Pathways
This target selection option seeks to reduce redundancy for critical and sustaining capabilities without necessarily constraining them or imposing restrictions on capacity. For various reasons, states may host multiple facilities capable of fulfilling the needs of a given capability. For instance, a state may have originally operated a gaseous diffusion enrichment plant for its production of military uranium, before also constructing a more modern gas centrifuge enrichment plant. Both plants may operate in parallel, providing redundancy. Similarly, a state may have dispersed its nuclear weapons assembly and disassembly facilities across a number of sites to harden against foreign military strikes. These redundant facilities develop additional parallel pathways for certain production processes. They are common and are to be expected in many military as well as civilian programmes. For example, to produce fissile material, the Manhattan project, the first programme to produce nuclear weapons, invested in both the plutonium and uranium routes simultaneously. Examples abound of programmes that for programmatic or security reasons decided to follow multiple routes or build multiple facilities in aid of production, such as investing in multiple uranium enrichment routes. The scope of action here covers these redundant pathways. Depending on the type of action applied, this could constrain the various redundant pathways, leaving only one, which could be selected to deliver benefits for verification and/or limitations on capacity, for example. This could be a potentially acceptable first step for states wishing to make a visible and verifiable commitment to irreversibility. While it does not hamper reversal of nuclear disarmament, it does place the nuclear weapons production complex in a more vulnerable position while also demonstrating a commitment to action which goes towards enhancing irreversibility in disarmament.
Target Nuclear Weapons Production Complex Facilities
A major impact to enhance irreversibility would be delivered through action to limit or shut down one or more of the critical capabilities that form part of the nuclear weapons production complex discussed in the previous section. The greatest enhancement would be achieved through the complete destruction of all capabilities. However, a disarming state may not wish to move directly to this maximum irreversibility position, for instance due to budgetary pressures, or because they are engaged in a phased disarmament process alongside a rival nuclear-armed state. In such cases, a detailed assessment of the state nuclear weapons production complex becomes critical to determine how the capabilities are supported, what alternative support they could draw upon, and how each contributes to the nuclear weapons complex. From this, a series of facilities can be assessed as potential targets, selected based on the value to the complex, and the feasibility and acceptability of actions from the second axis, described below.
Target Nuclear Weapons R&D
While these are beyond the scope of the nuclear weapons production complex as defined here, capabilities and facilities for nuclear weapons research, development and testing also contribute to the sustainment and potential reconstitution of nuclear weapons. Longer periods of disarmament prior to an eventual reversal will lead to an increased need to reacquire lost knowledge and skills, and so the longer a state is engaged in disarmament or is disarmed, the greater the degree of R&D activities that would be required for nuclear weapons to re-emerge, as suggested in literature characterising the nuclear weapons production complex as a sociotechnical system (Sims and Henke Citation2012). In this scenario, these R&D activities are likely to blend into production activities to some extent, even if the state later constructs pure production facilities. Where production capabilities to do exist within R&D facilities it is prudent to consider these as production facilities for the purposes of enhancing irreversibility in disarmament and the application of the framework presented later in this paper. Disarmament planners are encouraged to independently assess R&D facilities regarding both their current role and capabilities, and their potential for conversion to a production role in a rearmament scenario. This may involve disaggregating some facilities to split desirable/necessary activities, such as radioactive waste management, from production capabilities occurring within the same facility.
Action to constrain research and development facilities will further strengthen irreversibility in nuclear disarmament by hampering the ability to guarantee that existing nuclear weapons will continue to be safe and effective, while all but eliminating the capability to design and test new weapons and components for them.Footnote17 This risk to the ability to guarantee existing nuclear weapons remain safe pending dismantlement means that some capability will be needed for this purpose at least until all weapons are dismantled.
Large research and development organisations may host facilities which can also produce weapons and weapon components. For example, Sandia National Laboratory in the United States “manufactures some specialized components, like neutron generators, and maintains a backup capability to produce batteries and high-explosive components” (Woolf and Werner Citation2021, 15). Research and development facilities are not considered further here, as these contribute to the capabilities primarily through the development of knowledge and skills rather than as production facilities, and these are not the focus of this article. However, as was suggested when discussing the need for skilled machinists in pit fabrication, skills and knowledge are critical elements of nuclear weapons complexes, and must not be ignored (Buffett Citation2013).
Target Civil Nuclear Infrastructure
When seeking irreversibility in nuclear disarmament, the connection between the peaceful and military uses of nuclear technology would ideally be weakened or broken, such that states can continue to enjoy the benefits of nuclear energy, medicine, and so on. However, civil nuclear facilities can be turned towards military purposes, particularly to produce fissile material. As this link cannot be fully broken, there is a risk that these civil nuclear capabilities might be misused to facilitate disarmament reversal. Irreversibility can thus be enhanced through action on civil nuclear fuel cycle facilities of concern, particularly enrichment and reprocessing facilities (Carless, Redus, and Dryden Citation2021). This paper focuses primarily on the nuclear weapons production complex, and further studies would be needed to explore what measures could apply to civil nuclear infrastructure to influence the potential for re-armament.
