Abstract
It is increasingly evident that cells and molecules of the immune system play significant roles in neurodevelopment. As perinatal infection is associated with the development of neurodevelopmental disorders, previous research has focused on demonstrating that the induction of neuroinflammation in the developing brain is capable of causing neuropathology and behavioral changes. Recent studies, however, have revealed that immune cells and molecules in the brain can influence neurodevelopment without the induction of overt inflammation, identifying neuroimmune activities as integral parts of normal neurodevelopment. This mini-review describes the shift in literature that has moved from emphasizing the intrusion of inflammatory events as a main culprit of neurodevelopmental disorders to evaluating the deviation of the normal neuroimmune activities in neurodevelopment as a potential pathogenic mechanism.
Introduction
Mammalian neurodevelopment is a complex and intricately regulated process that allows the formation of the mature central nervous system (CNS) that dictates the autonomic life-sustaining functions of the internal organs, controls motor activities of the muscles, performs cognitive assessments of the environment, and produces affective responses to emotional stimuli. This process involves the productionCitation1 and migration of neurons,Citation2 elimination of unwanted neurons,Citation3 formation and reshaping of neurocircuits,Citation4 interaction and integration of glial cells,Citation5–7 and incorporation of early life experiences into the maturation processes of the CNS.Citation8,Citation9 During neurodevelopment, both intrinsic and extrinsic factors could derail its proper course. Thus, perinatal injury or infection are well-known extrinsic risk factors that are associated with the development of psychiatric or psychological disorders later in life.Citation10–12 These risk factors could produce significant pathology if they are combined with genetic mutations (intrinsic risk factors) that predispose the individuals to developmental mental disorders.Citation13–16 A critical facilitator of neurodevelopmental diseases that has been spotlighted by recent research is neuroinflammation.Citation17–21 Obviously, injury and infection of the CNS are likely to trigger neuroinflammation as part of the immune reaction to wounding and invading pathogens. The potential bystander damage a neuroinflammatory reaction might inflict upon the developing nervous tissue was thought to be an important pathogenic mechanism of neurodevelopmental diseases.Citation22,Citation23 Although this thesis was initially supported by animal models of relatively severe perinatal injury or infection/inflammation and by those of CNS inflammatory cytokine over-expression,Citation24–27 frank inflammation in the CNS is not common in most neurodevelopmental diseases. Therefore, the influence of less blatant “neuroinflammatory” activities may be more important in causing most of the aberrant neurodevelopmental changes. New discoveries have now identified non-inflammatory neuroimmune activities in the regulation of physiological processes of the nervous system, suggesting that immune cells in the CNS, eg, microgliaCitation5 and T cells,Citation28 and immune signal molecules, eg, cytokines,Citation29–31 chemokinesCitation32,Citation33 and complements,Citation34,Citation35 are capable of modulating neuronal function and neuronal connectivity without eliciting the attendant traditional immunological or inflammatory activities these factors were previously known for. In this mini-review, we will briefly summarize the history of research that has implicated neuroinflammation in neurodevelopmental diseases and discuss how current research has shifted its focus to a broader context that encompasses the impact of non-inflammatory neuroimmune activities on neurodevelopment.
Origin of Immune-Driven Neurodevelopmental Diseases Theory
The impact of immune activity on mental health might first have been deduced from historical observations that almost every form of CNS disease may follow an infectious epidemic. For example, various neuropsychiatric symptoms were noted as early as the years following the 1385 German Flu. Similar observations were made after the Russian Flu of 1889 and the Spanish Flu of 1918.Citation36 Mental illnesses also appeared after infectious diseases caused by other viruses and bacteria such as HIV, Zika virus, and Group B streptococcus.Citation37 Currently, the COVID-19 pandemic is well-known to cause long COVID symptoms which include many psychiatric comorbidities.Citation38 As infections inevitably stimulate the immune system, an immune-driven pathogenic mechanism for CNS diseases may be surmised.
Not only may adults display psychopathic sequela from infectious diseases, but their offspring also show higher rates of developing mental or neurological disorders.Citation39–42 In addition, perinatal infection and childhood infection have been found to increase the risk of developing mental illness later in life.Citation39,Citation41,Citation43–45 These findings point to the possibility that immune activation in the developing nervous system during the perinatal period or in early childhood could significantly influence neurodevelopment, thereby causing behavioral aberrations later in life.
This notion has been extensively tested in animal models of maternal immune activation (MIA) or perinatal immune activation (PIA). The majority of these studies used E. coli, lipopolysaccharide (LPS), or polyIC to stimulate immune activity in the fetal brain or in the postnatal CNS. LPS is a component of the bacterial cell wall and polyIC is a synthetic analog of viral double-stranded RNA. LPS and polyIC do not cause infection; rather, they induce immune activation, mimicking those induced by bacterial or viral infection, respectively. These studies clearly show that activation of the immune system without a true infection can alter neurodevelopmentCitation46 and produce behavioral phenotypes that resemble various neurological and psychological disorders.Citation46–49 A common consequence of immune activation is the induction of cytokines. Cytokines are the communication molecules of the immune system that stimulate immune cell proliferation and the production of effector molecules designed to destroy and clear invading pathogens. Not surprisingly, MIA and PIA can drive increased expression of inflammatory cytokines in the developing brain.Citation50–52 Further, the expression levels of inflammatory cytokines in these models often correlate with behavioral outcomes,Citation53 and brain specific transgenic over-expression of inflammatory cytokines during development results in significant neurological pathologies.Citation27 In addition, blockade of activities of inflammatory cytokines with their specific inhibitors can alleviate MIA- or PIA-induced neurological and behavioral changes.Citation24,Citation54–57 Thus, increased inflammatory cytokine expression in the perinatal brain has been considered a critical mediating event that derails neurodevelopment.
Bystander Damage Hypothesis
The mechanisms by which neuroinflammatory activities alter neurodevelopment remain poorly understood, although an obvious postulation is bystander damage. A full-blown inflammatory response should induce heightened expression of inflammatory cytokines, infiltration of immune cells to the infected or injured tissue,Citation58 and the production of reactive oxygen species (ROS).Citation59 Therefore, bystander damage to the developing brain from immune activation could occur if: (1) inflammatory cytokines at high levels are neurotoxic by themselves, (2) the recruited peripheral leukocytes can cause neuronal injury, and (3) ROS causes neurotoxicity. These three mechanisms may not be mutually exclusive. In in vitro culture systems, adding inflammatory cytokines, especially TNFa, were shown to cause neuronal death,Citation60–62 sometimes via induction of ROS in glial cells.Citation63 In addition, infiltrating neutrophils can cause neuronal death through the release of extracellular proteases.Citation64 In vivo, these mechanisms are probably the cause of neuropathology found in transgenic animals with brain-specific over-expression of inflammatory cytokines.Citation27 It should be noted that, while there is no doubt that bystander damage following frank CNS inflammation in the developing brain can cause neurological and psychiatric pathologies, it is often associated with gross structural changes in the brain, eg, enlargement of cerebral ventriclesCitation65–67 and reduced hippocampal volume.Citation68,Citation69 Although gross structural abnormalities have indeed been associated with neurodevelopmental diseases,Citation70,Citation71 these changes are not obligatory for these disorders, suggesting that subtler immune-driven pathogenic mechanisms need to be investigated.
One important conceptual advance is the “two-hit” or “multiple-hit” theory. According to this theory, perinatal immune activation causes subtle changes in development, which prime the system such that, upon receiving a second “hit” simultaneously or later in life, pathological behaviors are finally elicited. An excellent example here is a body of work from Bilbo et al showing that neonatal infection with E. coli primed infected rats such that later a low dose LPS challenge induced memory deficit only in rats with the prior neonatal infection.Citation22 They were also able to demonstrate that neonatal infection caused long-lasting re-programming of microglial cells in the brain that allows the second hit of a low dose of LPS to induce high levels of brain IL-1β that is required for the manifestation of memory impairment. A shortcoming of this specific two-hit theory is that the impact of the first hit directly on neural circuit development was not addressed. Other two-hit models exist. For example, transient blockade of glutamate neurotransmission together with early life social isolation (which could cause neuroimmune activation in the brain) can produce schizophrenia-like behaviors later in life.Citation72 In addition, MIA cooperates with neonatal hypoxia ischemia to promote autism-like behaviors in offspring.Citation73 These multiple-hit theories are also supported by clinical evidence showing that genetic risk factors and early life neuroimmune disturbances can combine to produce developmental psychiatric diseases.Citation74–76 These studies also revealed that different risk factors (genetic, inflammatory, stress, nutritional) may combine at different time points of neurodevelopment to cause mental health disorders. A corollary challenge, however, is that there are a very high number of combinatorial possibilities for these “hits” and each type of “combinatorial multiple-hit” could represent a different pathogenic mechanism for a given disease, rendering discovering common underlining mechanisms difficult.
Brave Neuroimmune World
Recent advances in biotechnology have significantly improved our ability to observe and manipulate genes, proteins, cells and neural circuits. As a result, an unexpected revelation is that immune cells and immune molecules were found to have normal functions in the CNS outside of the context of immune stimulation. Rather than using the word neuroinflammatory to describe something associated with or evolved from injurious or infectious stimulations, the word neuroimmune is now employed to encompass entities and events related to non-inflammatory CNS functions executed by cells and molecules previously believed to belong to the immune system. In this new world of the neuroimmune system, microglia, astrocytes, mast cells, and T cells are recognized to modulate numerous aspects of neurodevelopment, whereas cytokines, chemokines, completements, and MHC molecules are found to participate in the homeostatic and circuit-organizing activities of the CNS. This broader perspective of neuroimmune integration invites a rethinking of how the immune system might impact neurodevelopment and related mental health disorders. Whereas the previous paradigms would primarily see increased neuroinflammatory activity as a risk, the current paradigm would also examine the deleterious effects of inappropriate down-regulation of neuroimmune activity. Further, low-level increase of neuroimmune activity, which does not result in frank inflammatory responses in the brain and is not caused by infection and injury, has been analyzed to uncover how deviation from homeostatic neuroimmune activities can produce significant neurodevelopmental deficits.
Present but Different
The CNS has long been considered a site of immunological privilege.Citation77 Physically, most immune cells and immune signal molecules are prevented from entering the CNS by the blood–brain barrier (BBB);Citation78 functionally, transplanted tissue that is rejected in the periphery may survive in the CNS, indicating a muted environment for immune activation.Citation79 The notion of brain immunological privilege, however, does not translate to the absence of an immune system in the CNS.
The most abundant CNS immune cells are the microglia. These cells are closely related to peripheral macrophages and monocytes. They were initially considered to be derived from bone marrow hematopoiesis, similar to their peripheral counterparts. It is now established that most microglia originate in the yolk sac early in embryonic development.Citation80 They then populate the primitive brain and co-evolve with the developing nervous system to adapt to this unique environment. A small percentage of microglia may still be derived from peripheral monocytes, especially when significant neurodegeneration is present in the brain.Citation81,Citation82 Furthermore, monocyte-derived microglia may produce higher amounts of inflammatory cytokinesCitation83 and contribute more to the pathogenesis of neurodevelopmental diseases.Citation73 Most of the CNS microglia display distinct phenotypes compared to peripheral monocytes. For example, microglia express low levels of the cell surface marker CD45, such that immunohistochemical labeling of CD45 generally stains infiltrating leukocytes but not microglia.Citation58 In addition, although microglia are the primary producer of the inflammatory cytokine interleukin-1 (IL-1), they do not express the receptor for IL-1 (IL-1R) in the unstimulated brain, thus preventing autocrine amplification of inflammatory reaction that can be induced in the peripheral monocytes.Citation84 Further, microglia may even mediate anti-inflammatory activities induced by peripheral LPS preconditioning.Citation85 These findings suggest that microglia may perform similar inflammatory functions as peripheral macrophages, but at a somewhat temperate level. On the other hand, microglia are now discovered to modulate neural functions without the presence of inflammation.
The function of microglia in the normal brain was suggested by the finding that “resting microglia” are highly mobile; that is, their processes are constantly in motion to surveil the CNS environment.Citation86 During development, neurons produced in excess undergo apoptosis and produce “find-me” signals to guide microglia to remove them.Citation87,Citation88 Interestingly, after phagocytosing dead neurons, microglia can also secrete factors to promote the genesis of new neurons,Citation89 thereby modulating both life and death of neurons. In addition, developmental imbalance of excitatory/inhibitory circuit activity can lead to apoptosis of just the excitatory dendritic spines which are then deleted by activated microglia.Citation90 An even finer modulatory modality is microglial synaptic pruning. An excellent body of recent work has established that microglia prune less efficient synapses in an activity-dependent manner.Citation91,Citation92 This process is guided by a specific synaptic “eat-me” signal,Citation93 fine-tuned by inhibitory pathways to prevent inappropriate pruning,Citation94 and mediated by opsonization of activated complement molecules.Citation91 In the absence of neuroinflammation, such microglial synaptic pruning is an integral part of normal brain developmentCitation95,Citation96 and deficiency in neuron–microglia signaling in this process impairs neural connectivity and social behavior.Citation97 Different from inflammation-triggered phagocytosis of the whole dead cell body, ultrastructural analysis found that microglia can focus their phagocytic activity just on the synapse by nibbling on the presynaptic structure and enveloping the postsynaptic spine head with filopodia.Citation98 Even more exquisite is the phenomenon that under certain conditions microglia can displace inhibitory presynaptic terminals on neuronal cell bodies,Citation99,Citation100 thereby altering the functional characteristics of neurocircuits. Further, microglia-derived BDNF can play a role in promoting synaptogenesis, thus allowing microglia to modulate both the initiation and elimination of synaptic structures.Citation101 Another way in which microglia influence neural function is that they can guide the migration of CNS precursor cells by secreting chemotactic factors for neural stem cells.Citation102 These new findings demonstrate that these professional CNS immune cells have a gentler side: in addition to destroying invading pathogens and clearing debris and waste materials, they modulate neurotransmission and neural connectivity during development and in adult homeostasis. It is these non-inflammatory functions that are beginning to be recognized as critical activities that might be involved in the pathogenesis of neurodevelopmental diseases.