Second Axis: Types of Action on Facilities
This section develops the second axis of the framework. Whereas the first axis of the framework considered which facilities or combinations thereof can be considered for action, the second axis explores the range of possible actions that can be taken to influence irreversibility of nuclear disarmament. These possible actions can be divided into two groups. The first group addresses the pace and schedule of operations within a facility in a way that would impact on its outputs. The second group entails introducing measures that can physically or mechanically alter how a facility is constituted rather than just the rate at which it functions. Disarmament planners should take care when considering facilities necessary to the process of disarmament. Such facilities should not be subjected to disablement, conversion or dismantling until their duties in support of disarmament are completed, although caps, freezes and modifications to the facility for the purposes of verification and transparency may be implemented as part of the process.
Influencing Operations – Cap
The lowest level of action is to reduce operation at a facility without fully stopping it. The application of a cap on production or a limitation on the scale of operations in facilities would have a relatively minor ability to enhance irreversibility of disarmament because any functional cap on operation, unaccompanied by structural changes, can be easily and speedily reversed, thus possibly allowing re-armament. The Joint Comprehensive Plan of Action placed a range of limitations on Iranian nuclear facilities, including limiting uranium enrichment operations to a single facility at Natanz, with a further limitation on the permitted number of operational gas centrifuges at both the Natanz and Fordow facilities (UN Security Council Citation2015, 11). These measures were able to maintain relatively high nuclear latency within the state (Fitzpatrick Citation2020). However, since the breakdown of the agreement, Iran has taken a range of steps to breach these limitations, and was able to increase its production of enriched uranium rapidly to concerning levels. This increase could likely have been conducted even more quickly but was restrained as an attempt to coerce a US return to the agreement (Monshipouri Citation2022, 211–212).
Influencing Operations – Freeze
As the next step to enhance irreversibility, operations might be frozen – stopped completely – and facilities might be mothballed – shut down but maintained in a state where they could be later reactivated. In contrast to capping, this would mean the facility would be no longer operating, rendering the capability it delivered unavailable, assuming that there were no alternative facilities which could be used instead. However, if the state took measures to maintain the shutdown facility in good, operable condition then it would be possible to reactivate it rapidly, meaning that if the state chose to reverse its disarmament, then the capability it delivered could be made available again at short notice. This being the case, the effectiveness of freezing can be very limited, almost down to the level of being effectively as if no action had been carried out. However, the longer a freeze remains in place, the greater the detriment to the skills, knowledge and availability of the facility staff, creating delays and challenges should it become desirable to reactivate it. Likewise, unless full-scale maintenance activities are conducted, the facility and its equipment could fall into disrepair.
The 1994 Agreed Framework between the United States and North Korea placed freezes on the operation of its reactor and plutonium reprocessing facility (Hecker Citation2023, 33). While the country was able to restart these facilities after the agreement ended in 2002, the halt to plutonium production during that time set back the country’s weapons programme significantly, and according to Siegfried Hecker, North Korea has yet to recover from this (Hecker Citation2023, 83). This demonstrates that freezes on facility operation can be effective in enhancing irreversibility in disarmament, with impacts lasting beyond the period of the freeze itself.
Influencing Infrastructure – Modify for Transparency and Verification
Nuclear weapons facilities can be designed or retrofitted with features to enhance irreversibility or non-proliferation while still be able to operate (International Atomic Energy Agency Citation2010, 1). This is principally achieved through the reduction in secrecy at a site by allowing a degree of foreign or international verification over activities. Systems developed for the purposes of applying IAEA safeguards will likely be useful for this purpose. This will mean that facility operations can continue according to a disarmament strategy, with watchers gaining confidence in the state’s commitment to disarmament. In turn, the surveilled state will likely feel dissuaded from reversing disarmament due to the strong likelihood of this being identified and the resulting backlash from the international community. As discussed above, secrecy is a cornerstone of nuclear weapons production complexes, both as a matter of policy and culture, and so this verification would need to be performed in such a way as to avoid the transfer of sensitive information on the technical characteristics and capabilities of nuclear weapons to unapproved parties.