This new understanding has begun to be applied to studies investigating animal models of neurodevelopmental disorders. For example, Lebovitz et al used a mouse model of maternal microbiome dysbiosis (MMD) to cause social behavior deficits in offspring.Citation103 These offspring also showed microglial dystrophy and increased Cx3cr1, a microglia-specific receptor, expressed in the prefrontal cortex, suggesting microglial dysfunction and altered microglia–neuron interaction. Treating the MMD dams with the probiotic Lactobacillus attenuated the MMD-induced social deficits in offspring and prevented microglial morphological dystrophy and the over-expression of Cx3cr1. In addition, Cx3cr1 knockout prevented MMD-induced social deficits in the offspring. No frank neuroinflammation was observed in this study, demonstrating in this model that altered non-inflammatory microglial function is the cause of neurodevelopment psychopathology. On the other hand, deletion of Cx3cr1 during the early postnatal period by itself resulted in deficient synaptic pruning, weak synaptic neurotransmission, and autism-like behaviors.Citation97 Thus, changes in Cx3cr1 in either direction could result in developmental abnormalities, indicating that the neurophysiological functions of microglia, not just the neuroinflammatory activities of microglia, can be involved in the pathogenesis of developmental disorders.
Other Neuroimmune Actors in Neurodevelopment
While the neuroimmune functions of microglia are gaining prominence, other cells and microglia-interacting molecules are also emerging as critical neuroimmune influencers of neurodevelopment.
Astrocytes are the other major glial cells in the CNS. After CNS injury or infection, astrocytes are activated by inflammatory cytokines. In response, astrocytes can exacerbate or inhibit neuroinflammation depending on specific circumstances.Citation104 For example, stimulation of the receptor of interleukin-1 (IL-1) on astrocytes could result in an inhibition of microglial production of inflammatory cytokinesCitation58 and reduce excitotoxin-induced neuronal apoptosis.Citation105 On the other hand, during epilepsy, astrocyte activation can augment inflammation and increase neural damage.Citation106 In homeostasis of the adult CNS, astrocytes regulate interaction between neuronal activity and brain microcirculation, neurotransmitter levels in synaptic clefts, extracellular K+ concentration, CNS water content, synaptogenesis and synaptic pruning.Citation107 It is perceivable that these neuromodulatory functions of astrocytes could be altered by neuroimmune factors during neurodevelopment, thereby contributing to the pathogenesis of neurodevelopmental disorders.
Indeed, initial studies discovered that developmental astrocytes promote efficient synaptogenesis.Citation108 Later, synaptogenic molecules produced from developing astrocytes such as thrombospondins (TSP1 and 2)Citation109 and hevinCitation110 were identified. Interestingly, TSP plays important roles in peripheral inflammation and injury,Citation111 and hevin is involved in tissue remodeling,Citation112 adding these molecules to the list of neuroimmune factors. In addition, transcriptomic analysis revealed that astrocytes express phagocytic pathway proteinsCitation113 and the two phagocytic receptors, Megf10 and Mertk, were found to mediate astrocytic synaptic pruning during development.Citation114 Outside the context of normal neurodevelopment, astrocytic phagocytosis through these receptors is involved in the clearance of apoptotic neurons and debrisCitation115–117 during CNS tissue injury. Thus, astrocytic phagocytosis may also straddle two kingdoms: inflammatory astrocyte phagocytosis is part of post-injury tissue repair, whereas non-inflammatory astrocyte phagocytosis participates in neurodevelopmental synaptic structuring.
Another modality by which neuroimmune activities of the astrocytes can influence neurodevelopment is through cytokines and cytokine receptors. For example, astrocytic TGFb can promote the formation of inhibitory synapsesCitation118 and astrocytic IL-10 receptor mediates a feedback inhibition of microglial activation, which indirectly impact neurodevelopment.Citation119 Astrocytes can also produce the alarmin IL-33, which regulates microglial metabolism to facilitate synaptic pruning during development.Citation30,Citation120 In addition, astrocyte produced TNFa can mediate synaptic scaling in response to prolonged blockade of neuronal activityCitation121 by regulating neuronal glutamate and GABA receptor trafficking.Citation122 Whether this functional modulation of neuronal function by astrocytes is involved in the pathogenesis of neurodevelopmental disorders remains to be determined.
Consistent with the notion that multiple non-inflammatory neuroimmune activities of astrocytes might influence neurodevelopment, associational studies and animal models have now linked astrocyte dysfunction with several developmental disorders including intellectual disability,Citation7 autism,Citation123,Citation124 and schizophrenia.Citation125–127
Other immune cell types exist in the brain at extremely low levels. However, recent studies have found powerful influences of these cells on the functions of the brain. For example, antigen-reactive CD4+ T cells was found to support cognitive task performanceCitation28,Citation128 and meningeal γδ T cells can regulate anxiety-like behavior via its production of IL-17a.Citation129 The detailed mechanisms regarding how these T cell influences might impact neurodevelopment await further elucidation. Equally intriguing is the finding that mast cells in the developing preoptic area of the hypothalamus (POA) drive masculinization of the brain and inhibition of mast cells in the POA during the critical period for sexual differentiation which blunts neuronal and microglial changes in this region and adult sex behavior.Citation130 Most likely, these rare immune cells influence the developing brain by releasing neural active immune molecules at low levels that do not result in the frank inflammatory responses that they typically induce in the periphery.
There is also an expanding list of classical immune molecules that are now recognized to participate in the development of the nervous system. For example, chemokines that were traditionally known for their chemotactic activities in leukocytes were found in mothers’ milk to promote postnatal hippocampal neuronal proliferation in offspring,Citation33 and the critical antigen presentation molecule MHC was found to modulate synaptic plasticity during developmentCitation131,Citation132 and its CNS expression to be regulated by neuronal activity.Citation133 Furthermore, the complement system, which was known to opsonize and destroy invading pathogens or infected cells in an immune response, has now been established to play important roles in mediating synaptic pruning by glial cells.Citation34,Citation35,Citation91 Again, the complement system behaves differently in the non-inflammatory brain from its action in the periphery, which would cause chemotactic effects from activated complement fragments and attack a complement-marked whole cell rather than a small portion of a cell (synapse). These neuroimmune molecules have also been identified in human studies as risk factors for the pathogenesis of neurodevelopmental disorders such as schizophrenia and autism.Citation134,Citation135
Concluding Remarks
This mini-review is not meant to provide an exhaustive survey of all the neuroimmune elements involved in neurodevelopment. Rather, we wanted to sketch a broad outline of how this field has evolved and point out the important conceptual shift that is occurring in current literature. In contrast to the overt inflammatory activities in the brain, many non-inflammatory neuroimmune modulations of different aspects of neurodevelopment – from neuronal proliferation and migration to synaptogenesis and synaptic pruning – have been discovered (). Relevant references are also presented in for easy visualization. One emerging salient question is why do these non-inflammatory neuroimmune activities not induce full-blown inflammatory responses as they would in peripheral immune responses? There are several possibilities: (1) neuroimmune cells have different characteristics (eg, microglia are transcriptomically quite different from macrophagesCitation136) to their peripheral counterparts or reside outside of brain parenchyma (eg, T cells are mostly found in the glymphatic system in the meningesCitation137); (2) some of the collaborating immune system elements might be missing in the CNS parenchyma (eg, few professional antigen-presenting cells are found in the brain parenchyma, allowing MHC molecules to function outside the context of antigen presentationCitation138); and (3) alternative signaling systems might be deployed in the CNS (eg, alternatively spliced IL-1 receptor accessory protein is expressed in neurons, diverting IL-1 stimulated inflammatory gene expression to neuronal-specific activitiesCitation139). These possibilities suggest novel mechanisms to explain why neuroimmune activities in the developing brain may not simply cause traditional inflammatory responses but engender CNS-specific roles to influence neurodevelopment too.
Table 1 A Summary of Neurophysiological Functions and Neuroinflammatory Activities of Microglia and Astrocytes in Relation to Neurodevelopmental Diseases and Health
Figure 1 Schematic diagram showing neuroimmune activities involved in neurodevelopment. Microglia and astrocytes play a role in appropriate neurodevelopment and contribute to the pathophysiology of neurodevelopmental diseases. In frank inflammation, which is stimulated by perinatal immune activation, astrocytes and microglia are activated by immune stimulus. This activation leads to production of proinflammatory cytokines (TNFa, IL-1β) and damage to the blood–brain barrier. Infiltration of immune cells to the brain parenchyma together with astrocyte and microglial activation results in neuronal damage. Under physiological conditions, astrocytes and microglia play critical roles during neurodevelopment and homeostasis. Astrocyte- and microglia-driven cytokines regulate 5 critical processes of neurodevelopment that are crucial for developing a healthy brain: neuronal proliferation, synaptogenesis, synaptic pruning, neuronal migration, and neural circuitry regulation. Created with BioRender.com.
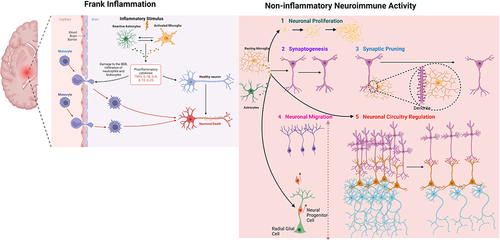
Disclosure
The authors report no conflicts of interest in this work.
Acknowledgments
This study was funded by the National Institute of Neurological Disorders and Stroke (grant R01-NS-116914 to NQ). The diagram was created with Biorender.com.
References
- Jiang X, Nardelli J. Cellular and molecular introduction to brain development. Neurobiol Dis. 2016;92(Pt A):3–17. doi:10.1016/j.nbd.2015.07.007
- Stouffer MA, Golden JA, Francis F. Neuronal migration disorders: focus on the cytoskeleton and epilepsy. Neurobiol Dis. 2016;92(Pt A):18–45. doi:10.1016/j.nbd.2015.08.003
- Yamaguchi Y, Miura M. Programmed cell death in neurodevelopment. Dev Cell. 2015;32(4):478–490. doi:10.1016/j.devcel.2015.01.019
- Batool S, Raza H, Zaidi J, Riaz S, Hasan S, Syed NI. Synapse formation: from cellular and molecular mechanisms to neurodevelopmental and neurodegenerative disorders. J Neurophysiol. 2019;121(4):1381–1397. doi:10.1152/jn.00833.2018
- de Moura AB, Abitante MS, Silva RH, Quevedo J, Reus GZ. Microglial activation in the neurodevelopment: a narrative review. Curr Mol Med. 2022;22(8):722–734. doi:10.2174/1566524021666211018112757
- Pierre WC, Smith PLP, Londono I, Chemtob S, Mallard C, Lodygensky GA. Neonatal microglia: the cornerstone of brain fate. Brain Behav Immun. 2017;59:333–345. doi:10.1016/j.bbi.2016.08.018
- Wang B, Zou L, Li M, Zhou L. Astrocyte: a foe or a friend in intellectual disability-related diseases. Front Synaptic Neurosci. 2022;14:877928. doi:10.3389/fnsyn.2022.877928
- McLaughlin KA, Sheridan MA, Lambert HK. Childhood adversity and neural development: deprivation and threat as distinct dimensions of early experience. Neurosci Biobehav Rev. 2014;47:578–591. doi:10.1016/j.neubiorev.2014.10.012
- Sheridan MA, McLaughlin KA. Dimensions of early experience and neural development: deprivation and threat. Trends Cogn Sci. 2014;18(11):580–585. doi:10.1016/j.tics.2014.09.001
- Kim J, Erice C, Rohlwink UK, Tucker EW. Infections in the developing brain: the role of the neuro-immune axis. Front Neurol. 2022;13:805786. doi:10.3389/fneur.2022.805786
- Sewell E, Roberts J, Mukhopadhyay S. Association of infection in neonates and long-term neurodevelopmental outcome. Clin Perinatol. 2021;48(2):251–261. doi:10.1016/j.clp.2021.03.001
- Salmaso N, Jablonska B, Scafidi J, Vaccarino FM, Gallo V. Neurobiology of premature brain injury. Nat Neurosci. 2014;17(3):341–346. doi:10.1038/nn.3604
- Jasthi D, Kollikonda S, Karnati S. Clinical course and long-term follow-up of a preterm infant with non-fatal respiratory distress syndrome due to heterozygous ABCA3 gene mutation: a case report and review of literature. J Neonatal Perinatal Med. 2022;15(3):653–658. doi:10.3233/NPM-210879
- Duffney LJ, Valdez P, Tremblay MW, et al. Epigenetics and autism spectrum disorder: a report of an autism case with mutation in H1 linker histone HIST1H1E and literature review. Am J Med Genet B Neuropsychiatr Genet. 2018;177(4):426–433. doi:10.1002/ajmg.b.32631
- Bourque DK, Hartley T, Nikkel SM, et al. A de novo mutation in RPL10 causes a rare X-linked ribosomopathy characterized by syndromic intellectual disability and epilepsy: a new case and review of the literature. Eur J Med Genet. 2018;61(2):89–93. doi:10.1016/j.ejmg.2017.10.011
- Sebat J, Levy DL, McCarthy SE. Rare structural variants in schizophrenia: one disorder, multiple mutations; one mutation, multiple disorders. Trends Genet. 2009;25(12):528–535. doi:10.1016/j.tig.2009.10.004
- Han VX, Patel S, Jones HF, Dale RC. Maternal immune activation and neuroinflammation in human neurodevelopmental disorders. Nat Rev Neurol. 2021;17(9):564–579. doi:10.1038/s41582-021-00530-8
- Carthy E, Ellender T. Histamine, neuroinflammation and neurodevelopment: a review. Front Neurosci. 2021;15:680214. doi:10.3389/fnins.2021.680214
- Savino R, Carotenuto M, Polito AN, et al. Analyzing the potential biological determinants of autism spectrum disorder: from neuroinflammation to the kynurenine pathway. Brain Sci. 2020;10(9):631. doi:10.3390/brainsci10090631
- Mattei D, Notter T. Basic concept of microglia biology and neuroinflammation in relation to psychiatry. Curr Top Behav Neurosci. 2020;44:9–34. doi:10.1007/7854_2018_83
- Bordeleau M, Fernandez de Cossio L, Chakravarty MM, Tremblay ME. From maternal diet to neurodevelopmental disorders: a story of neuroinflammation. Front Cell Neurosci. 2020;14:612705. doi:10.3389/fncel.2020.612705
- Bilbo SD, Schwarz JM. The immune system and developmental programming of brain and behavior. Front Neuroendocrinol. 2012;33(3):267–286. doi:10.1016/j.yfrne.2012.08.006
- Lammert CR, Frost EL, Bellinger CE, et al. AIM2 inflammasome surveillance of DNA damage shapes neurodevelopment. Nature. 2020;580(7805):647–652. doi:10.1038/s41586-020-2174-3
- Brien ME, Hughes K, Girard S. Prenatal administration of IL-1Ra attenuate the neurodevelopmental impacts following non-pathogenic inflammation during pregnancy. Sci Rep. 2021;11(1):23404. doi:10.1038/s41598-021-02927-3