Influencing Infrastructure – Disable
The next potential action is disablement, where the facility remains standing, but action is taken to alter its design or configuration to prevent it from fulfilling its function (Albright and Brannan Citation2007). Unlike capping or freezing this does not pertain just to operational slowdown or halt but involves physical changes to make resumption of operation more challenging. Disablement can happen across a spectrum and its effectiveness in constraining a critical or sustaining capability is related to the function of the facility to be disabled and the ease through which the specific disabling actions can be reversed. Two examples of disablement from current or recent international practice can shed further light on the concept. Firstly, under the Joint Comprehensive Plan of Action (JCPOA), the calandriaFootnote18 of the Arak heavy water reactor, which could be used for high-quality plutonium production, was filled with concrete. Doing so effectively rendered this very large and critical piece of nuclear reactor equipment unusable to the point where it could never realistically be put back into service, while also making the facility effectively inoperable due to its continued presence within the reactor hall. Thus, while the facility was not decommissioned, it was disabled almost to the same level of effectiveness from an irreversibility perspective. Reversal of this disablement would necessitate the removal of the calandria, likely requiring careful breaking up the concrete as a first step, removal of old and damaged equipment, and reinstallation of a replacement, a likely long and costly process. Infilling of the calandria was thus highly irreversible.
Conversely, disablement actions may also be relatively easily reversed. The 2007 talks with North Korea regarding disablement of its nuclear programme led to a series of 11 actions, several of which pertained to the removal of equipment from facilities and its storage, actions which were readily reversible and could have allowed for the facilities affected to be returned to operation within 6–18 months, depending on how many of these actions were completed and their extent (Hecker Citation2008). Disablement can thus range in reversibility from trivial to almost the point of facility dismantlement, depending on how it is undertaken.
Influencing Infrastructure – Convert
Another potential action that results in a variable level of reversibility is the conversion of the facility to other uses besides its nuclear weapons role. Conversion to other uses will likely necessitate the removal of nuclear weapons-related equipment at minimum, and the likely adaptation of the facility to its new role.Footnote19 The degree of reversibility will be contingent upon exactly what is done to achieve the conversion, which in turn is driven in part by the new use of the facility. Most nuclear facilities are highly specialised and may not be readily adapted to other uses beyond perhaps the storage and/or processing of civil nuclear materials. For instance, a facility might be converted from a plutonium pit casting workshop to instead manufacture plutonium-based alloy fuel for fast reactors, or a military enrichment facility might have its centrifuge cascades reconfigured to produce low-enriched uranium fuel for use in nuclear power plants or sale on the international commercial market.
While such repurposing may make economic sense in many ways (retention of a local workforce with relevant existing skillsets, use of existing security and safety features, potential to use or modify existing equipment and processes), this presents a relatively low degree of irreversibility. Conversely, consider the scenario of converting the pit casting workshop to a completely different purpose, such as a medical centre. This might see the facility’s interior completely reconfigured, equipment dismantled, now-unnecessary security features removed, and so on. A range of middle ground conversion scenarios are also possible, where some proportion of original features, equipment or services are retained. Thus, as with disablement, reversibility in cases of facility conversion is variable. It may be more effective than disablement as some use cases will require a complete reconfiguration, or less effective given that disablement indicates actions motivated by enhancement of irreversibility, rather than motivated, at least in part, by continued use of the facility in some other capacity.
Influencing Infrastructure – Dismantle
The most effective action to enhance irreversibility is the complete decommissioning, dismantling and demolition of the facility, potentially including even foundations and supporting infrastructure, making it effectively as if the facility had never been built. The facility’s former location could be said to have returned to a “green field” status, ready for release to civilian uses. Incomplete dismantling/demolition is also possible, for instance, demolishing the facility but leaving supporting infrastructure and/or foundations in place. The effort required to reconstitute the capability at a later date on the same site will be driven by what remains, although the new facility would have to be substantially akin to its predecessor to reap the benefits of this.
Conclusion
Achieving nuclear disarmament in a way that affords higher levels of irreversibility will entail thinking beyond just getting rid of nuclear weapons. It will also pose challenging questions about the risk emanating from the nuclear infrastructure in a state, military and civilian, and in particular its weapons production complex. This article has explored the key processes and facilities that form the core of nuclear weapons production and therefore are critical to the nuclear weapons production complex. Suggestions are also offered for how nuclear weapons production complexes might be managed using a framework for enhancing irreversibility in a world which is progressing towards or has achieved a state of nuclear disarmament.
The status of these production complexes will have important consequences when considering the level of irreversibility achieved in any nuclear disarmament process. This is because such complexes provide the capability to produce and maintain nuclear weapons and therefore they would have a direct impact on the potential for re-armament. While this does not preclude the use of other routes to re-armament, such as through the use of civil and dual-use nuclear facilities, it emphasizes the importance of the nuclear weapons production complex as the primary, tried and tested, weaponisation route. Furthermore, adopting these alternative routes to produce weapons would be more expensive and time consuming. It therefore becomes important to understand the constitution and set up of the nuclear weapons production complex in a disarming state.