- Jiang NM, Cowan M, Moonah SN, Petri WA. The impact of systemic inflammation on neurodevelopment. Trends Mol Med. 2018;24(9):794–804. doi:10.1016/j.molmed.2018.06.008
- Allswede DM, Cannon TD. Prenatal inflammation and risk for schizophrenia: a role for immune proteins in neurodevelopment. Dev Psychopathol. 2018;30(3):1157–1178. doi:10.1017/S0954579418000317
- Campbell IL, Hofer MJ, Pagenstecher A. Transgenic models for cytokine-induced neurological disease. Biochim Biophys Acta. 2010;1802(10):903–917. doi:10.1016/j.bbadis.2009.10.004
- Radjavi A, Smirnov I, Kipnis J. Brain antigen-reactive CD4+ T cells are sufficient to support learning behavior in mice with limited T cell repertoire. Brain Behav Immun. 2014;35:58–63. doi:10.1016/j.bbi.2013.08.013
- Nguyen PT, Dorman LC, Pan S, et al. Microglial remodeling of the extracellular matrix promotes synapse plasticity. Cell. 2020;182(2):388–403 e15. doi:10.1016/j.cell.2020.05.050
- Vainchtein ID, Chin G, Cho FS, et al. Astrocyte-derived interleukin-33 promotes microglial synapse engulfment and neural circuit development. Science. 2018;359(6381):1269–1273. doi:10.1126/science.aal3589
- Ribeiro M, Brigas HC, Temido-Ferreira M, et al. Meningeal gammadelta T cell-derived IL-17 controls synaptic plasticity and short-term memory. Sci Immunol. 2019;4(40):eaay5199. doi:10.1126/sciimmunol.aay5199
- Biber K, Zuurman MW, Dijkstra IM, Boddeke HW. Chemokines in the brain: neuroimmunology and beyond. Curr Opin Pharmacol. 2002;2(1):63–68. doi:10.1016/S1471-4892(01)00122-9
- Liu B, Zupan B, Laird E, et al. Maternal hematopoietic TNF, via milk chemokines, programs hippocampal development and memory. Nat Neurosci. 2014;17(1):97–105. doi:10.1038/nn.3596
- Chen Y, Chu JMT, Chang RCC, Wong GTC. The complement system in the central nervous system: from neurodevelopment to neurodegeneration. Biomolecules. 2022;12(2):337.
- Coulthard LG, Hawksworth OA, Woodruff TM. Complement: the emerging architect of the developing brain. Trends Neurosci. 2018;41(6):373–384. doi:10.1016/j.tins.2018.03.009
- Stefano GB. Historical insight into infections and disorders associated with neurological and psychiatric sequelae similar to long COVID. Med Sci Monit. 2021;27:e931447. doi:10.12659/MSM.931447
- Tucci V, Moukaddam N, Meadows J, Shah S, Galwankar SC, Kapur GB. The forgotten plague: psychiatric manifestations of Ebola, Zika, and emerging infectious diseases. J Glob Infect Dis. 2017;9(4):151–156. doi:10.4103/jgid.jgid_66_17
- Zhao YJ, Jin Y, Rao WW, et al. The prevalence of psychiatric comorbidities during the SARS and COVID-19 epidemics: a systematic review and meta-analysis of observational studies. J Affect Disord. 2021;287:145–157. doi:10.1016/j.jad.2021.03.016
- Wedderburn CJ, Weldon E, Bertran-Cobo C, et al. Early neurodevelopment of HIV-exposed uninfected children in the era of antiretroviral therapy: a systematic review and meta-analysis. Lancet Child Adolesc Health. 2022;6(6):393–408. doi:10.1016/S2352-4642(22)00071-2
- Wedderburn CJ, Groenewold NA, Roos A, et al. Early structural brain development in infants exposed to HIV and antiretroviral therapy in utero in a South African birth cohort. J Int AIDS Soc. 2022;25(1):e25863. doi:10.1002/jia2.25863
- O’Connor TG, Ciesla AA, Sefair AV, et al. Maternal prenatal infection and anxiety predict neurodevelopmental outcomes in middle childhood. J Psychopathol Clin Sci. 2022;131(4):422–434. doi:10.1037/abn0000746
- Nudel R, Thompson WK, Borglum AD, et al. Maternal pregnancy-related infections and autism spectrum disorder-the genetic perspective. Transl Psychiatry. 2022;12(1):334. doi:10.1038/s41398-022-02068-9
- Sevenoaks T, Wedderburn CJ, Donald KA, et al. Association of maternal and infant inflammation with neurodevelopment in HIV-exposed uninfected children in a South African birth cohort. Brain Behav Immun. 2021;91:65–73. doi:10.1016/j.bbi.2020.08.021
- Hall HA, Speyer LG, Murray AL, Auyeung B. Prenatal maternal infections and children’s socioemotional development: findings from the UK Millennium Cohort Study. Eur Child Adolesc Psychiatry. 2021;30(10):1641–1650. doi:10.1007/s00787-020-01644-y
- Harden LM, Leahy S, Lala SG, et al. South African children: a matched cohort study of neurodevelopmental impairment in survivors of invasive Group B Streptococcus disease aged 5 to 8 years. Clin Infect Dis. 2022;74(Suppl_1):S5–S13. doi:10.1093/cid/ciab814
- Kalish BT, Kim E, Finander B, et al. Maternal immune activation in mice disrupts proteostasis in the fetal brain. Nat Neurosci. 2021;24(2):204–213. doi:10.1038/s41593-020-00762-9
- Vigli D, Palombelli G, Fanelli S, et al. Maternal immune activation in mice only partially recapitulates the autism spectrum disorders symptomatology. Neuroscience. 2020;445:109–119. doi:10.1016/j.neuroscience.2020.05.009
- Talukdar PM, Abdul F, Maes M, et al. Maternal immune activation causes schizophrenia-like behaviors in the offspring through activation of immune-inflammatory, oxidative and apoptotic pathways, and lowered antioxidant defenses and neuroprotection. Mol Neurobiol. 2020;57(10):4345–4361. doi:10.1007/s12035-020-02028-8
- Shin Yim Y, Park A, Berrios J, et al. Reversing behavioural abnormalities in mice exposed to maternal inflammation. Nature. 2017;549(7673):482–487. doi:10.1038/nature23909
- Oskvig DB, Elkahloun AG, Johnson KR, Phillips TM, Herkenham M. Maternal immune activation by LPS selectively alters specific gene expression profiles of interneuron migration and oxidative stress in the fetus without triggering a fetal immune response. Brain Behav Immun. 2012;26(4):623–634. doi:10.1016/j.bbi.2012.01.015
- Arrode-Bruses G, Bruses JL. Maternal immune activation by poly I:C induces expression of cytokines IL-1beta and IL-13, chemokine MCP-1 and colony stimulating factor VEGF in fetal mouse brain. J Neuroinflammation. 2012;9:83. doi:10.1186/1742-2094-9-83
- Boulanger-Bertolus J, Pancaro C, Mashour GA. Increasing role of maternal immune activation in neurodevelopmental disorders. Front Behav Neurosci. 2018;12:230. doi:10.3389/fnbeh.2018.00230