The article outlines the critical and sustaining capabilities that would be needed within any functional and reliable nuclear weapons production complex designed to produce and maintain simple fission weapons. These capabilities are also relevant to the production of more advanced weapons systems. Fusion materials production is not included within this article, but for these weapons an additional critical capability would be required – the production of tritium and/or lithium deuteride. These capabilities would also be required to produce more advanced weapon designs, including boosted and fusion weapons. Various nuclear-armed states have taken a range of national approaches in their complexes, which exhibit both divergences and commonalities between them. A survey of the individual weapons production complex in a disarming state is thus essential in moving from the generic capabilities herein discussed to an understanding of the state’s real complex. A key consideration here is the degree of integration and separation between the military and civilian fuel cycles and understanding the links between the production complex and other associated activities and sites, such as military storage and handling sites, research and development infrastructure, and nuclear testing facilities and capabilities. When examined alongside the historical development of the complex and its bureaucratic set up, these can provide a detailed picture of how a nuclear weapons programme was constituted, how it functions today, and the potential for disarmament reversal if that complex, or elements thereof, were to be used for this purpose.
The presence and operability of nuclear weapons production complex facilities can have considerable consequences for the irreversibility of nuclear disarmament. If all nuclear weapons are disarmed but the production complex remains operable and indeed operational, albeit in care and maintenance, nuclear disarmament remains readily reversible, with states able to use these facilities and any extent stocks of nuclear material to rapidly produce new weapons. Conversely, taking action on one or more critical capabilities within the complex will greatly increase the barriers to disarmament reversal, with the challenges of doing so increasing with the strength of action taken and the number and range of facilities involved. This special issue has emphasized the utility of considering irreversibility as a spectrum reflecting various degrees of the potential for re-armament rather than as a binary of either readily reversible or completely irreversible, the latter of which is highly likely to be unachievable. In applying that broad conception, a range of potential actions emerge that can be applied to segments or the entirety of the production complex. To various levels, these would constrain or hinder the normal operation of the complex either through capping or freezing certain operations or by altering the physical infrastructure of its key facilities. Each of these actions will have a distinct impact on the prospect of re-armament. For example, intuitively, a cap on production is more easily reversed than the dismantlement of a facility and therefore the latter makes re-armament more challenging. However, given the wide range of facilities as well as potential actions there are numerous possible facility-action pairings to evaluate and compare. This becomes even more complex for real nuclear weapons production complexes with subtle differences between the types of action that may be taken, given the selection of actual facilities to which these are applied. This article has outlined a framework with two axes and analysed the potential irreversibility impacts that brought by these axes largely separately. However, as illustrated, these axes suggest a matrix of action/facility pairings. Future work is recommended to develop a method to systematically evaluate what each of the different potential action/facility pairings would mean for irreversibility, comparing their feasibility and cost against their value in making re-armament more challenging. This would provide a valuable tool for disarmament planners in quantifying their range of options and ultimately deciding on a strategy. Ultimately, any serious consideration of irreversibility will entail engaging with searching questions about how to deal with the facilities and infrastructure that enable weapons production, and which thus carry within them the seeds of re-armament in a disarmed world.
Disclosure Statement
No potential conflict of interest was reported by the author(s).
Additional information
Notes on contributors
Hassan Elbahtimy
Hassan Elbahtimy is Senior Lecturer in the Department of War studies at King’s College London and previously served as Director of the Centre for Science and Security Studies (CSSS) and as Trustee and Executive Committee member of the British International Studies Association (BISA). He has written widely on international security and arms control and disarmament issues including in Foreign Affairs, Journal of Strategic Studies, Security Studies, and the Nonproliferation Review, among others. His research was awarded the McElveny Grand Prize by the Nonproliferation Review.
Ross Peel
Ross Peel is a Research and Knowledge Transfer Manager in the Centre for Science and Security Studies (CSSS) in the Department of War Studies at King’s College London. In this role, he leads research activities on nuclear security and safeguards, and examines the role of nuclear power plants in war and as tools of international diplomacy. Prior to joining King’s College London, Ross spent time in industry as a Nuclear Systems Consulting Engineer with Nuclear-21 Ltd. He gained his PhD in nuclear energy engineering from the University of Sheffield in 2017.
Notes
1 See the definition in Elbahtimy (Citation2023).
2 For an exposition and critique of the limitations of the nuclear proliferation paradigm see Pelopidas (Citation2011).
3 While the production, maintenance and disposal functions are more familiar components of the complex, recycling is the process through which fissile material are extracted from a warhead to be re-used for other purposes. Fissile material pits are formed from highly purified radioactive metals or alloys, which can be chemically purified and recycled apart from small quantities lost through radioactive decay, chemical processing losses, and pit forging/machining. The material’s useful life is limited by its isotopic composition, which denatures over time, eventually passing a point where its expected performance falls below acceptable levels, and it must be disposed of or subjected to chemical purification and refabrication.