- Ratnayake U, Quinn T, Walker DW, Dickinson H. Cytokines and the neurodevelopmental basis of mental illness. Front Neurosci. 2013;7:180. doi:10.3389/fnins.2013.00180
- Kelly SB, Stojanovska V, Zahra VA, et al. Interleukin-1 blockade attenuates white matter inflammation and oligodendrocyte loss after progressive systemic lipopolysaccharide exposure in near-term fetal sheep. J Neuroinflammation. 2021;18(1):189. doi:10.1186/s12974-021-02238-4
- Hsieh CT, Lee YJ, Lee JW, et al. Interleukin-1 receptor antagonist ameliorates the pain hypersensitivity, spinal inflammation and oxidative stress induced by systemic lipopolysaccharide in neonatal rats. Neurochem Int. 2020;135:104686. doi:10.1016/j.neuint.2020.104686
- Semple BD, O’Brien TJ, Gimlin K, et al. Interleukin-1 receptor in seizure susceptibility after traumatic injury to the pediatric brain. J Neurosci. 2017;37(33):7864–7877. doi:10.1523/JNEUROSCI.0982-17.2017
- Pang Y, Tien LT, Zhu H, et al. Interleukin-1 receptor antagonist reduces neonatal lipopolysaccharide-induced long-lasting neurobehavioral deficits and dopaminergic neuronal injury in adult rats. Int J Mol Sci. 2015;16(4):8635–8654. doi:10.3390/ijms16048635
- Liu X, Nemeth DP, McKim DB, et al. Cell-type-specific interleukin 1 receptor 1 signaling in the brain regulates distinct neuroimmune activities. Immunity. 2019;50(3):764–766. doi:10.1016/j.immuni.2019.02.012
- Meng S, Chanda P, Thandavarayan RA, Cooke JP. Transflammation: how innate immune activation and free radicals drive nuclear reprogramming. Antioxid Redox Signal. 2018;29(2):205–218. doi:10.1089/ars.2017.7364
- Ye L, Huang Y, Zhao L, et al. IL-1beta and TNF-alpha induce neurotoxicity through glutamate production: a potential role for neuronal glutaminase. J Neurochem. 2013;125(6):897–908. doi:10.1111/jnc.12263
- Westmoreland SV, Kolson D, Gonzalez-Scarano F. Toxicity of TNF alpha and platelet activating factor for human NT2N neurons: a tissue culture model for human immunodeficiency virus dementia. J Neurovirol. 1996;2(2):118–126. doi:10.3109/13550289609146545
- Thornton P, Pinteaux E, Allan SM, Rothwell NJ. Matrix metalloproteinase-9 and urokinase plasminogen activator mediate interleukin-1-induced neurotoxicity. Mol Cell Neurosci. 2008;37(1):135–142. doi:10.1016/j.mcn.2007.09.002
- Piani D, Spranger M, Frei K, Schaffner A, Fontana A. Macrophage-induced cytotoxicity of N-methyl-D-aspartate receptor positive neurons involves excitatory amino acids rather than reactive oxygen intermediates and cytokines. Eur J Immunol. 1992;22(9):2429–2436. doi:10.1002/eji.1830220936
- Allen C, Thornton P, Denes A, et al. Neutrophil cerebrovascular transmigration triggers rapid neurotoxicity through release of proteases associated with decondensed DNA. J Immunol. 2012;189(1):381–392. doi:10.4049/jimmunol.1200409
- Pang Y, Fan LW, Zheng B, Cai Z, Rhodes PG. Role of interleukin-6 in lipopolysaccharide-induced brain injury and behavioral dysfunction in neonatal rats. Neuroscience. 2006;141(2):745–755. doi:10.1016/j.neuroscience.2006.04.007
- Saberi H, Tanha RR, Derakhshanrad N, Soltaninejad MJ. Acute presentation of third ventricular cavernous malformation following COVID-19 infection in a pregnant woman: a case report. Neurochirurgie. 2022;68(2):228–231. doi:10.1016/j.neuchi.2021.03.010
- Juca E, Pessoa A, Ribeiro E, et al. Hydrocephalus associated to congenital Zika syndrome: does shunting improve clinical features? Childs Nerv Syst. 2018;34(1):101–106. doi:10.1007/s00381-017-3636-2
- Piontkewitz Y, Arad M, Weiner I. Abnormal trajectories of neurodevelopment and behavior following in utero insult in the rat. Biol Psychiatry. 2011;70(9):842–851. doi:10.1016/j.biopsych.2011.06.007
- Arad M, Piontkewitz Y, Albelda N, Shaashua L, Weiner I. Immune activation in lactating dams alters sucklings’ brain cytokines and produces non-overlapping behavioral deficits in adult female and male offspring: a novel neurodevelopmental model of sex-specific psychopathology. Brain Behav Immun. 2017;63:35–49. doi:10.1016/j.bbi.2017.01.015
- Takei N, Lewis S, Jones P, Harvey I, Murray RM. Prenatal exposure to influenza and increased cerebrospinal fluid spaces in schizophrenia. Schizophr Bull. 1996;22(3):521–534. doi:10.1093/schbul/22.3.521
- Lim KO, Beal DM, Harvey RL Jr, et al. Brain dysmorphology in adults with congenital rubella plus schizophrenialike symptoms. Biol Psychiatry. 1995;37(11):764–776. doi:10.1016/0006-3223(94)00219-S
- Castillo-Gomez E, Perez-Rando M, Belles M, et al. Early social isolation stress and perinatal NMDA receptor antagonist treatment induce changes in the structure and neurochemistry of inhibitory neurons of the adult amygdala and prefrontal cortex. eNeuro. 2017;4(2):ENEURO.0034–17.2017. doi:10.1523/ENEURO.0034-17.2017
- Chen HR, Chen CW, Mandhani N, et al. Monocytic infiltrates contribute to autistic-like behaviors in a two-hit model of neurodevelopmental defects. J Neurosci. 2020;40(49):9386–9400. doi:10.1523/JNEUROSCI.1171-20.2020
- Servetti M, Pisciotta L, Tassano E, et al. Neurodevelopmental disorders in patients with complex phenotypes and potential complex genetic basis involving non-coding genes, and double CNVs. Front Genet. 2021;12:732002. doi:10.3389/fgene.2021.732002
- Fraguas D, Diaz-Caneja CM, Corripio I, et al. Gene-environment interaction as a predictor of early adjustment in first episode psychosis. Schizophr Res. 2017;189:196–203. doi:10.1016/j.schres.2017.02.021
- Grova N, Schroeder H, Olivier JL, Turner JD. Epigenetic and neurological impairments associated with early life exposure to persistent organic pollutants. Int J Genomics. 2019;2019:2085496. doi:10.1155/2019/2085496
- Simpson E. A historical perspective on immunological privilege. Immunol Rev. 2006;213:12–22. doi:10.1111/j.1600-065X.2006.00434.x
- Quan N, Banks WA. Brain-immune communication pathways. Brain Behav Immun. 2007;21(6):727–735. doi:10.1016/j.bbi.2007.05.005
- Niederkorn JY, Stein-Streilein J. History and physiology of immune privilege. Ocul Immunol Inflamm. 2010;18(1):19–23. doi:10.3109/09273940903564766
- Ginhoux F, Greter M, Leboeuf M, et al. Fate mapping analysis reveals that adult microglia derive from primitive macrophages. Science. 2010;330(6005):841–845.
- Yan P, Kim KW, Xiao Q, et al. Peripheral monocyte-derived cells counter amyloid plaque pathogenesis in a mouse model of Alzheimer’s disease. J Clin Invest. 2022;132(11). doi:10.1172/JCI152565
- Chen HR, Chen CW, Kuo YM, et al. Monocytes promote acute neuroinflammation and become pathological microglia in neonatal hypoxic-ischemic brain injury. Theranostics. 2022;12(2):512–529. doi:10.7150/thno.64033
- McKim DB, Weber MD, Niraula A, et al. Microglial recruitment of IL-1beta-producing monocytes to brain endothelium causes stress-induced anxiety. Mol Psychiatry. 2018;23(6):1421–1431. doi:10.1038/mp.2017.64
- Zhu L, Liu X, Nemeth DP, et al. Interleukin-1 causes CNS inflammatory cytokine expression via endothelia-microglia bi-cellular signaling. Brain Behav Immun. 2019;81:292–304. doi:10.1016/j.bbi.2019.06.026
- Nemeth DP, Liu X, McKim DB, et al. Dynamic interleukin-1 receptor type 1 signaling mediates microglia-vasculature interactions following repeated systemic LPS. J Inflamm Res. 2022;15:1575–1590. doi:10.2147/JIR.S350114
- Nimmerjahn A, Kirchhoff F, Helmchen F. Resting microglial cells are highly dynamic surveillants of brain parenchyma in vivo. Science. 2005;308(5726):1314–1318. doi:10.1126/science.1110647
- Sokolowski JD, Chabanon-Hicks CN, Han CZ, Heffron DS, Mandell JW. Fractalkine is a “find-me” signal released by neurons undergoing ethanol-induced apoptosis. Front Cell Neurosci. 2014;8:360. doi:10.3389/fncel.2014.00360
- Thion MS, Ginhoux F, Garel S. Microglia and early brain development: an intimate journey. Science. 2018;362(6411):185–189. doi:10.1126/science.aat0474
- Diaz-Aparicio I, Paris I, Sierra-Torre V, et al. Microglia actively remodel adult hippocampal neurogenesis through the phagocytosis secretome. J Neurosci. 2020;40(7):1453–1482. doi:10.1523/JNEUROSCI.0993-19.2019
- Parellada E, Gasso P. Glutamate and microglia activation as a driver of dendritic apoptosis: a core pathophysiological mechanism to understand schizophrenia. Transl Psychiatry. 2021;11(1):271. doi:10.1038/s41398-021-01385-9
- Schafer DP, Lehrman EK, Kautzman AG, et al. Microglia sculpt postnatal neural circuits in an activity and complement-dependent manner. Neuron. 2012;74(4):691–705. doi:10.1016/j.neuron.2012.03.026
- Hong S, Dissing-Olesen L, Stevens B. New insights on the role of microglia in synaptic pruning in health and disease. Curr Opin Neurobiol. 2016;36:128–134. doi:10.1016/j.conb.2015.12.004
- Scott-Hewitt N, Perrucci F, Morini R, et al. Local externalization of phosphatidylserine mediates developmental synaptic pruning by microglia. EMBO J. 2020;39(16):e105380.
- Lehrman EK, Wilton DK, Litvina EY, et al. CD47 protects synapses from excess microglia-mediated pruning during development. Neuron. 2018;100(1):120–34 e6. doi:10.1016/j.neuron.2018.09.017
- Paolicelli RC, Gross CT. Microglia in development: linking brain wiring to brain environment. Neuron Glia Biol. 2011;7(1):77–83. doi:10.1017/S1740925X12000105
- Paolicelli RC, Bolasco G, Pagani F, et al. Synaptic pruning by microglia is necessary for normal brain development. Science. 2011;333(6048):1456–1458. doi:10.1126/science.1202529
- Zhan Y, Paolicelli RC, Sforazzini F, et al. Deficient neuron-microglia signaling results in impaired functional brain connectivity and social behavior. Nat Neurosci. 2014;17(3):400–406. doi:10.1038/nn.3641
- Weinhard L, Di Bartolomei G, Bolasco G, et al. Microglia remodel synapses by presynaptic trogocytosis and spine head filopodia induction. Nat Commun. 2018;9(1):1228. doi:10.1038/s41467-018-03566-5
- Chen Z, Jalabi W, Hu W, et al. Microglial displacement of inhibitory synapses provides neuroprotection in the adult brain. Nat Commun. 2014;5:4486. doi:10.1038/ncomms5486
- Wan Y, Feng B, You Y, et al. Microglial displacement of GABAergic synapses is a protective event during complex febrile seizures. Cell Rep. 2020;33(5):108346. doi:10.1016/j.celrep.2020.108346
- Parkhurst CN, Yang G, Ninan I, et al. Microglia promote learning-dependent synapse formation through brain-derived neurotrophic factor. Cell. 2013;155(7):1596–1609. doi:10.1016/j.cell.2013.11.030
- Aarum J, Sandberg K, Haeberlein SL, Persson MA. Migration and differentiation of neural precursor cells can be directed by microglia. Proc Natl Acad Sci U S A. 2003;100(26):15983–15988. doi:10.1073/pnas.2237050100
- Lebovitz Y, Kowalski EA, Wang X, et al. Lactobacillus rescues postnatal neurobehavioral and microglial dysfunction in a model of maternal microbiome dysbiosis. Brain Behav Immun. 2019;81:617–629. doi:10.1016/j.bbi.2019.07.025
- Han RT, Kim RD, Molofsky AV, Liddelow SA. Astrocyte-immune cell interactions in physiology and pathology. Immunity. 2021;54(2):211–224. doi:10.1016/j.immuni.2021.01.013
- Todd L, Palazzo I, Suarez L, et al. Reactive microglia and IL1beta/IL-1R1-signaling mediate neuroprotection in excitotoxin-damaged mouse retina. J Neuroinflammation. 2019;16(1):118. doi:10.1186/s12974-019-1505-5
- Aronica E, Ravizza T, Zurolo E, Vezzani A. Astrocyte immune responses in epilepsy. Glia. 2012;60(8):1258–1268. doi:10.1002/glia.22312
- Hart CG, Karimi-Abdolrezaee S. Recent insights on astrocyte mechanisms in CNS homeostasis, pathology, and repair. J Neurosci Res. 2021;99(10):2427–2462. doi:10.1002/jnr.24922
- Pfrieger FW, Barres BA. Synaptic efficacy enhanced by glial cells in vitro. Science. 1997;277(5332):1684–1687. doi:10.1126/science.277.5332.1684
- Christopherson KS, Ullian EM, Stokes CC, et al. Thrombospondins are astrocyte-secreted proteins that promote CNS synaptogenesis. Cell. 2005;120(3):421–433. doi:10.1016/j.cell.2004.12.020
- Kucukdereli H, Allen NJ, Lee AT, et al. Control of excitatory CNS synaptogenesis by astrocyte-secreted proteins Hevin and SPARC. Proc Natl Acad Sci U S A. 2011;108(32):E440–E449. doi:10.1073/pnas.1104977108