4 It is of course possible to produce weapons at the at a slow rate within suitable R&D facilities as was done in the in the United States under the Manhattan Project, yet such capacity is much more limited compared to the industrial scale capacity to produce these weapons that was achieved later as the complex grew and expanded.
5 United States Code, Title 50, Ch 42, § 2501, para (6).
6 Defence Nuclear Organisation website. Available online: https://www.gov.uk/government/organisations/defence-nuclear-organisation/about
7 The Convention is in UN Document A/62/650 and provides a definition of “Nuclear Weapons Production Facility” as “any nuclear facility which produces materials which have been or may be used for military purposes, including such a reactor, a plant for processing nuclear material irradiated in a reactor, a plant for separating the isotopes of nuclear material, a plant for processing or fabricating nuclear material, a plant for the construction or assembly of nuclear weapon components, or a facility or plant of such other type as may be deemed a Nuclear Weapons Production Facility by the Technical Secretariat”.
8 For details of States Parties’ legal obligations under the non-proliferation treaty, see, e.g. Joyner (Citation2011).
9 Examples of nuclear espionage which would have contributed to the early requirement for secrecy include several identified individuals who worked on the Manhattan Project and provided secrets to the Soviet Union, such as Klaus Fuchs and David Greenglass (see, e.g. Rhodes (Citation1995)). Examples of early state-to-state nuclear cooperation in nuclear weapons include the sharing of information on nuclear weapons between the United States and the United Kingdom under the 1958 Mutual Defence Agreement (see, e.g. Baylis (Citation2008)) and the cooperation between France and Israel in the late 1940s and 1950s (see, e.g. Pinkus (Citation2002)).
10 The texts of the Voluntary Offer Agreements with the five NPT Nuclear Weapons States can be found on the International Atomic Energy Agency website here: https://www.iaea.org/topics/safeguards-legal-framework/more-on-safeguards-agreements
11 The United States, for instance, produces tritium through the Watts Bar facility, an otherwise “civil” nuclear power plant (Woolf and Werner Citation2021, 19, 23, 31).
12 See the excellent reports and data compiled by the International Panel on Fissile Materials available here: https://fissilematerials.org/.
13 The five states whose first nuclear explosion took place before 1967 have all stopped or are believed to have stopped fissile material production. The other four states with known active nuclear weapons programmes are still producing fissile material. This is according to the individual country reports from the International Panel for Fissile Materials, available online from https://fissilematerials.org/.
14 Details of electromagnetic separation processes can be found in US Department of Energy Office of History and Heritage Resources (Citationn.d., 165–169). Details of Iraq’s use of this process are available in Albright, and Hibbs (Citation1991).
15 Example radiation dose rates from US spent fuel assemblies are ~ 100 Gray per hour 10 years after removal from a reactor, meaning that a fatal dose of radiation would be received within minutes, and severe long-term health effects would occur within seconds, according to US NRC Office of Public Affairs (Citation2019, 1).
16 A breakdown of the components of thermonuclear weapons and the US experience in dismantling them in the 1990s can be found in US Congress, Office of Technology Assessment (Citation1993, 35–39).
17 For a comparative overview of nuclear weapons R&D capabilities: Medalia et al. (Citation2013).
18 In heavy water reactors, the calandria is a large tank with numerous parallel tubes running through it, filled with heavy water providing the neutron moderating function. The fuel is placed within the tubes running through the calandria, and coolant passes through the tubes.
19 For some proposals on conversion of Russian facilities after the end of the cold war see: Bunn (Citation1997).
References
- Ackland, L. 2002. Making a Real Killing: Rocky Flats and the Nuclear West. Albuquerque, NM: University of New Mexico Press.
- Albright, D., and P. Brannan. 2007. Disabling DPRK Nuclear Facilities. United States Institute of Peace Working Papers series. United States Institute of Peace. https://www.usip.org/publications/2007/10/disabling-dprk-nuclear-facilities/.
- Albright, D., and M. Hibbs. 1991. “Iraq’s Nuclear Hide-And-Seek.” Bulletin of the Atomic Scientists 47 (7): 14–23. https://doi.org/10.1080/00963402.1991.11460006.
- Baker, R. D., S. S. Hecker, and D. R. Harbur. 1983. “Plutonium - A Wartime Nightmare but a Metallurgist’s Dream.” Los Alamos Science, Winter/spring 1983:142–151. https://sgp.fas.org/othergov/doe/lanl/lib-www/pubs/00285891.pdf.
- Baylis, J. 2008. “The 1958 Anglo-American Mutual Defence Agreement: The Search for Nuclear Interdependence.” Journal of Strategic Studies 31 (3): 425–466. https://doi.org/10.1080/01402390802024726.
- Bischak, G. 1988. “Facing the Second Generation of the Nuclear Weapons Complex: Renewal of the Nuclear Production Base or Economic Conversion?” Bulletin of Peace Proposals 19 (1): 81–97. https://doi.org/10.1177/096701068801900106.
- Bodansky, D. 2007. Getting to the Core of the Nuclear Fuel Cycle: From the Mining of Uranium to the Disposal of Nuclear Waste. International Atomic Energy Agency. https://www.iaea.org/sites/default/files/18/10/nuclearfuelcycle.pdf.
- Buffett, S. J. 2013. Tacit Knowledge Involvement in the Production of Nuclear Weapons: A Critical Component of a Credible US Nuclear Deterrent in the 21st Century. https://apps.dtic.mil/sti/pdfs/AD1018881.pdf.
- Bunn, M. 1997. The Nuclear Weapons Complexes: Meeting the Conversion Challenge: A Proposal for Expanded Action. Russian-American Nuclear Security Advisory Council. https://dash.harvard.edu/bitstream/handle/1/27413768/Bunn_The_Nuclear_Weapons_Complexes_1997.pdf?sequence=1.
- Carless, T. S., K. Redus, and R. Dryden. 2021. “Estimating Nuclear Proliferation and Security Risks in Emerging Markets Using Bayesian Belief Networks.” Energy Policy 159:112549. https://doi.org/10.1016/j.enpol.2021.112549.
- Coggeshall, K., V. Grant, and W. Spivey. 2021. “Pit production explained.” National Security Science 46–52. https://cdn.lanl.gov/files/nss-winter-2021-online_cacdf.pdf.
- Comptroller and Auditor General. 2018. The Defence Nuclear Enterprise: A Landscape Review. HC 1003. https://www.nao.org.uk/wp-content/uploads/2018/05/The-Defence-Nuclear-Enterprise-a-landscape-review.pdf.
- Congressional Budget Office. 2023. Projected Costs of U.S. Nuclear Forces, 2023 to 2032. https://www.cbo.gov/publication/59365.
- Deputy Assistant Secretary of Defense for Nuclear Matters. 2020. ‘Chapter 4: Nuclear Weapons’ in Nuclear Matters Handbook 2020 [ Revised]. https://www.acq.osd.mil/ncbdp/nm/NMHB2020rev/chapters/chapter4.html.
- Elbahtimy, H. 2023. “Approaching Irreversibility in Global Nuclear Politics.” Journal of Peace and Nuclear Disarmament.
- Feiveson, H. A., A. Glaser, Z. Mian, and F. N. Von Hippel. 2014. Unmaking the Bomb: A Fissile Material Approach to Nuclear Disarmament and Nonproliferation. Cambridge, MA: MIT Press.
- Fitzpatrick, M. 2020. ‘Iran’s Nuclear Latency Needs to Be Guarded, Not Goaded’. The Survival Editor’s Blog, 6 March. https://www.iiss.org/blogs/survival-blog/2020/03/iran-low-enriched-uranium-stockpile-iaea-report.
- Forge, J. 2010. “A Note on the Definition of “Dual use”.” Science and Engineering Ethics 16 (1): 111–118. https://doi.org/10.1007/s11948-009-9159-9.
- Fuhrmann, M. 2009. “Spreading Temptation: Proliferation and Peaceful Nuclear Cooperation Agreements.” International Security 34 (1): 7–41. https://doi.org/10.1162/isec.2009.34.1.7.
- Guinnessy, P. 2001. “Pakistan Reshuffles Weapons Program.” Physics Today 54 (5): 28. https://doi.org/10.1063/1.1381095.
- Hecker, S. 2008. Report of Visit to the Democratic People’s Republic of North Korea (DPRK) Pyongyang and the Nuclear Center at Yongbyon, Feb. 12-16, 2008.
- Hecker, S. 2023. Hinge Points – an Inside Look at North Korea’s Nuclear Program. Redwood City, CA: Stanford University Press.
- Holden, N. E. 1981. The Uranium Half-Lives: A Critical Review. Brookhaven National Laboratory. https://inis.iaea.org/collection/NCLCollectionStore/_Public/13/691/13691196.pdf.
- International Atomic Energy Agency. 2010. “Technical Features to Enhance Proliferation Resistance of Nuclear Energy Systems Vol. NF-T-4.5.” In IAEA Nuclear Energy Series (pp. 1). Vienna: International Atomic Energy Agency.
- International Atomic Energy Agency. 2020. “Design Principles and Approaches for Radioactive Waste Repositories.” In IAEA Nuclear Energy Series No. NW-T-1.27 (pp. 3-11). Vienna: International Atomic Energy Agency
- Joyner, D. H. 2011. Interpreting the Nuclear Non-Proliferation Treaty. Oxford, UK: Oxford University Press.