- Tabary M, Gheware A, Penaloza HF, Lee JS. The matricellular protein thrombospondin-1 in lung inflammation and injury. Am J Physiol Cell Physiol. 2022;323(3):C857–C865. doi:10.1152/ajpcell.00182.2022
- Sullivan MM, Sage EH. Hevin/SC1, a matricellular glycoprotein and potential tumor-suppressor of the SPARC/BM-40/Osteonectin family. Int J Biochem Cell Biol. 2004;36(6):991–996. doi:10.1016/j.biocel.2004.01.017
- Cahoy JD, Emery B, Kaushal A, et al. A transcriptome database for astrocytes, neurons, and oligodendrocytes: a new resource for understanding brain development and function. J Neurosci. 2008;28(1):264–278. doi:10.1523/JNEUROSCI.4178-07.2008
- Chung WS, Clarke LE, Wang GX, et al. Astrocytes mediate synapse elimination through MEGF10 and MERTK pathways. Nature. 2013;504(7480):394–400. doi:10.1038/nature12776
- Iram T, Ramirez-Ortiz Z, Byrne MH, et al. Megf10 is a receptor for C1Q that mediates clearance of apoptotic cells by astrocytes. J Neurosci. 2016;36(19):5185–5192. doi:10.1523/JNEUROSCI.3850-15.2016
- Morizawa YM, Hirayama Y, Ohno N, et al. Reactive astrocytes function as phagocytes after brain ischemia via ABCA1-mediated pathway. Nat Commun. 2017;8(1):28. doi:10.1038/s41467-017-00037-1
- Diniz LP, Matias I, Siqueira M, Stipursky J, Gomes FCA. Astrocytes and the TGF-beta 1 pathway in the healthy and diseased brain: a double-edged sword. Mol Neurobiol. 2019;56(7):4653–4679. doi:10.1007/s12035-018-1396-y
- Diniz LP, Tortelli V, Garcia MN, et al. Astrocyte transforming growth factor beta 1 promotes inhibitory synapse formation via CaM kinase II signaling. Glia. 2014;62(12):1917–1931. doi:10.1002/glia.22713
- Xie L, Zhang N, Zhang Q, et al. Inflammatory factors and amyloid beta-induced microglial polarization promote inflammatory crosstalk with astrocytes. Aging. 2020;12(22):22538–22549. doi:10.18632/aging.103663
- He D, Xu H, Zhang H, et al. Disruption of the IL-33-ST2-AKT signaling axis impairs neurodevelopment by inhibiting microglial metabolic adaptation and phagocytic function. Immunity. 2022;55(1):159–73 e9. doi:10.1016/j.immuni.2021.12.001
- Stellwagen D, Malenka RC. Synaptic scaling mediated by glial TNF-alpha. Nature. 2006;440(7087):1054–1059. doi:10.1038/nature04671
- Konefal SC, Stellwagen D. Tumour necrosis factor-mediated homeostatic synaptic plasticity in behavioural models: testing a role in maternal immune activation. Philos Trans R Soc Lond B Biol Sci. 2017;372(1715). doi:10.1098/rstb.2016.0160
- Li J, Rubini P, Tang Y, Illes P. Astrocyte-derived ATP: a new etiological factor for autism spectrum disorder. Neurosci Bull. 2022;38(1):104–106. doi:10.1007/s12264-021-00788-4
- Petrelli F, Bezzi P. mGlu5-mediated signalling in developing astrocyte and the pathogenesis of autism spectrum disorders. Curr Opin Neurobiol. 2018;48:139–145. doi:10.1016/j.conb.2017.12.014
- Wei Y, Xiao L, Fan W, et al. Astrocyte activation, but not microglia, is associated with the experimental mouse model of schizophrenia induced by chronic ketamine. J Mol Neurosci. 2022;72(9):1902–1915. doi:10.1007/s12031-022-02046-2
- Gravina A, Wilson JL, Bacciu D, Grimes KJ, Priami C. Controlling astrocyte-mediated synaptic pruning signals for schizophrenia drug repurposing with deep graph networks. PLoS Comput Biol. 2022;18(5):e1009531. doi:10.1371/journal.pcbi.1009531
- de Oliveira Figueiredo EC, Cali C, Petrelli F, Bezzi P. Emerging evidence for astrocyte dysfunction in schizophrenia. Glia. 2022;70(9):1585–1604. doi:10.1002/glia.24221
- Song EJ, Jeon SG, Kim KA, Kim JI, Moon M. Restricted CD4+ T cell receptor repertoire impairs cognitive function via alteration of Th2 cytokine levels. Neurogenesis. 2017;4(1):e1256856. doi:10.1080/23262133.2016.1256856
- Alves de lima K, Rustenhoven J, Da Mesquita S, et al. Meningeal gammadelta T cells regulate anxiety-like behavior via IL-17a signaling in neurons. Nat Immunol. 2020;21(11):1421–1429. doi:10.1038/s41590-020-0776-4
- Lenz KM, Pickett LA, Wright CL, Davis KT, Joshi A, McCarthy MM. Mast cells in the developing brain determine adult sexual behavior. J Neurosci. 2018;38(37):8044–8059. doi:10.1523/JNEUROSCI.1176-18.2018
- Shatz CJ. MHC class I: an unexpected role in neuronal plasticity. Neuron. 2009;64(1):40–45. doi:10.1016/j.neuron.2009.09.044
- Goddard CA, Butts DA, Shatz CJ. Regulation of CNS synapses by neuronal MHC class I. Proc Natl Acad Sci U S A. 2007;104(16):6828–6833. doi:10.1073/pnas.0702023104
- Lv D, Shen Y, Peng Y, Liu J, Miao F, Zhang J. Neuronal MHC Class I expression is regulated by activity driven calcium signaling. PLoS One. 2015;10(8):e0135223. doi:10.1371/journal.pone.0135223
- Mou TM, Lane MV, Ireland DDC, Verthelyi D, Tonelli LH, Clark SM. Association of complement component 4 with neuroimmune abnormalities in the subventricular zone in schizophrenia and autism spectrum disorders. Neurobiol Dis. 2022;173:105840. doi:10.1016/j.nbd.2022.105840
- Bergen SE, O’Dushlaine CT, Ripke S, et al. Genome-wide association study in a Swedish population yields support for greater CNV and MHC involvement in schizophrenia compared with bipolar disorder. Mol Psychiatry. 2012;17(9):880–886. doi:10.1038/mp.2012.73
- Hammond TR, Dufort C, Dissing-Olesen L, et al. Single-cell RNA sequencing of microglia throughout the mouse lifespan and in the injured brain reveals complex cell-state changes. Immunity. 2019;50(1):253–71 e6. doi:10.1016/j.immuni.2018.11.004
- Louveau A, Smirnov I, Keyes TJ, et al. Structural and functional features of central nervous system lymphatic vessels. Nature. 2015;523(7560):337–341. doi:10.1038/nature14432
- Constant O, Maarifi G, Blanchet FP, Van de Perre P, Simonin Y, Salinas S. Role of dendritic cells in viral brain infections. Front Immunol. 2022;13:862053. doi:10.3389/fimmu.2022.862053
- Smith DE, Lipsky BP, Russell C, et al. A central nervous system-restricted isoform of the interleukin-1 receptor accessory protein modulates neuronal responses to interleukin-1. Immunity. 2009;30(6):817–831. doi:10.1016/j.immuni.2009.03.020