- Kelley, R. E. 2020. A Technical Retrospective of the Former South African Nuclear Weapon Programme. Stockholm International Peace Research Institute. https://www.sipri.org/sites/default/files/2020-10/sa_nuclear_technical_retrospective_kelley_2.pdf.
- Kristensen, H. M., M. Korda, and E. Johns. 2023. “French Nuclear Weapons, 2023.” Bulletin of the Atomic Scientists 79 (4): 272–281. https://doi.org/10.1080/00963402.2023.2223088.
- Lashley, J. C., M. Blau, K. P. Staudhammer, and R. A. Pereyra. 1999. “In situ Purification, Alloying and Casting Methodology for Metallic Plutonium.” Journal of Nuclear Materials 274 (3): 315–319. https://doi.org/10.1016/s0022-3115(99)00074-4.
- Lauf, D., and B. Buchheister. 2023. Cleaning Up America’s Nuclear Weapons Complex: 2023 Update for Governors. National Governors Association. https://www.nga.org/wp-content/uploads/2023/04/2023-Governors-Guide_FINAL.pdf.
- MacKenzie, D., and G. Spinardi. 1995. “Tacit Knowledge, Weapons Design, and the Uninvention of Nuclear Weapons.” American Journal of Sociology 101 (1): 44–99. https://doi.org/10.1086/230699.
- Martz, J. C., and A. J. Schwartz. 2003. “Plutonium: Aging Mechanisms and Weapon Pit Lifetime Assessment.” JOM: The Journal of the Minerals, Metals & Materials Society 55 (9): 19–23. https://doi.org/10.1007/s11837-003-0023-0.
- Mazarr, M. J. 1995. “Virtual nuclear arsenals.” Survival 37 (3): 7–26. https://doi.org/10.1080/00396339508442800.
- Medalia, J. E. 2015. “Nuclear Weapon “Pit” Production: Options to Help Meet a Congressional Requirement.” Congressional Research Service R44033. https://crsreports.congress.gov/product/pdf/R/R44033.
- Medalia, J., D. E. Mix, P. Belkin, M. B. Nikitin, S. A. Kan, J. Zanotti, and P. K. Kerr. 2013. Nuclear Weapons R&D Organizations in Nine Nations. CRS Report.
- Miller, N. L. 2017. “Why Nuclear Energy Programs Rarely Lead to Proliferation.” International Security 42 (2): 40–77. https://doi.org/10.1162/ISEC_a_00293.
- Mills, C. 2023a. Nuclear Weapons at a Glance: United Kingdom. House of Commons Research Briefing number 9077. https://researchbriefings.files.parliament.uk/documents/CBP-9077/CBP-9077.pdf.
- Mills, C. 2023b. Replacing the Uk’s Strategic Nuclear Deterrent: Progress of the Dreadnought Class. London: House of Commons. https://researchbriefings.files.parliament.uk/documents/CBP-8010/CBP-8010.pdf.
- Monshipouri, M. 2022. In the Shadow of Mistrust: The Geopolitics and Diplomacy of US-Iran Relations. Oxford, UK: Oxford University Press. https://doi.org/10.1093/oso/9780197659632.003.0007.
- Murray, R. L., and K. E. Holbert. 2015. Nuclear Energy – an Introduction to the Concepts, Systems, and Applications of Nuclear Processes. Waltham, MA: Butterworth-Heinemann.
- Netter, P. 2012. “Reprocessing of Spent Oxide Fuel from Nuclear Power Reactors.” In Nuclear Fuel Cycle Science and Engineering, edited by I. Crossland, 485–488. Cambridge, United Kingdom: Woodhead Publishing Limited.
- Pelopidas, B. 2011. “The Oracles of Proliferation: How Experts Maintain a Biased Historical Reading That Limits Policy Innovation.” The Nonproliferation Review 18 (1): 297–314. https://doi.org/10.1080/10736700.2011.549185.
- Petit, A. 1994. “Transparency at the Level of Safeguards Implementation.” JNMM (Journal of the Institute of Nuclear Materials Management) 22 (4): 25–26.
- Pinkus, B. 2002. “Atomic Power to Israel’s Rescue: French-Israeli Nuclear Cooperation, 1949-1957.” Israel Studies 7 (1): 104–138. https://doi.org/10.2979/ISR.2002.7.1.104.
- Plummer, D. W., and W. H. Greenwood. 1998. The History of Nuclear Weapon Safety Devices. Sandia National Laboratory, SAND-98-1184C. https://www.cs.columbia.edu/~smb/nsam-160/671923.pdf.
- Podvig, P. L., and O. Bukharin. 2004. Russian Strategic Nuclear Forces (1st MIT Press Pbk.). Cambridge, MA: MIT Press.
- Rhodes, R. 1995. Dark Sun: The Making of the Atomic Bomb. New York: Simon & Schuster.
- Ritchie, N. 2023. “Irreversibility and Nuclear Disarmament: Unmaking Nuclear Weapon Complexes.” Journal for Peace & Nuclear Disarmament 1–26. https://doi.org/10.1080/25751654.2023.2282737.
- Rockwood, L. 2013. Legal Framework for IAEA Safeguards. https://www.iaea.org/sites/default/files/16/12/legalframeworkforsafeguards.pdf.
- Schaper, A., and H. Müller. 2009. “Torn Apart: Nuclear Secrecy and Openness in Democratic Nuclear-Weapon States.” In Democracy and Security, edited by M. Evangelista, H. Muller, and N. Schoernig, 159–182, London: Routledge.
- Schell, J. 2000. The Fate of the Earth and the Abolition. Redwood City, CA: Stanford University Press.
- Schneider, M., and A. Froggatt. 2023. The World Nuclear Industry Status Report 2022. 3rd ed. https://www.worldnuclearreport.org/-World-Nuclear-Industry-Status-Report-2022-.html.
- Schneider, M., and M. V. Ramana. 2023. “Nuclear Energy and the Non-Proliferation Treaty: A Retrospective Examination.” Journal for Peace & Nuclear Disarmament 6 (1): 1–10. https://doi.org/10.1080/25751654.2023.2205572.
- Schwartz, S. 1998. Atomic Audit: The Costs and Consequences of U.S. Nuclear Weapons Since 1940. Washington, DC: Brookings Institution Press.
- Sims, B., and C. R. Henke. 2012. “Repairing Credibility: Repositioning Nuclear Weapons Knowledge After the Cold War.” Social Studies of Science 42 (3): 324–347. https://doi.org/10.1177/0306312712437778.
- Stulberg, A. N. & M. Fuhrmann, Eds. 2013. The Nuclear Renaissance and International Security. Redwood City, CA: Stanford University Press.
- UN Security Council. 2015. Security Council Resolution 2231 (2015) [On Joint Comprehensive Plan of Action (JCPOA) on the Islamic Republic of Iran’s Nuclear Programme]. https://www.undocs.org/Home/Mobile?FinalSymbol=S%2FRES%2F2231(2015).
- US Congress, Office of Technology Assessment. 1993. Dismantling the Bomb and Managing the Nuclear Materials. OTA-O-572. Washington, DC: US Government Printing Office.
- US Department of Defense. 2022. Nuclear Posture Review. Washington DC: US Department of Defense. https://fas.org/wp-content/uploads/2023/07/2022-Nuclear-Posture-Review.pdf.
- US Department of Energy Office of History and Heritage Resources. n.d. ‘Electromagnetic Separation’. The Manhattan Project, an Interactive History. https://www.osti.gov/opennet/manhattan-project-history/Processes/UraniumSeparation/electromagnetic.html.
- US NRC Office of Public Affairs. 2019. Radioactive Waste. https://www.nrc.gov/docs/ML0501/ML050110277.pdf.
- Weaver, J. 2015. One in a Million Given the Accident: Assuring Nuclear Weapon Safety. Center for Strategic and International Studies. https://www.osti.gov/biblio/1426902.
- Weiss, L. 2007. “U.S.-India Nuclear Cooperation – Better Later Than Sooner.” The Nonproliferation Review 14 (3): 429–457. https://doi.org/10.1080/10736700701611738.
- Wellerstein, A. 2021. Restricted Data: The History of Nuclear Secrecy in the United States. Chicago, IL: University of Chicago Press.
- Westwick, P. J. 2000. “In the Beginning: The Origin of Nuclear Secrecy.” Bulletin of the Atomic Scientists 56 (6): 43–49. https://doi.org/10.1080/00963402.2000.11457012.
- Wilks, J. 2012. ‘Uranium Conversion and Enrichment,’ in Nuclear Fuel Cycle Science and Engineering, edited I. Crossland, 158–165. Cambridge, United Kingdom: Woodhead Publishing Limited.
- Woolf, A. F., and J. D. Werner. 2021. The U.S. Nuclear Weapons Complex: Overview of Department of Energy Sites. Congressional Research Service R45306. https://crsreports.congress.gov/product/pdf/R/R45306.
- World Nuclear Association. 2023. ‘US Nuclear Power Policy’. https://world-nuclear.org/information-library/country-profiles/countries-t-z/usa-nuclear-power-policy.aspx.
- Zimmerman, P. D. 1993. “Technical Barriers to Nuclear Proliferation.” Security Studies 2 (3–4): 349. https://doi.org/10.1080/09636419309347530.